Abstract
1. Mag-fura-2 fluorescence microscopy and whole-cell patch-clamp recordings were used to measure glutamate-induced changes in the intracellular free Mg2+ concentration ([Mg2+]i) and Mg2+ currents, respectively, in cultured forebrain neurones from fetal rats in the absence of extracellular Na+ (Nao+) and Ca2+ (Cao2+). 2. Increasing the extracellular Mg2+ concentration ([Mg2+]o) from 9 to 70 mM significantly enhanced the maximum [Mg2+]i induced by a 5 min 100 microM glutamate plus 1 microM glycine stimulation ([Mg2+]i,5 min) from 2.04 +/- 0.07 to 2.98 +/- 0.20 mM. Increasing [Mg2+]o from 9 to 70 mM also significantly enhanced the initial rate of rise in [Mg2+]i upon glutamate stimulation from 0.41 +/- 0.02 to 0.81 +/- 0.08 mM min-1. 3. The glutamate-stimulated increase in [Mg2+]i was not altered by prior depletion of intracellular free Na+ (Nai+). For paired stimulations in single neurones, the mean [Mg2+]i,5 min was 1.95 +/- 0.17 mM under Na(+)-depleted conditions and 1.94 +/- 0.16 mM under control conditions. 4. The glutamate-stimulated increase in [Mg2+]i was significantly reduced when NMDA channel-permeant Cs+ or K+ ions