Abstract
1. Intra- and extracellular recordings were made from in vitro preparations of the lobster (Homarus gammarus) stomatogastric nervous system to study the nature and origin of pacemaker-like activity in a primary mechanoreceptor neurone, the anterior gastric receptor (AGR), whose two bilateral stretch-sensitive dendrites ramify in the tendon of powerstroke muscle GM1 of the gastric mill system. 2. Although the AGR is known to be autoactive, we report here that in 20% of our preparations, rather than autogenic tonic discharge, the receptor fired spontaneously in discrete bursts comprising three to ten action potentials and repeating at cycle frequencies of 0.5-2.5 Hz in the absence of mechanical stimulation. Intrasomatic recordings revealed that such rhythmic bursting was driven by slow oscillations in membrane potential, the frequency of which was voltage sensitive and dependent upon the level of stretch applied to the receptor terminals of the AGR. 3. Autoactive bursting of the AGR originated from an endogenous oscillatory mechanism in the sensory dendrites themselves, since (i) during both steady, repetitive firing and bursting, somatic and axonal impulses were always preceded 1:1 by dendritic action potentials, (ii) hyperpolarizing the AGR cell body to block triggering of axonal impulses revealed attenuated somatic spikes that continued to originate from the two peripheral dendrites, (iii) the timing of burst firing could be phase reset by brief electrical stimulation of either dendrite, and (iv) spontaneous bursting