Abstract
1. Amino acids with a charged or a polar residue in the putative pore region, between lysine 346 and glutamate 372 of the alpha-subunit of the cGMP-gated channel from bovine rods were mutated to a different amino acid. The mRNA encoding for the wild-type, i.e. the alpha-subunit, or mutant channels was injected in Xenopus laevis oocytes. 2. When glutamate 363 was mutated to asparagine, serine or alanine, the current activated by a steady cGMP concentration declined in mutant channels. No current decline was observed when glutamate 363 was mutated to aspartate, glutamine or glycine, when theronine 359, 360 and 364 were mutated to alanine or when other charged residues in the pore region were neutralized. 3. The amount of current decline and its time course were significantly voltage dependent. In mutant E363A the current decline developed within about 1.5 s at -100 mV, but in about 6 s at +100 mV. In the same mutant, the current declined to about 55% of its initial level at +100 mV and to about 10% at -100 mV. 4. The current decline in mutants E363A, E363S and E363N was only moderately dependent on the cGMP concentration (from 10 to 1000 microM) and was not caused by a reduced affinity of the mutant channels for cGMP. Analysis of current fluctuations at a single-channel level indicated that current decline was primarily caused by a decrease of the open probability. 5. The wild-type channel was not permeable to dimethylammonium. When glutamate 363 was replaced by a smaller residue such as serine, mutant channels became permeable to dimethylammonium. 6. The current decline observed in mutant channels is reminiscent of desensitization of ligand-gated channels and of inactivation of voltage-gated channels. These results suggest also that gating and permeation through the cGMP-gated channel from bovine rods are intrinsically coupled and that glutamate 363 is part of the molecular structure controlling both the gating and the narrowest region of the pore.
