Abstract
1. Membrane voltage (Vm) recordings were obtained from isolated rat pinealocytes using the patch-clamp technique. In parallel to the electrophysiological experiments, intracellular Ca2+ measurements were performed using fura-2. 2. The resting Vm averaged -43 mV and replacement of extracellular NaCl by KCl completely depolarized the cells. This indicates that the resting Vm is dominated by a K+ conductance. Single-channel recordings revealed the presence of a large conductance Ca(2+)-activated charybdotoxin-sensitive K+ channel. 3. Application of ACh (100 microM) depolarized the pinealocytes on average by 16 mV. The depolarizing effect of ACh was mimicked by nicotine (50 microM) and was prevented by tubocurarine (100 microM). 4. The ACh-induced depolarization was largely abolished in the absence of extracellular Na+, but was not significantly affected by extracellular Ca2+ removal. 5. Application of ACh (100 microM) caused an increase in [Ca2+]i. This increase was completely dependent on the presence of extracellular Ca2+ and was largely reduced after extracellular Na+ removal. Nifedipine (1 microM) reduced the ACh-induced increase in [Ca2+]i by about 50%. 6. Our findings indicate that in rat pinealocytes stimulation of a nicotinic ACh receptor (nAChR) induces depolarization mainly by Na+ influx via the nAChR. The depolarization then activates L-type Ca2+ channels, which are responsible for the nifedipine-sensitive portion of the