continued to be expressed by an AGR dendrite after physical isolation from the GM1 muscle and the stomatogastric nervous system. 4. Although a given AGR in vitro could switch spontaneously from dendritic bursting to tonic firing and vice versa, exogenous application of micromolar (or less) concentrations of the neuropeptide F1 (TNRNFLRFamide) to the dendritic membrane could rapidly and reversibly switch the receptor firing pattern from repetitive firing to the bursting mode. Exposure of the somatic and axonal membrane of the AGR to F1 was without effect, as were applications of other neuroactive substances such as serotonin, octopamine and proctolin. 5. We conclude that, as for many oscillatory neurones of the central nervous system, the intrinsic activity pattern of this peripheral sensory neurone may be dynamically conferred by extrinsic modulatory influences, presumably according to computational demands. Moreover, the ability of the AGR to behave as an endogenous burster imparts considerable integrative complexity since, in this activity mode, sensory coding not only occurs through the frequency modulation of on-going dendritic bursts but also via changes in the duration of individual bursts and their inherent spike frequencies.
Full text
PDF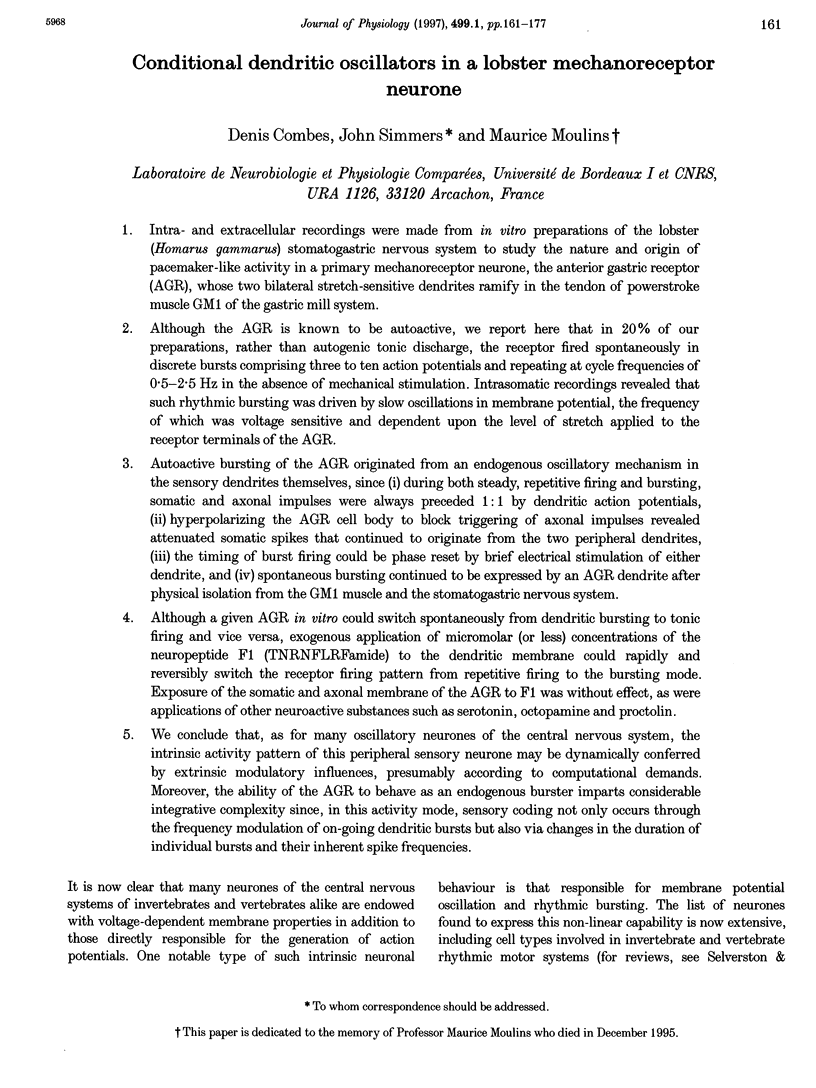
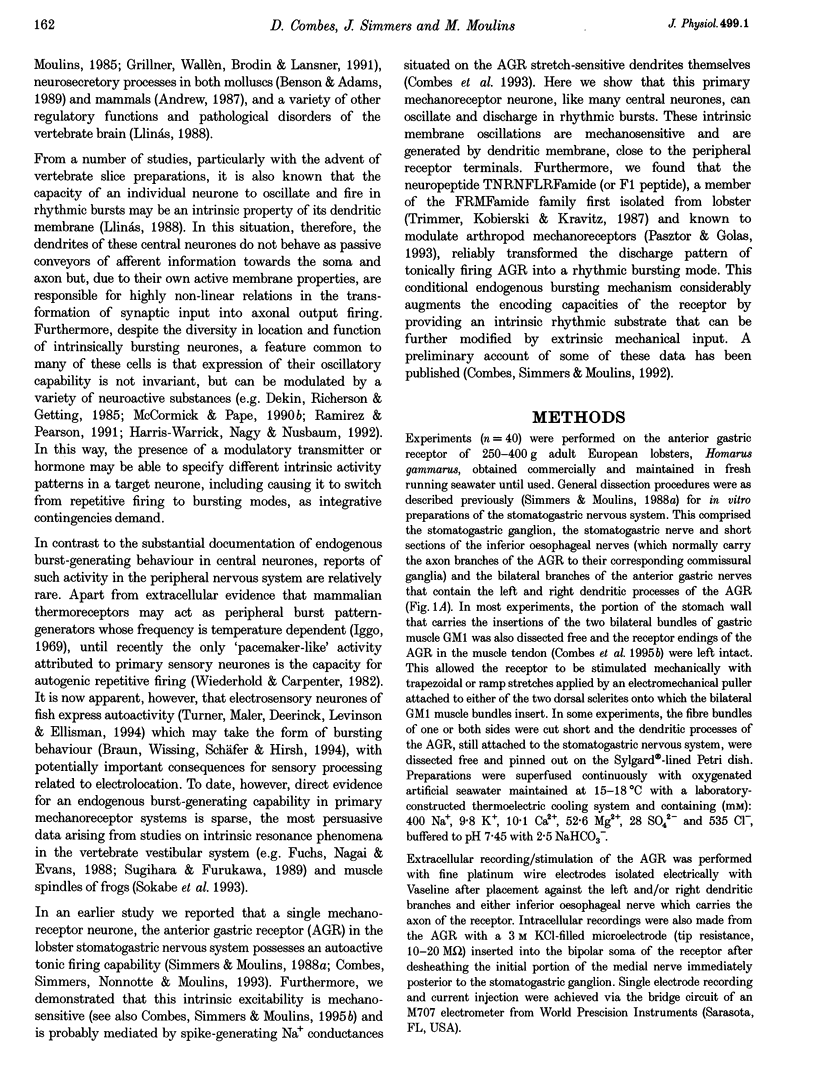
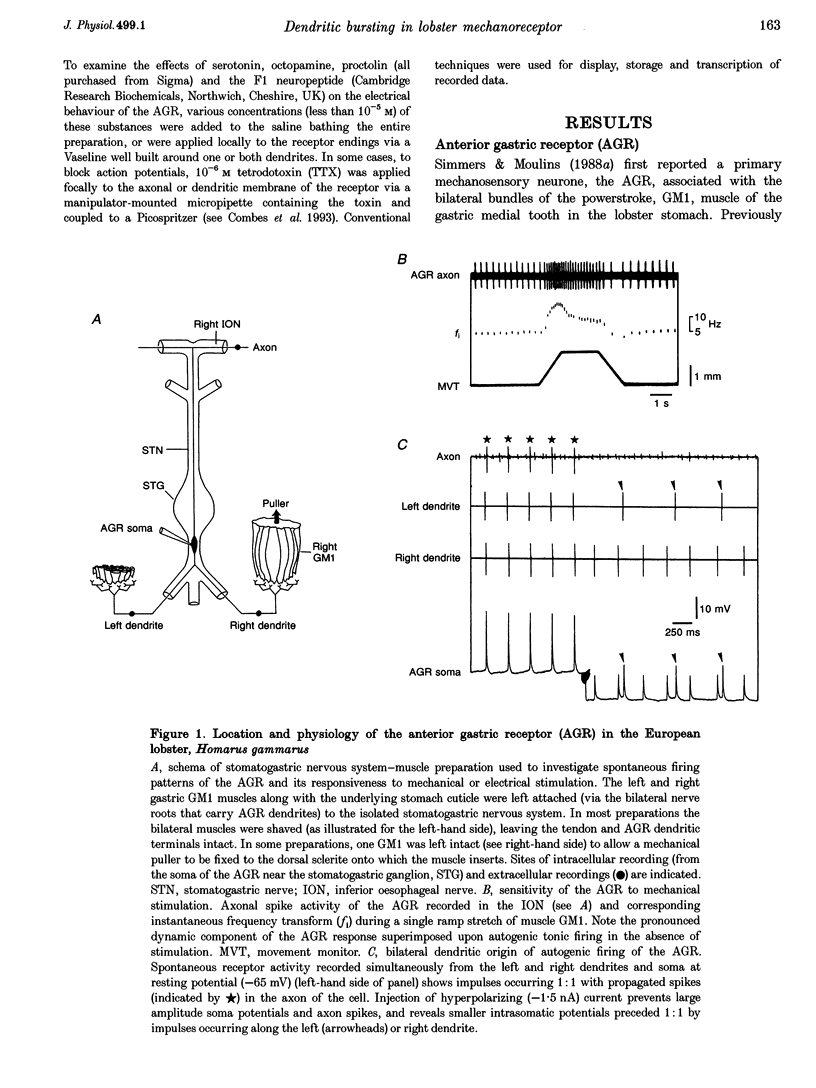
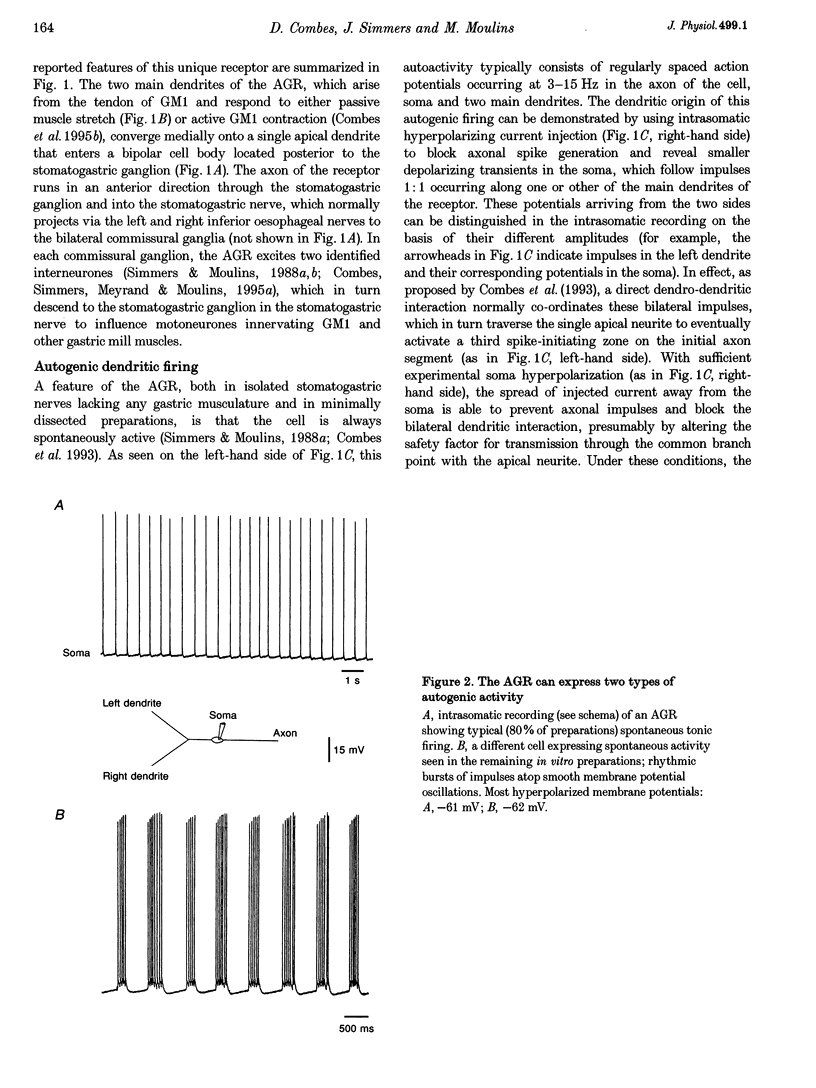
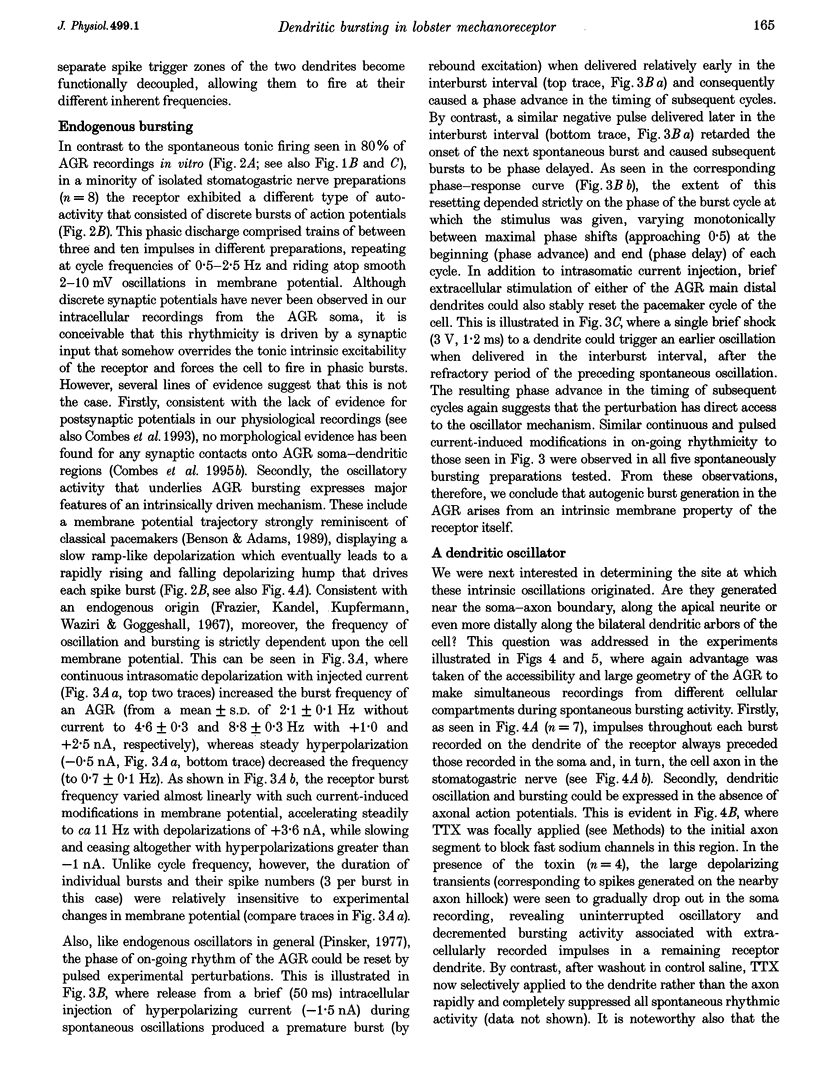
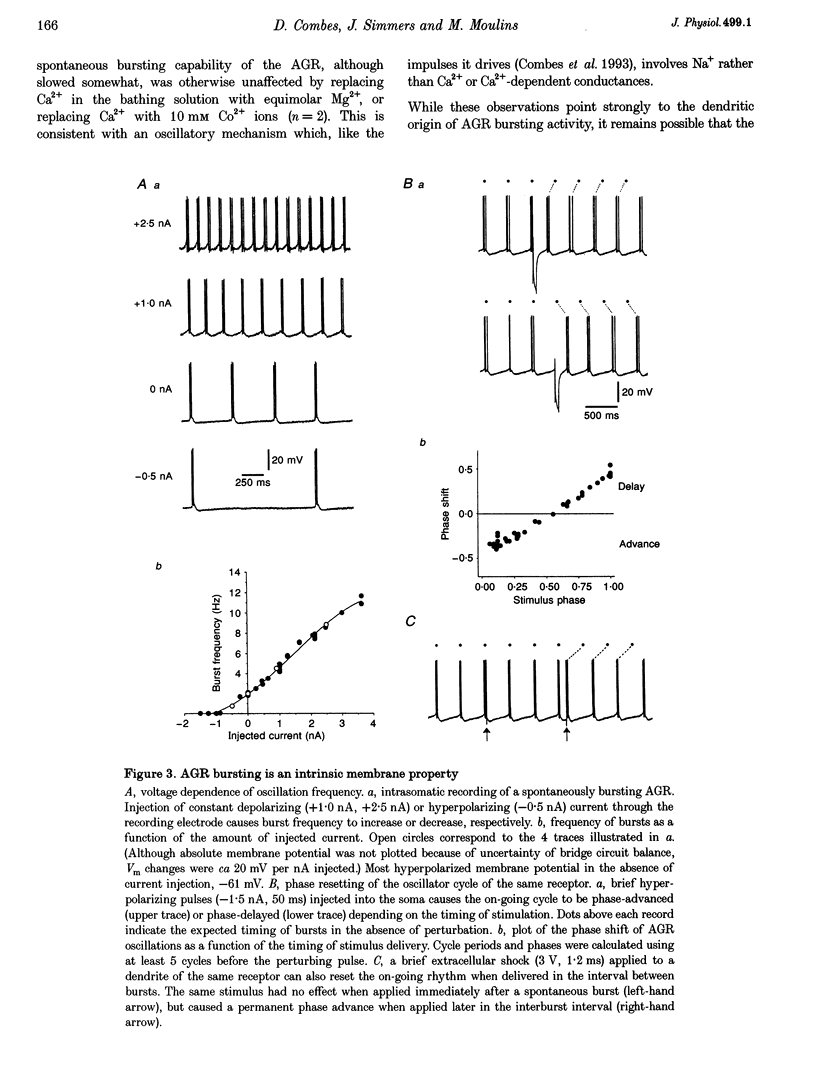
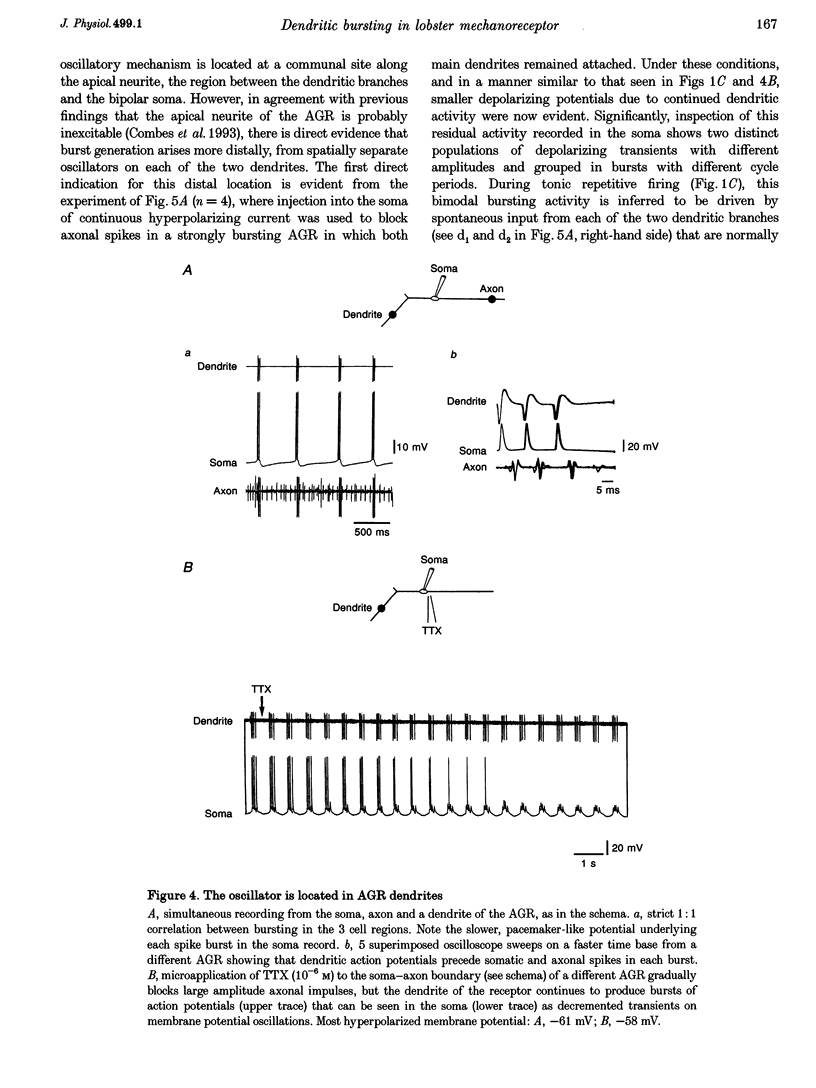