Full text
PDF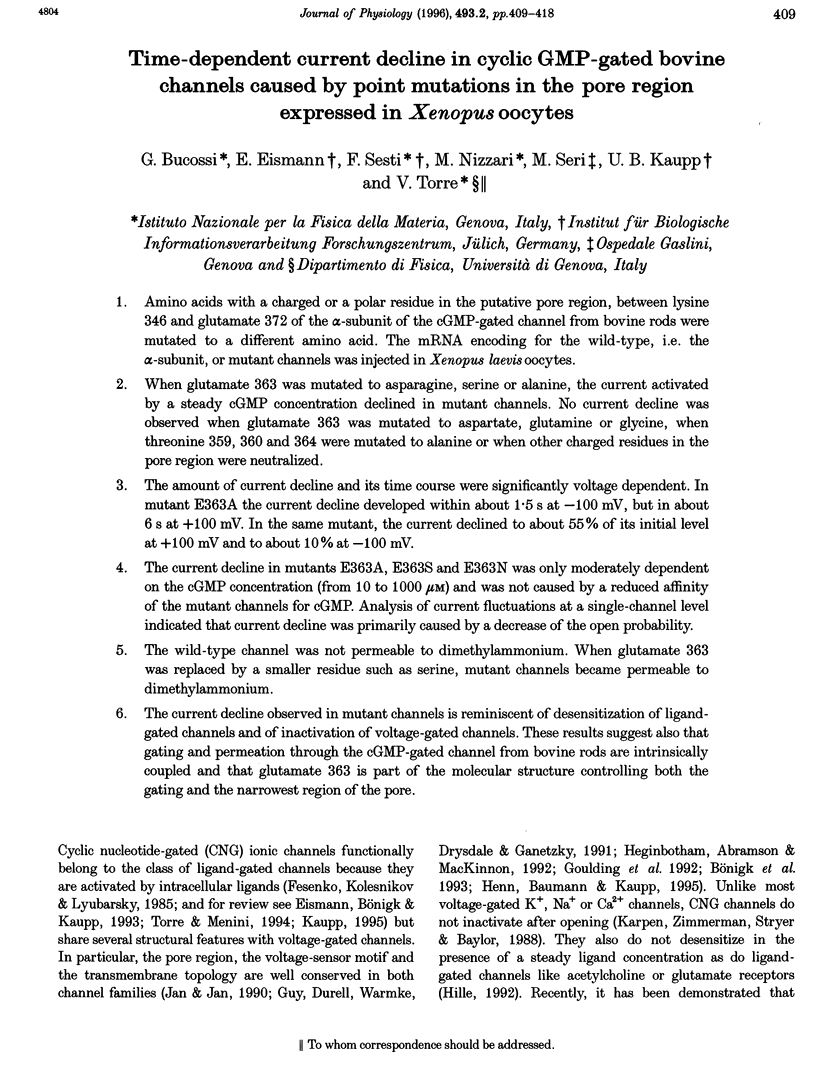
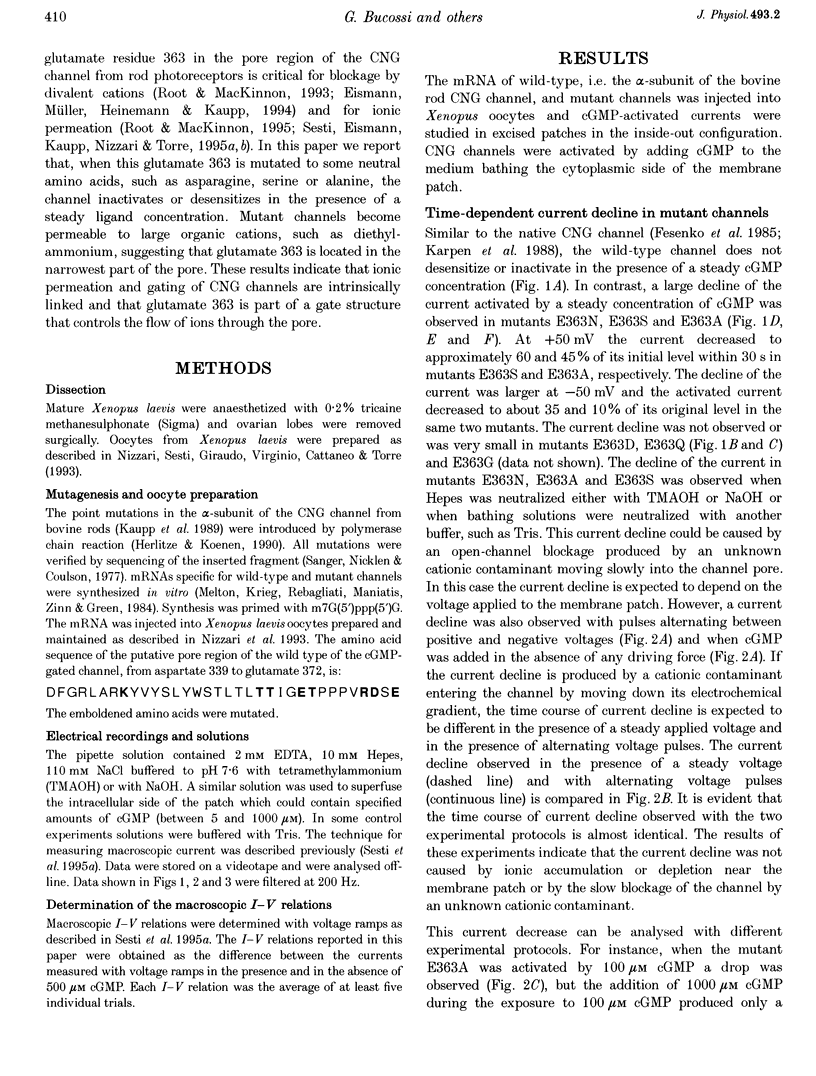
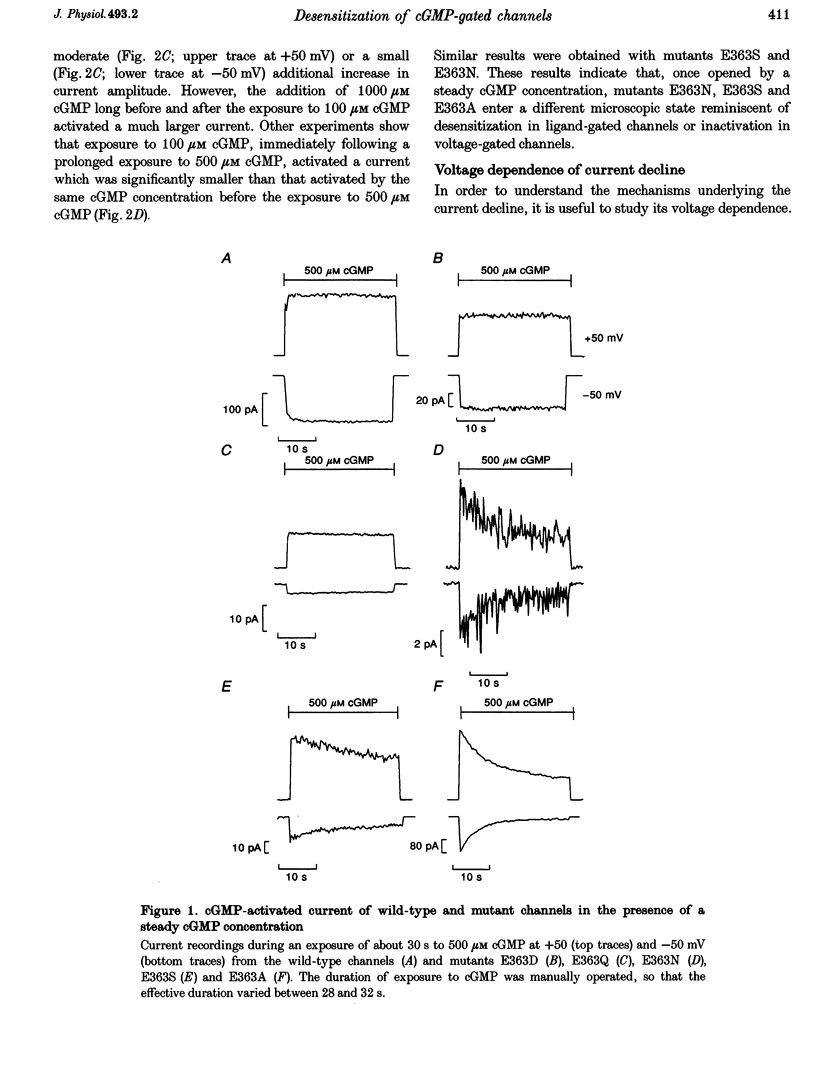
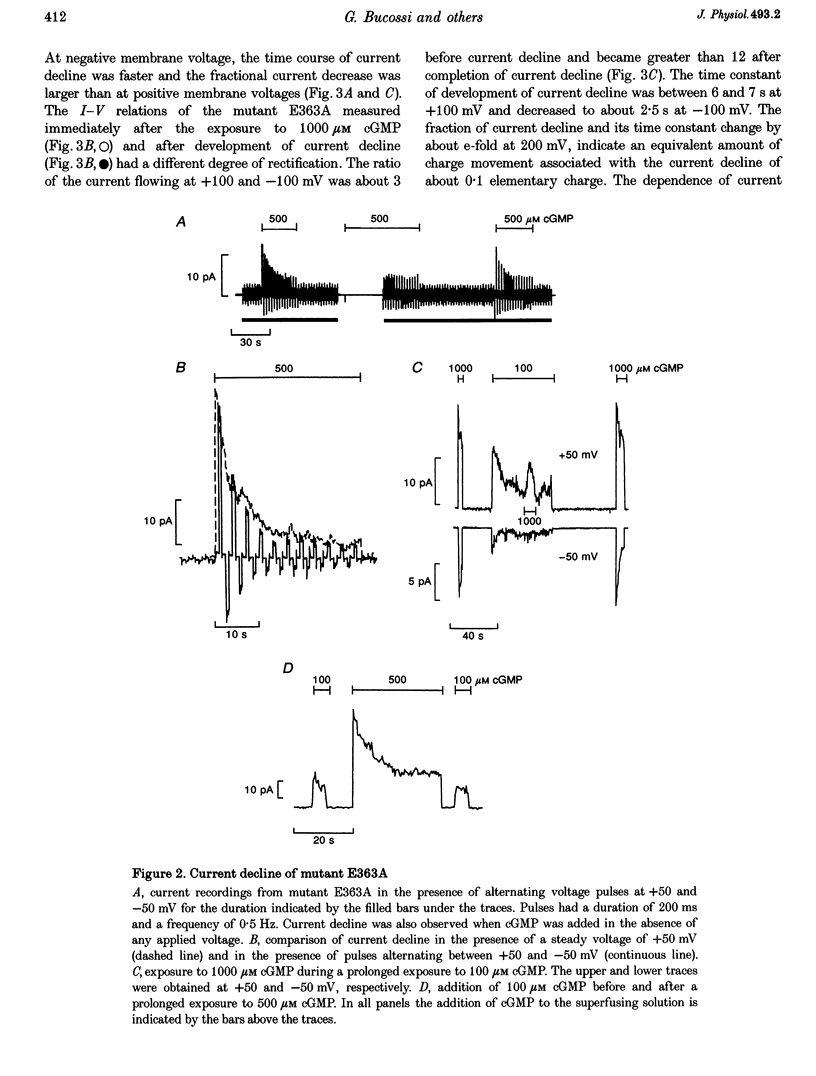
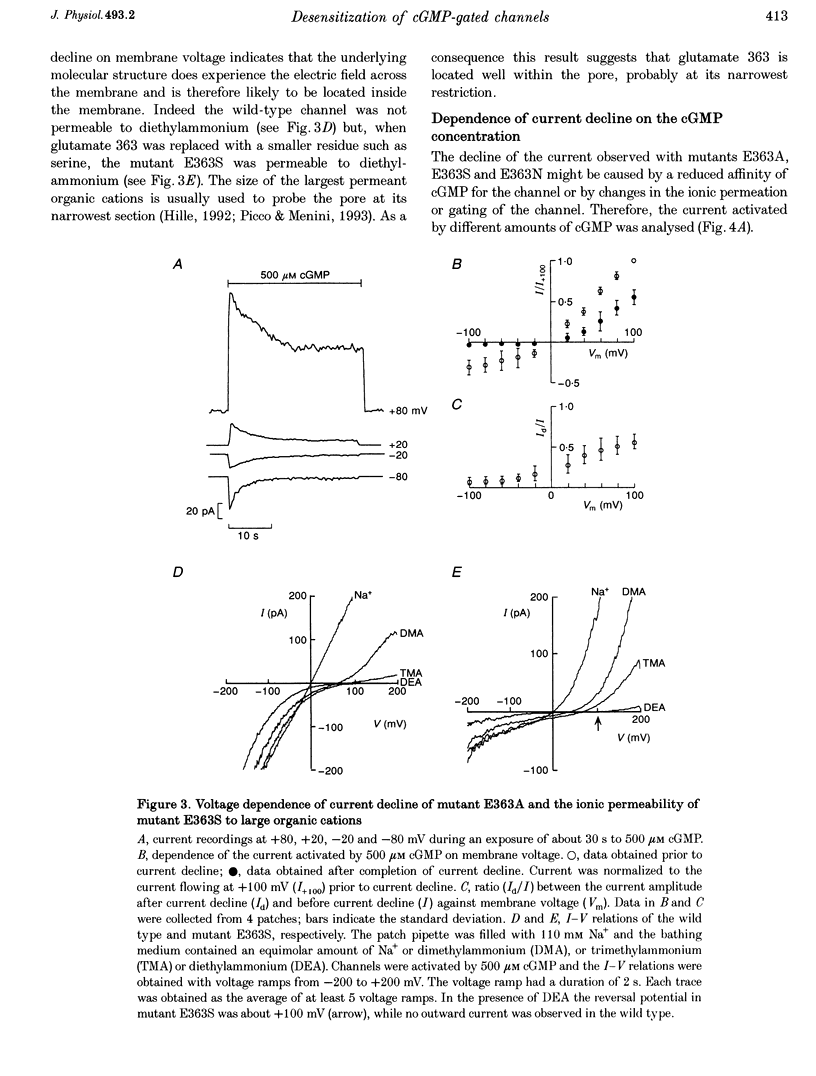
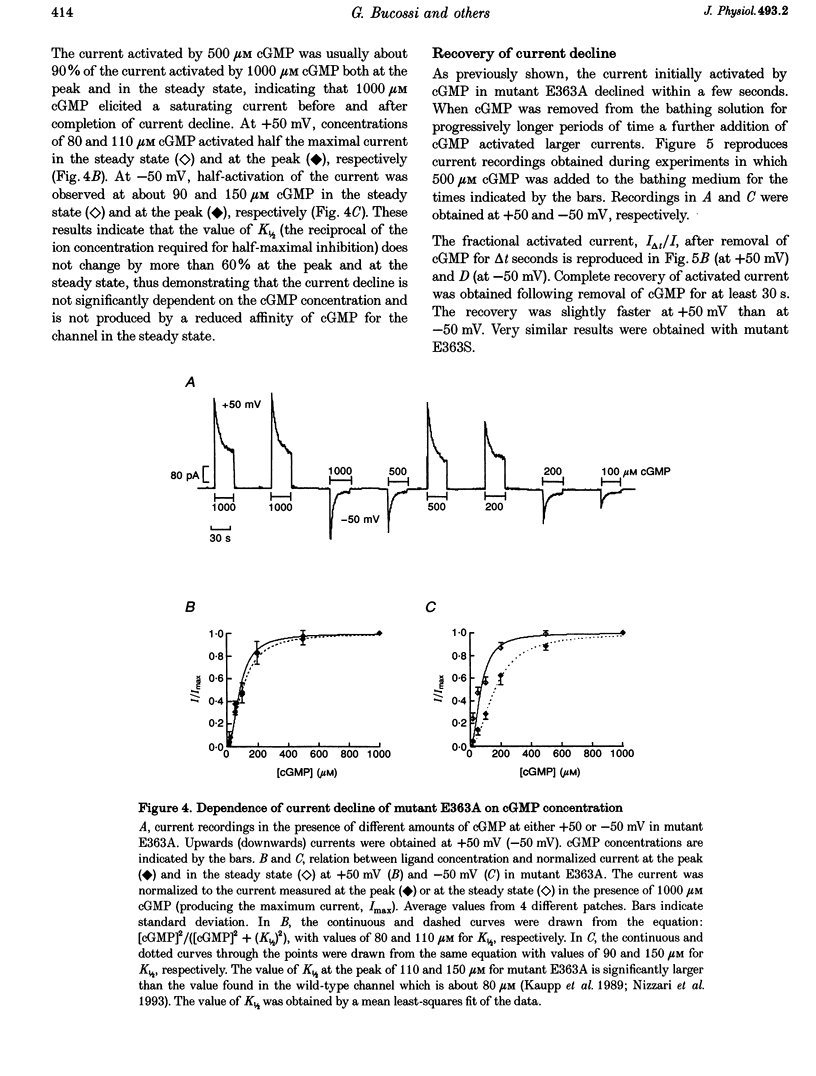
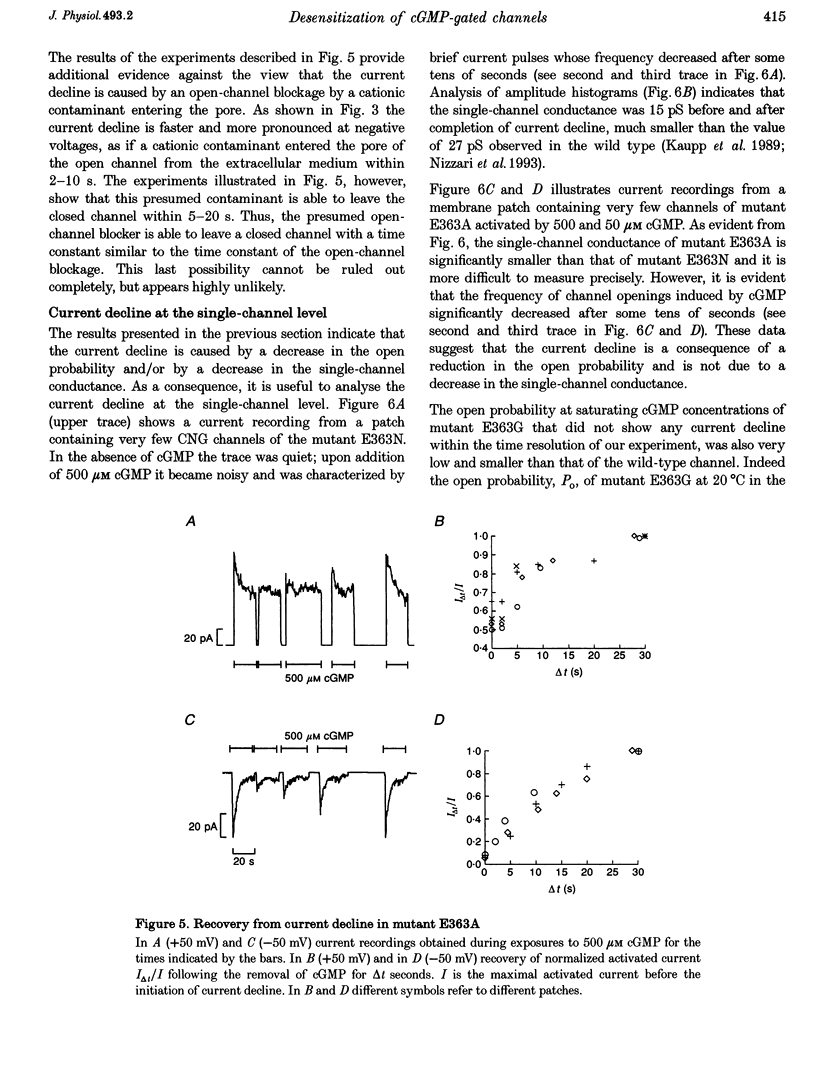
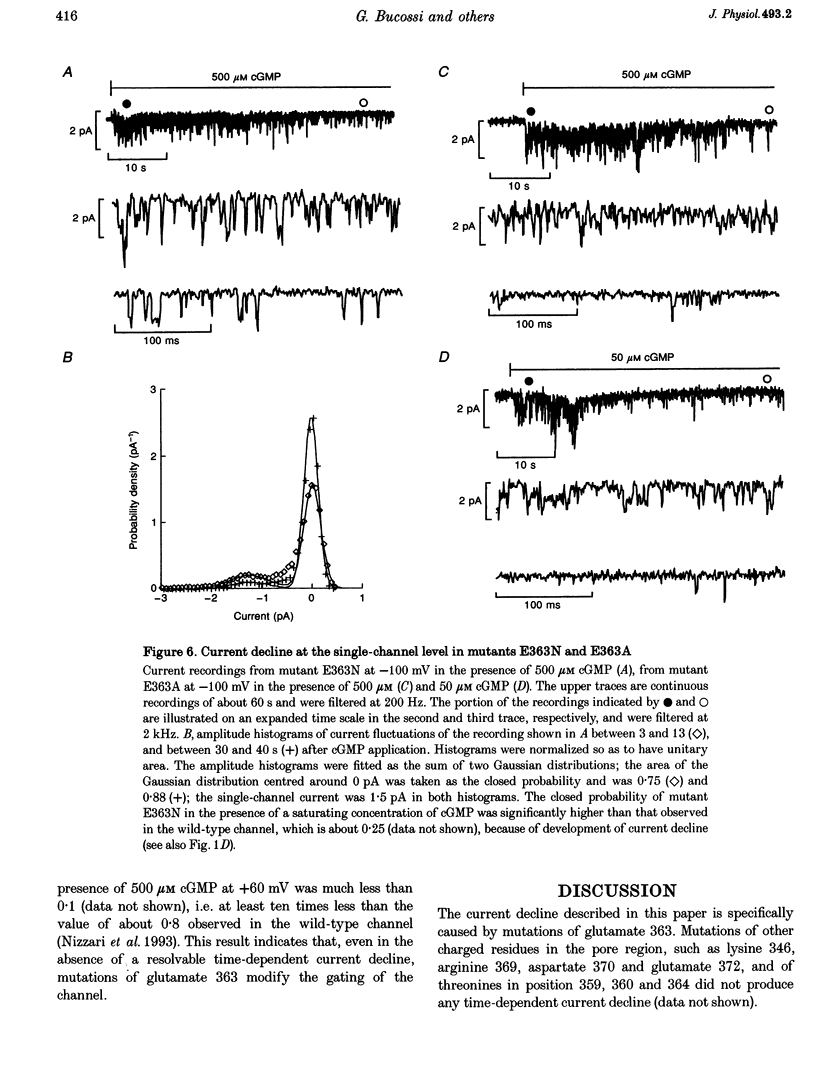
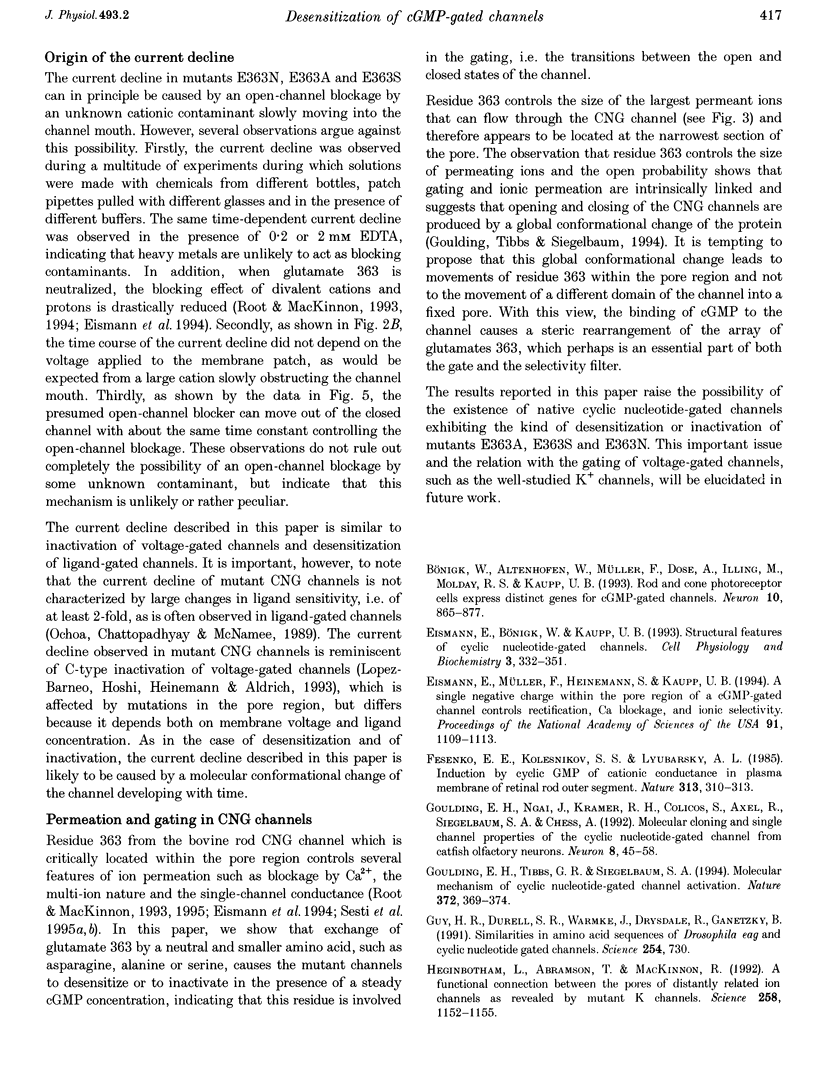
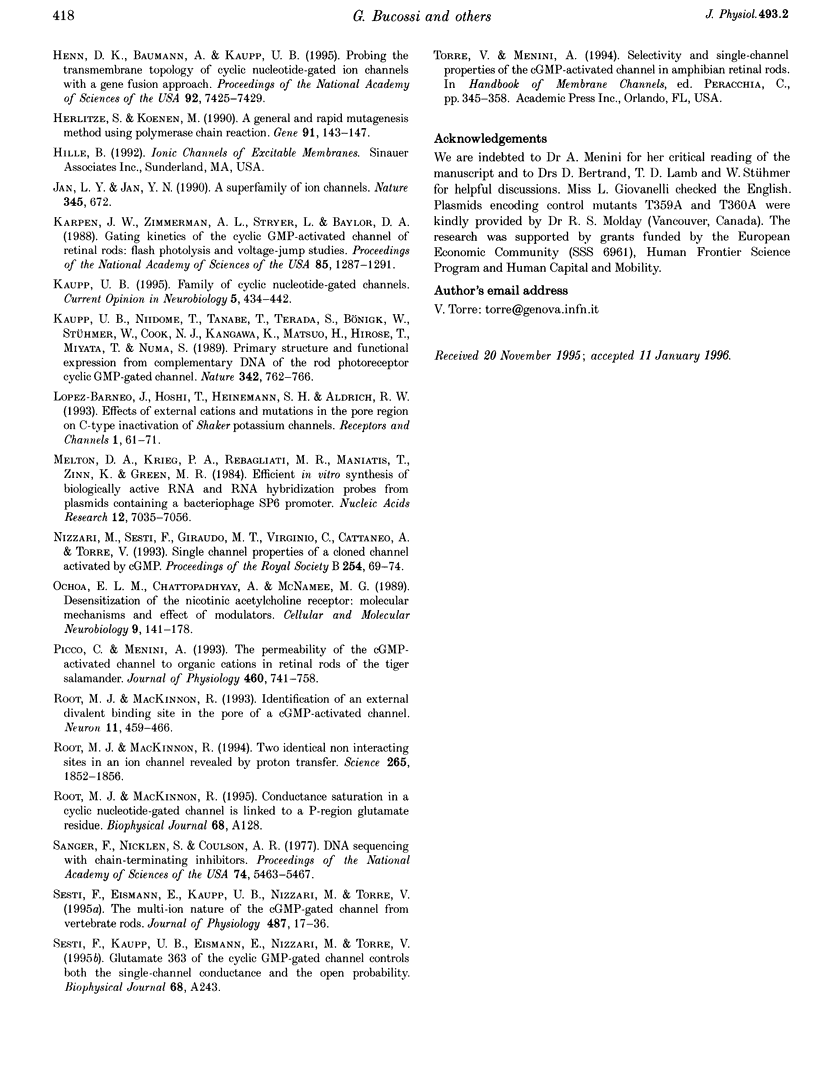
Selected References
These references are in PubMed. This may not be the complete list of references from this article.
- Bönigk W., Altenhofen W., Müller F., Dose A., Illing M., Molday R. S., Kaupp U. B. Rod and cone photoreceptor cells express distinct genes for cGMP-gated channels. Neuron. 1993 May;10(5):865–877. doi: 10.1016/0896-6273(93)90202-3. [DOI] [PubMed] [Google Scholar]
