Abstract
1. The present study was undertaken to document the excitability changes produced by prolonged high-frequency trains of impulses in cutaneous afferents of six human subjects. 2. Trains of supramaximal stimuli at 200 Hz for 2 min or less produced a prolonged depression in excitability, consistent with activation of the electrogenic Na+-K+ pump. Trains of longer duration resulted in an initial period of hyperexcitability which, with 10 min trains, was associated with the sensation of paraesthesiae in all subjects. This transient hyperexcitability gradually gave way to a long-lasting period of hypoexcitability. 3. The excitability changes were reproducible, and were accompanied by corresponding changes in supernormality, refractoriness, strength-duration time constant and rheobase current, suggesting that the changes in axonal excitability reflected a change in membrane potential. 4. The transient increase in excitability that follows tetanic trains of 10 min had qualitatively similar effects on cutaneous axons as ischaemia or application of a depolarizing current. The post-tetanic changes in the supernormal period of sensory axons were those expected from the changes in excitability, without evidence of a gross distortion in its time course, as has been previously demonstrated in a hyperstimulated human motor axon. 5. It is concluded that the post-tetanic hyperexcitability of human sensory axons is probably driven by increased K+ accumulation in the restricted diffusion space under the myelin sheath, much as in motor axons, the differences in behaviour of sensory and motor axons being explicable by greater inward rectification in sensory axons.
Full text
PDF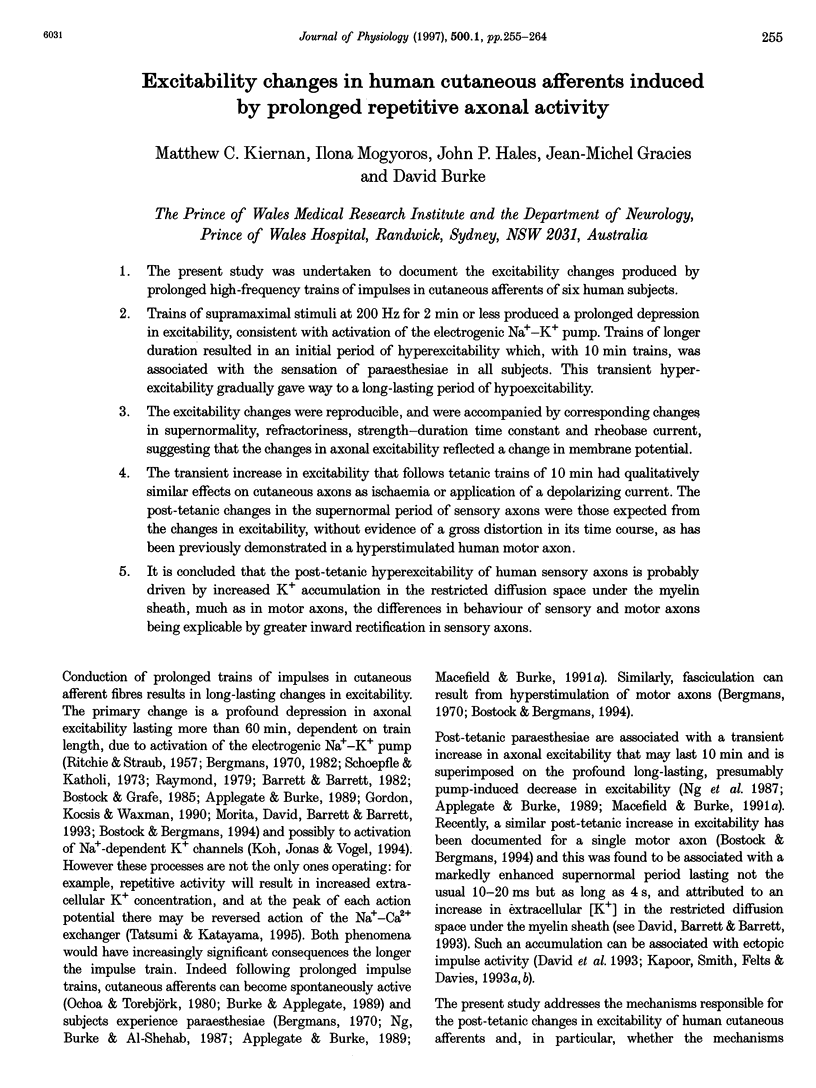
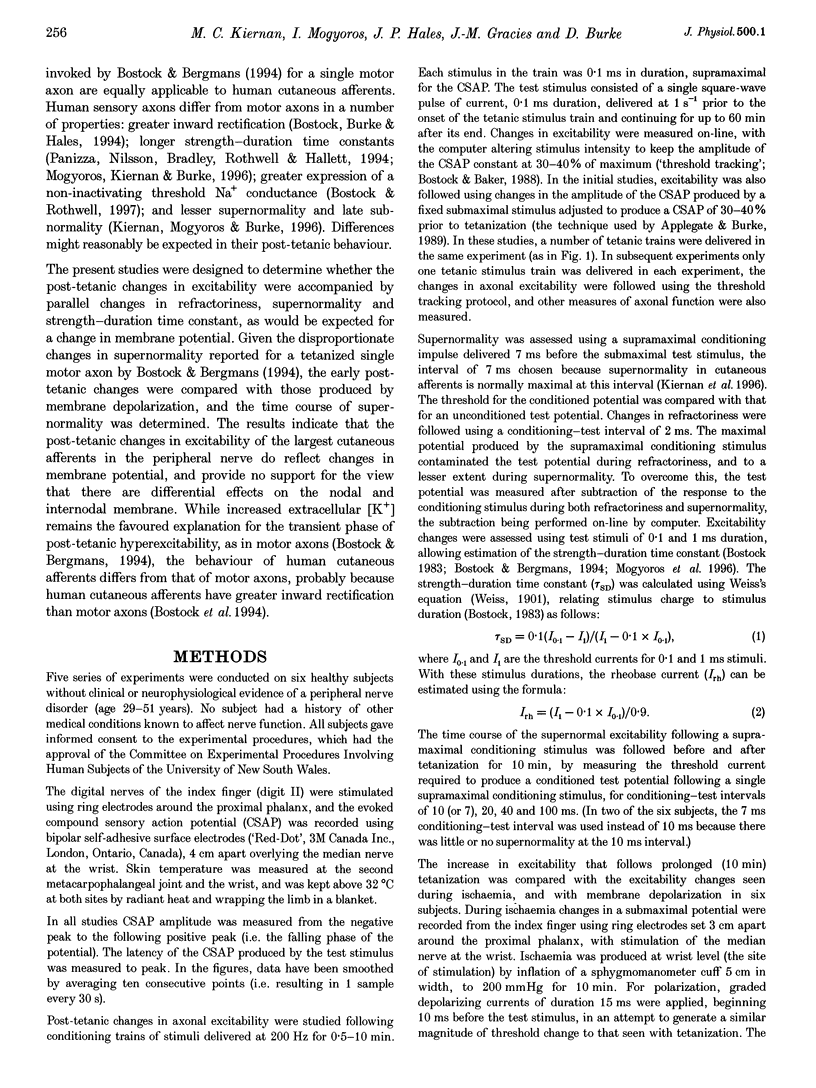
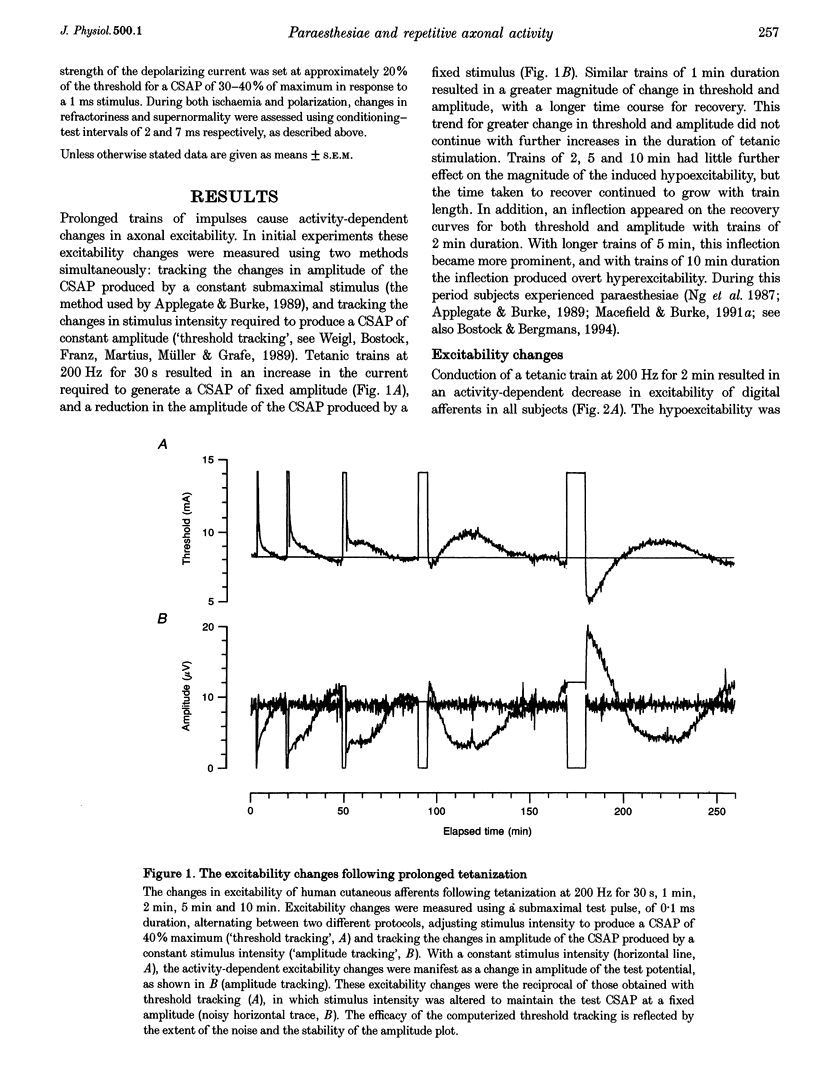
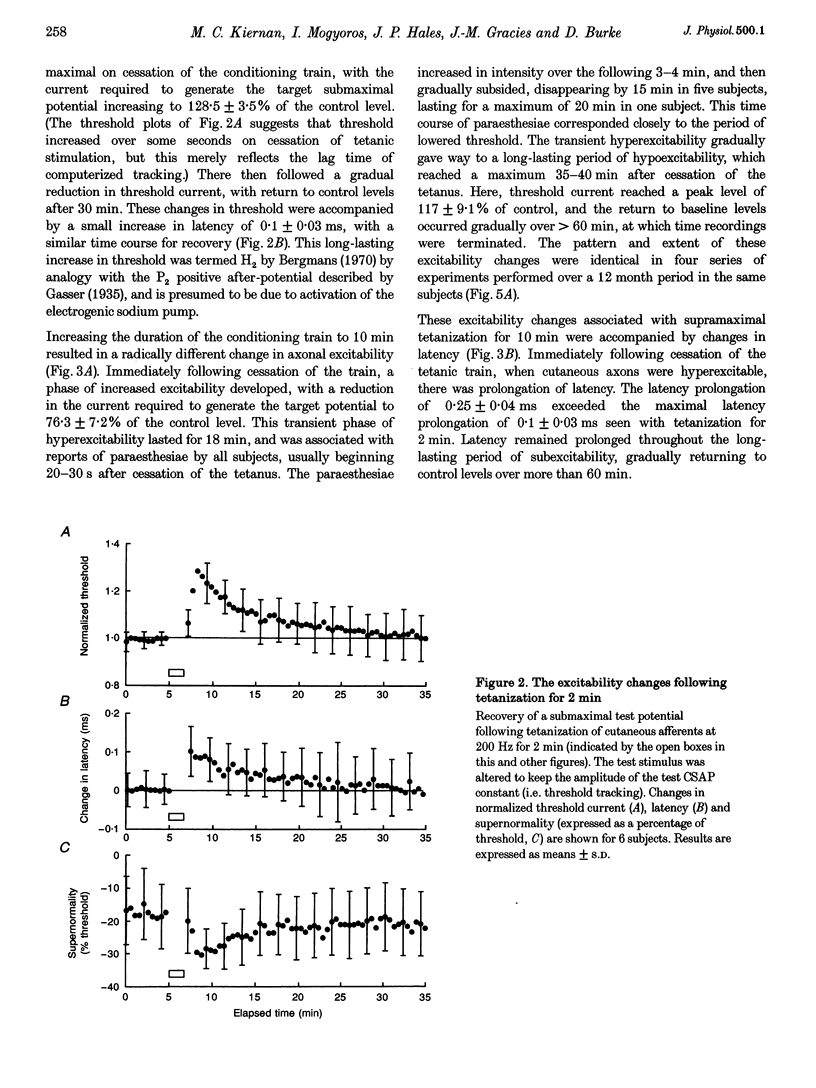