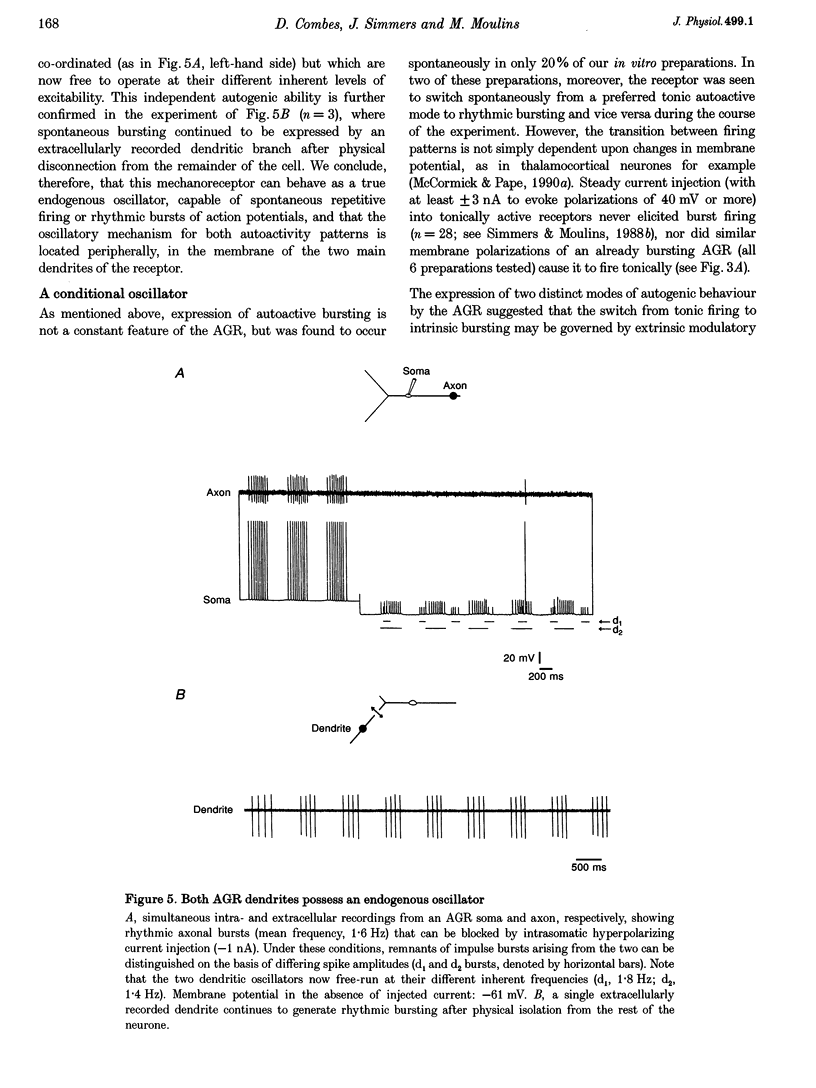
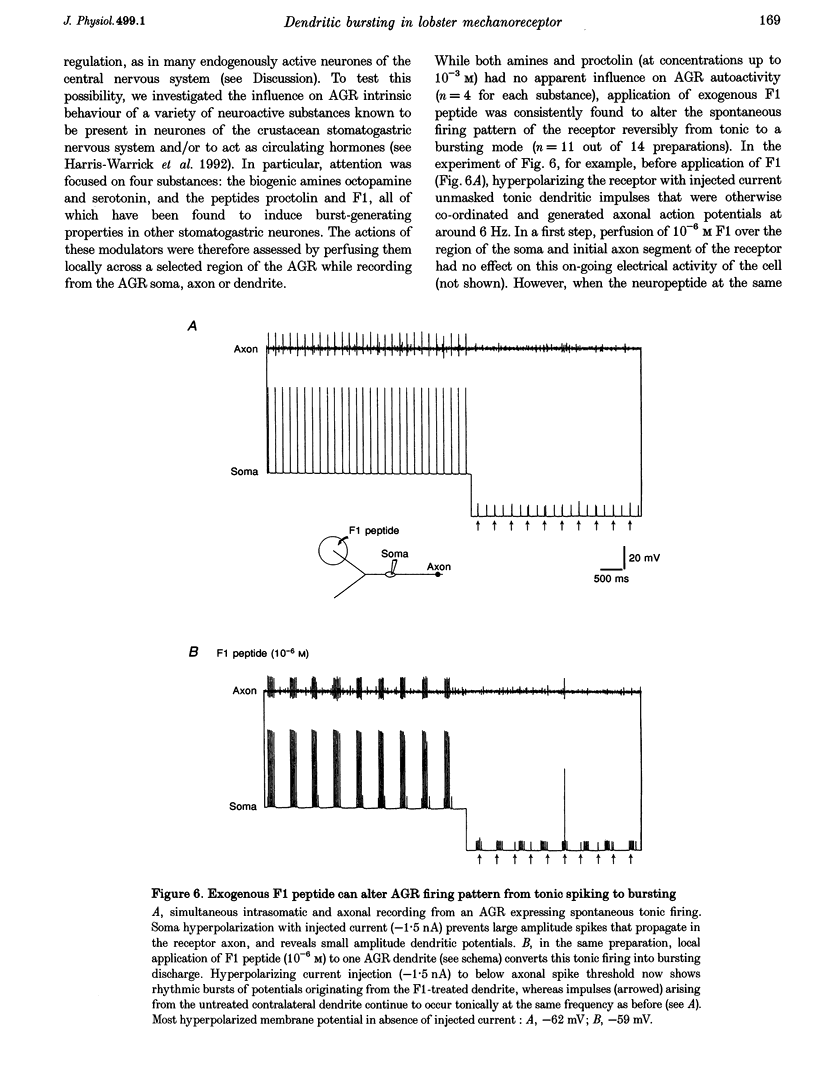
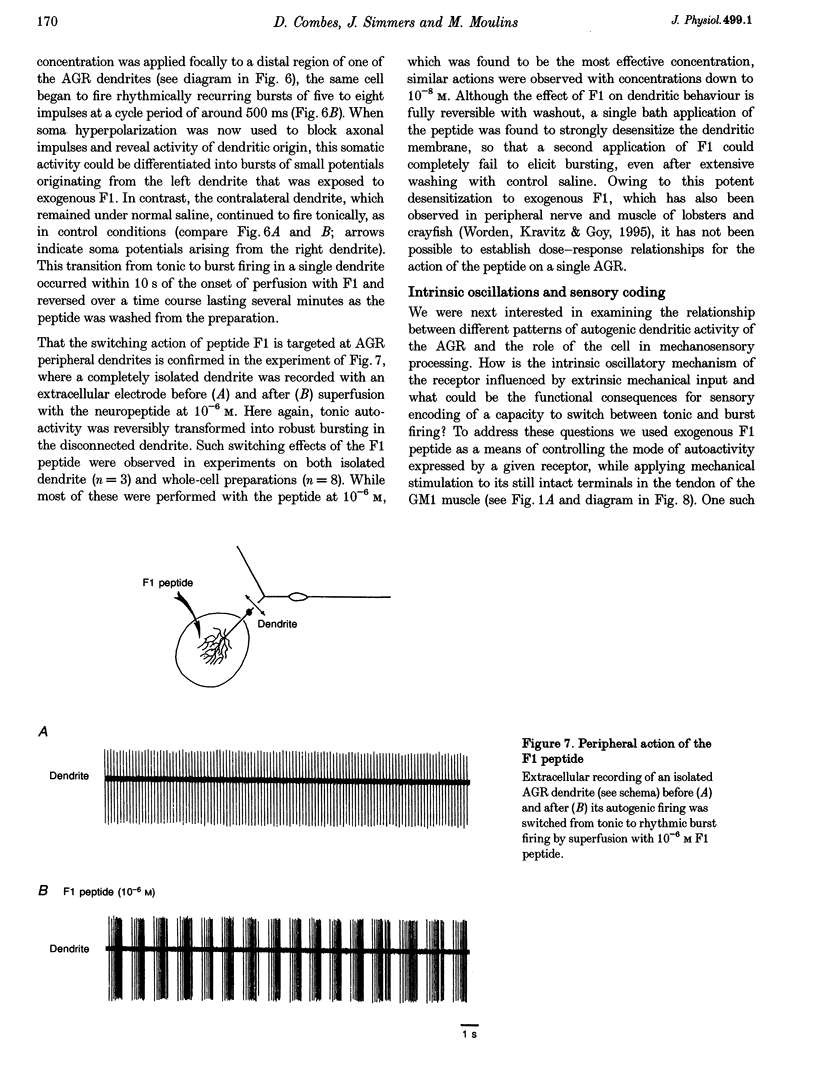
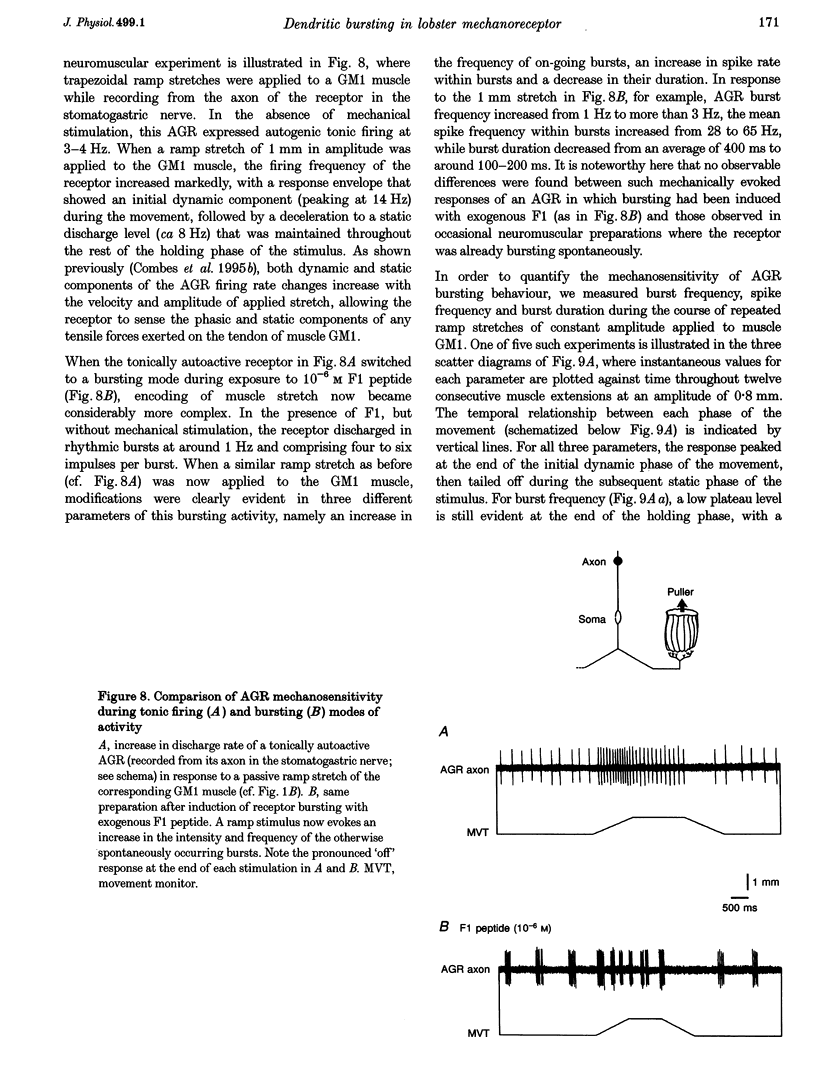
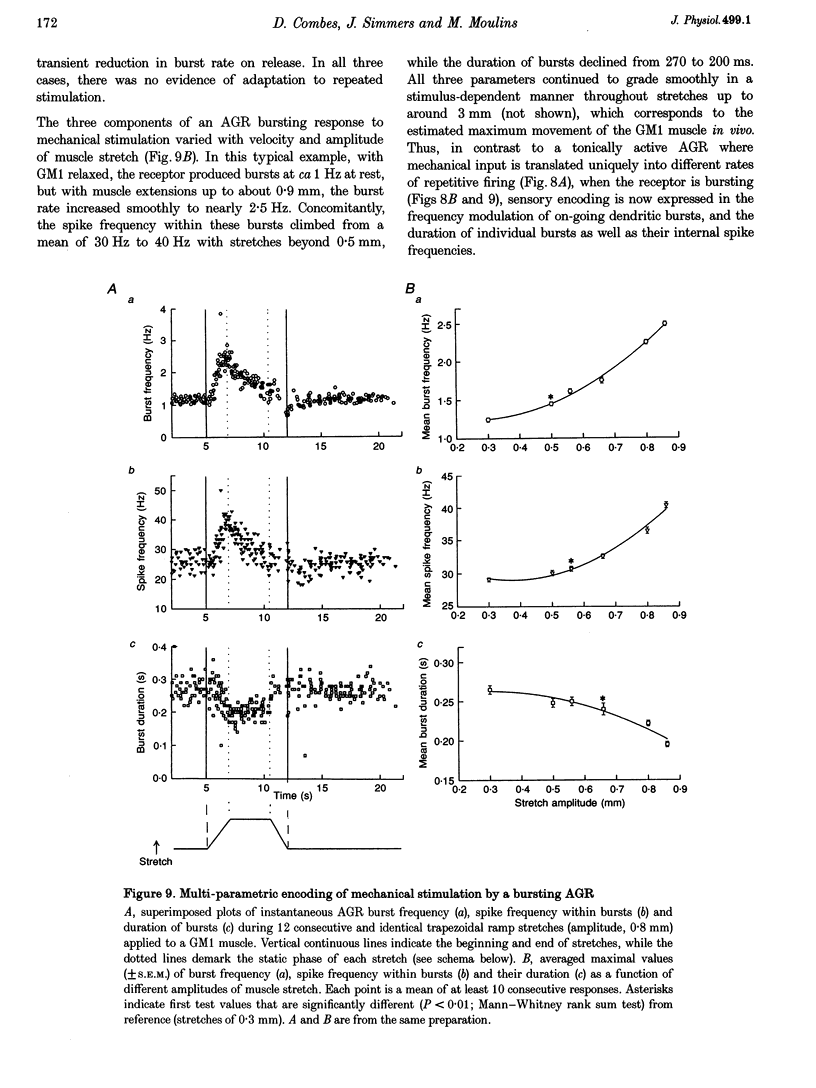
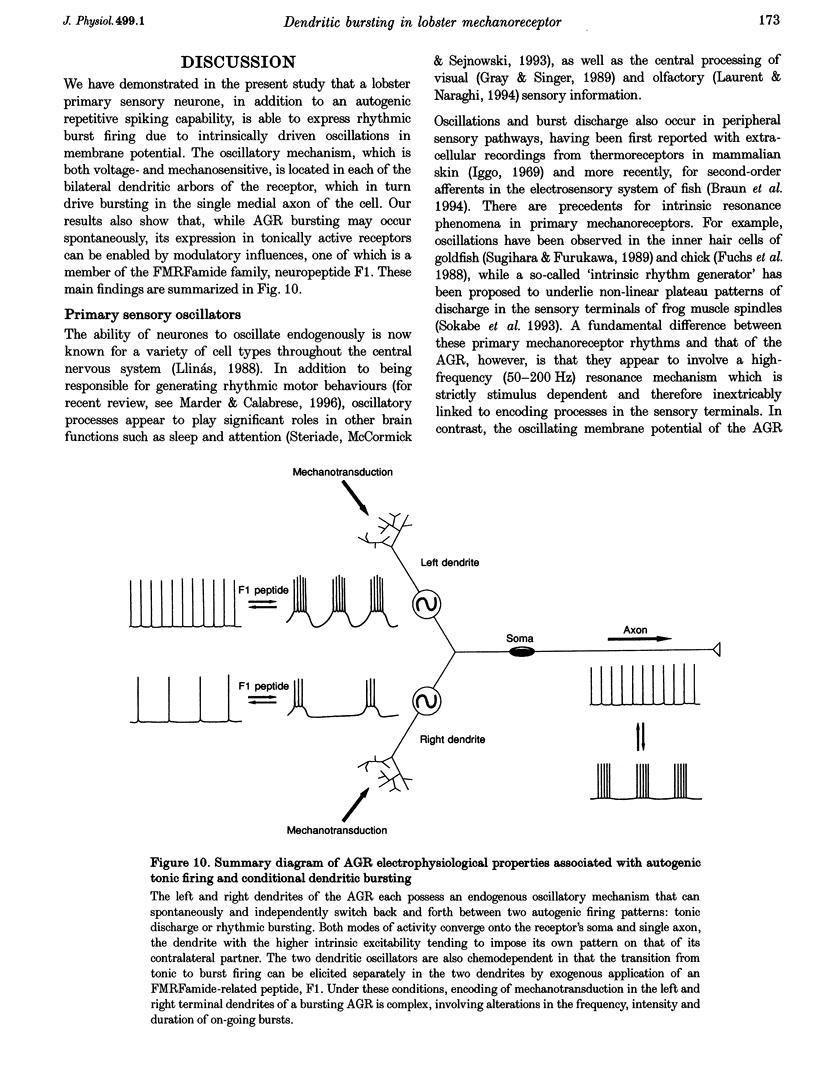
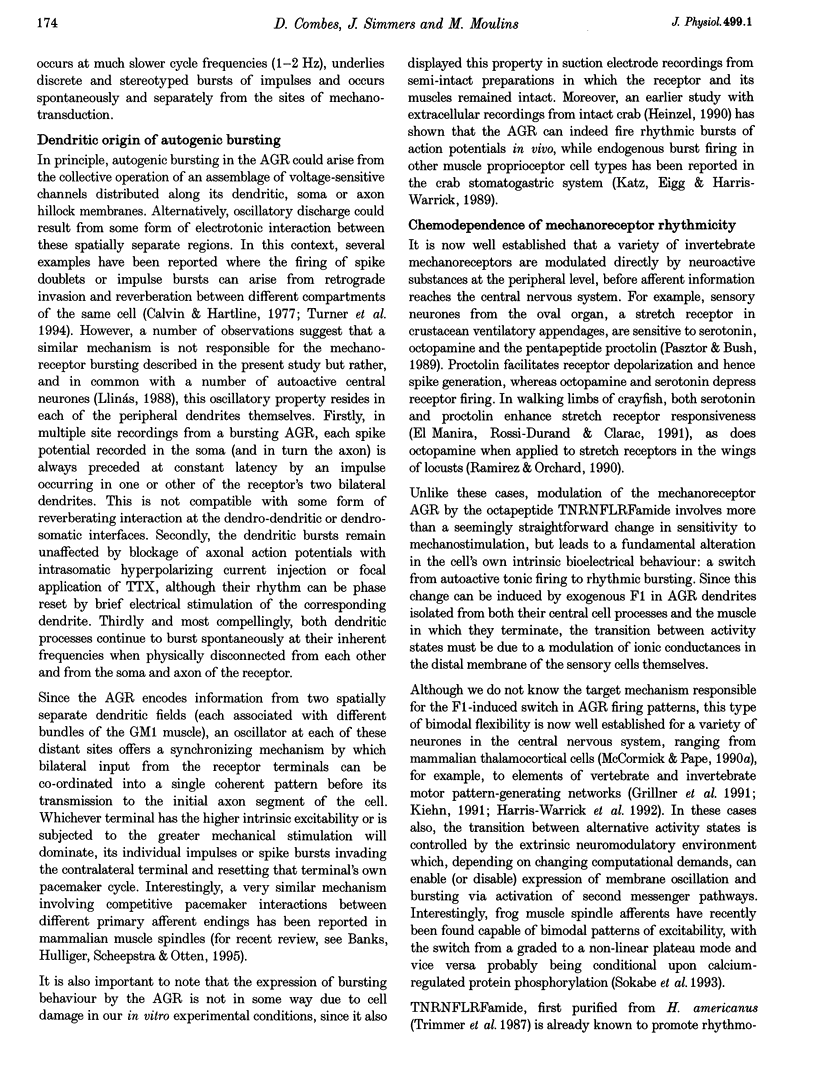
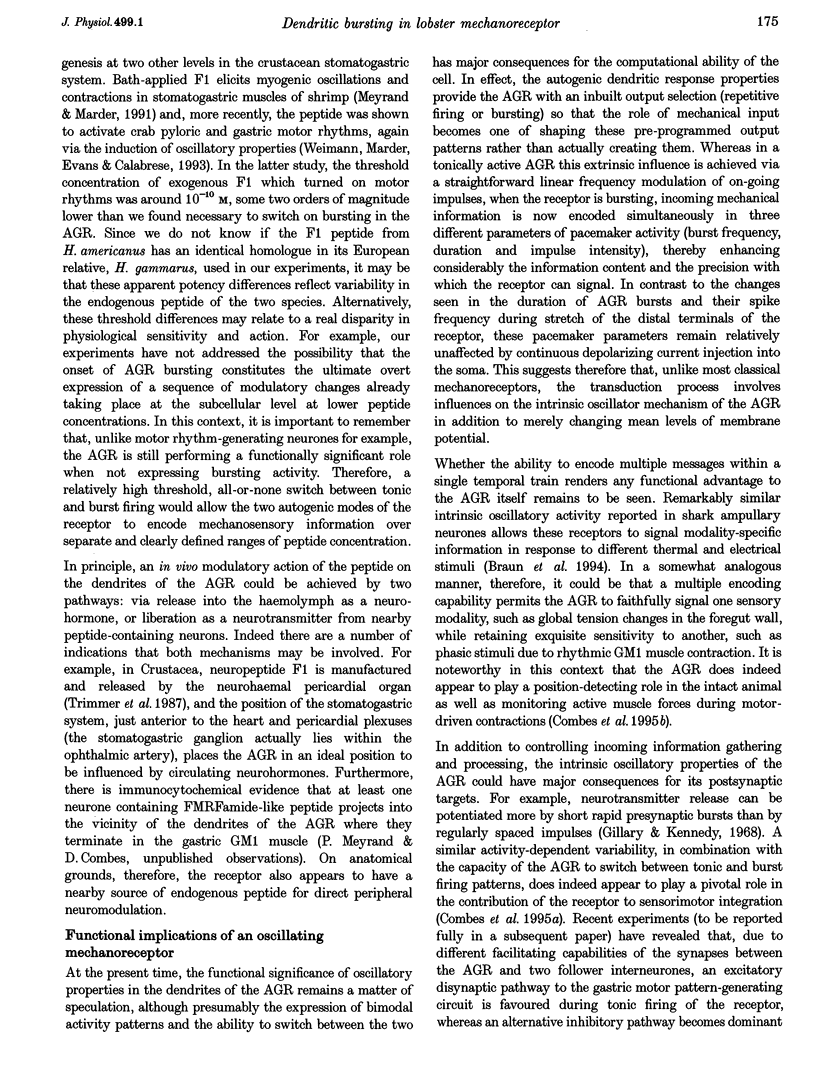
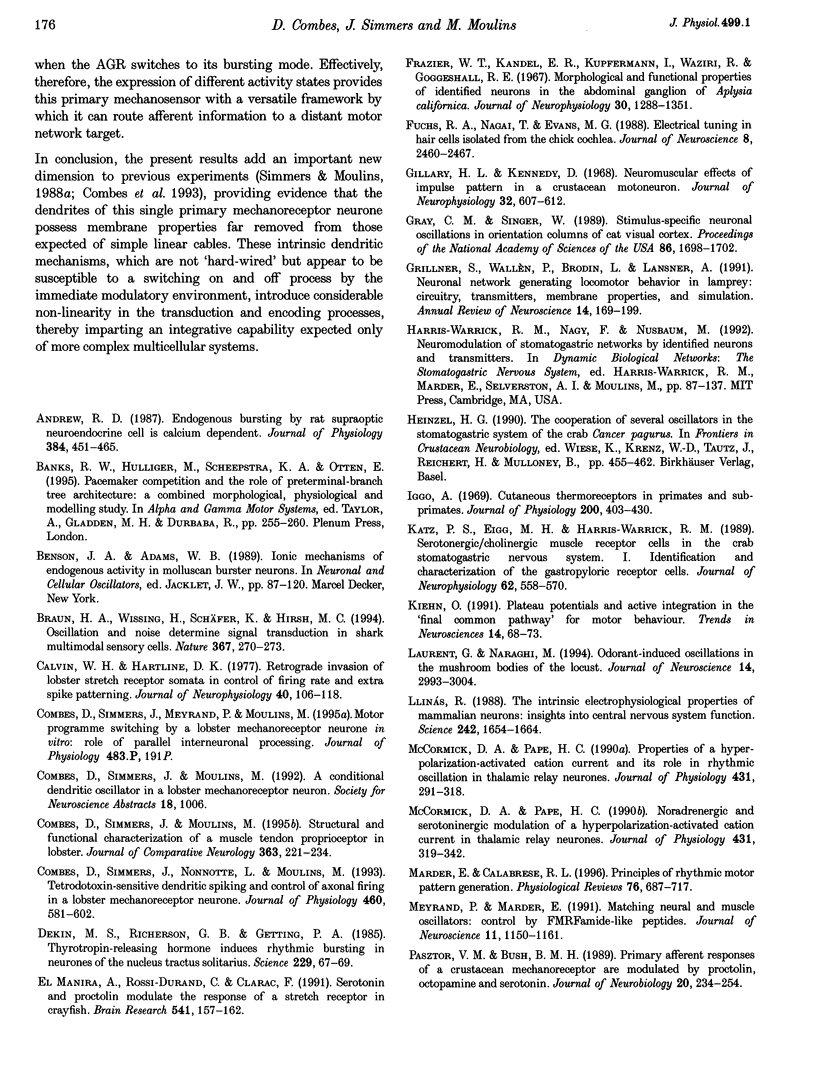
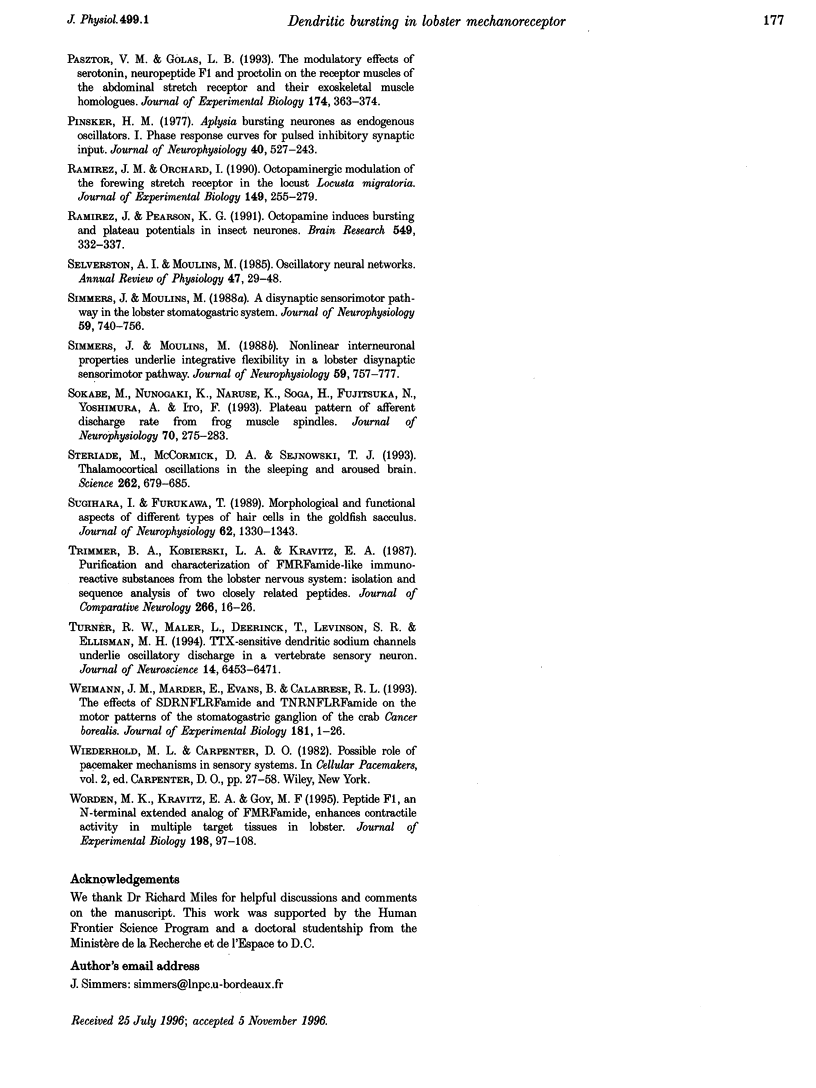
Selected References
These references are in PubMed. This may not be the complete list of references from this article.