- Eismann E., Müller F., Heinemann S. H., Kaupp U. B. A single negative charge within the pore region of a cGMP-gated channel controls rectification, Ca2+ blockage, and ionic selectivity. Proc Natl Acad Sci U S A. 1994 Feb 1;91(3):1109–1113. doi: 10.1073/pnas.91.3.1109. [DOI] [PMC free article] [PubMed] [Google Scholar]
- Goulding E. H., Ngai J., Kramer R. H., Colicos S., Axel R., Siegelbaum S. A., Chess A. Molecular cloning and single-channel properties of the cyclic nucleotide-gated channel from catfish olfactory neurons. Neuron. 1992 Jan;8(1):45–58. doi: 10.1016/0896-6273(92)90107-o. [DOI] [PubMed] [Google Scholar]
- Goulding E. H., Tibbs G. R., Siegelbaum S. A. Molecular mechanism of cyclic-nucleotide-gated channel activation. Nature. 1994 Nov 24;372(6504):369–374. doi: 10.1038/372369a0. [DOI] [PubMed] [Google Scholar]
- Guy H. R., Durell S. R., Warmke J., Drysdale R., Ganetzky B. Similarities in amino acid sequences of Drosophila eag and cyclic nucleotide-gated channels. Science. 1991 Nov 1;254(5032):730–730. doi: 10.1126/science.1658932. [DOI] [PubMed] [Google Scholar]