were used as the Na+ substitute instead of the presumably NMDA channel-impermeant ions N-methyl-D-glucamine (NMDG), Tris or sucrose. The mean [Mg2+]i,5 min was 0.56 +/- 0.06 and 0.74 +/- 0.08 mM in the presence of Cs+ or K+, respectively, compared with 2.13 +/- 0.10, 1.93 +/- 0.11 and 2.07 +/- 0.22 mM in the presence of NMDG, Tris or sucrose, respectively. 5. In whole-cell recordings performed with Cs+ as the primary intracellular cation, application of 100 microM NMDA plus 10 microM glycine induced inward currents that reversed around -55 mV in an extracellular solution containing 70 mM Mg2+ and 31 mM NMDG as the only cations. The currents were reversibly inhibited by DL-2-amino-5-phosphonovaleric acid (APV). In an extracellular solution containing 2 mM Mg2+ and 140 mM NMDG, NMDA plus glycine activated outward currents at potentials more depolarized than -90 mV. 6. In whole-cell recordings made with NMDG as the principal cation in the patch pipette, application of NMDA plus glycine in the 70 mM Mg2+ extracellular solution induced inward currents at voltages more negative than +15 mV. The ratio of the current measured under these conditions to the current measured in an extracellular solution containing Na+ as the principal cation (0.073:1) was nearly constant from cell to cell. 7. Following a 5 min glutamate stimulation in the presence of 9 mM Mg2+, [Mg2+]i returned to basal levels at a mean rate of 58.1 +/- 2.1 microM min-1. Complete removal of Nao+ significantly inhibited the rate of recovery to 31% of control. Raising [Mg2+]o to 30 mM in combination with removal of Nao+ did not inhibit recovery significantly more than either manipulation alone (28% of control). 