Abstract
1. We have shown previously, with experimental and computer models, how a '40 Hz' (gamma) oscillation can arise in networks of hippocampal interneurones, involving mutual GABAA-mediated synaptic inhibition and a source of tonic excitatory input. Here, we explore implications of this model for some hippocampal network phenomena in the rat in vitro and in vivo. 2. A model network was constructed of 1024 CA3 pyramidal cells and 256 interneurones. AMPA (alpha-amino-3-hydroxy-5-methyl-4-isoxazole propionic acid), NMDA (N-methyl-D-aspartate), GABAA and GABAB receptors were simulated on pyramidal cells and on interneurones. 3. In both model and experiment, the frequency of network oscillations, in the gamma range, depended upon three parameters: GABAA conductance and decay time constant in interneurone-->interneurone connections, and the driving current to the interneurones. 4. The model of gamma rhythm predicts an average zero phase lag between firing of pyramidal cells and interneurones, as observed in the rat hippocampus in vivo. The model also reproduces a gamma rhythm whose frequency changes with time, at theta frequency (about 5 Hz). This occurs when there is 5 Hz modulation of a tonic signal to chandelier and basket cells. 5. Synchronized bursts can be produced in the model by several means, including partial blockade of GABAA receptors or of AMPA receptors on interneurones, or by augmenting AMPA-mediated EPSCs. In all of these cases, the burst can be followed by a 'tail' of