- Heginbotham L., Abramson T., MacKinnon R. A functional connection between the pores of distantly related ion channels as revealed by mutant K+ channels. Science. 1992 Nov 13;258(5085):1152–1155. doi: 10.1126/science.1279807. [DOI] [PubMed] [Google Scholar]
- Henn D. K., Baumann A., Kaupp U. B. Probing the transmembrane topology of cyclic nucleotide-gated ion channels with a gene fusion approach. Proc Natl Acad Sci U S A. 1995 Aug 1;92(16):7425–7429. doi: 10.1073/pnas.92.16.7425. [DOI] [PMC free article] [PubMed] [Google Scholar]
- Herlitze S., Koenen M. A general and rapid mutagenesis method using polymerase chain reaction. Gene. 1990 Jul 2;91(1):143–147. doi: 10.1016/0378-1119(90)90177-s. [DOI] [PubMed] [Google Scholar]
- Jan L. Y., Jan Y. N. A superfamily of ion channels. Nature. 1990 Jun 21;345(6277):672–672. doi: 10.1038/345672a0. [DOI] [PubMed] [Google Scholar]
- Kaupp U. B. Family of cyclic nucleotide gated ion channels. Curr Opin Neurobiol. 1995 Aug;5(4):434–442. doi: 10.1016/0959-4388(95)80002-6. [DOI] [PubMed] [Google Scholar]
- Kaupp U. B., Niidome T., Tanabe T., Terada S., Bönigk W., Stühmer W., Cook N. J., Kangawa K., Matsuo H., Hirose T. Primary structure and functional expression from complementary DNA of the rod photoreceptor cyclic GMP-gated channel. Nature. 1989 Dec 14;342(6251):762–766. doi: 10.1038/342762a0. [DOI] [PubMed] [Google Scholar]
- López-Barneo J., Hoshi T., Heinemann S. H., Aldrich R. W. Effects of external cations and mutations in the pore region on C-type inactivation of Shaker potassium channels. Receptors Channels. 1993;1(1):61–71. [PubMed] [Google Scholar]