8. These results suggest that glutamate-stimulated increases in [Mg2+]i that occur in the absence of Nao+ and Cao2+ result from Mg2+ entry through NMDA-activated ion channels. Furthermore, recovery from a glutamate-induced Mg2+ load appears to be primarily due to Mg2+ efflux via a mechanism whose characteristics are consistent with Na(+)-Mg2+ exchange.
Full text
PDF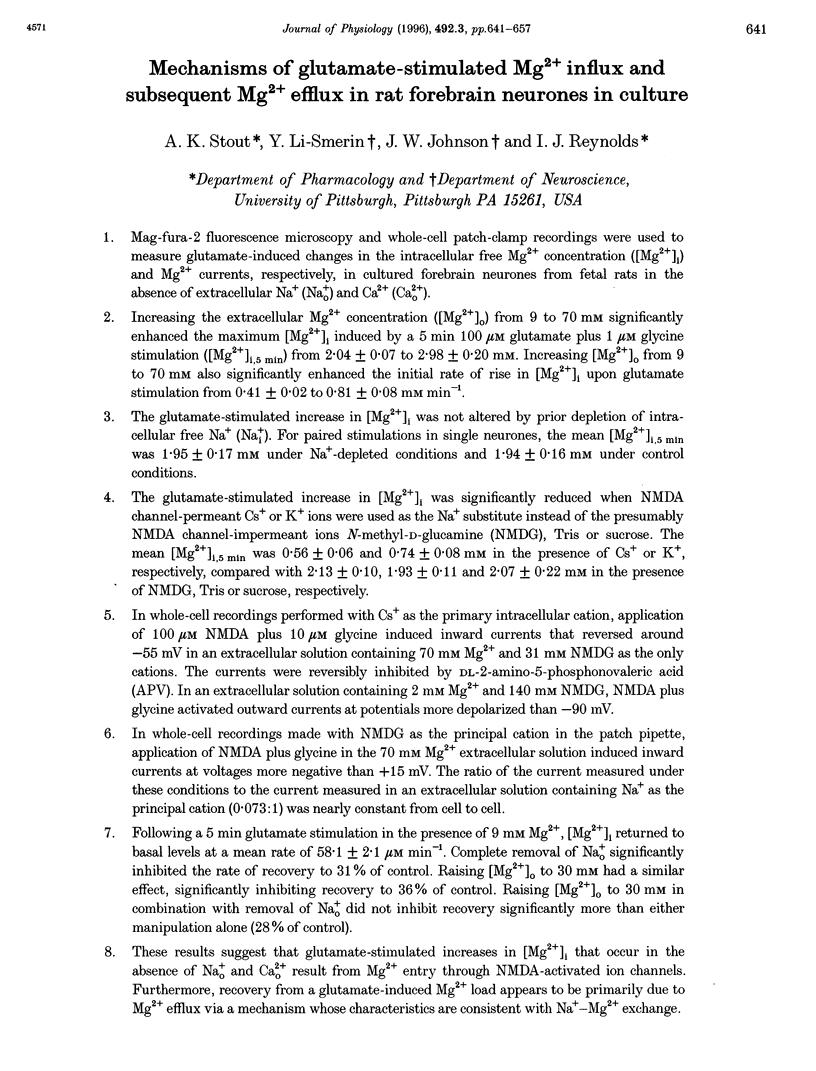
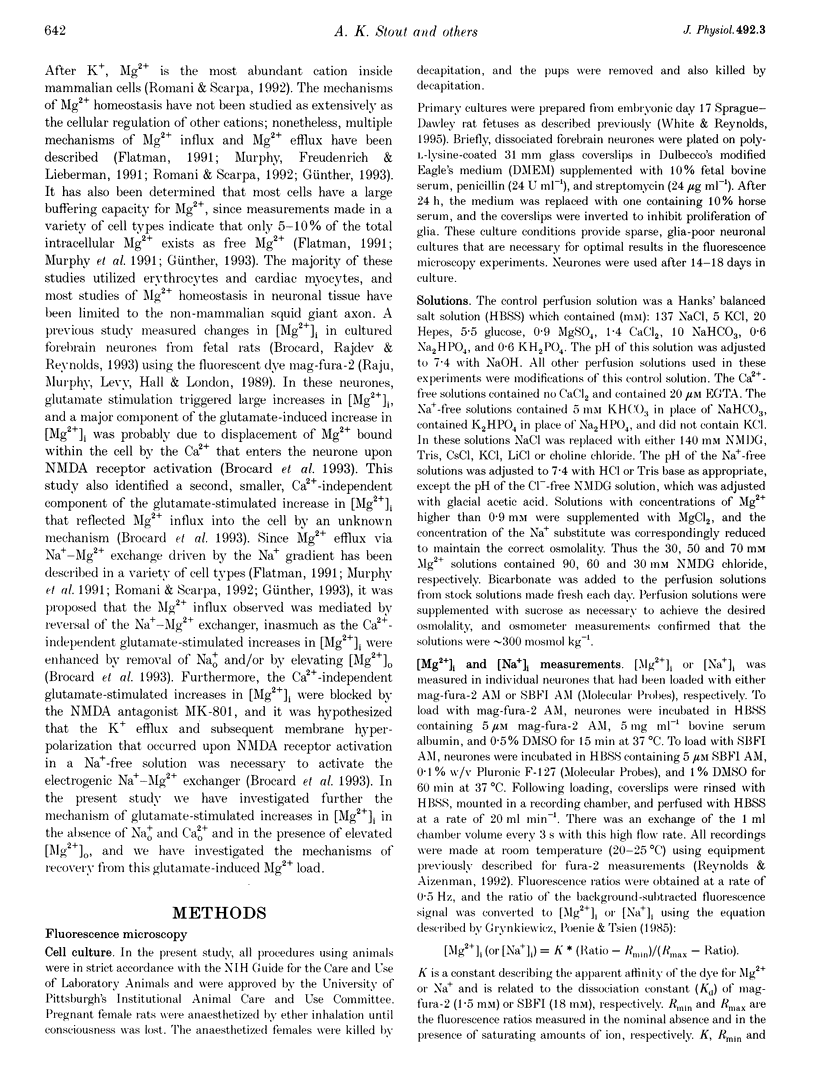
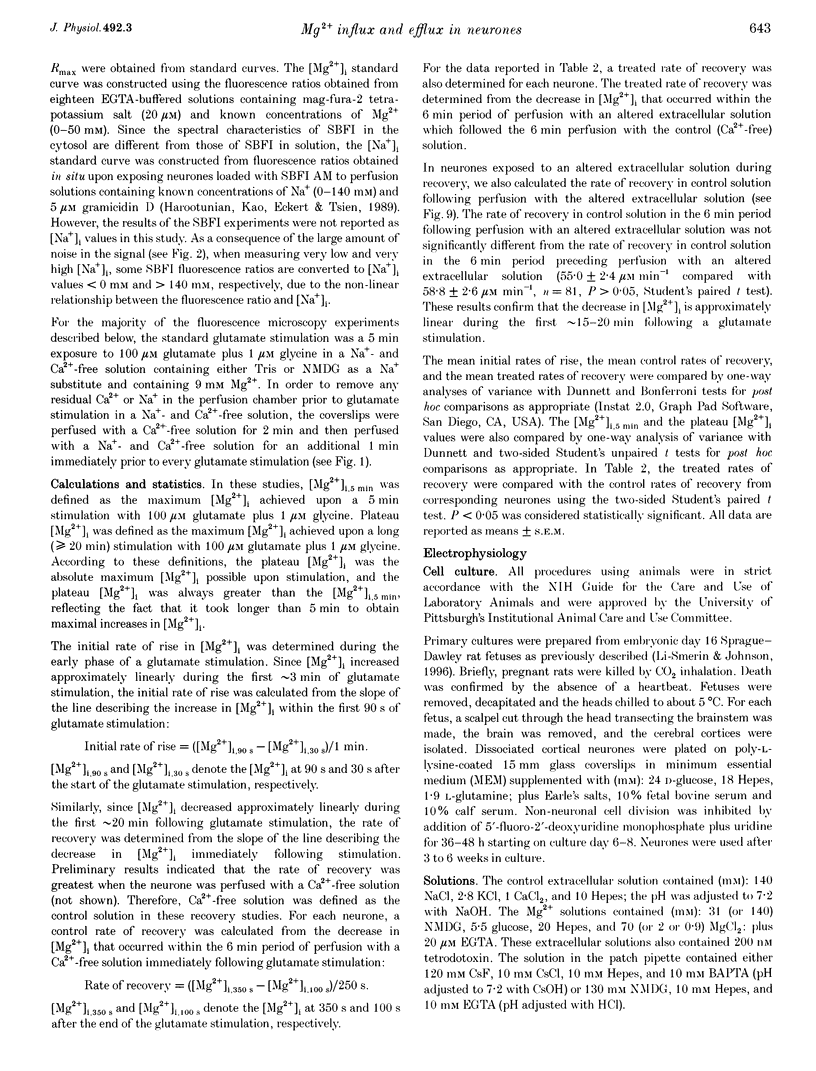
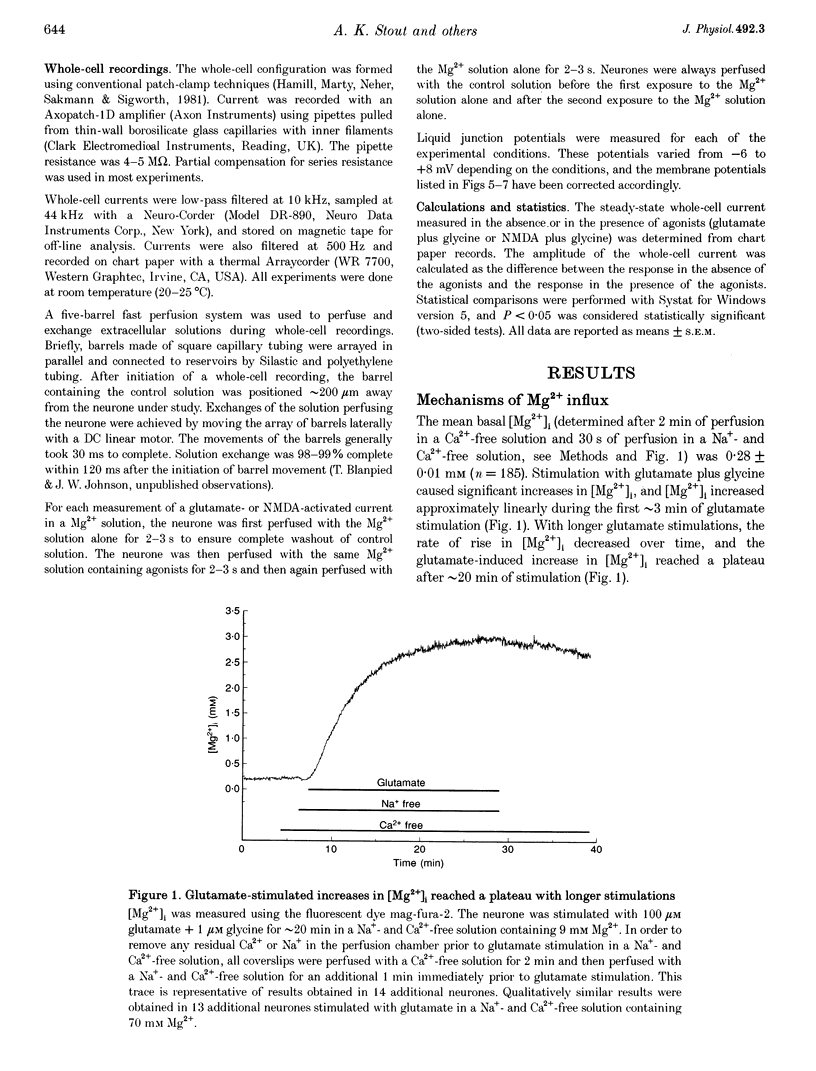
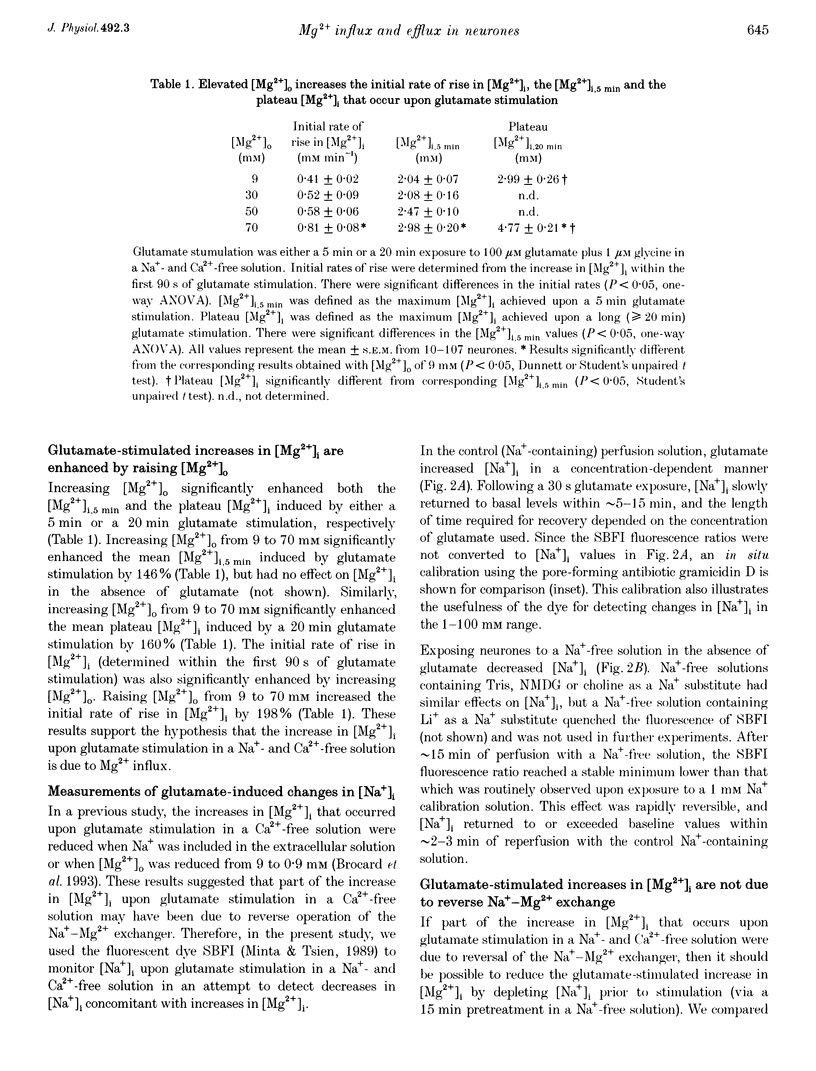
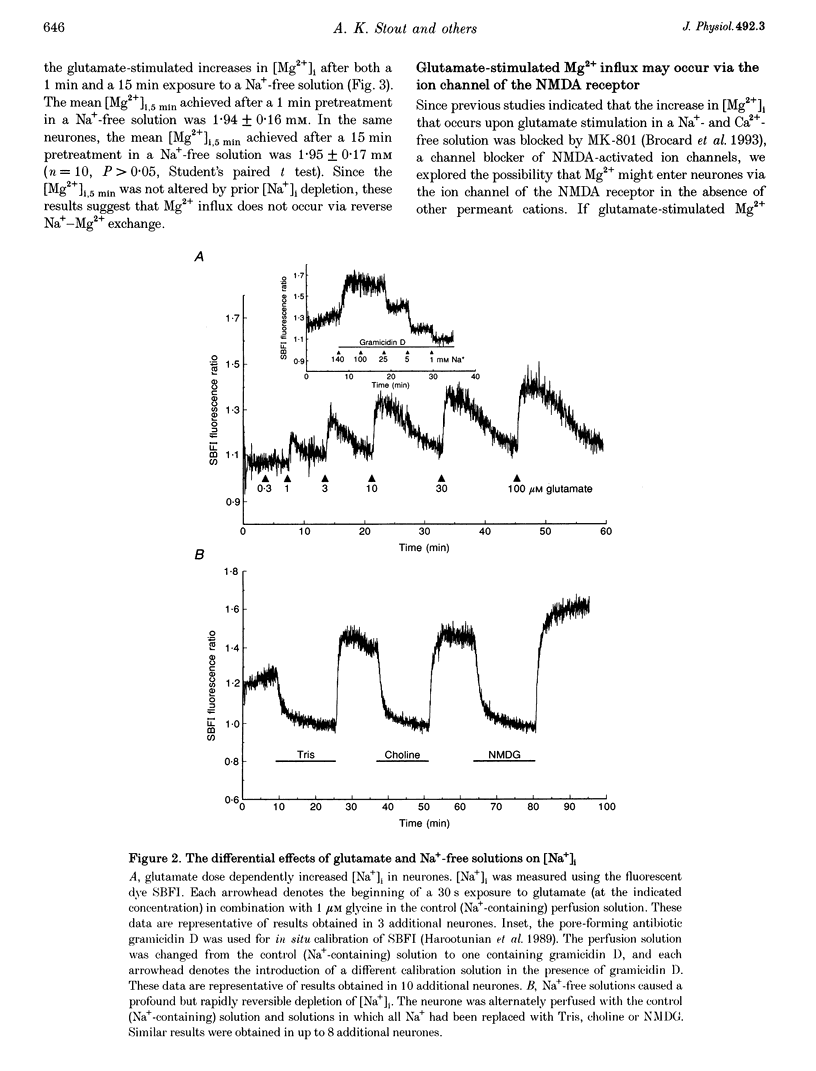