transiently occurring gamma waves, a phenomenon observed in the hippocampus in vivo following sharp waves. This tail occurs in the model because of delayed excitation of the interneurones by the synchronized burst. A tail of gamma activity was found after synchronized epileptiform bursts both in the hippocampal slice (CA3 region) and in vivo. 6. Our data suggest that gamma-frequency EEG activity arises in the hippocampus when pools of interneurones receive a tonic or slowly varying excitation. The frequency of the oscillation depends upon the strength of this excitation and on the parameters regulating the inhibitory coupling between the interneurones. The interneurone network output is then imposed upon pyramidal neurones in the form of rhythmic synchronized IPSPs.
Full text
PDF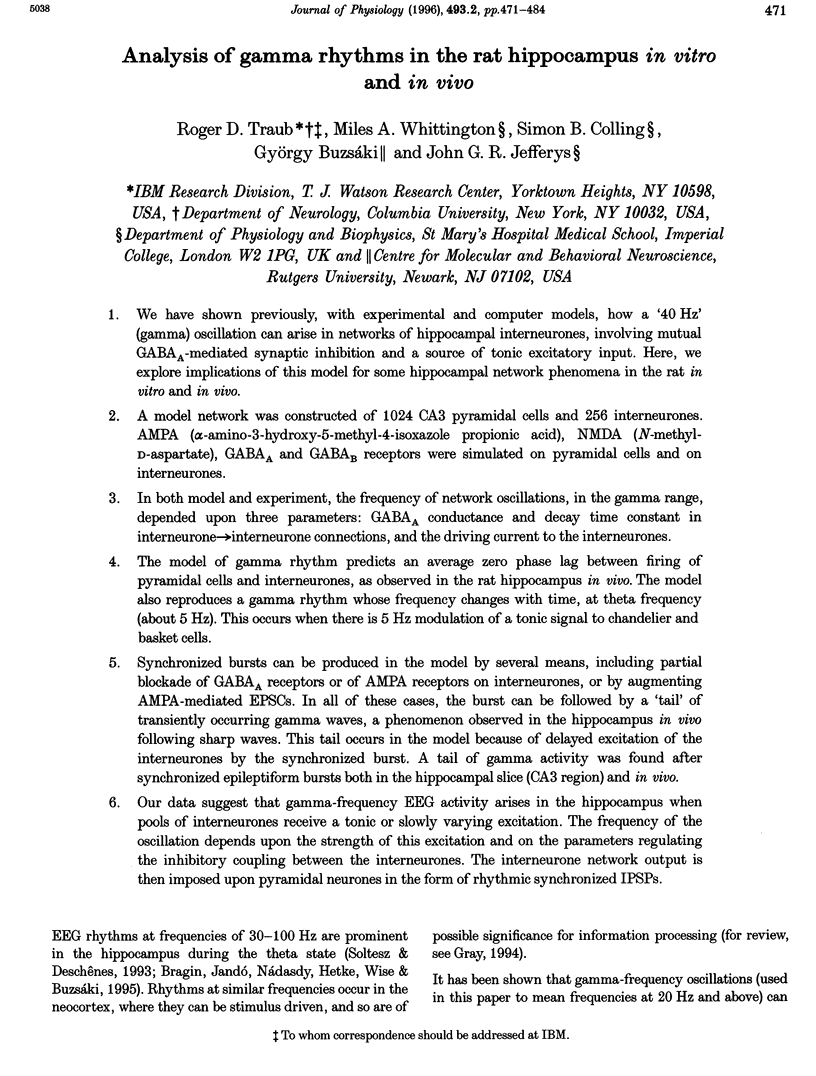
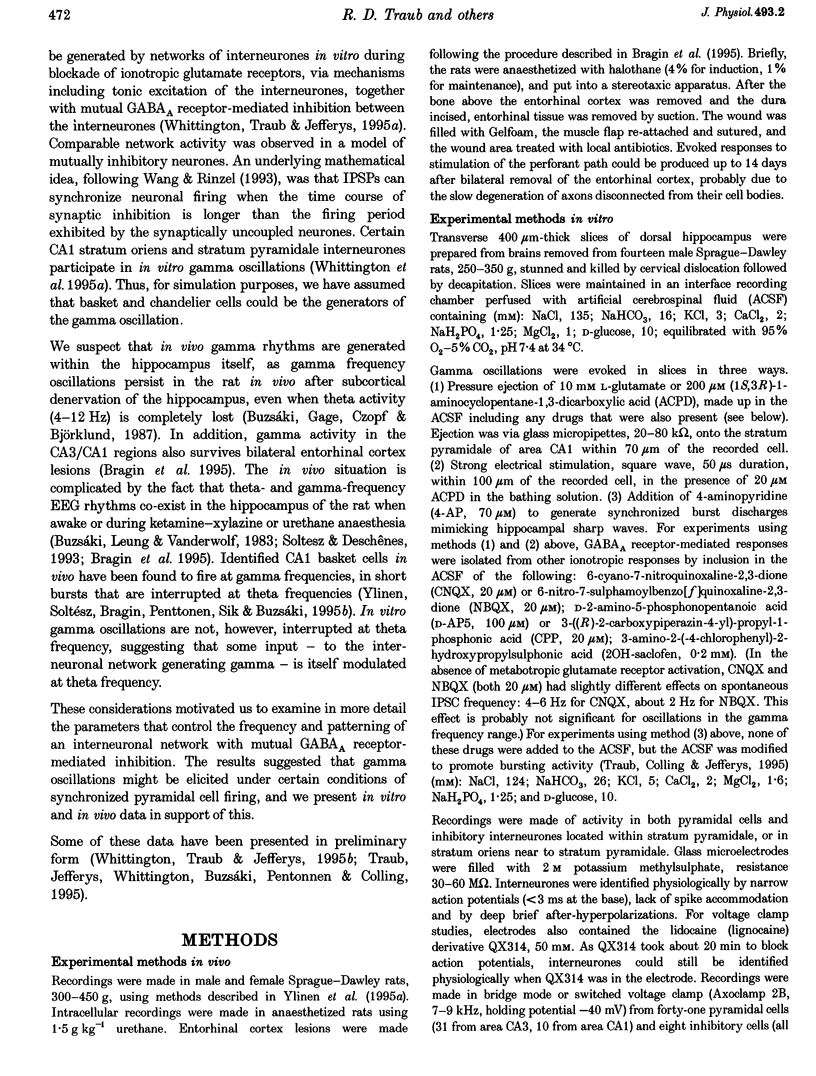
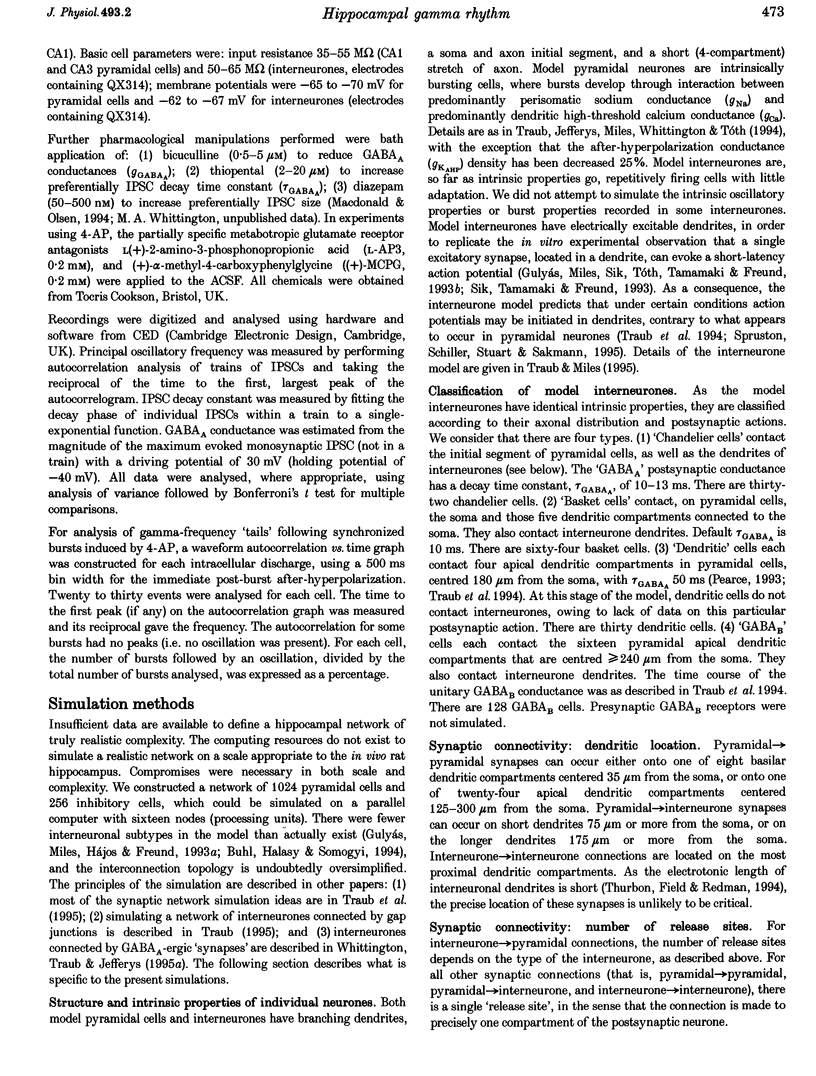
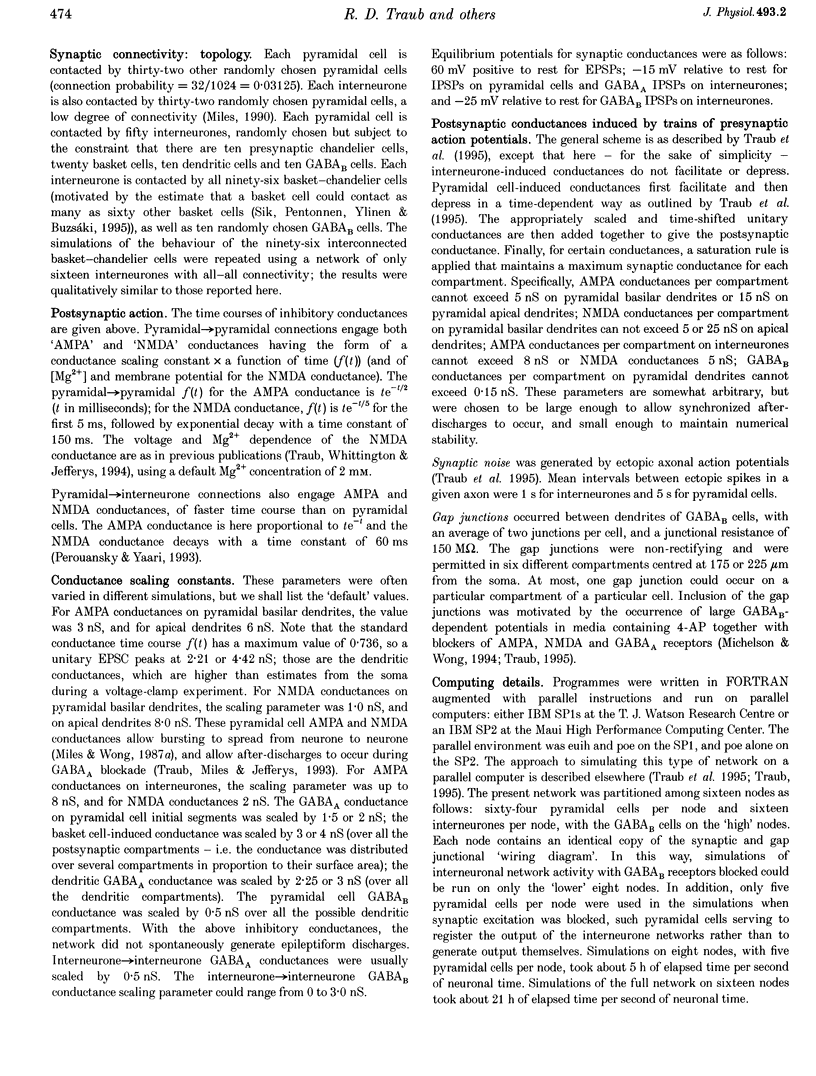
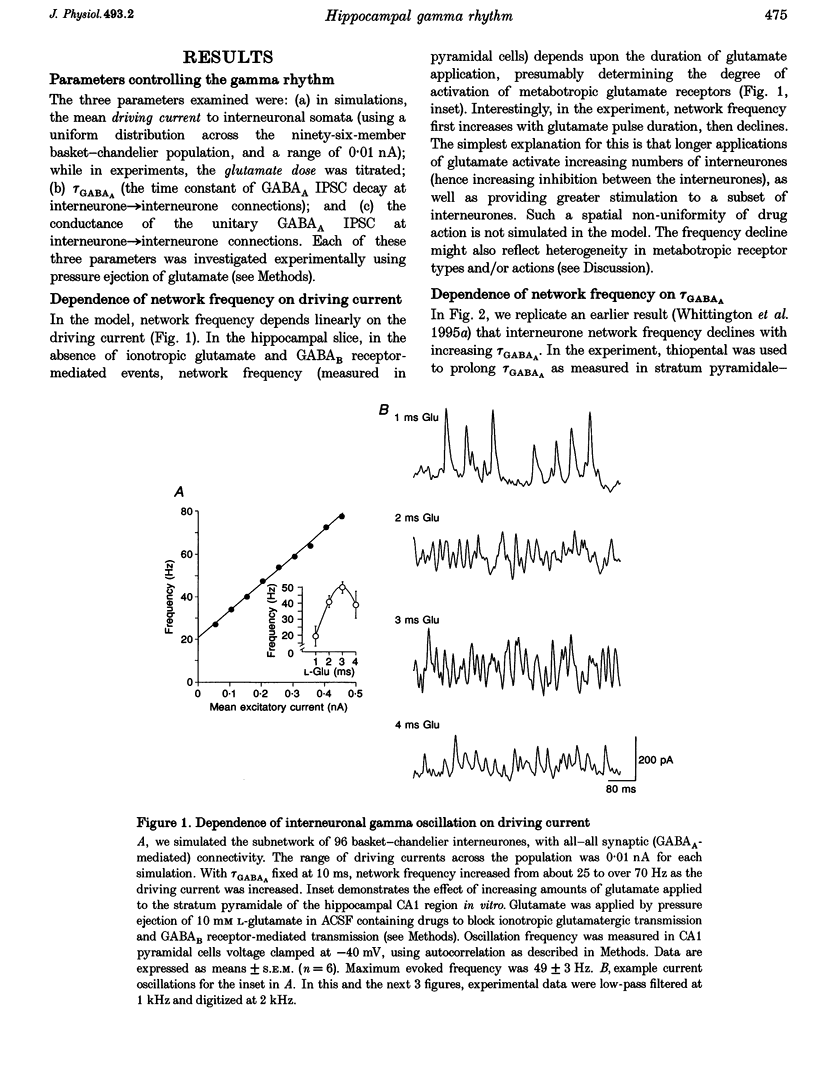