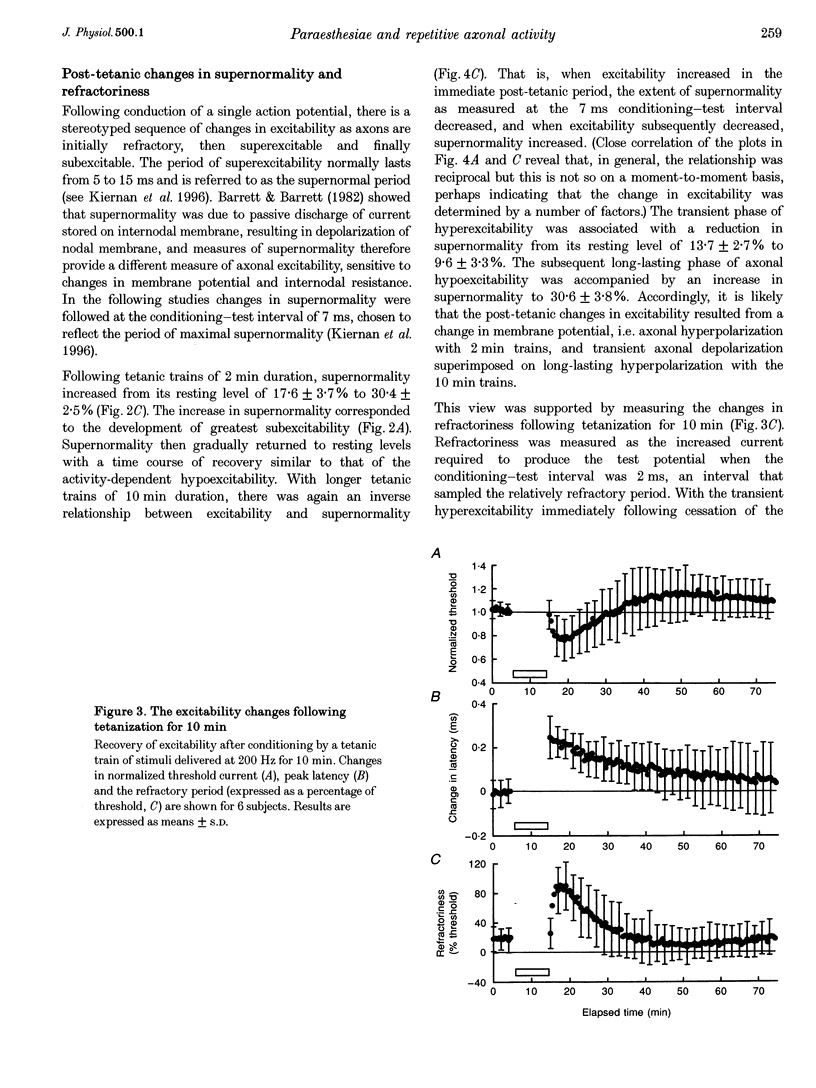
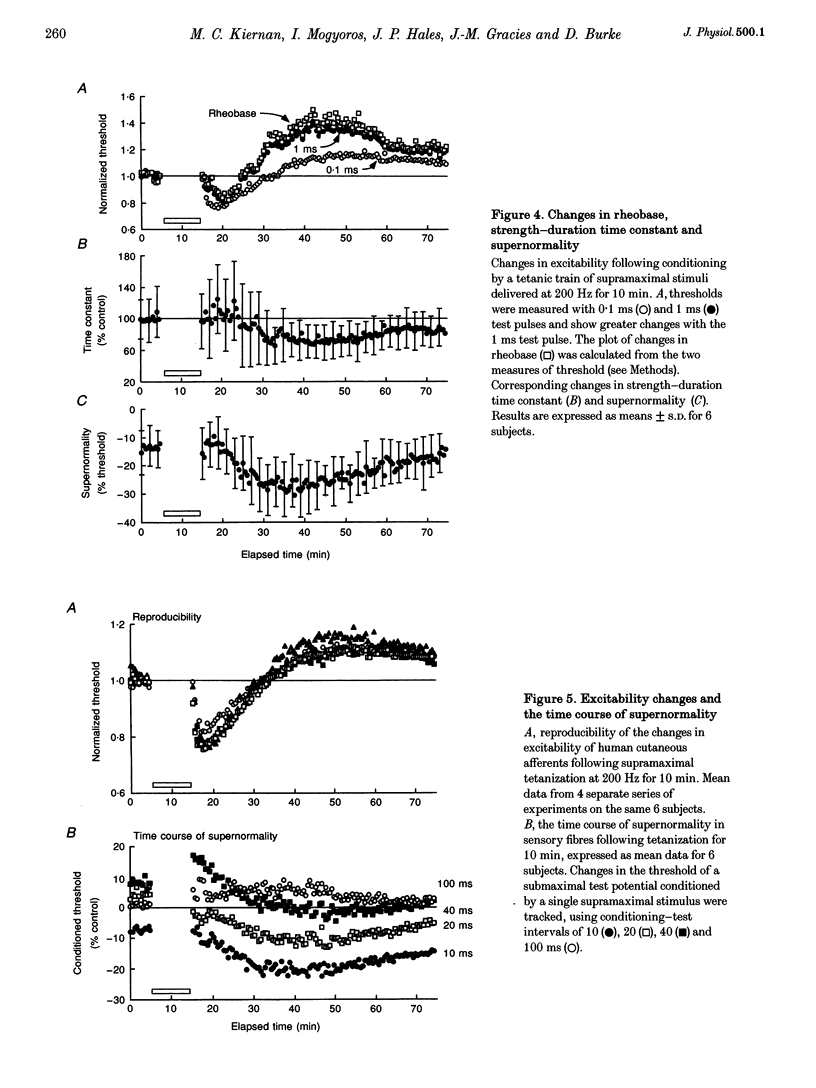
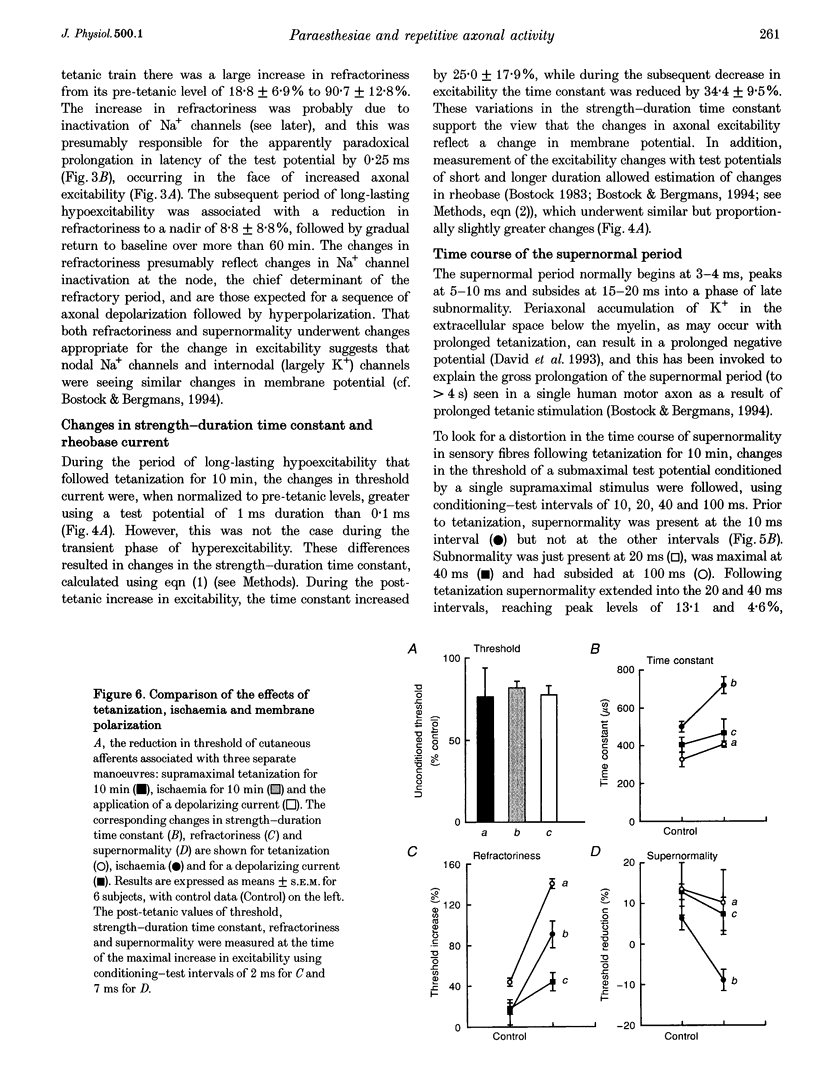
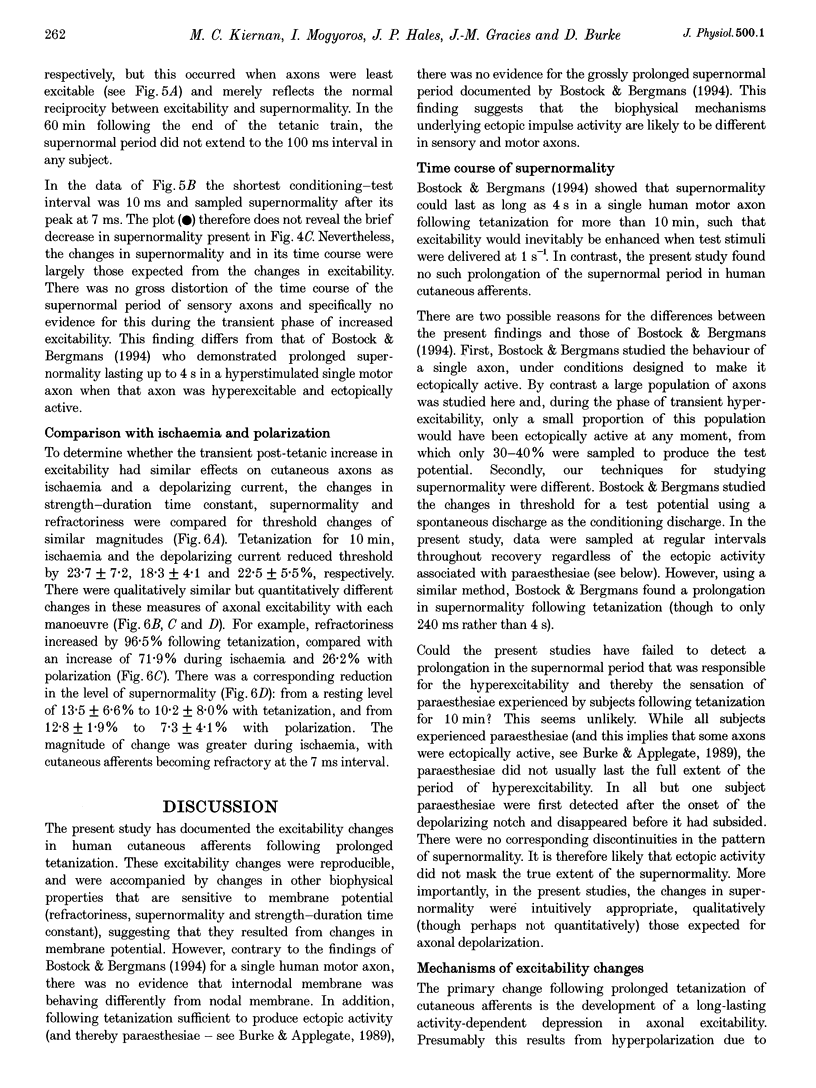
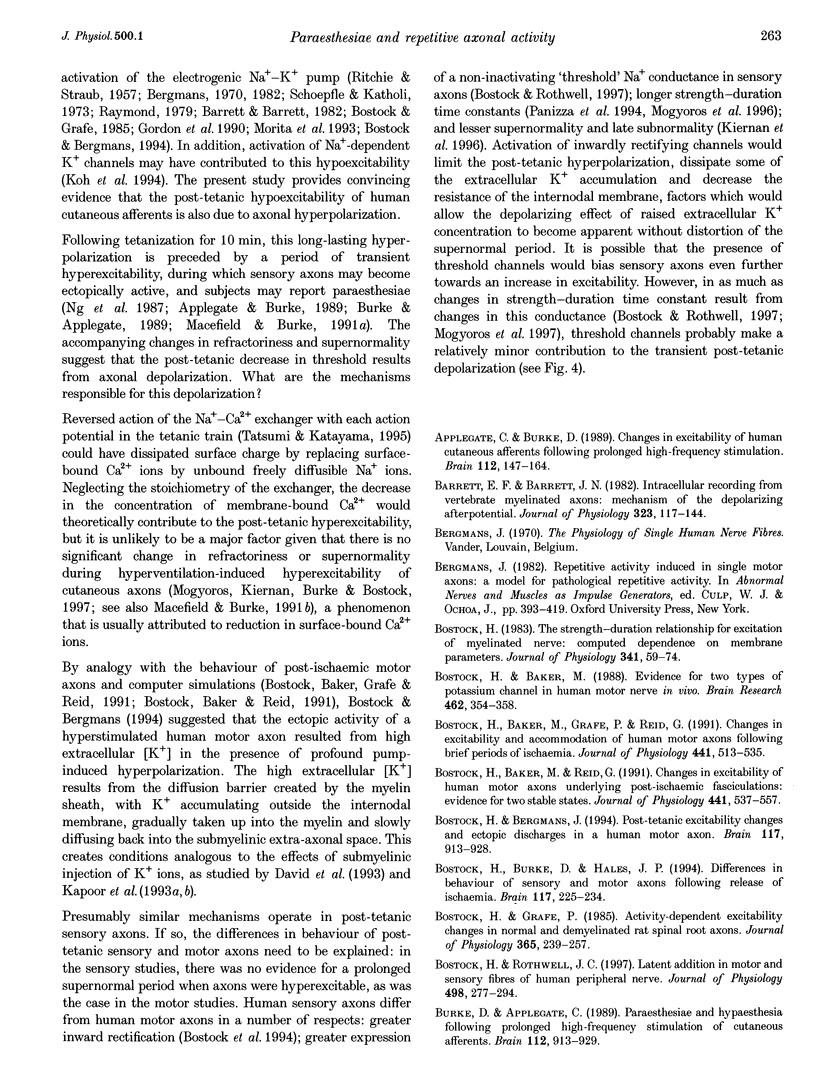
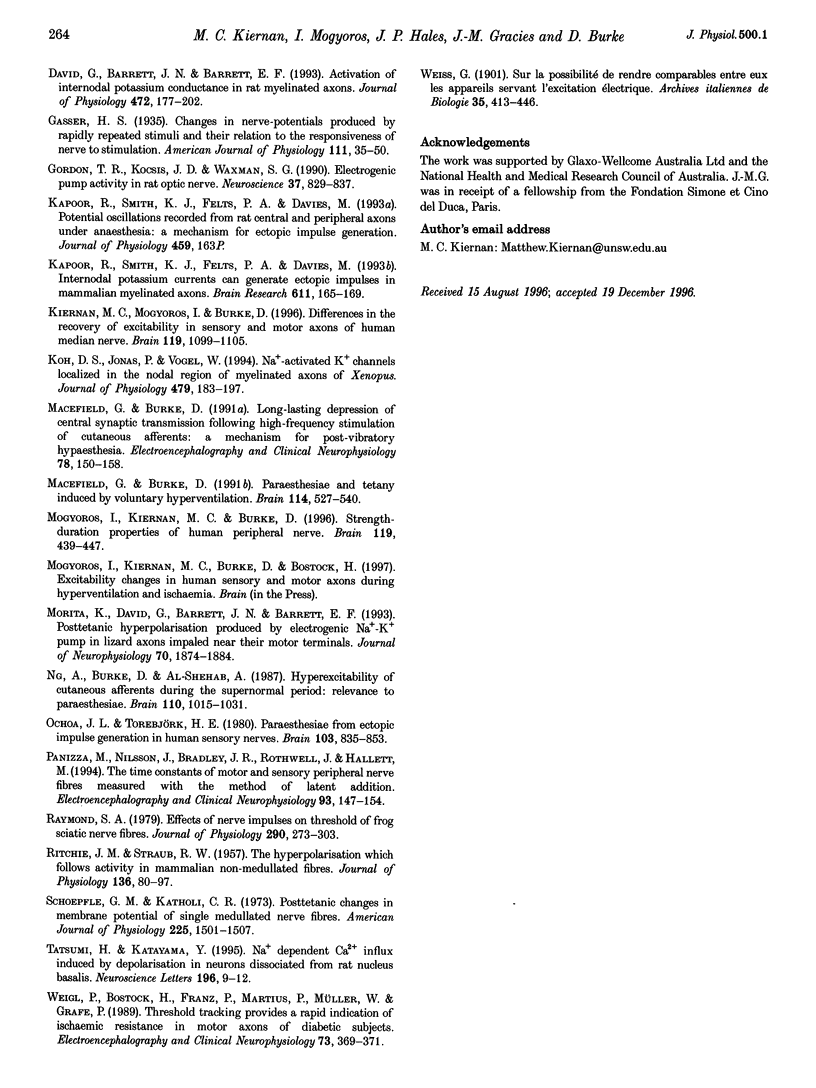
Images in this article
Selected References
These references are in PubMed. This may not be the complete list of references from this article.
- Applegate C., Burke D. Changes in excitability of human cutaneous afferents following prolonged high-frequency stimulation. Brain. 1989 Feb;112(Pt 1):147–164. doi: 10.1093/brain/112.1.147. [DOI] [PubMed] [Google Scholar]
- Barrett E. F., Barrett J. N. Intracellular recording from vertebrate myelinated axons: mechanism of the depolarizing afterpotential. J Physiol. 1982 Feb;323:117–144. doi: 10.1113/jphysiol.1982.sp014064. [DOI] [PMC free article] [PubMed] [Google Scholar]