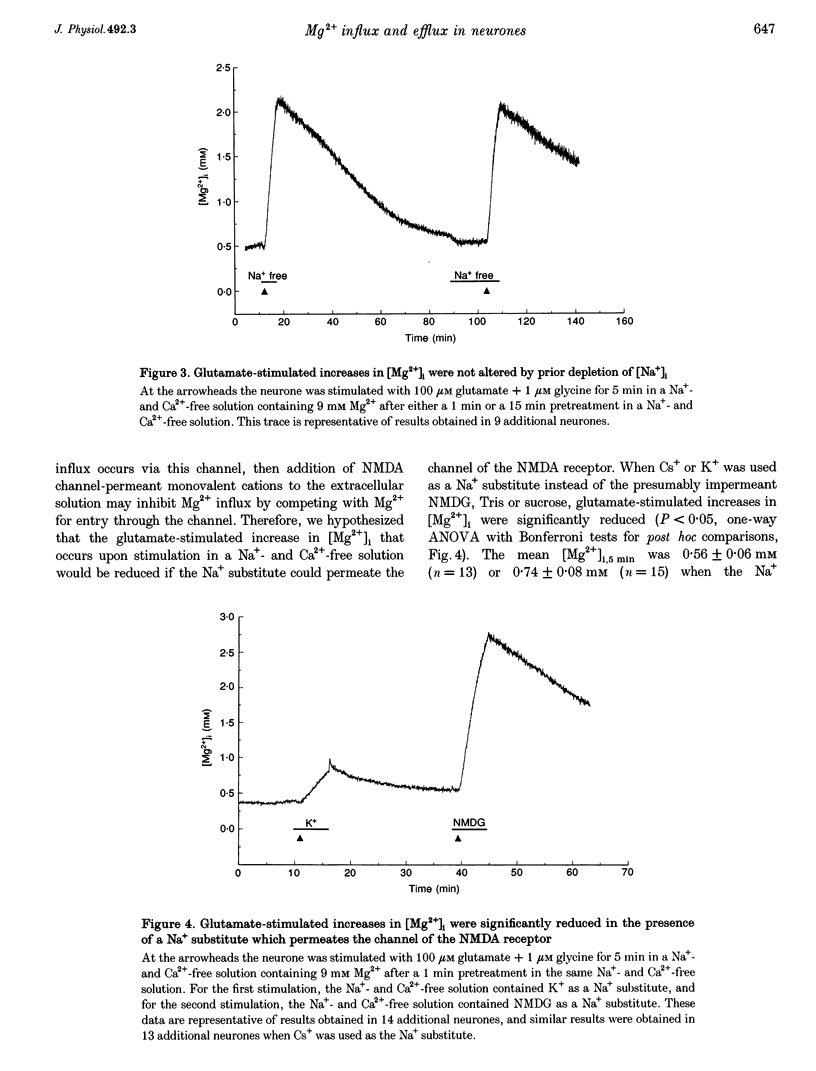
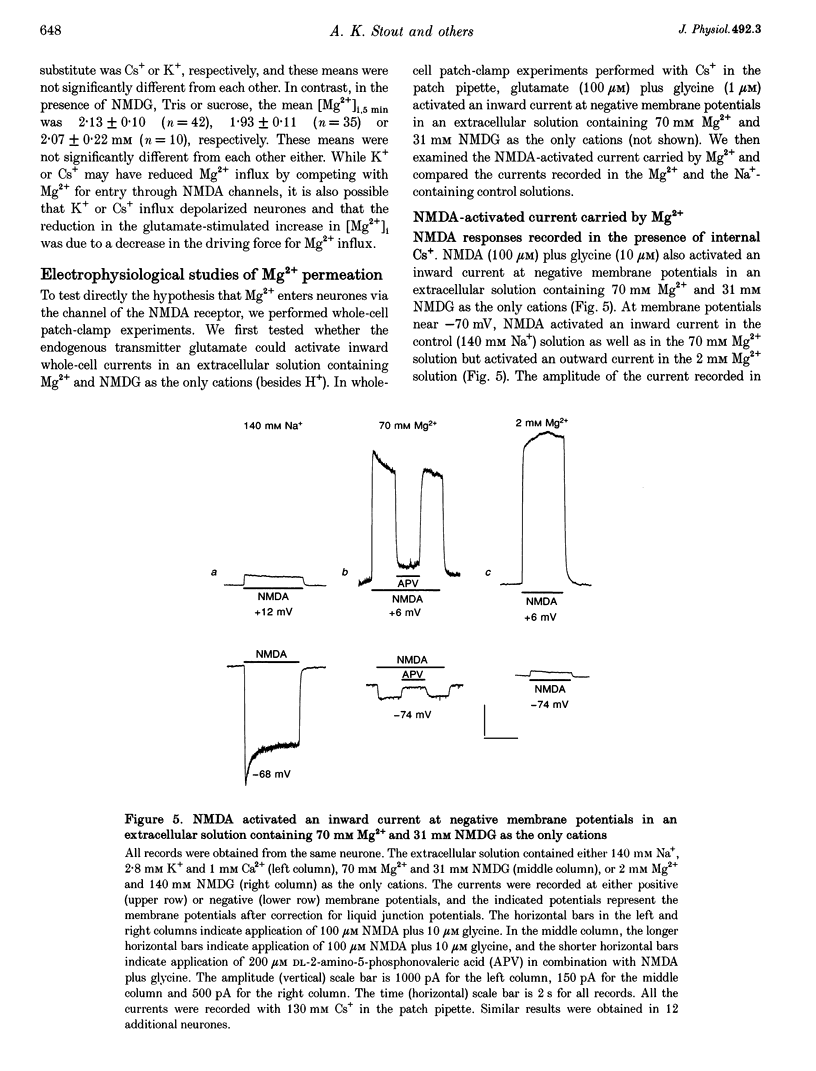
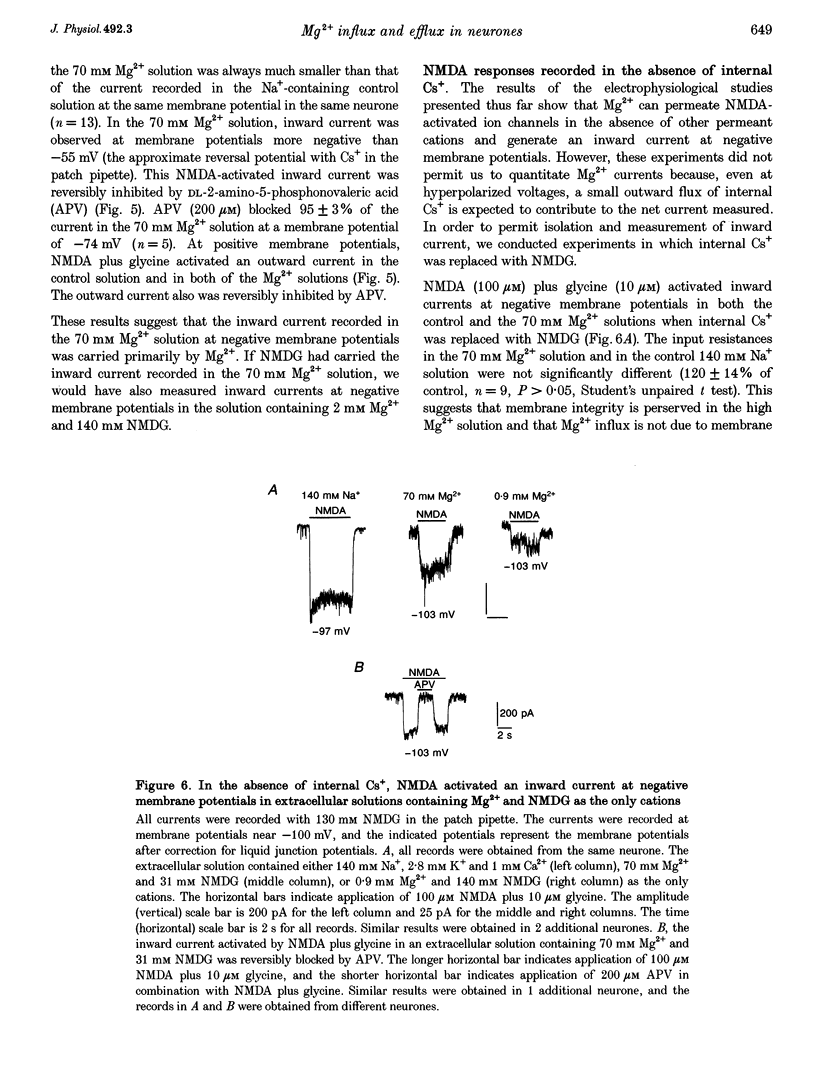
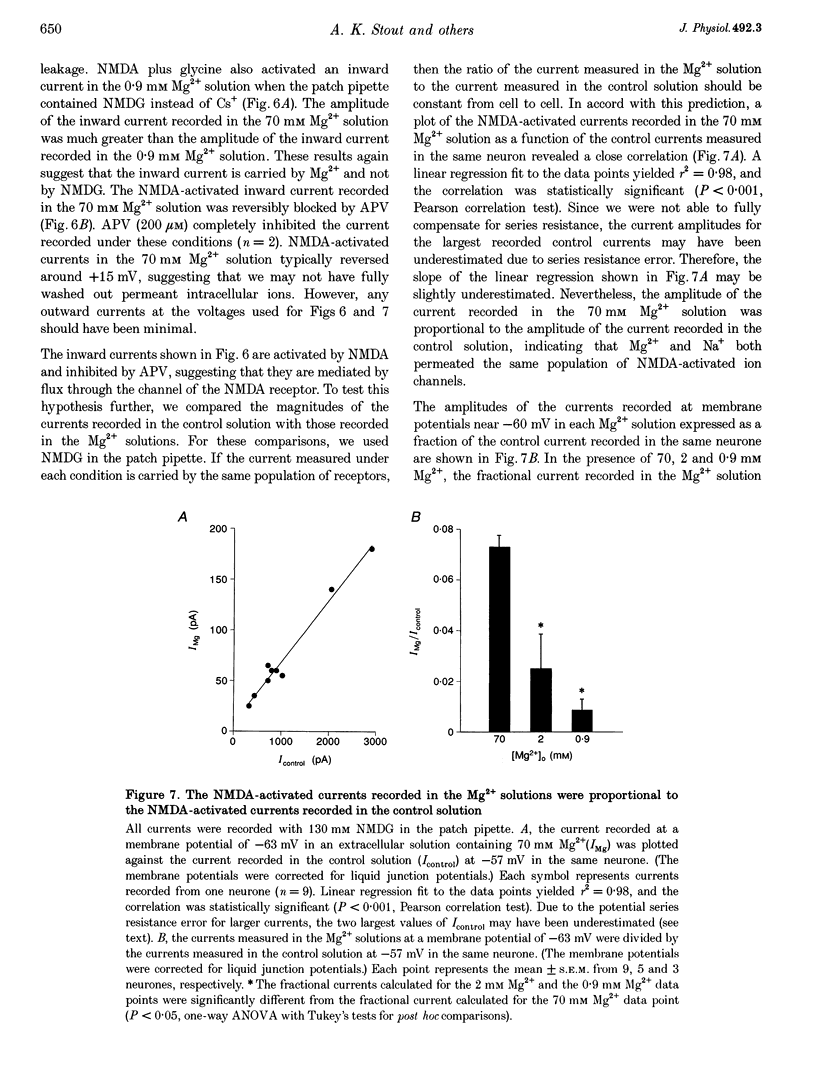
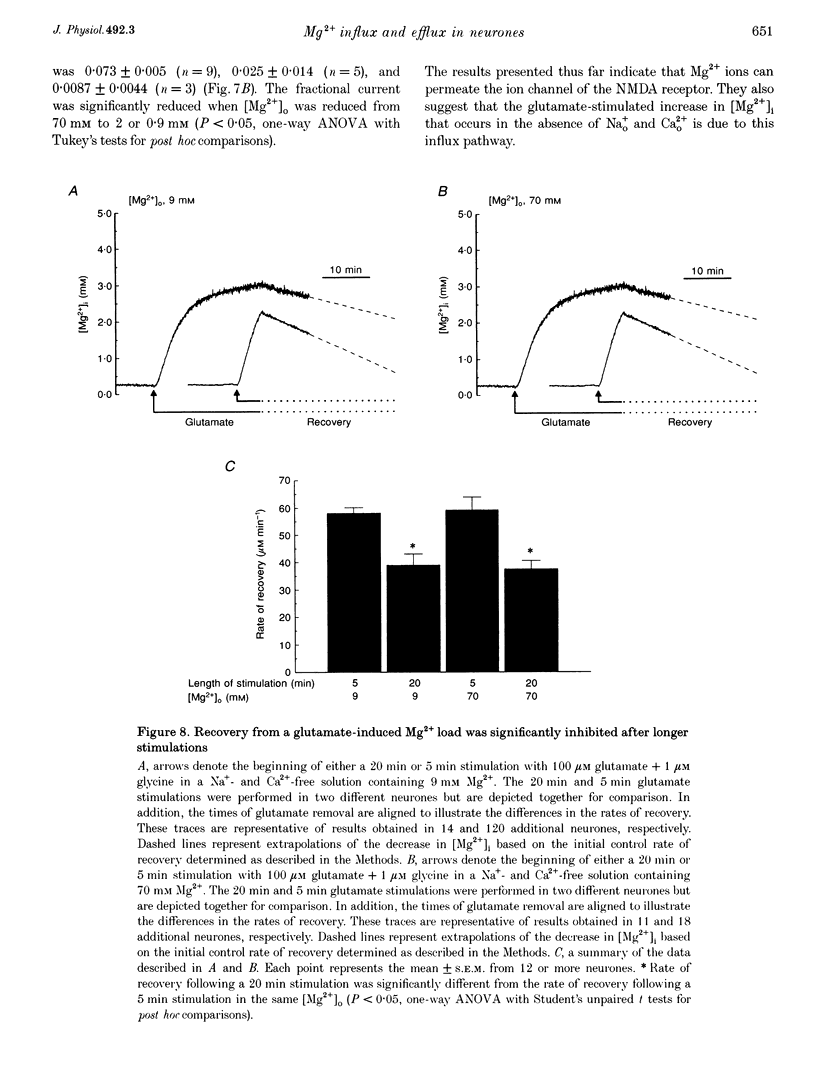
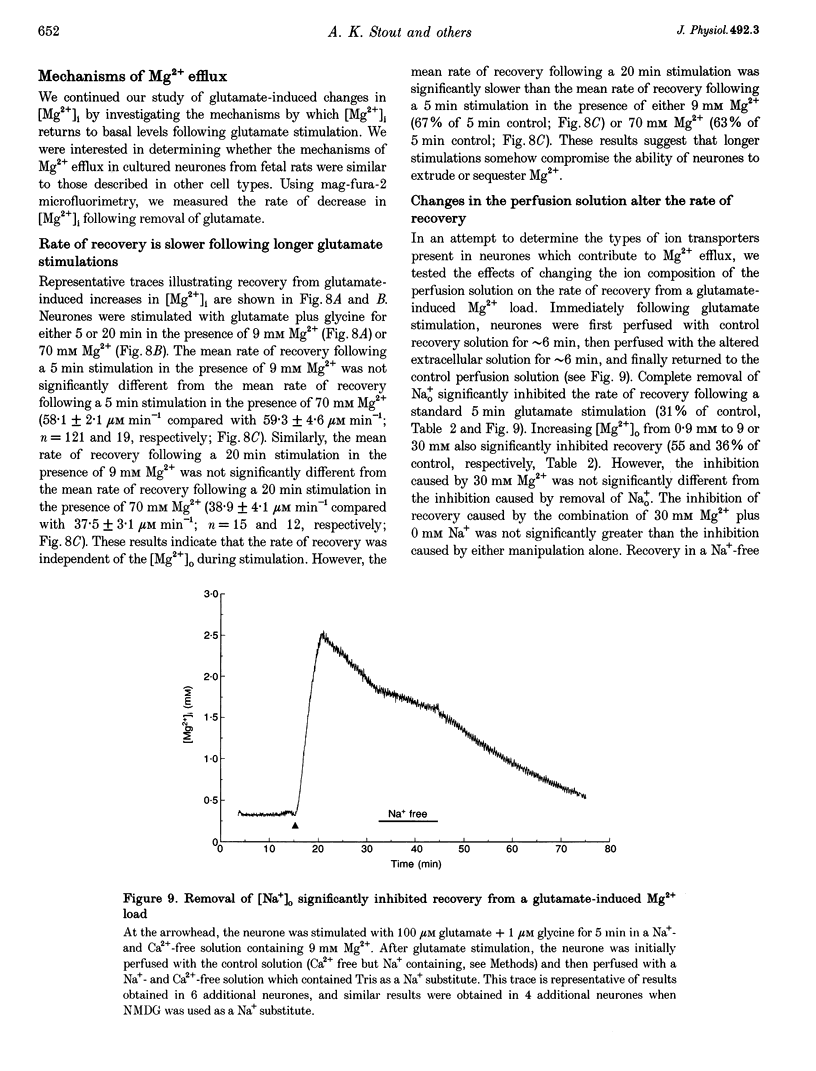
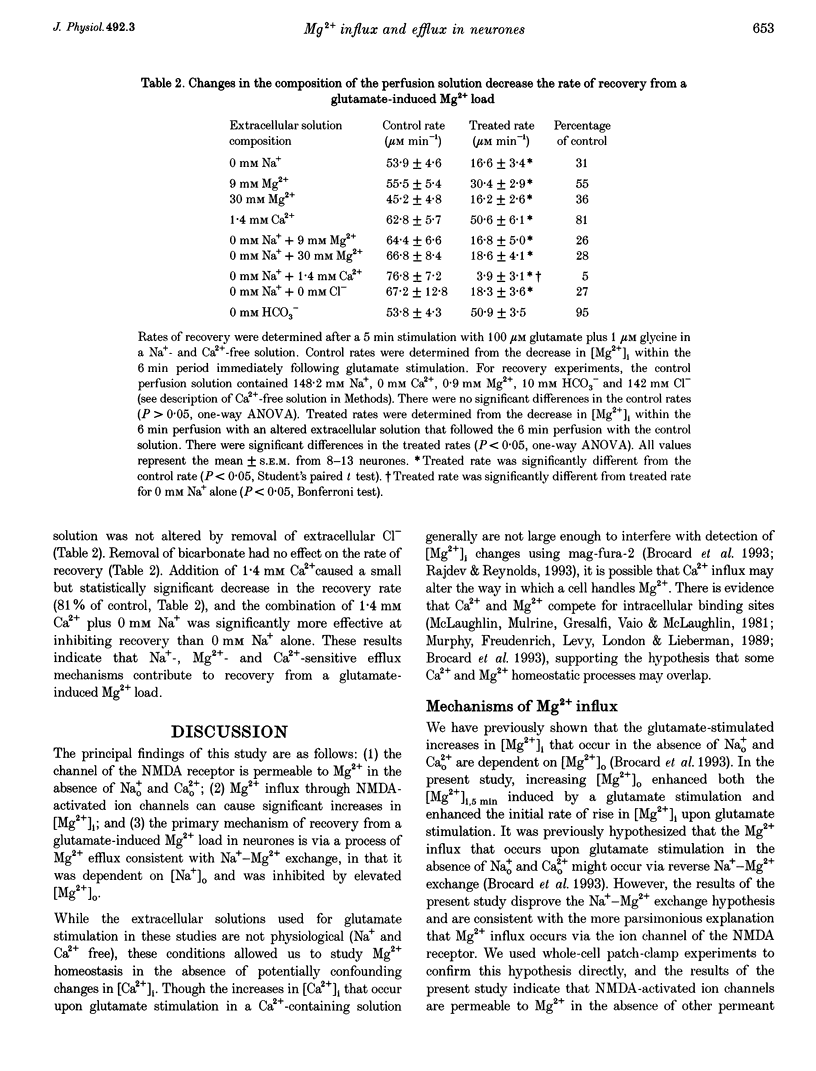
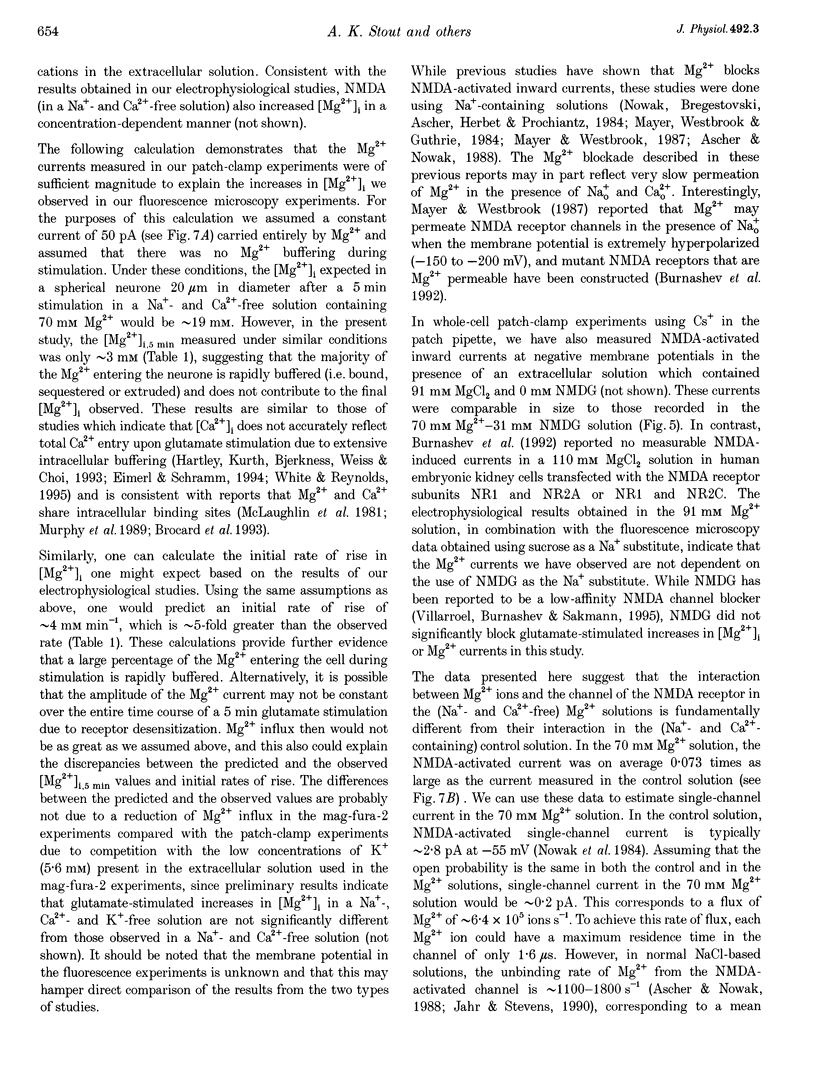
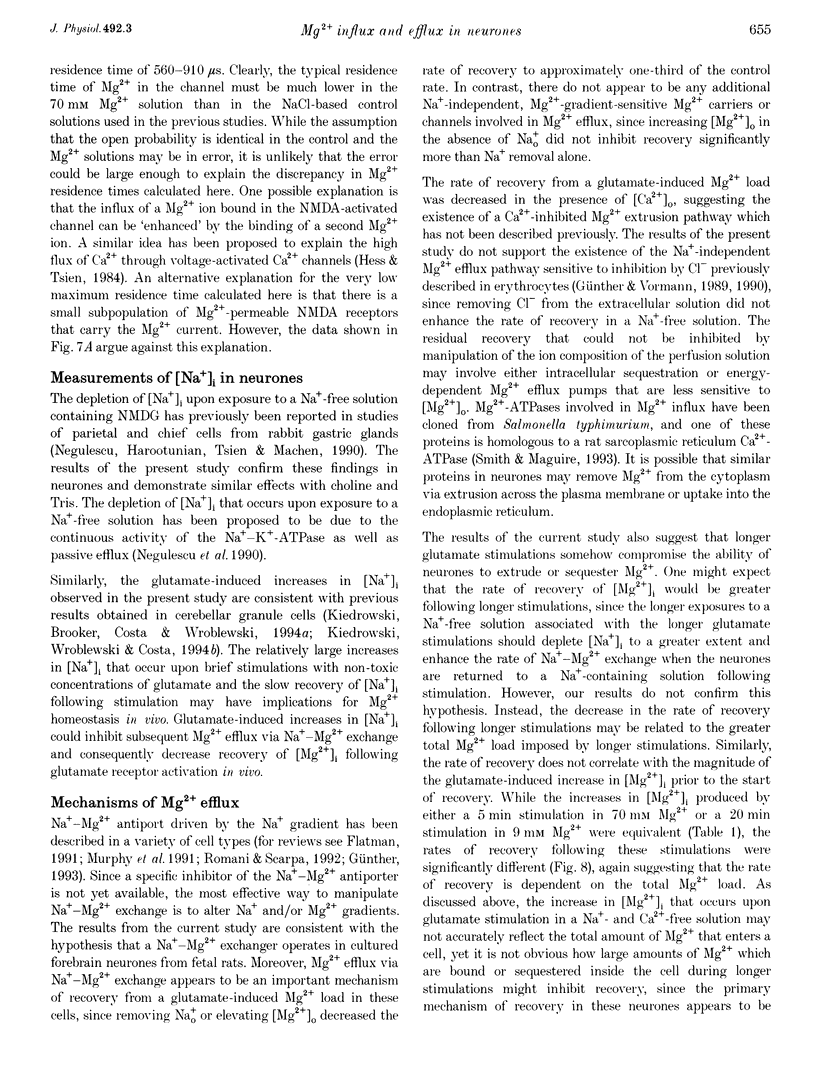
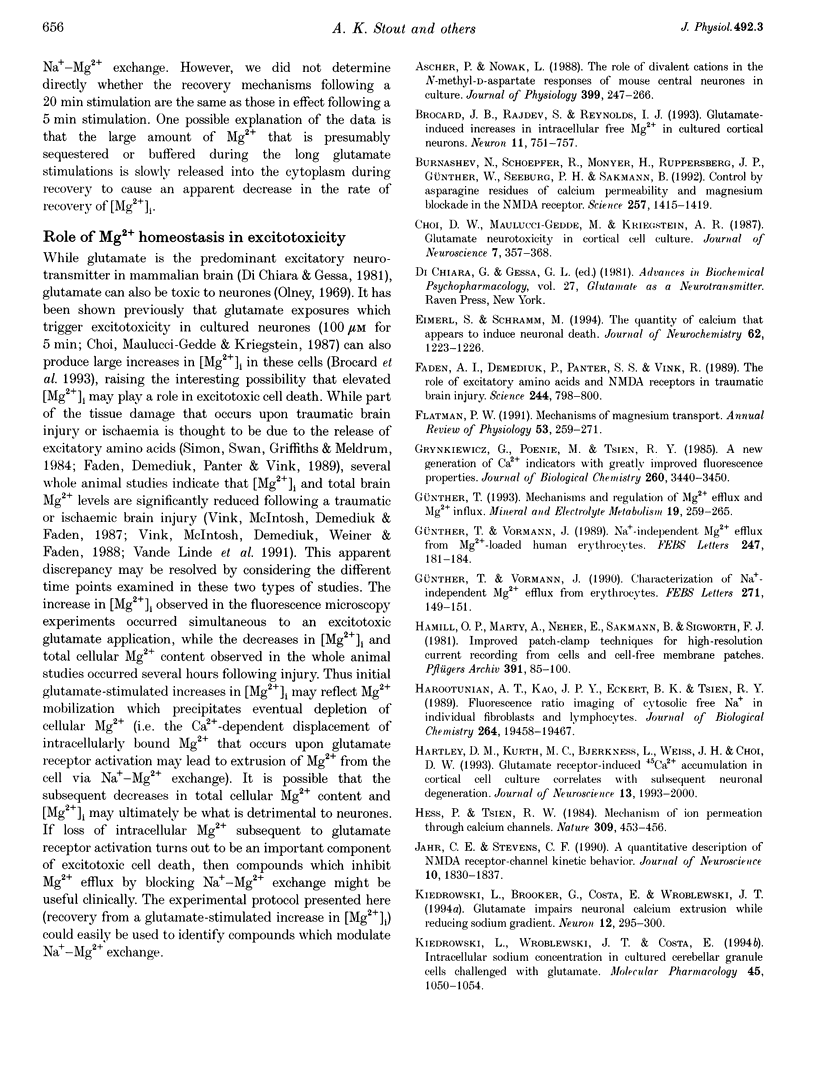
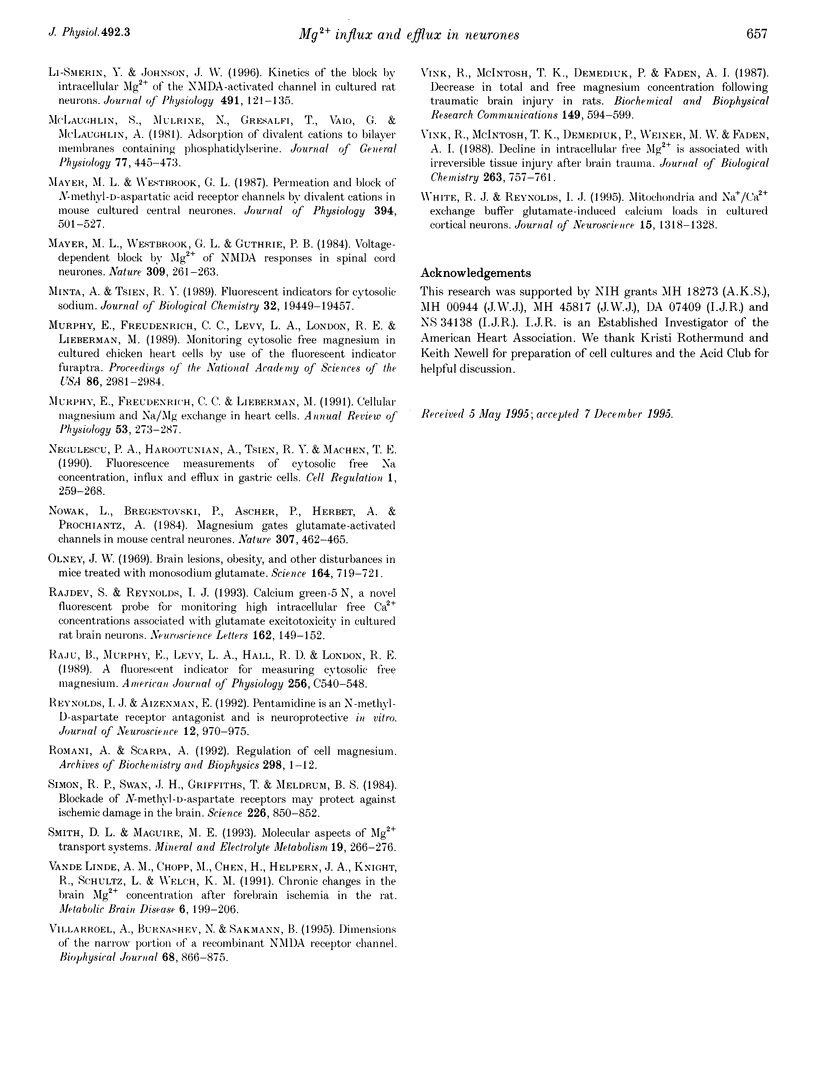
Selected References
These references are in PubMed. This may not be the complete list of references from this article.