intracellular Ca2+ increase. Ca2+ influx via the nAChR probably also contributes to the observed rise in [Ca2+]i.
Full text
PDF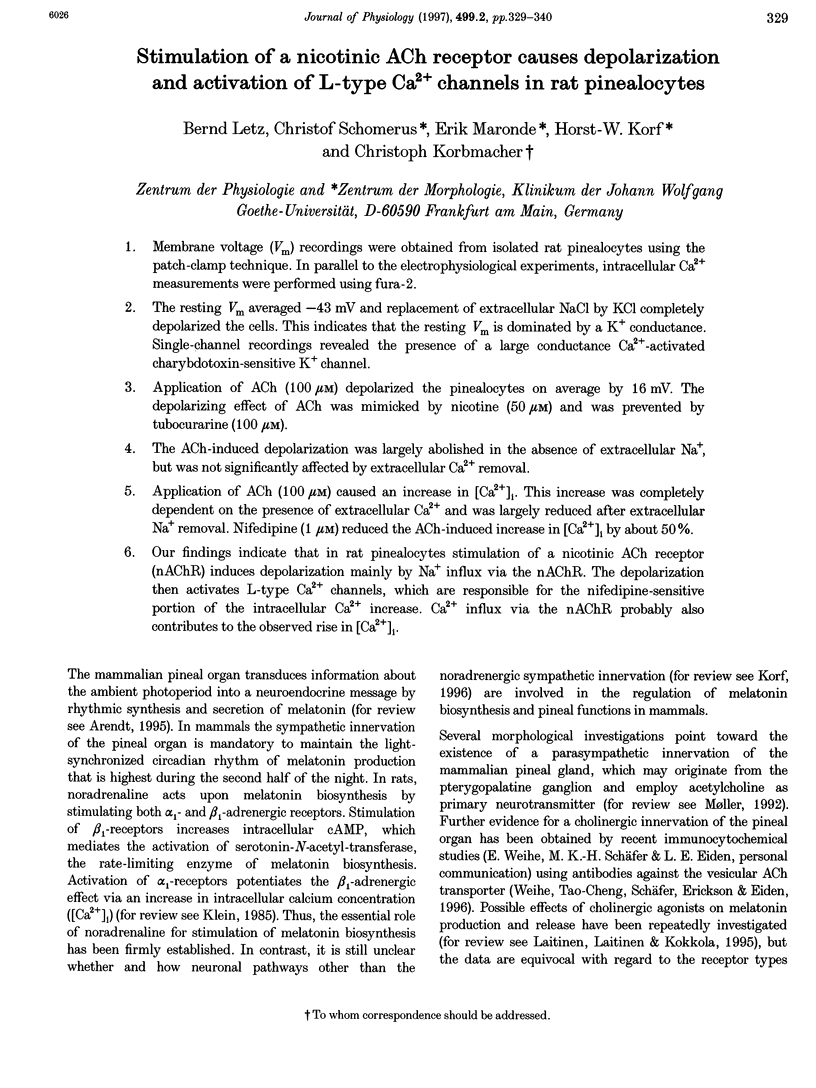
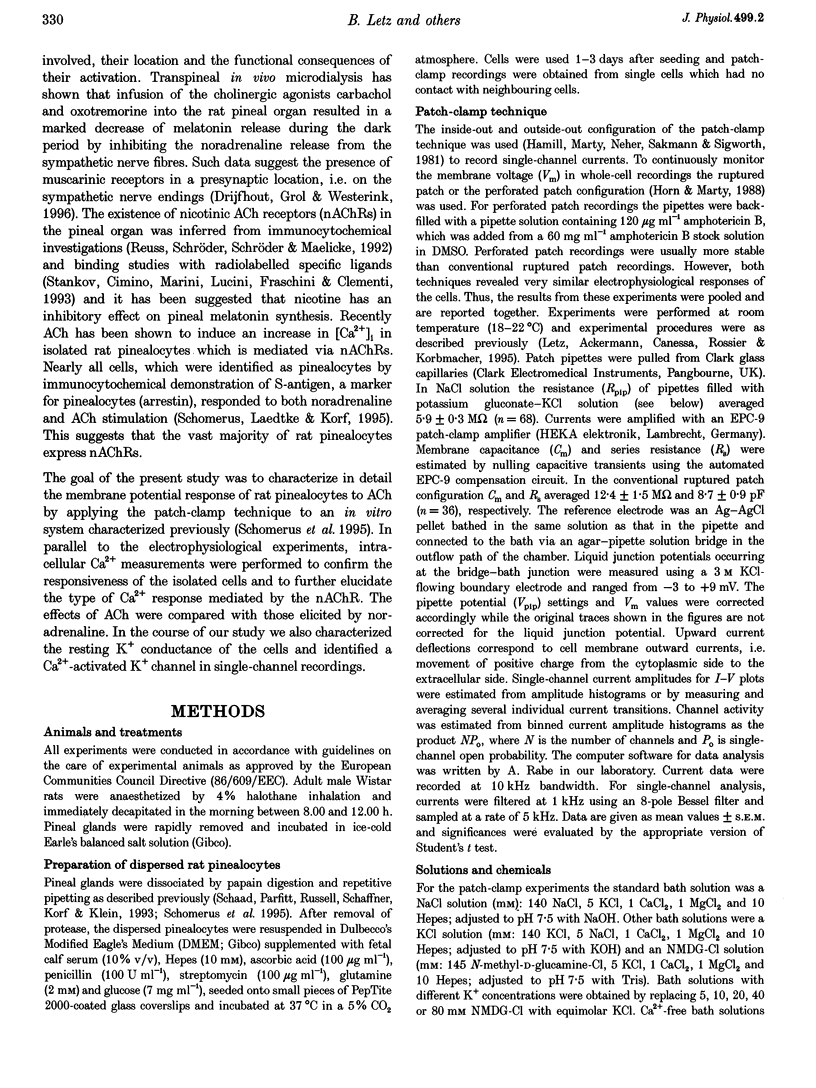
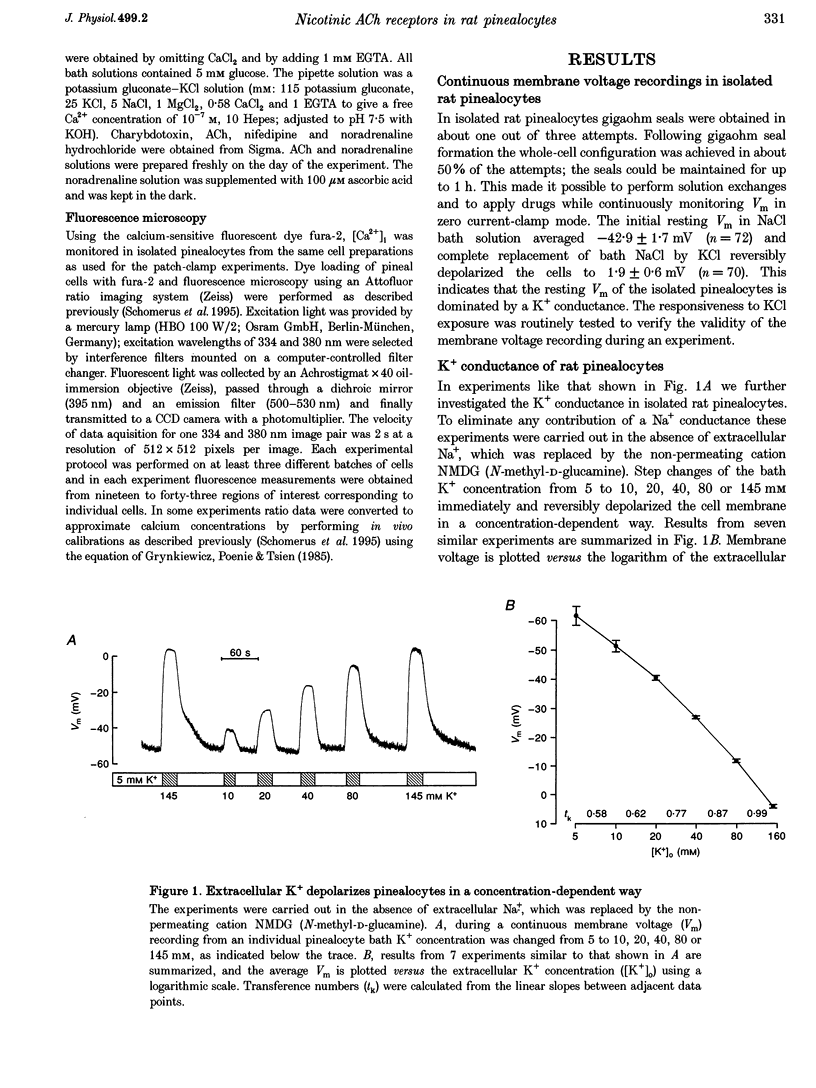
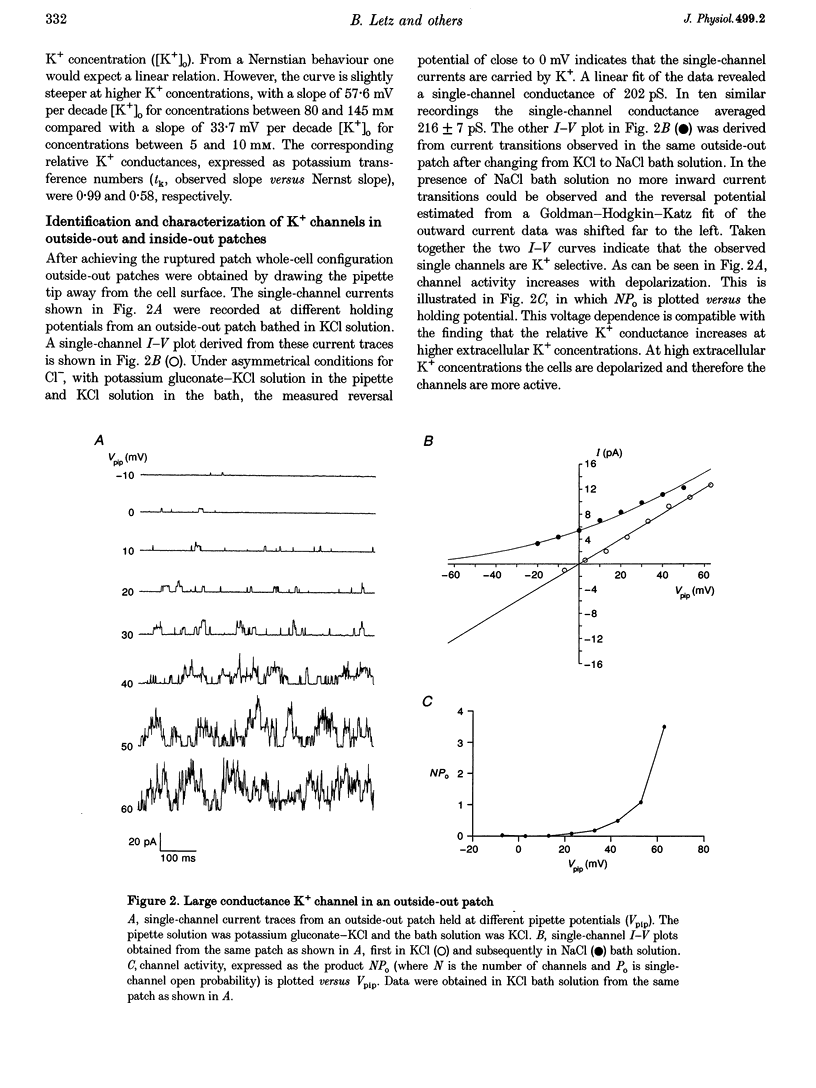
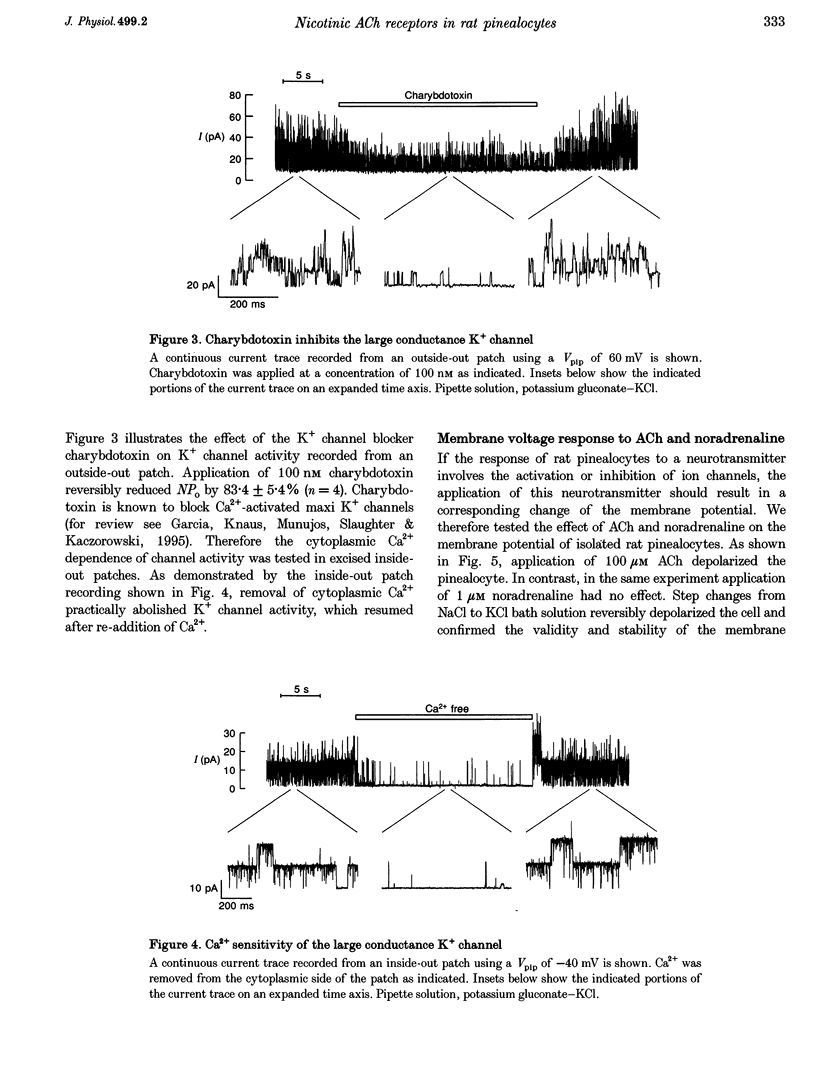
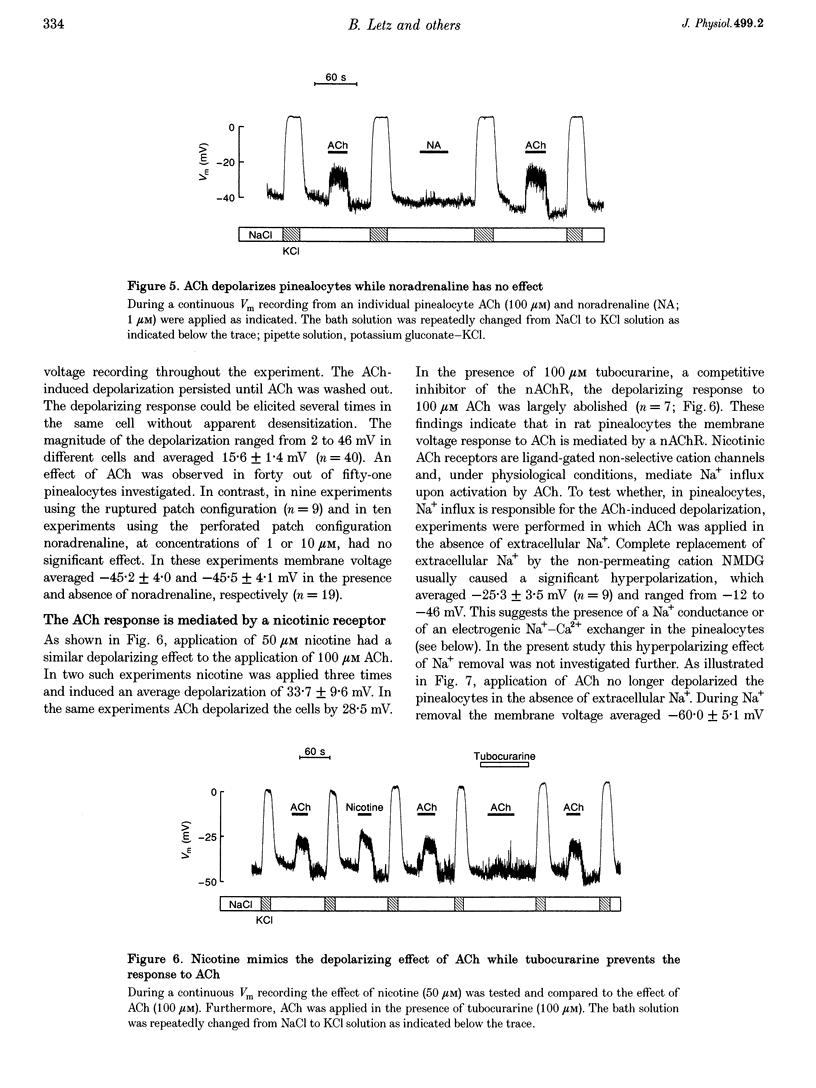