- Andrew R. D. Endogenous bursting by rat supraoptic neuroendocrine cells is calcium dependent. J Physiol. 1987 Mar;384:451–465. doi: 10.1113/jphysiol.1987.sp016463. [DOI] [PMC free article] [PubMed] [Google Scholar]
- Braun H. A., Wissing H., Schäfer K., Hirsch M. C. Oscillation and noise determine signal transduction in shark multimodal sensory cells. Nature. 1994 Jan 20;367(6460):270–273. doi: 10.1038/367270a0. [DOI] [PubMed] [Google Scholar]
- Calvin W. H., Hartline D. K. Retrograde invasion of lobster stretch receptor somata in control of firing rate and extra spike patterning. J Neurophysiol. 1977 Jan;40(1):106–118. doi: 10.1152/jn.1977.40.1.106. [DOI] [PubMed] [Google Scholar]
- Combes D., Simmers J., Moulins M. Structural and functional characterization of a muscle tendon proprioceptor in lobster. J Comp Neurol. 1995 Dec 11;363(2):221–234. doi: 10.1002/cne.903630205. [DOI] [PubMed] [Google Scholar]
- Combes D., Simmers J., Nonnotte L., Moulins M. Tetrodotoxin-sensitive dendritic spiking and control of axonal firing in a lobster mechanoreceptor neurone. J Physiol. 1993 Jan;460:581–602. doi: 10.1113/jphysiol.1993.sp019488. [DOI] [PMC free article] [PubMed] [Google Scholar]
- Dekin M. S., Richerson G. B., Getting P. A. Thyrotropin-releasing hormone induces rhythmic bursting in neurons of the nucleus tractus solitarius. Science. 1985 Jul 5;229(4708):67–69. doi: 10.1126/science.3925552. [DOI] [PubMed] [Google Scholar]
- Fuchs P. A., Nagai T., Evans M. G. Electrical tuning in hair cells isolated from the chick cochlea. J Neurosci. 1988 Jul;8(7):2460–2467. doi: 10.1523/JNEUROSCI.08-07-02460.1988. [DOI] [PMC free article] [PubMed] [Google Scholar]
- Gillary H. L., Kennedy D. Neuromuscular effects of impulse pattern in a crustacean motoneuron. J Neurophysiol. 1969 Jul;32(4):607–612. doi: 10.1152/jn.1969.32.4.607. [DOI] [PubMed] [Google Scholar]
- Gray C. M., Singer W. Stimulus-specific neuronal oscillations in orientation columns of cat visual cortex. Proc Natl Acad Sci U S A. 1989 Mar;86(5):1698–1702. doi: 10.1073/pnas.86.5.1698. [DOI] [PMC free article] [PubMed] [Google Scholar]
- Grillner S., Wallén P., Brodin L., Lansner A. Neuronal network generating locomotor behavior in lamprey: circuitry, transmitters, membrane properties, and simulation. Annu Rev Neurosci. 1991;14:169–199. doi: 10.1146/annurev.ne.14.030191.001125. [DOI] [PubMed] [Google Scholar]
- Iggo A. Cutaneous thermoreceptors in primates and sub-primates. J Physiol. 1969 Feb;200(2):403–430. doi: 10.1113/jphysiol.1969.sp008701. [DOI] [PMC free article] [PubMed] [Google Scholar]
- Katz P. S., Eigg M. H., Harris-Warrick R. M. Serotonergic/cholinergic muscle receptor cells in the crab stomatogastric nervous system. I. Identification and characterization of the gastropyloric receptor cells. J Neurophysiol. 1989 Aug;62(2):558–570. doi: 10.1152/jn.1989.62.2.558. [DOI] [PubMed] [Google Scholar]
- Kiehn O. Plateau potentials and active integration in the 'final common pathway' for motor behaviour. Trends Neurosci. 1991 Feb;14(2):68–73. doi: 10.1016/0166-2236(91)90023-n. [DOI] [PubMed] [Google Scholar]
- Laurent G., Naraghi M. Odorant-induced oscillations in the mushroom bodies of the locust. J Neurosci. 1994 May;14(5 Pt 2):2993–3004. doi: 10.1523/JNEUROSCI.14-05-02993.1994. [DOI] [PMC free article] [PubMed] [Google Scholar]
- Llinás R. R. The intrinsic electrophysiological properties of mammalian neurons: insights into central nervous system function. Science. 1988 Dec 23;242(4886):1654–1664. doi: 10.1126/science.3059497. [DOI] [PubMed] [Google Scholar]
- Marder E., Calabrese R. L. Principles of rhythmic motor pattern generation. Physiol Rev. 1996 Jul;76(3):687–717. doi: 10.1152/physrev.1996.76.3.687. [DOI] [PubMed] [Google Scholar]
- McCormick D. A., Pape H. C. Noradrenergic and serotonergic modulation of a hyperpolarization-activated cation current in thalamic relay neurones. J Physiol. 1990 Dec;431:319–342. doi: 10.1113/jphysiol.1990.sp018332. [DOI] [PMC free article] [PubMed] [Google Scholar]
- McCormick D. A., Pape H. C. Properties of a hyperpolarization-activated cation current and its role in rhythmic oscillation in thalamic relay neurones. J Physiol. 1990 Dec;431:291–318. doi: 10.1113/jphysiol.1990.sp018331. [DOI] [PMC free article] [PubMed] [Google Scholar]
- Meyrand P., Marder E. Matching neural and muscle oscillators: control by FMRFamide-like peptides. J Neurosci. 1991 Apr;11(4):1150–1161. doi: 10.1523/JNEUROSCI.11-04-01150.1991. [DOI] [PMC free article] [PubMed] [Google Scholar]
- Pasztor V. M., Bush B. M. Primary afferent responses of a crustacean mechanoreceptor are modulated by proctolin, octopamine, and serotonin. J Neurobiol. 1989 Jun;20(4):234–254. doi: 10.1002/neu.480200406. [DOI] [PubMed] [Google Scholar]
- Pinsker H. M. Aplysia bursting neurons as endogenous oscillators. I. Phase-response curves for pulsed inhibitory synaptic input. J Neurophysiol. 1977 May;40(3):527–543. doi: 10.1152/jn.1977.40.3.527. [DOI] [PubMed] [Google Scholar]
- Ramirez J. M., Pearson K. G. Octopamine induces bursting and plateau potentials in insect neurones. Brain Res. 1991 May 24;549(2):332–337. doi: 10.1016/0006-8993(91)90477-d. [DOI] [PubMed] [Google Scholar]
- Selverston A. I., Moulins M. Oscillatory neural networks. Annu Rev Physiol. 1985;47:29–48. doi: 10.1146/annurev.ph.47.030185.000333. [DOI] [PubMed] [Google Scholar]
- Simmers J., Moulins M. A disynaptic sensorimotor pathway in the lobster stomatogastric system. J Neurophysiol. 1988 Mar;59(3):740–756. doi: 10.1152/jn.1988.59.3.740. [DOI] [PubMed] [Google Scholar]
- Simmers J., Moulins M. Nonlinear interneuronal properties underlie integrative flexibility in a lobster disynaptic sensorimotor pathway. J Neurophysiol. 1988 Mar;59(3):757–777. doi: 10.1152/jn.1988.59.3.757. [DOI] [PubMed] [Google Scholar]
- Sokabe M., Nunogaki K., Naruse K., Soga H., Fujitsuka N., Yoshimura A., Ito F. Plateau pattern of afferent discharge rate from frog muscle spindles. J Neurophysiol. 1993 Jul;70(1):275–283. doi: 10.1152/jn.1993.70.1.275. [DOI] [PubMed] [Google Scholar]
- Steriade M., McCormick D. A., Sejnowski T. J. Thalamocortical oscillations in the sleeping and aroused brain. Science. 1993 Oct 29;262(5134):679–685. doi: 10.1126/science.8235588. [DOI] [PubMed] [Google Scholar]
- Sugihara I., Furukawa T. Morphological and functional aspects of two different types of hair cells in the goldfish sacculus. J Neurophysiol. 1989 Dec;62(6):1330–1343. doi: 10.1152/jn.1989.62.6.1330. [DOI] [PubMed] [Google Scholar]
- Trimmer B. A., Kobierski L. A., Kravitz E. A. Purification and characterization of FMRFamidelike immunoreactive substances from the lobster nervous system: isolation and sequence analysis of two closely related peptides. J Comp Neurol. 1987 Dec 1;266(1):16–26. doi: 10.1002/cne.902660103. [DOI] [PubMed] [Google Scholar]
- Turner R. W., Maler L., Deerinck T., Levinson S. R., Ellisman M. H. TTX-sensitive dendritic sodium channels underlie oscillatory discharge in a vertebrate sensory neuron. J Neurosci. 1994 Nov;14(11 Pt 1):6453–6471. doi: 10.1523/JNEUROSCI.14-11-06453.1994. [DOI] [PMC free article] [PubMed] [Google Scholar]
- Weimann J. M., Marder E., Evans B., Calabrese R. L. The effects of SDRNFLRFamide and TNRNFLRFamide on the motor patterns of the stomatogastric ganglion of the crab Cancer borealis. J Exp Biol. 1993 Aug;181:1–26. doi: 10.1242/jeb.181.1.1. [DOI] [PubMed] [Google Scholar]
- Worden M. K., Kravitz E. A., Goy M. F. Peptide F1, an N-terminally extended analog of FMRFamide, enhances contractile activity in multiple target tissues in lobster. J Exp Biol. 1995 Jan;198(Pt 1):97–108. doi: 10.1242/jeb.198.1.97. [DOI] [PubMed] [Google Scholar]
- el Manira A., Rossi-Durand C., Clarac F. Serotonin and proctolin modulate the response of a stretch receptor in crayfish. Brain Res. 1991 Feb 8;541(1):157–162. doi: 10.1016/0006-8993(91)91091-e. [DOI] [PubMed] [Google Scholar]