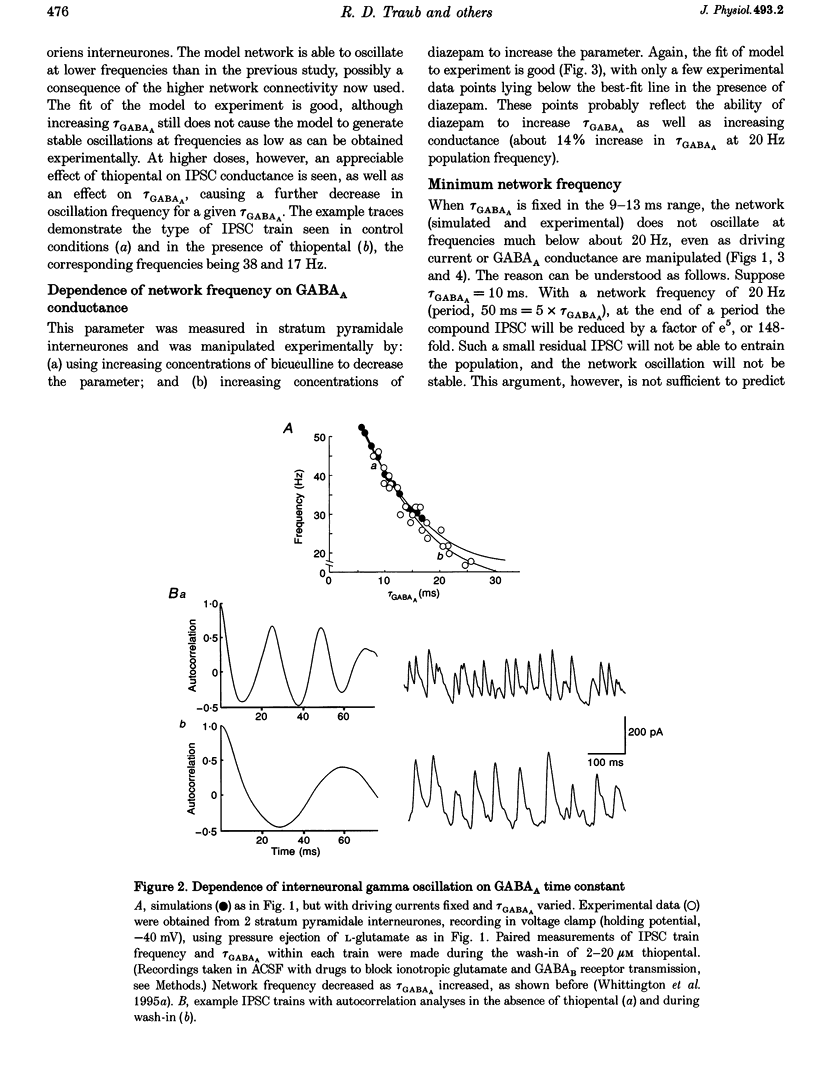
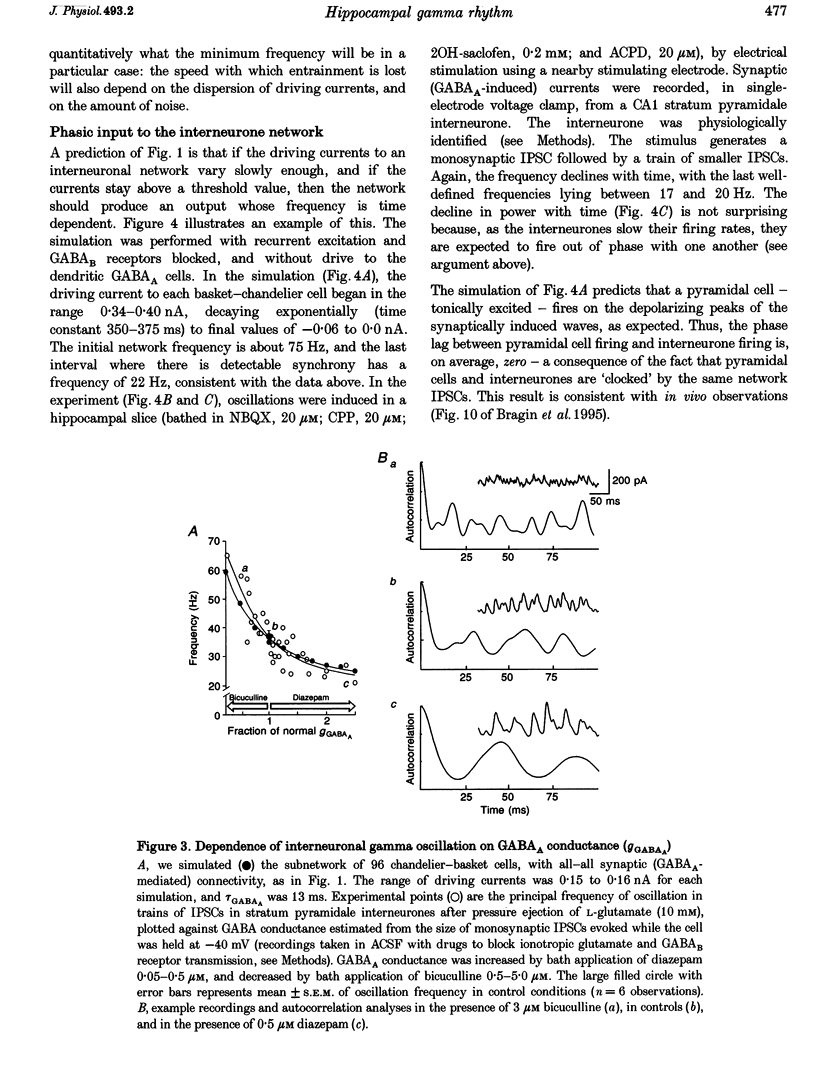
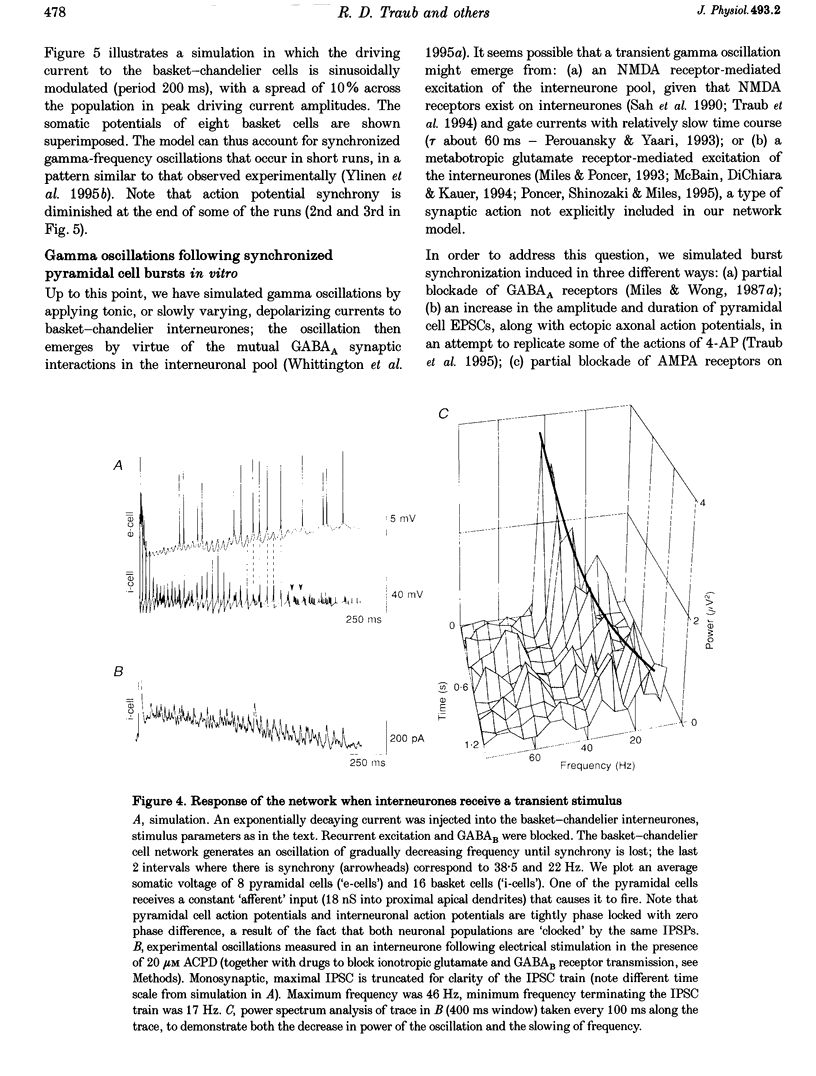
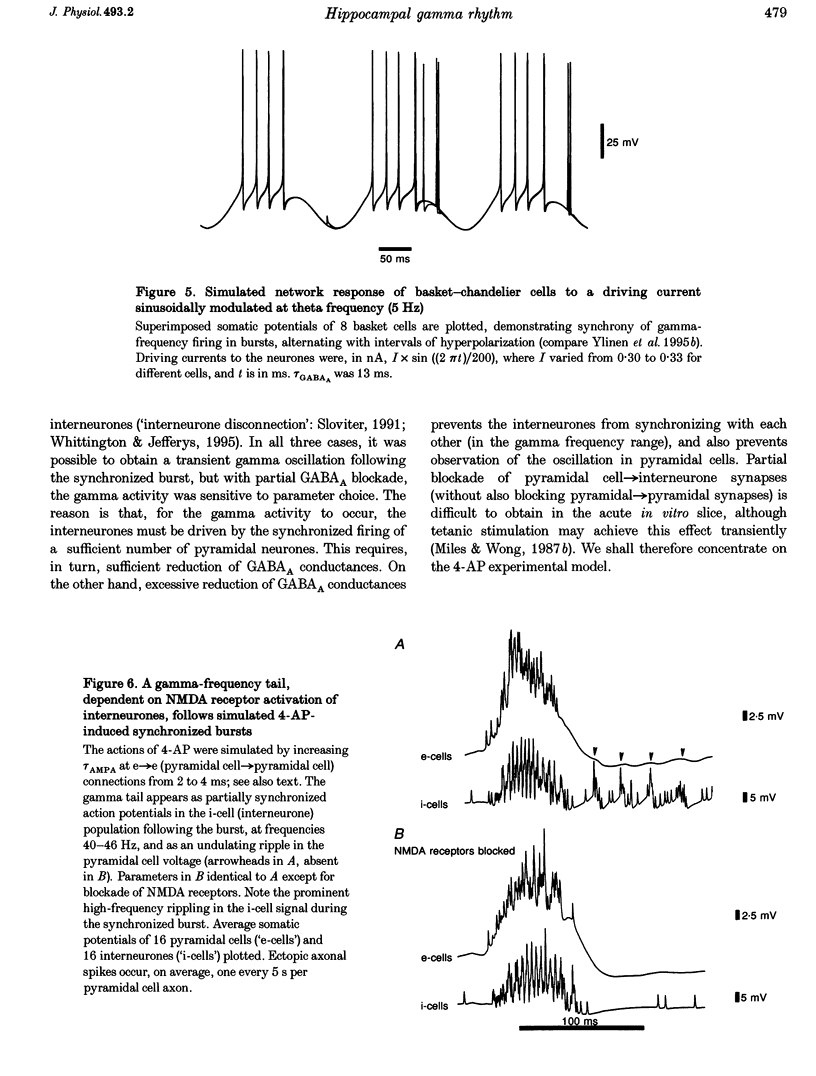
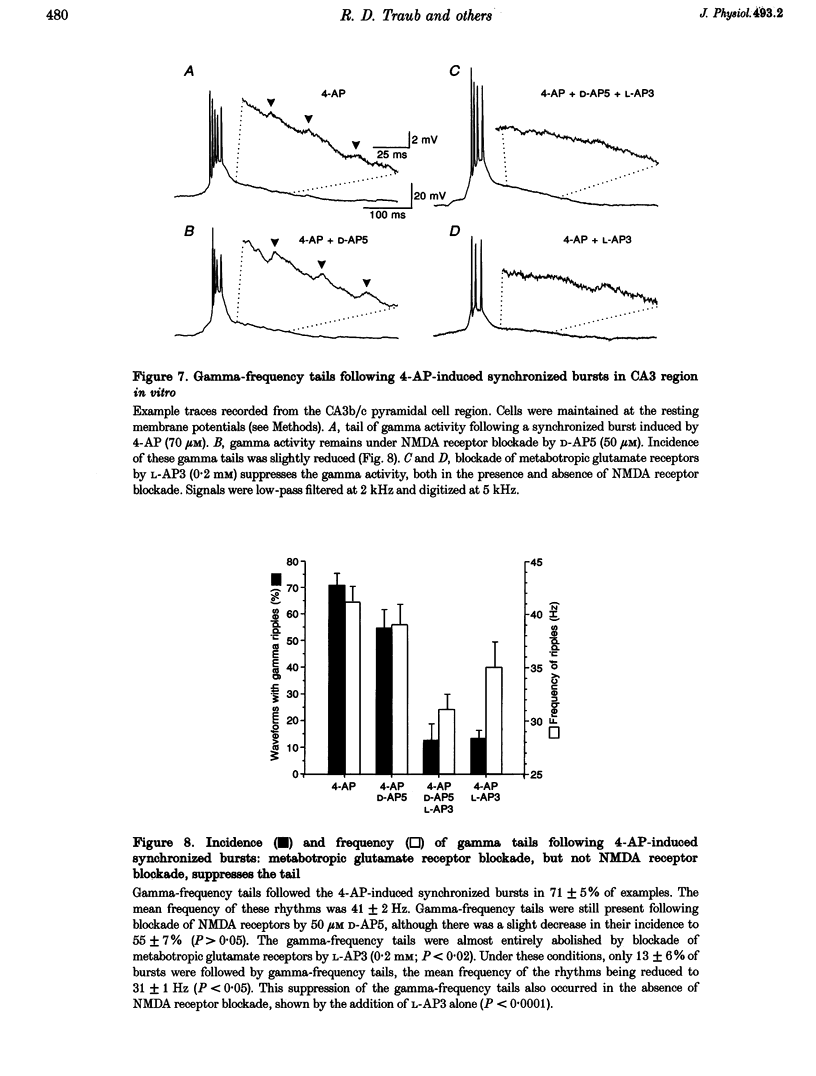
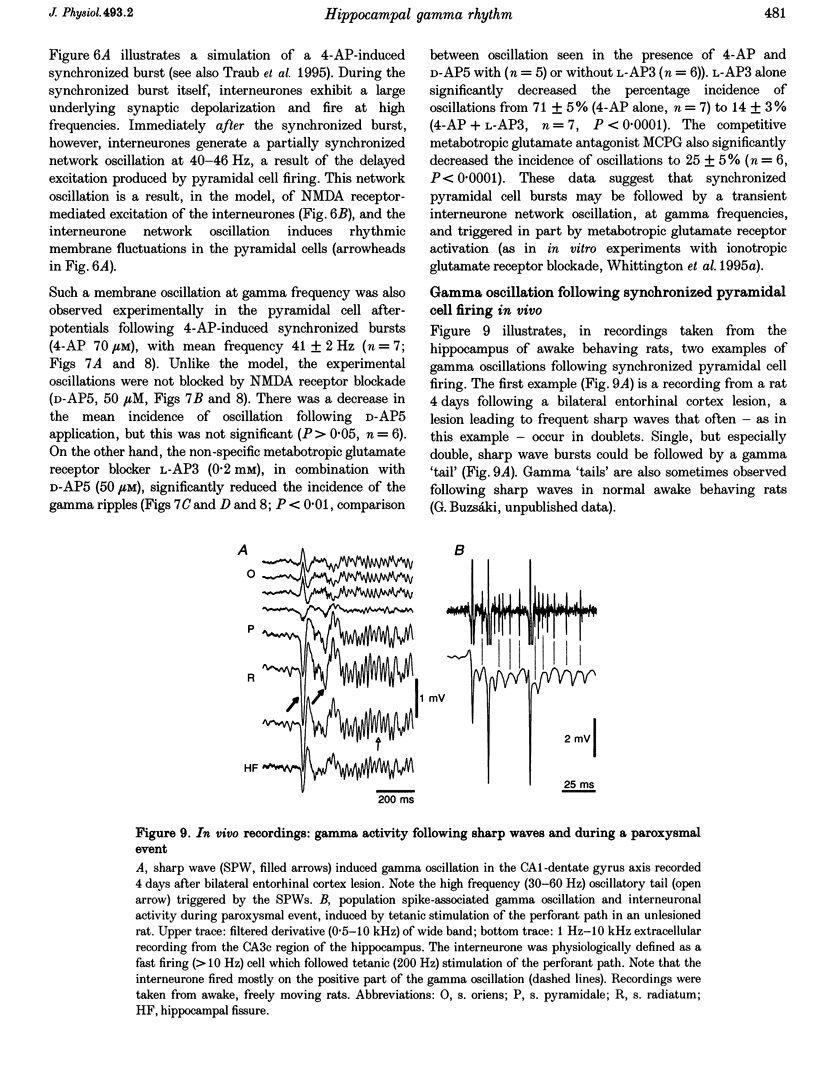
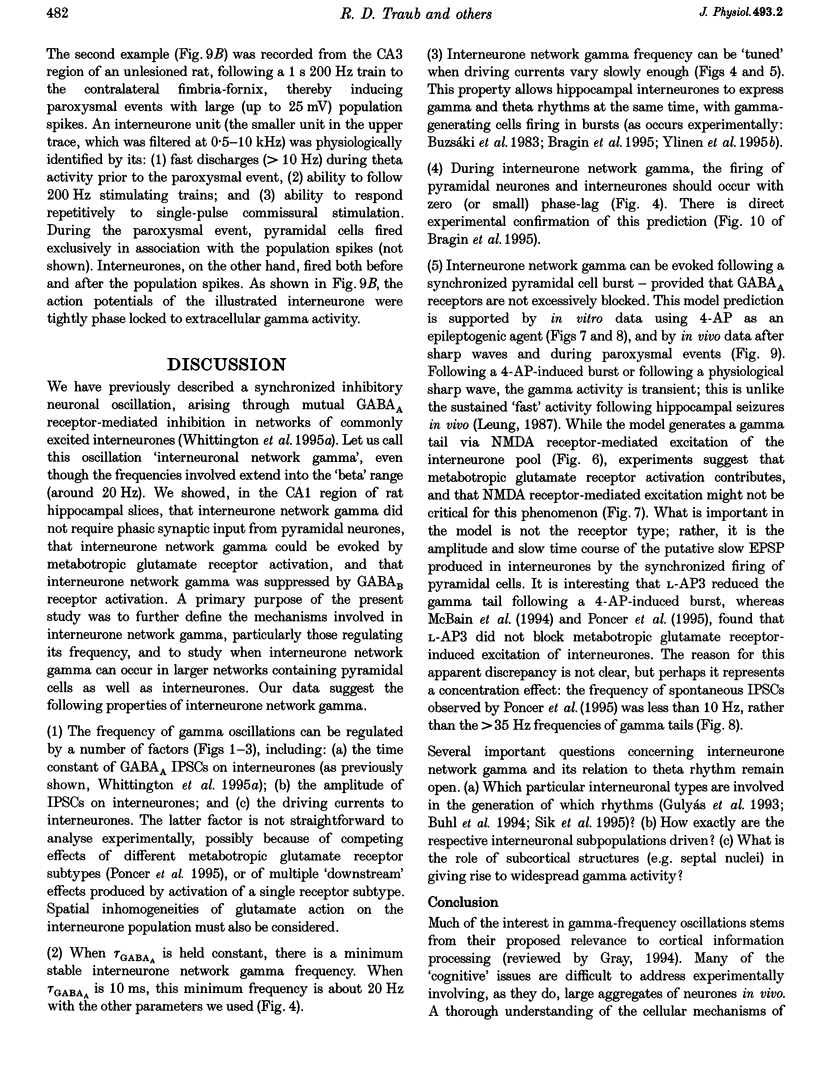
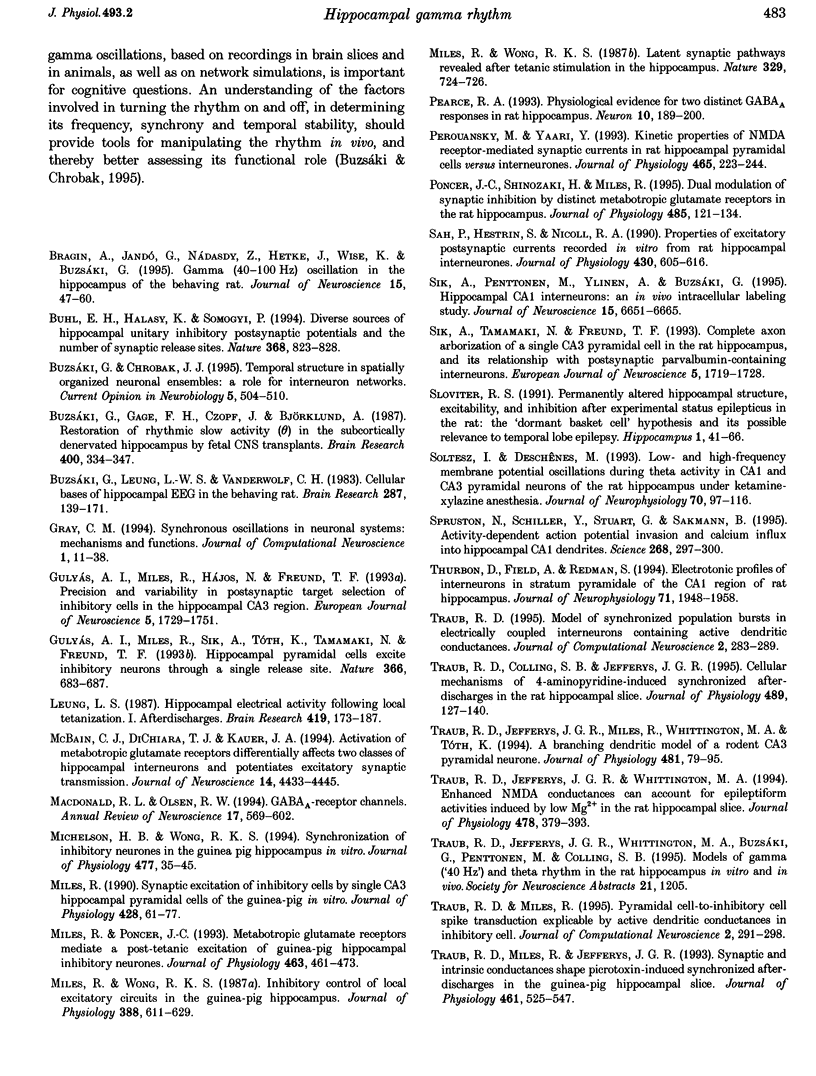
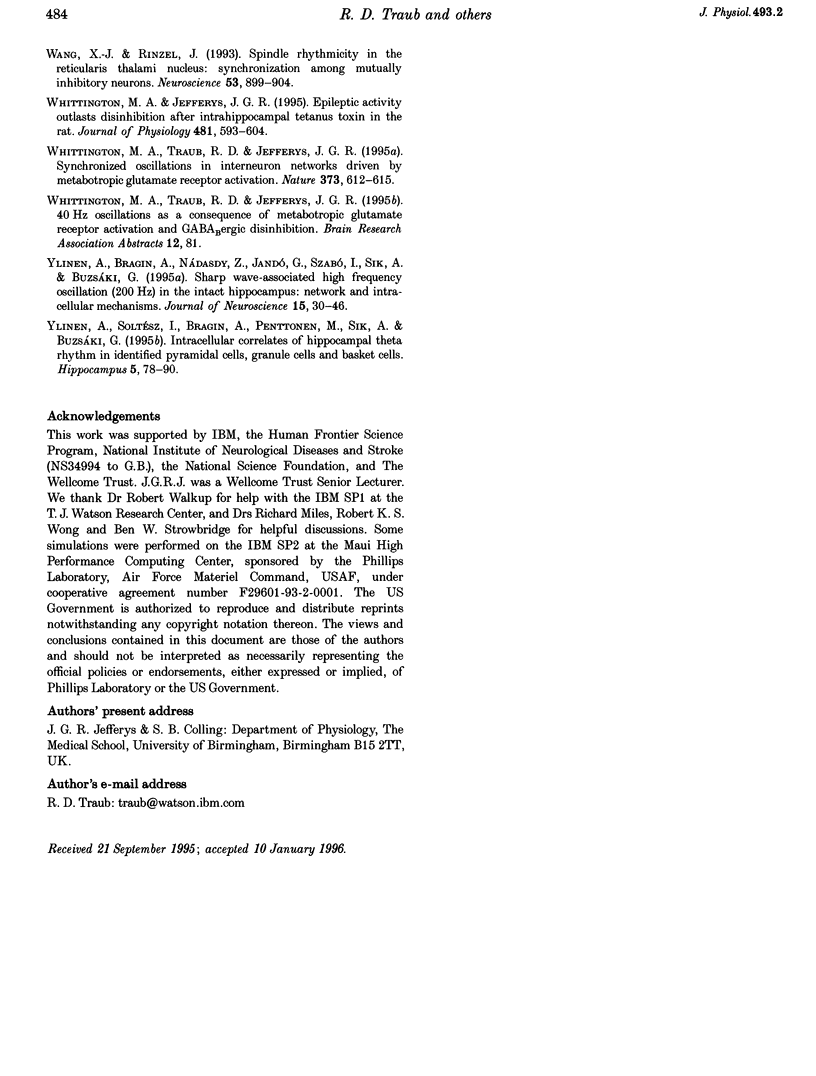
Images in this article
Selected References
These references are in PubMed. This may not be the complete list of references from this article.