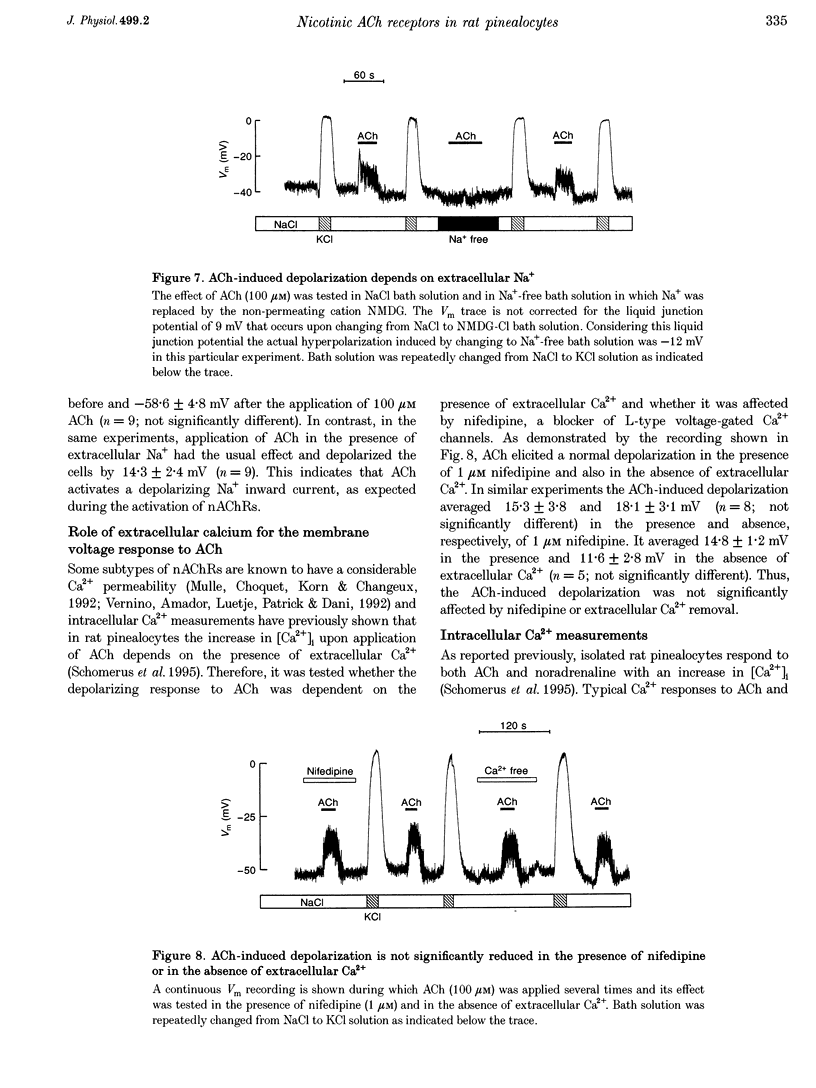
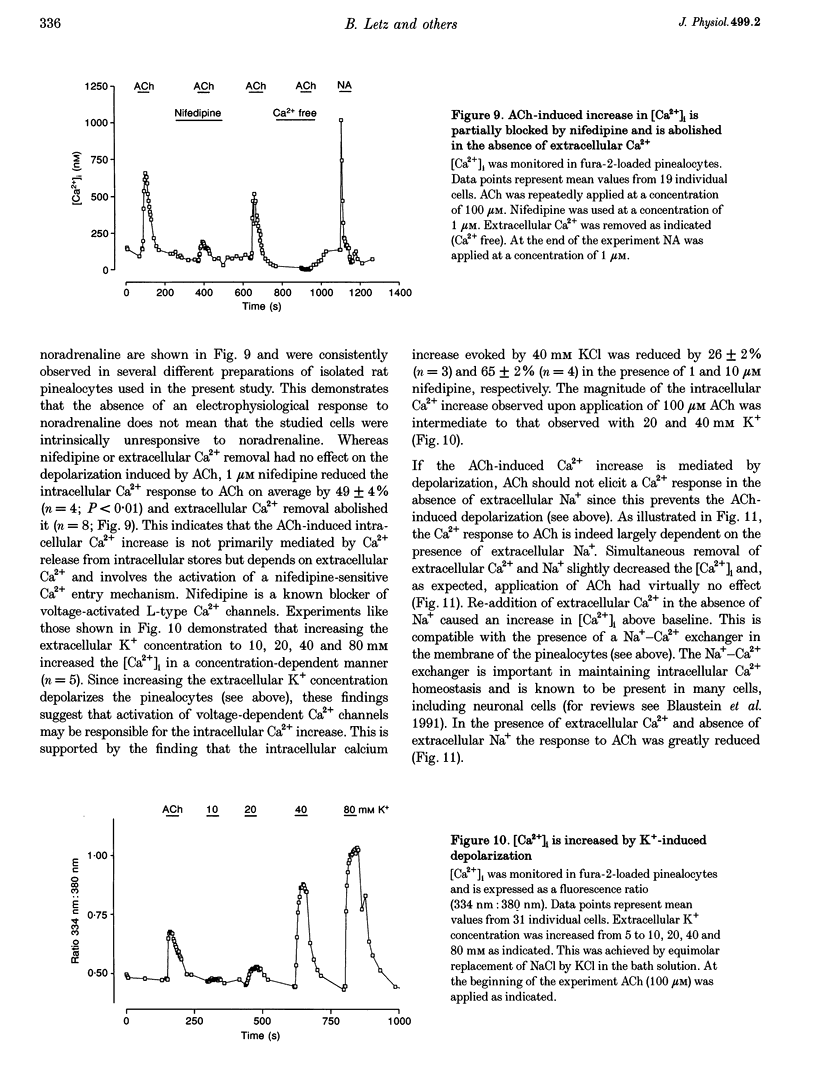
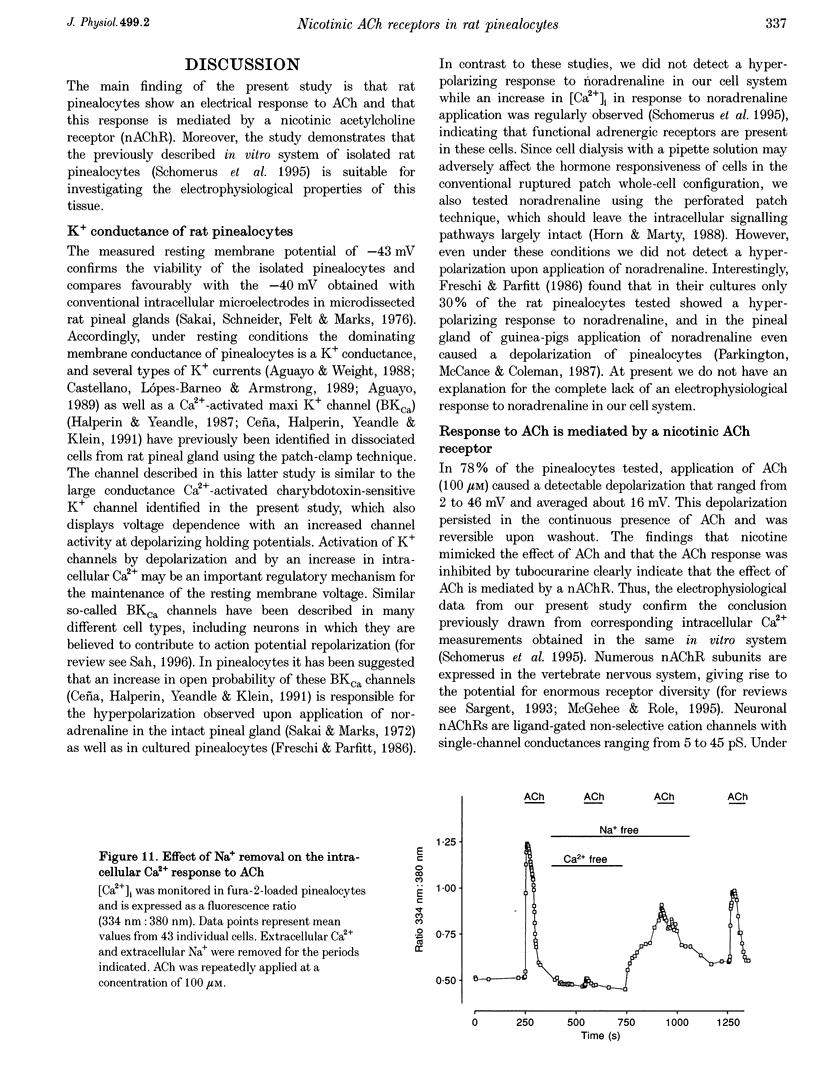
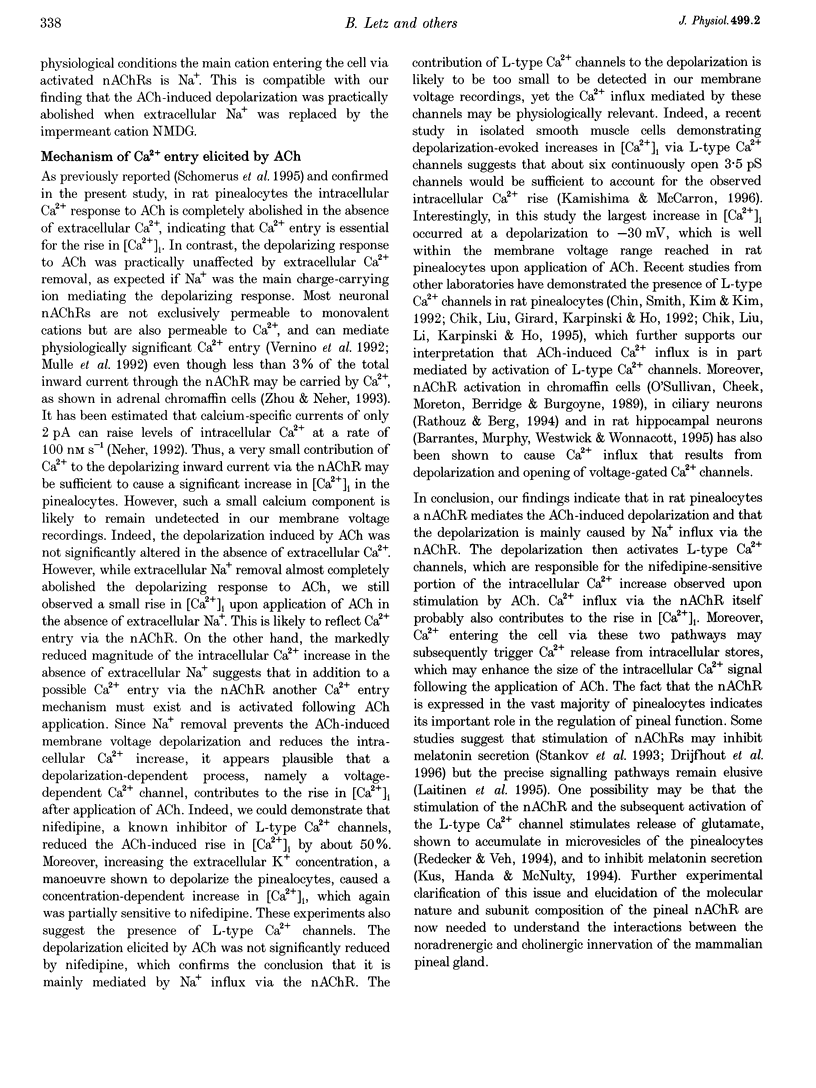
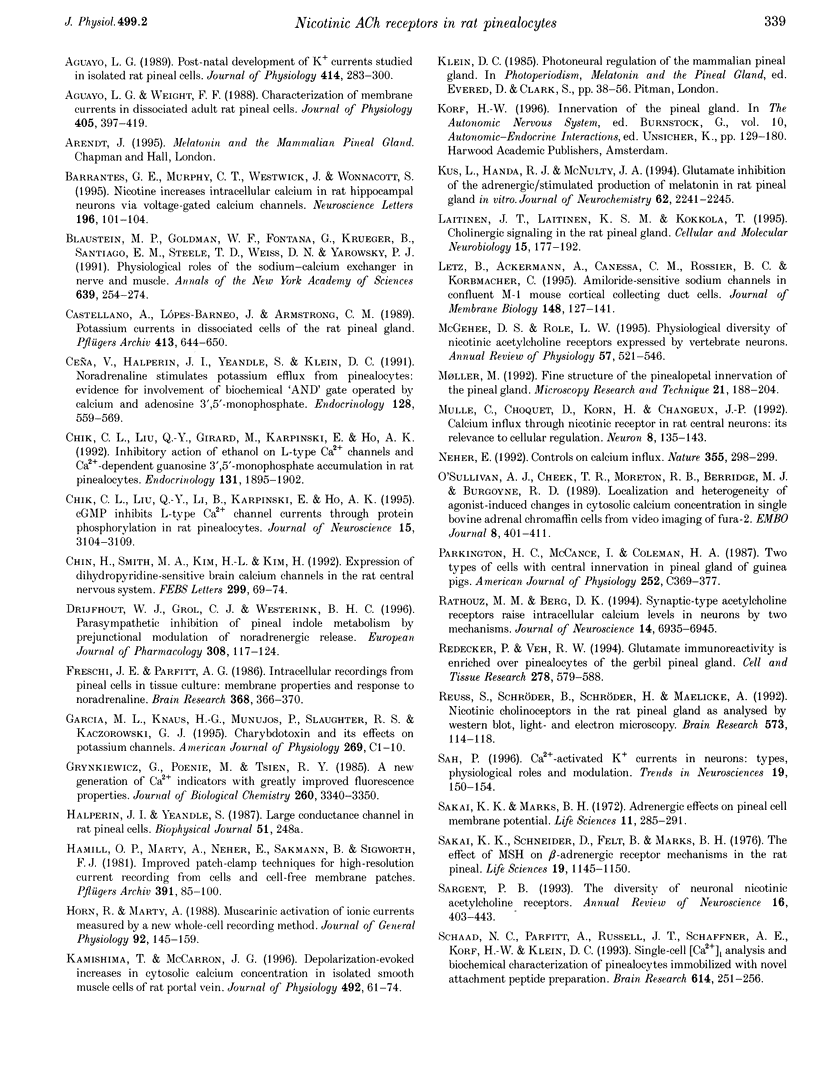
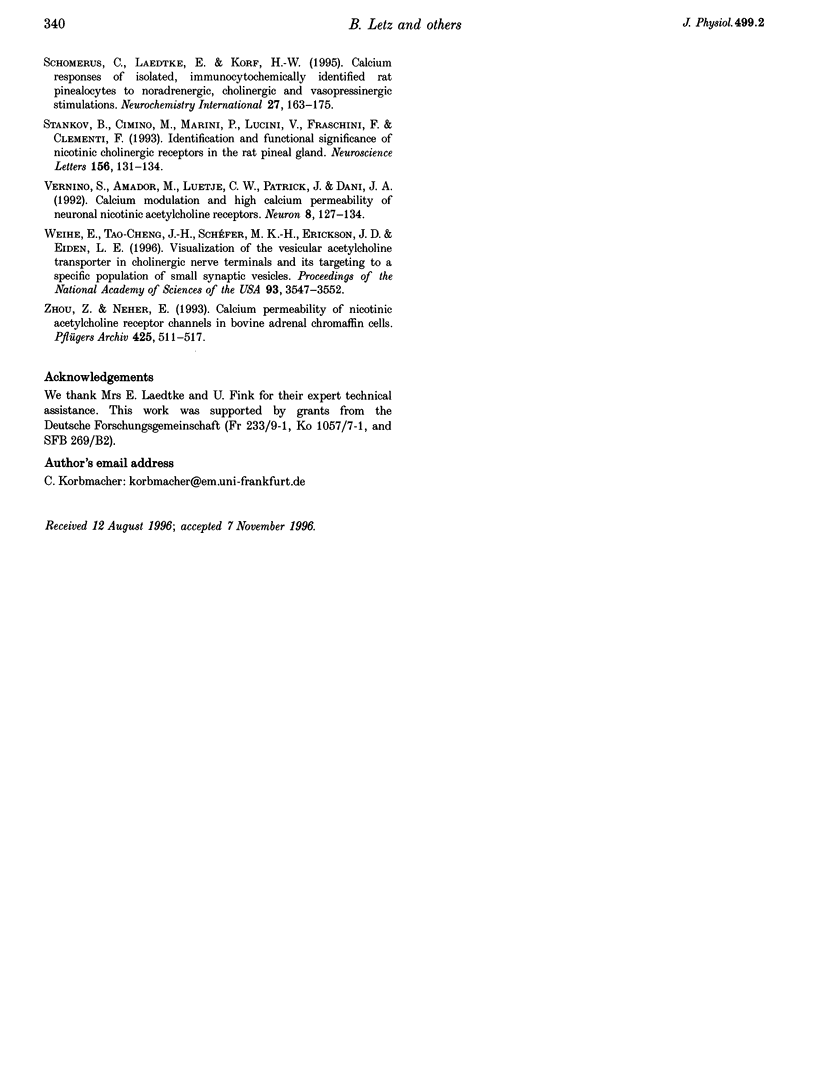
Selected References
These references are in PubMed. This may not be the complete list of references from this article.