- Ascher P., Nowak L. The role of divalent cations in the N-methyl-D-aspartate responses of mouse central neurones in culture. J Physiol. 1988 May;399:247–266. doi: 10.1113/jphysiol.1988.sp017078. [DOI] [PMC free article] [PubMed] [Google Scholar]
- Brocard J. B., Rajdev S., Reynolds I. J. Glutamate-induced increases in intracellular free Mg2+ in cultured cortical neurons. Neuron. 1993 Oct;11(4):751–757. doi: 10.1016/0896-6273(93)90084-5. [DOI] [PubMed] [Google Scholar]
- Burnashev N., Schoepfer R., Monyer H., Ruppersberg J. P., Günther W., Seeburg P. H., Sakmann B. Control by asparagine residues of calcium permeability and magnesium blockade in the NMDA receptor. Science. 1992 Sep 4;257(5075):1415–1419. doi: 10.1126/science.1382314. [DOI] [PubMed] [Google Scholar]
- Choi D. W., Maulucci-Gedde M., Kriegstein A. R. Glutamate neurotoxicity in cortical cell culture. J Neurosci. 1987 Feb;7(2):357–368. doi: 10.1523/JNEUROSCI.07-02-00357.1987. [DOI] [PMC free article] [PubMed] [Google Scholar]
- Eimerl S., Schramm M. The quantity of calcium that appears to induce neuronal death. J Neurochem. 1994 Mar;62(3):1223–1226. doi: 10.1046/j.1471-4159.1994.62031223.x. [DOI] [PubMed] [Google Scholar]
- Faden A. I., Demediuk P., Panter S. S., Vink R. The role of excitatory amino acids and NMDA receptors in traumatic brain injury. Science. 1989 May 19;244(4906):798–800. doi: 10.1126/science.2567056. [DOI] [PubMed] [Google Scholar]
- Flatman P. W. Mechanisms of magnesium transport. Annu Rev Physiol. 1991;53:259–271. doi: 10.1146/annurev.ph.53.030191.001355. [DOI] [PubMed] [Google Scholar]
- Grynkiewicz G., Poenie M., Tsien R. Y. A new generation of Ca2+ indicators with greatly improved fluorescence properties. J Biol Chem. 1985 Mar 25;260(6):3440–3450. [PubMed] [Google Scholar]
- Günther T. Mechanisms and regulation of Mg2+ efflux and Mg2+ influx. Miner Electrolyte Metab. 1993;19(4-5):259–265. [PubMed] [Google Scholar]
- Günther T., Vormann J. Characterization of Na(+)-independent Mg2+ efflux from erythrocytes. FEBS Lett. 1990 Oct 1;271(1-2):149–151. doi: 10.1016/0014-5793(90)80394-x. [DOI] [PubMed] [Google Scholar]
- Günther T., Vormann J. Na+-independent Mg2+ efflux from Mg2+-loaded human erythrocytes. FEBS Lett. 1989 Apr 24;247(2):181–184. doi: 10.1016/0014-5793(89)81329-8. [DOI] [PubMed] [Google Scholar]
- Hamill O. P., Marty A., Neher E., Sakmann B., Sigworth F. J. Improved patch-clamp techniques for high-resolution current recording from cells and cell-free membrane patches. Pflugers Arch. 1981 Aug;391(2):85–100. doi: 10.1007/BF00656997. [DOI] [PubMed] [Google Scholar]
- Harootunian A. T., Kao J. P., Eckert B. K., Tsien R. Y. Fluorescence ratio imaging of cytosolic free Na+ in individual fibroblasts and lymphocytes. J Biol Chem. 1989 Nov 15;264(32):19458–19467. [PubMed] [Google Scholar]
- Hartley D. M., Kurth M. C., Bjerkness L., Weiss J. H., Choi D. W. Glutamate receptor-induced 45Ca2+ accumulation in cortical cell culture correlates with subsequent neuronal degeneration. J Neurosci. 1993 May;13(5):1993–2000. doi: 10.1523/JNEUROSCI.13-05-01993.1993. [DOI] [PMC free article] [PubMed] [Google Scholar]
- Jahr C. E., Stevens C. F. A quantitative description of NMDA receptor-channel kinetic behavior. J Neurosci. 1990 Jun;10(6):1830–1837. doi: 10.1523/JNEUROSCI.10-06-01830.1990. [DOI] [PMC free article] [PubMed] [Google Scholar]
- Kiedrowski L., Wroblewski J. T., Costa E. Intracellular sodium concentration in cultured cerebellar granule cells challenged with glutamate. Mol Pharmacol. 1994 May;45(5):1050–1054. [PubMed] [Google Scholar]
- Mayer M. L., Westbrook G. L., Guthrie P. B. Voltage-dependent block by Mg2+ of NMDA responses in spinal cord neurones. Nature. 1984 May 17;309(5965):261–263. doi: 10.1038/309261a0. [DOI] [PubMed] [Google Scholar]
- Minta A., Tsien R. Y. Fluorescent indicators for cytosolic sodium. J Biol Chem. 1989 Nov 15;264(32):19449–19457. [PubMed] [Google Scholar]
- Negulescu P. A., Harootunian A., Tsien R. Y., Machen T. E. Fluorescence measurements of cytosolic free Na concentration, influx and efflux in gastric cells. Cell Regul. 1990 Feb;1(3):259–268. doi: 10.1091/mbc.1.3.259. [DOI] [PMC free article] [PubMed] [Google Scholar]
- Nowak L., Bregestovski P., Ascher P., Herbet A., Prochiantz A. Magnesium gates glutamate-activated channels in mouse central neurones. Nature. 1984 Feb 2;307(5950):462–465. doi: 10.1038/307462a0. [DOI] [PubMed] [Google Scholar]
- Olney J. W. Brain lesions, obesity, and other disturbances in mice treated with monosodium glutamate. Science. 1969 May 9;164(3880):719–721. doi: 10.1126/science.164.3880.719. [DOI] [PubMed] [Google Scholar]
- Rajdev S., Reynolds I. J. Calcium green-5N, a novel fluorescent probe for monitoring high intracellular free Ca2+ concentrations associated with glutamate excitotoxicity in cultured rat brain neurons. Neurosci Lett. 1993 Nov 12;162(1-2):149–152. doi: 10.1016/0304-3940(93)90582-6. [DOI] [PubMed] [Google Scholar]
- Raju B., Murphy E., Levy L. A., Hall R. D., London R. E. A fluorescent indicator for measuring cytosolic free magnesium. Am J Physiol. 1989 Mar;256(3 Pt 1):C540–C548. doi: 10.1152/ajpcell.1989.256.3.C540. [DOI] [PubMed] [Google Scholar]
- Romani A., Scarpa A. Regulation of cell magnesium. Arch Biochem Biophys. 1992 Oct;298(1):1–12. doi: 10.1016/0003-9861(92)90086-c. [DOI] [PubMed] [Google Scholar]
- Simon R. P., Swan J. H., Griffiths T., Meldrum B. S. Blockade of N-methyl-D-aspartate receptors may protect against ischemic damage in the brain. Science. 1984 Nov 16;226(4676):850–852. doi: 10.1126/science.6093256. [DOI] [PubMed] [Google Scholar]
- Smith D. L., Maguire M. E. Molecular aspects of Mg2+ transport systems. Miner Electrolyte Metab. 1993;19(4-5):266–276. [PubMed] [Google Scholar]
- Vande Linde A. M., Chopp M., Chen H., Helpern J. A., Knight R., Schultz L., Welch K. M. Chronic changes in the brain Mg2+ concentration after forebrain ischemia in the rat. Metab Brain Dis. 1991 Dec;6(4):199–206. doi: 10.1007/BF00996919. [DOI] [PubMed] [Google Scholar]
- Vink R., McIntosh T. K., Demediuk P., Faden A. I. Decrease in total and free magnesium concentration following traumatic brain injury in rats. Biochem Biophys Res Commun. 1987 Dec 16;149(2):594–599. doi: 10.1016/0006-291x(87)90409-8. [DOI] [PubMed] [Google Scholar]