- Bragin A., Jandó G., Nádasdy Z., Hetke J., Wise K., Buzsáki G. Gamma (40-100 Hz) oscillation in the hippocampus of the behaving rat. J Neurosci. 1995 Jan;15(1 Pt 1):47–60. doi: 10.1523/JNEUROSCI.15-01-00047.1995. [DOI] [PMC free article] [PubMed] [Google Scholar]
- Buhl E. H., Halasy K., Somogyi P. Diverse sources of hippocampal unitary inhibitory postsynaptic potentials and the number of synaptic release sites. Nature. 1994 Apr 28;368(6474):823–828. doi: 10.1038/368823a0. [DOI] [PubMed] [Google Scholar]
- Buzsáki G., Chrobak J. J. Temporal structure in spatially organized neuronal ensembles: a role for interneuronal networks. Curr Opin Neurobiol. 1995 Aug;5(4):504–510. doi: 10.1016/0959-4388(95)80012-3. [DOI] [PubMed] [Google Scholar]
- Buzsáki G., Gage F. H., Czopf J., Björklund A. Restoration of rhythmic slow activity (theta) in the subcortically denervated hippocampus by fetal CNS transplants. Brain Res. 1987 Jan 6;400(2):334–347. doi: 10.1016/0006-8993(87)90632-9. [DOI] [PubMed] [Google Scholar]
- Buzsáki G., Leung L. W., Vanderwolf C. H. Cellular bases of hippocampal EEG in the behaving rat. Brain Res. 1983 Oct;287(2):139–171. doi: 10.1016/0165-0173(83)90037-1. [DOI] [PubMed] [Google Scholar]
- Gray C. M. Synchronous oscillations in neuronal systems: mechanisms and functions. J Comput Neurosci. 1994 Jun;1(1-2):11–38. doi: 10.1007/BF00962716. [DOI] [PubMed] [Google Scholar]
- Gulyás A. I., Miles R., Hájos N., Freund T. F. Precision and variability in postsynaptic target selection of inhibitory cells in the hippocampal CA3 region. Eur J Neurosci. 1993 Dec 1;5(12):1729–1751. doi: 10.1111/j.1460-9568.1993.tb00240.x. [DOI] [PubMed] [Google Scholar]
- Gulyás A. I., Miles R., Sík A., Tóth K., Tamamaki N., Freund T. F. Hippocampal pyramidal cells excite inhibitory neurons through a single release site. Nature. 1993 Dec 16;366(6456):683–687. doi: 10.1038/366683a0. [DOI] [PubMed] [Google Scholar]
- Leung L. W. Hippocampal electrical activity following local tetanization. I. Afterdischarges. Brain Res. 1987 Sep 1;419(1-2):173–187. doi: 10.1016/0006-8993(87)90581-6. [DOI] [PubMed] [Google Scholar]
- Macdonald R. L., Olsen R. W. GABAA receptor channels. Annu Rev Neurosci. 1994;17:569–602. doi: 10.1146/annurev.ne.17.030194.003033. [DOI] [PubMed] [Google Scholar]
- McBain C. J., DiChiara T. J., Kauer J. A. Activation of metabotropic glutamate receptors differentially affects two classes of hippocampal interneurons and potentiates excitatory synaptic transmission. J Neurosci. 1994 Jul;14(7):4433–4445. doi: 10.1523/JNEUROSCI.14-07-04433.1994. [DOI] [PMC free article] [PubMed] [Google Scholar]
- Michelson H. B., Wong R. K. Synchronization of inhibitory neurones in the guinea-pig hippocampus in vitro. J Physiol. 1994 May 15;477(Pt 1):35–45. doi: 10.1113/jphysiol.1994.sp020169. [DOI] [PMC free article] [PubMed] [Google Scholar]
- Miles R., Poncer J. C. Metabotropic glutamate receptors mediate a post-tetanic excitation of guinea-pig hippocampal inhibitory neurones. J Physiol. 1993 Apr;463:461–473. doi: 10.1113/jphysiol.1993.sp019605. [DOI] [PMC free article] [PubMed] [Google Scholar]
- Miles R. Synaptic excitation of inhibitory cells by single CA3 hippocampal pyramidal cells of the guinea-pig in vitro. J Physiol. 1990 Sep;428:61–77. doi: 10.1113/jphysiol.1990.sp018200. [DOI] [PMC free article] [PubMed] [Google Scholar]
- Miles R., Wong R. K. Inhibitory control of local excitatory circuits in the guinea-pig hippocampus. J Physiol. 1987 Jul;388:611–629. doi: 10.1113/jphysiol.1987.sp016634. [DOI] [PMC free article] [PubMed] [Google Scholar]
- Miles R., Wong R. K. Latent synaptic pathways revealed after tetanic stimulation in the hippocampus. Nature. 1987 Oct 22;329(6141):724–726. doi: 10.1038/329724a0. [DOI] [PubMed] [Google Scholar]
- Pearce R. A. Physiological evidence for two distinct GABAA responses in rat hippocampus. Neuron. 1993 Feb;10(2):189–200. doi: 10.1016/0896-6273(93)90310-n. [DOI] [PubMed] [Google Scholar]
- Perouansky M., Yaari Y. Kinetic properties of NMDA receptor-mediated synaptic currents in rat hippocampal pyramidal cells versus interneurones. J Physiol. 1993 Jun;465:223–244. doi: 10.1113/jphysiol.1993.sp019674. [DOI] [PMC free article] [PubMed] [Google Scholar]
- Poncer J. C., Shinozaki H., Miles R. Dual modulation of synaptic inhibition by distinct metabotropic glutamate receptors in the rat hippocampus. J Physiol. 1995 May 15;485(Pt 1):121–134. doi: 10.1113/jphysiol.1995.sp020717. [DOI] [PMC free article] [PubMed] [Google Scholar]
- Sah P., Hestrin S., Nicoll R. A. Properties of excitatory postsynaptic currents recorded in vitro from rat hippocampal interneurones. J Physiol. 1990 Nov;430:605–616. doi: 10.1113/jphysiol.1990.sp018310. [DOI] [PMC free article] [PubMed] [Google Scholar]