- Aguayo L. G. Post-natal development of K+ currents studied in isolated rat pineal cells. J Physiol. 1989 Jul;414:283–300. doi: 10.1113/jphysiol.1989.sp017688. [DOI] [PMC free article] [PubMed] [Google Scholar]
- Aguayo L. G., Weight F. F. Characterization of membrane currents in dissociated adult rat pineal cells. J Physiol. 1988 Nov;405:397–419. doi: 10.1113/jphysiol.1988.sp017339. [DOI] [PMC free article] [PubMed] [Google Scholar]
- Barrantes G. E., Murphy C. T., Westwick J., Wonnacott S. Nicotine increases intracellular calcium in rat hippocampal neurons via voltage-gated calcium channels. Neurosci Lett. 1995 Aug 18;196(1-2):101–104. doi: 10.1016/0304-3940(95)11859-u. [DOI] [PubMed] [Google Scholar]
- Blaustein M. P., Goldman W. F., Fontana G., Krueger B. K., Santiago E. M., Steele T. D., Weiss D. N., Yarowsky P. J. Physiological roles of the sodium-calcium exchanger in nerve and muscle. Ann N Y Acad Sci. 1991;639:254–274. doi: 10.1111/j.1749-6632.1991.tb17315.x. [DOI] [PubMed] [Google Scholar]
- Castellano A., López-Barneo J., Armstrong C. M. Potassium currents in dissociated cells of the rat pineal gland. Pflugers Arch. 1989 Apr;413(6):644–650. doi: 10.1007/BF00581815. [DOI] [PubMed] [Google Scholar]
- Ceña V., Halperin J. I., Yeandle S., Klein D. C. Norepinephrine stimulates potassium efflux from pinealocytes: evidence for involvement of biochemical "AND" gate operated by calcium and adenosine 3',5'-monophosphate. Endocrinology. 1991 Jan;128(1):559–569. doi: 10.1210/endo-128-1-559. [DOI] [PubMed] [Google Scholar]
- Chik C. L., Liu Q. Y., Girard M., Karpinski E., Ho A. K. Inhibitory action of ethanol on L-type Ca2+ channels and Ca(2+)-dependent guanosine 3',5'-monophosphate accumulation in rat pinealocytes. Endocrinology. 1992 Oct;131(4):1895–1902. doi: 10.1210/endo.131.4.1327722. [DOI] [PubMed] [Google Scholar]
- Chik C. L., Liu Q. Y., Li B., Karpinski E., Ho A. K. cGMP inhibits L-type Ca2+ channel currents through protein phosphorylation in rat pinealocytes. J Neurosci. 1995 Apr;15(4):3104–3109. doi: 10.1523/JNEUROSCI.15-04-03104.1995. [DOI] [PMC free article] [PubMed] [Google Scholar]
- Chin H., Smith M. A., Kim H. L., Kim H. Expression of dihydropyridine-sensitive brain calcium channels in the rat central nervous system. FEBS Lett. 1992 Mar 24;299(1):69–74. doi: 10.1016/0014-5793(92)80103-n. [DOI] [PubMed] [Google Scholar]
- Freschi J. E., Parfitt A. G. Intracellular recordings from pineal cells in tissue culture: membrane properties and response to norepinephrine. Brain Res. 1986 Mar 19;368(2):366–370. doi: 10.1016/0006-8993(86)90583-4. [DOI] [PubMed] [Google Scholar]
- Garcia M. L., Knaus H. G., Munujos P., Slaughter R. S., Kaczorowski G. J. Charybdotoxin and its effects on potassium channels. Am J Physiol. 1995 Jul;269(1 Pt 1):C1–10. doi: 10.1152/ajpcell.1995.269.1.C1. [DOI] [PubMed] [Google Scholar]
- Hamill O. P., Marty A., Neher E., Sakmann B., Sigworth F. J. Improved patch-clamp techniques for high-resolution current recording from cells and cell-free membrane patches. Pflugers Arch. 1981 Aug;391(2):85–100. doi: 10.1007/BF00656997. [DOI] [PubMed] [Google Scholar]
- Horn R., Marty A. Muscarinic activation of ionic currents measured by a new whole-cell recording method. J Gen Physiol. 1988 Aug;92(2):145–159. doi: 10.1085/jgp.92.2.145. [DOI] [PMC free article] [PubMed] [Google Scholar]
- Kamishima T., McCarron J. G. Depolarization-evoked increases in cytosolic calcium concentration in isolated smooth muscle cells of rat portal vein. J Physiol. 1996 Apr 1;492(Pt 1):61–74. doi: 10.1113/jphysiol.1996.sp021289. [DOI] [PMC free article] [PubMed] [Google Scholar]
- Kus L., Handa R. J., McNulty J. A. Glutamate inhibition of the adrenergic-stimulated production of melatonin in rat pineal gland in vitro. J Neurochem. 1994 Jun;62(6):2241–2245. doi: 10.1046/j.1471-4159.1994.62062241.x. [DOI] [PubMed] [Google Scholar]
- Laitinen J. T., Laitinen K. S., Kokkola T. Cholinergic signaling in the rat pineal gland. Cell Mol Neurobiol. 1995 Apr;15(2):177–192. doi: 10.1007/BF02073327. [DOI] [PubMed] [Google Scholar]
- Letz B., Ackermann A., Canessa C. M., Rossier B. C., Korbmacher C. Amiloride-sensitive sodium channels in confluent M-1 mouse cortical collecting duct cells. J Membr Biol. 1995 Nov;148(2):127–141. doi: 10.1007/BF00207269. [DOI] [PubMed] [Google Scholar]
- McGehee D. S., Role L. W. Physiological diversity of nicotinic acetylcholine receptors expressed by vertebrate neurons. Annu Rev Physiol. 1995;57:521–546. doi: 10.1146/annurev.ph.57.030195.002513. [DOI] [PubMed] [Google Scholar]