- Bostock H., Baker M. Evidence for two types of potassium channel in human motor axons in vivo. Brain Res. 1988 Oct 18;462(2):354–358. doi: 10.1016/0006-8993(88)90564-1. [DOI] [PubMed] [Google Scholar]
- Bostock H., Baker M., Grafe P., Reid G. Changes in excitability and accommodation of human motor axons following brief periods of ischaemia. J Physiol. 1991 Sep;441:513–535. doi: 10.1113/jphysiol.1991.sp018765. [DOI] [PMC free article] [PubMed] [Google Scholar]
- Bostock H., Baker M., Reid G. Changes in excitability of human motor axons underlying post-ischaemic fasciculations: evidence for two stable states. J Physiol. 1991 Sep;441:537–557. doi: 10.1113/jphysiol.1991.sp018766. [DOI] [PMC free article] [PubMed] [Google Scholar]
- Bostock H., Bergmans J. Post-tetanic excitability changes and ectopic discharges in a human motor axon. Brain. 1994 Oct;117(Pt 5):913–928. doi: 10.1093/brain/117.5.913. [DOI] [PubMed] [Google Scholar]
- Bostock H., Grafe P. Activity-dependent excitability changes in normal and demyelinated rat spinal root axons. J Physiol. 1985 Aug;365:239–257. doi: 10.1113/jphysiol.1985.sp015769. [DOI] [PMC free article] [PubMed] [Google Scholar]
- Bostock H., Rothwell J. C. Latent addition in motor and sensory fibres of human peripheral nerve. J Physiol. 1997 Jan 1;498(Pt 1):277–294. doi: 10.1113/jphysiol.1997.sp021857. [DOI] [PMC free article] [PubMed] [Google Scholar]
- Bostock H. The strength-duration relationship for excitation of myelinated nerve: computed dependence on membrane parameters. J Physiol. 1983 Aug;341:59–74. doi: 10.1113/jphysiol.1983.sp014792. [DOI] [PMC free article] [PubMed] [Google Scholar]
- Burke D., Applegate C. Paraesthesiae and hypaesthesia following prolonged high-frequency stimulation of cutaneous afferents. Brain. 1989 Aug;112(Pt 4):913–929. doi: 10.1093/brain/112.4.913. [DOI] [PubMed] [Google Scholar]