- Sik A., Penttonen M., Ylinen A., Buzsáki G. Hippocampal CA1 interneurons: an in vivo intracellular labeling study. J Neurosci. 1995 Oct;15(10):6651–6665. doi: 10.1523/JNEUROSCI.15-10-06651.1995. [DOI] [PMC free article] [PubMed] [Google Scholar]
- Sik A., Tamamaki N., Freund T. F. Complete axon arborization of a single CA3 pyramidal cell in the rat hippocampus, and its relationship with postsynaptic parvalbumin-containing interneurons. Eur J Neurosci. 1993 Dec 1;5(12):1719–1728. doi: 10.1111/j.1460-9568.1993.tb00239.x. [DOI] [PubMed] [Google Scholar]
- Sloviter R. S. Permanently altered hippocampal structure, excitability, and inhibition after experimental status epilepticus in the rat: the "dormant basket cell" hypothesis and its possible relevance to temporal lobe epilepsy. Hippocampus. 1991 Jan;1(1):41–66. doi: 10.1002/hipo.450010106. [DOI] [PubMed] [Google Scholar]
- Soltesz I., Deschênes M. Low- and high-frequency membrane potential oscillations during theta activity in CA1 and CA3 pyramidal neurons of the rat hippocampus under ketamine-xylazine anesthesia. J Neurophysiol. 1993 Jul;70(1):97–116. doi: 10.1152/jn.1993.70.1.97. [DOI] [PubMed] [Google Scholar]
- Spruston N., Schiller Y., Stuart G., Sakmann B. Activity-dependent action potential invasion and calcium influx into hippocampal CA1 dendrites. Science. 1995 Apr 14;268(5208):297–300. doi: 10.1126/science.7716524. [DOI] [PubMed] [Google Scholar]
- Thurbon D., Field A., Redman S. Electrotonic profiles of interneurons in stratum pyramidale of the CA1 region of rat hippocampus. J Neurophysiol. 1994 May;71(5):1948–1958. doi: 10.1152/jn.1994.71.5.1948. [DOI] [PubMed] [Google Scholar]
- Traub R. D., Colling S. B., Jefferys J. G. Cellular mechanisms of 4-aminopyridine-induced synchronized after-discharges in the rat hippocampal slice. J Physiol. 1995 Nov 15;489(Pt 1):127–140. doi: 10.1113/jphysiol.1995.sp021036. [DOI] [PMC free article] [PubMed] [Google Scholar]
- Traub R. D., Jefferys J. G., Miles R., Whittington M. A., Tóth K. A branching dendritic model of a rodent CA3 pyramidal neurone. J Physiol. 1994 Nov 15;481(Pt 1):79–95. doi: 10.1113/jphysiol.1994.sp020420. [DOI] [PMC free article] [PubMed] [Google Scholar]
- Traub R. D., Jefferys J. G., Whittington M. A. Enhanced NMDA conductance can account for epileptiform activity induced by low Mg2+ in the rat hippocampal slice. J Physiol. 1994 Aug 1;478(Pt 3):379–393. doi: 10.1113/jphysiol.1994.sp020259. [DOI] [PMC free article] [PubMed] [Google Scholar]
- Traub R. D., Miles R., Jefferys J. G. Synaptic and intrinsic conductances shape picrotoxin-induced synchronized after-discharges in the guinea-pig hippocampal slice. J Physiol. 1993 Feb;461:525–547. doi: 10.1113/jphysiol.1993.sp019527. [DOI] [PMC free article] [PubMed] [Google Scholar]
- Traub R. D., Miles R. Pyramidal cell-to-inhibitory cell spike transduction explicable by active dendritic conductances in inhibitory cell. J Comput Neurosci. 1995 Dec;2(4):291–298. doi: 10.1007/BF00961441. [DOI] [PubMed] [Google Scholar]
- Traub R. D. Model of synchronized population bursts in electrically coupled interneurons containing active dendritic conductances. J Comput Neurosci. 1995 Dec;2(4):283–289. doi: 10.1007/BF00961440. [DOI] [PubMed] [Google Scholar]
- Wang X. J., Rinzel J. Spindle rhythmicity in the reticularis thalami nucleus: synchronization among mutually inhibitory neurons. Neuroscience. 1993 Apr;53(4):899–904. doi: 10.1016/0306-4522(93)90474-t. [DOI] [PubMed] [Google Scholar]
- Whittington M. A., Jefferys J. G. Epileptic activity outlasts disinhibition after intrahippocampal tetanus toxin in the rat. J Physiol. 1994 Dec 15;481(Pt 3):593–604. doi: 10.1113/jphysiol.1994.sp020466. [DOI] [PMC free article] [PubMed] [Google Scholar]
- Whittington M. A., Traub R. D., Jefferys J. G. Synchronized oscillations in interneuron networks driven by metabotropic glutamate receptor activation. Nature. 1995 Feb 16;373(6515):612–615. doi: 10.1038/373612a0. [DOI] [PubMed] [Google Scholar]
- Ylinen A., Bragin A., Nádasdy Z., Jandó G., Szabó I., Sik A., Buzsáki G. Sharp wave-associated high-frequency oscillation (200 Hz) in the intact hippocampus: network and intracellular mechanisms. J Neurosci. 1995 Jan;15(1 Pt 1):30–46. doi: 10.1523/JNEUROSCI.15-01-00030.1995. [DOI] [PMC free article] [PubMed] [Google Scholar]
- Ylinen A., Soltész I., Bragin A., Penttonen M., Sik A., Buzsáki G. Intracellular correlates of hippocampal theta rhythm in identified pyramidal cells, granule cells, and basket cells. Hippocampus. 1995;5(1):78–90. doi: 10.1002/hipo.450050110. [DOI] [PubMed] [Google Scholar]