- Melton D. A., Krieg P. A., Rebagliati M. R., Maniatis T., Zinn K., Green M. R. Efficient in vitro synthesis of biologically active RNA and RNA hybridization probes from plasmids containing a bacteriophage SP6 promoter. Nucleic Acids Res. 1984 Sep 25;12(18):7035–7056. doi: 10.1093/nar/12.18.7035. [DOI] [PMC free article] [PubMed] [Google Scholar]
- Nizzari M., Sesti F., Giraudo M. T., Virginio C., Cattaneo A., Torre V. Single-channel properties of cloned cGMP-activated channels from retinal rods. Proc Biol Sci. 1993 Oct 22;254(1339):69–74. doi: 10.1098/rspb.1993.0128. [DOI] [PubMed] [Google Scholar]
- Ochoa E. L., Chattopadhyay A., McNamee M. G. Desensitization of the nicotinic acetylcholine receptor: molecular mechanisms and effect of modulators. Cell Mol Neurobiol. 1989 Jun;9(2):141–178. doi: 10.1007/BF00713026. [DOI] [PubMed] [Google Scholar]
- Picco C., Menini A. The permeability of the cGMP-activated channel to organic cations in retinal rods of the tiger salamander. J Physiol. 1993 Jan;460:741–758. doi: 10.1113/jphysiol.1993.sp019497. [DOI] [PMC free article] [PubMed] [Google Scholar]
- Root M. J., MacKinnon R. Identification of an external divalent cation-binding site in the pore of a cGMP-activated channel. Neuron. 1993 Sep;11(3):459–466. doi: 10.1016/0896-6273(93)90150-p. [DOI] [PubMed] [Google Scholar]
- Root M. J., MacKinnon R. Two identical noninteracting sites in an ion channel revealed by proton transfer. Science. 1994 Sep 23;265(5180):1852–1856. doi: 10.1126/science.7522344. [DOI] [PubMed] [Google Scholar]
- Sanger F., Nicklen S., Coulson A. R. DNA sequencing with chain-terminating inhibitors. Proc Natl Acad Sci U S A. 1977 Dec;74(12):5463–5467. doi: 10.1073/pnas.74.12.5463. [DOI] [PMC free article] [PubMed] [Google Scholar]
- Sesti F., Eismann E., Kaupp U. B., Nizzari M., Torre V. The multi-ion nature of the cGMP-gated channel from vertebrate rods. J Physiol. 1995 Aug 15;487(1):17–36. doi: 10.1113/jphysiol.1995.sp020858. [DOI] [PMC free article] [PubMed] [Google Scholar]