- David G., Barrett J. N., Barrett E. F. Activation of internodal potassium conductance in rat myelinated axons. J Physiol. 1993 Dec;472:177–202. doi: 10.1113/jphysiol.1993.sp019942. [DOI] [PMC free article] [PubMed] [Google Scholar]
- Gordon T. R., Kocsis J. D., Waxman S. G. Electrogenic pump (Na+/K(+)-ATPase) activity in rat optic nerve. Neuroscience. 1990;37(3):829–837. doi: 10.1016/0306-4522(90)90112-h. [DOI] [PubMed] [Google Scholar]
- Kapoor R., Smith K. J., Felts P. A., Davies M. Internodal potassium currents can generate ectopic impulses in mammalian myelinated axons. Brain Res. 1993 May 14;611(1):165–169. doi: 10.1016/0006-8993(93)91790-y. [DOI] [PubMed] [Google Scholar]
- Koh D. S., Jonas P., Vogel W. Na(+)-activated K+ channels localized in the nodal region of myelinated axons of Xenopus. J Physiol. 1994 Sep 1;479(Pt 2):183–197. doi: 10.1113/jphysiol.1994.sp020287. [DOI] [PMC free article] [PubMed] [Google Scholar]
- Macefield G., Burke D. Long-lasting depression of central synaptic transmission following prolonged high-frequency stimulation of cutaneous afferents: a mechanism for post-vibratory hypaesthesia. Electroencephalogr Clin Neurophysiol. 1991 Feb;78(2):150–158. doi: 10.1016/0013-4694(91)90115-k. [DOI] [PubMed] [Google Scholar]
- Mogyoros I., Kiernan M. C., Burke D. Strength-duration properties of human peripheral nerve. Brain. 1996 Apr;119(Pt 2):439–447. doi: 10.1093/brain/119.2.439. [DOI] [PubMed] [Google Scholar]
- Morita K., David G., Barrett J. N., Barrett E. F. Posttetanic hyperpolarization produced by electrogenic Na(+)-K+ pump in lizard axons impaled near their motor terminals. J Neurophysiol. 1993 Nov;70(5):1874–1884. doi: 10.1152/jn.1993.70.5.1874. [DOI] [PubMed] [Google Scholar]
- Ng A., Burke D., Al-Shehab A. Hyperexcitability of cutaneous afferents during the supernormal period. Relevance to paraesthesiae. Brain. 1987 Aug;110(Pt 4):1015–1031. doi: 10.1093/brain/110.4.1015. [DOI] [PubMed] [Google Scholar]
- RITCHIE J. M., STRAUB R. W. The hyperpolarization which follows activity in mammalian non-medullated fibres. J Physiol. 1957 Apr 3;136(1):80–97. doi: 10.1113/jphysiol.1957.sp005744. [DOI] [PMC free article] [PubMed] [Google Scholar]
- Raymond S. A. Effects of nerve impulses on threshold of frog sciatic nerve fibres. J Physiol. 1979 May;290(2):273–303. doi: 10.1113/jphysiol.1979.sp012771. [DOI] [PMC free article] [PubMed] [Google Scholar]
- Schoepfle G. M., Katholi C. R. Posttetanic changes in membrane potential of single medullated nerve fibers. Am J Physiol. 1973 Dec;225(6):1501–1507. doi: 10.1152/ajplegacy.1973.225.6.1501. [DOI] [PubMed] [Google Scholar]
- Tatsumi H., Katayama Y. Na+ dependent Ca2+ influx induced by depolarization in neurons dissociated from rat nucleus basalis. Neurosci Lett. 1995 Aug 18;196(1-2):9–12. doi: 10.1016/0304-3940(95)11823-f. [DOI] [PubMed] [Google Scholar]