- Mulle C., Choquet D., Korn H., Changeux J. P. Calcium influx through nicotinic receptor in rat central neurons: its relevance to cellular regulation. Neuron. 1992 Jan;8(1):135–143. doi: 10.1016/0896-6273(92)90115-t. [DOI] [PubMed] [Google Scholar]
- Møller M. Fine structure of the pinealopetal innervation of the mammalian pineal gland. Microsc Res Tech. 1992 May 1;21(3):188–204. doi: 10.1002/jemt.1070210303. [DOI] [PubMed] [Google Scholar]
- Neher E. Cell physiology. Controls on calcium influx. Nature. 1992 Jan 23;355(6358):298–299. doi: 10.1038/355298a0. [DOI] [PubMed] [Google Scholar]
- O'Sullivan A. J., Cheek T. R., Moreton R. B., Berridge M. J., Burgoyne R. D. Localization and heterogeneity of agonist-induced changes in cytosolic calcium concentration in single bovine adrenal chromaffin cells from video imaging of fura-2. EMBO J. 1989 Feb;8(2):401–411. doi: 10.1002/j.1460-2075.1989.tb03391.x. [DOI] [PMC free article] [PubMed] [Google Scholar]
- Parkington H. C., McCance I., Coleman H. A. Two types of cells with central innervation in pineal gland of guinea pigs. Am J Physiol. 1987 Apr;252(4 Pt 1):C369–C377. doi: 10.1152/ajpcell.1987.252.4.C369. [DOI] [PubMed] [Google Scholar]
- Rathouz M. M., Berg D. K. Synaptic-type acetylcholine receptors raise intracellular calcium levels in neurons by two mechanisms. J Neurosci. 1994 Nov;14(11 Pt 2):6935–6945. doi: 10.1523/JNEUROSCI.14-11-06935.1994. [DOI] [PMC free article] [PubMed] [Google Scholar]
- Redecker P., Veh R. W. Glutamate immunoreactivity is enriched over pinealocytes of the gerbil pineal gland. Cell Tissue Res. 1994 Dec;278(3):579–588. doi: 10.1007/BF00331377. [DOI] [PubMed] [Google Scholar]
- Reuss S., Schröder B., Schröder H., Maelicke A. Nicotinic cholinoceptors in the rat pineal gland as analyzed by western blot, light- and electron microscopy. Brain Res. 1992 Feb 21;573(1):114–118. doi: 10.1016/0006-8993(92)90119-t. [DOI] [PubMed] [Google Scholar]
- Sah P. Ca(2+)-activated K+ currents in neurones: types, physiological roles and modulation. Trends Neurosci. 1996 Apr;19(4):150–154. doi: 10.1016/s0166-2236(96)80026-9. [DOI] [PubMed] [Google Scholar]
- Sakai K. K., Marks B. H. Adrenergic effects on pineal cell membrane potential. Life Sci I. 1972 Mar 15;11(6):285–291. doi: 10.1016/0024-3205(72)90231-7. [DOI] [PubMed] [Google Scholar]
- Sakai K. K., Schneider D., Felt B., Marks B. H. The effect of alphaMSH on beta-adrenergic receptor mechanisms in the rat pineal. Life Sci. 1976 Oct 15;19(8):1145–1150. doi: 10.1016/0024-3205(76)90249-6. [DOI] [PubMed] [Google Scholar]
- Sargent P. B. The diversity of neuronal nicotinic acetylcholine receptors. Annu Rev Neurosci. 1993;16:403–443. doi: 10.1146/annurev.ne.16.030193.002155. [DOI] [PubMed] [Google Scholar]
- Schaad N. C., Parfitt A., Russell J. T., Schaffner A. E., Korf H. W., Klein D. C. Single-cell [Ca2+]i analysis and biochemical characterization of pinealocytes immobilized with novel attachment peptide preparation. Brain Res. 1993 Jun 18;614(1-2):251–256. doi: 10.1016/0006-8993(93)91042-q. [DOI] [PubMed] [Google Scholar]
- Schomerus C., Laedtke E., Korf H. W. Calcium responses of isolated, immunocytochemically identified rat pinealocytes to noradrenergic, cholinergic and vasopressinergic stimulations. Neurochem Int. 1995 Aug;27(2):163–175. doi: 10.1016/0197-0186(95)00029-8. [DOI] [PubMed] [Google Scholar]
- Stankov B., Cimino M., Marini P., Lucini V., Fraschini F., Clementi F. Identification and functional significance of nicotinic cholinergic receptors in the rat pineal gland. Neurosci Lett. 1993 Jun 25;156(1-2):131–134. doi: 10.1016/0304-3940(93)90456-u. [DOI] [PubMed] [Google Scholar]
- Vernino S., Amador M., Luetje C. W., Patrick J., Dani J. A. Calcium modulation and high calcium permeability of neuronal nicotinic acetylcholine receptors. Neuron. 1992 Jan;8(1):127–134. doi: 10.1016/0896-6273(92)90114-s. [DOI] [PubMed] [Google Scholar]
- Weihe E., Tao-Cheng J. H., Schäfer M. K., Erickson J. D., Eiden L. E. Visualization of the vesicular acetylcholine transporter in cholinergic nerve terminals and its targeting to a specific population of small synaptic vesicles. Proc Natl Acad Sci U S A. 1996 Apr 16;93(8):3547–3552. doi: 10.1073/pnas.93.8.3547. [DOI] [PMC free article] [PubMed] [Google Scholar]
- Zhou Z., Neher E. Calcium permeability of nicotinic acetylcholine receptor channels in bovine adrenal chromaffin cells. Pflugers Arch. 1993 Dec;425(5-6):511–517. doi: 10.1007/BF00374879. [DOI] [PubMed] [Google Scholar]