Abstract
1. Force measurements in isolated myofibrils (15 degrees C; sarcomere length, 2.10 microns) were used in this study to determine whether sarcomeric proteins are responsible for the large differences in the amounts of active and passive tension of cardiac versus skeletal muscle. Single myofibrils and bundles of two to four myofibrils were prepared from glycerinated tibialis anterior and sartorius muscles of the frog. Skinned frog atrial myocytes were used as a model for cardiac myofibrils. 2. Electron microscope analysis of the preparations showed that: (i) frog atrial myocytes contained a small and variable number of individual myofibrils (from 1 to 7); (ii) the mean cross-sectional area and mean number of myosin filaments of individual cardiac myofibrils did not differ significantly from those of single skeletal myofibrils; and (iii) the total myofibril cross-sectional area of atrial myocytes was on average comparable to that of bundles of two to four skeletal myofibrils. 3. In maximally activated skeletal preparations, values of active force ranged from 0.45 +/- 0.03 microN for the single myofibrils (mean +/- S.E.M.; n = 16) to 1.44 +/- 0.24 microN for the bundles of two to four myofibrils (n = 9). Maximum active force values of forty-five cardiac myocytes averaged 1.47 +/- 0.10 microN and exhibited a non-continuous distribution with peaks at intervals of about 0.5 microN. The results suggest that variation in active force among cardiac preparations mainly reflects variability in the number of myofibrils inside the myocytes and that individual cardiac myofibrils develop the same average amount of force as single skeletal myofibrils. 4. The mean sarcomere length-resting force relation of atrial myocytes could be superimposed on that of bundles of two to four skeletal myofibrils. This suggests that, for any given amount of strain, individual cardiac and skeletal sarcomeres bear essentially the same passive force. 5. The length-passive tension data of all preparations could be fitted by an exponential equation. Equation parameters obtained for both types of myofibrils were in reasonable agreement with those reported for larger preparations of frog skeletal muscle but were very different from those estimated for multicellular frog atrial preparations. It is concluded that myofibrils are the major determinant of resting tension in skeletal muscle; structures other than the myofibrils are responsible for the high passive stiffness of frog cardiac muscle.
Full text
PDF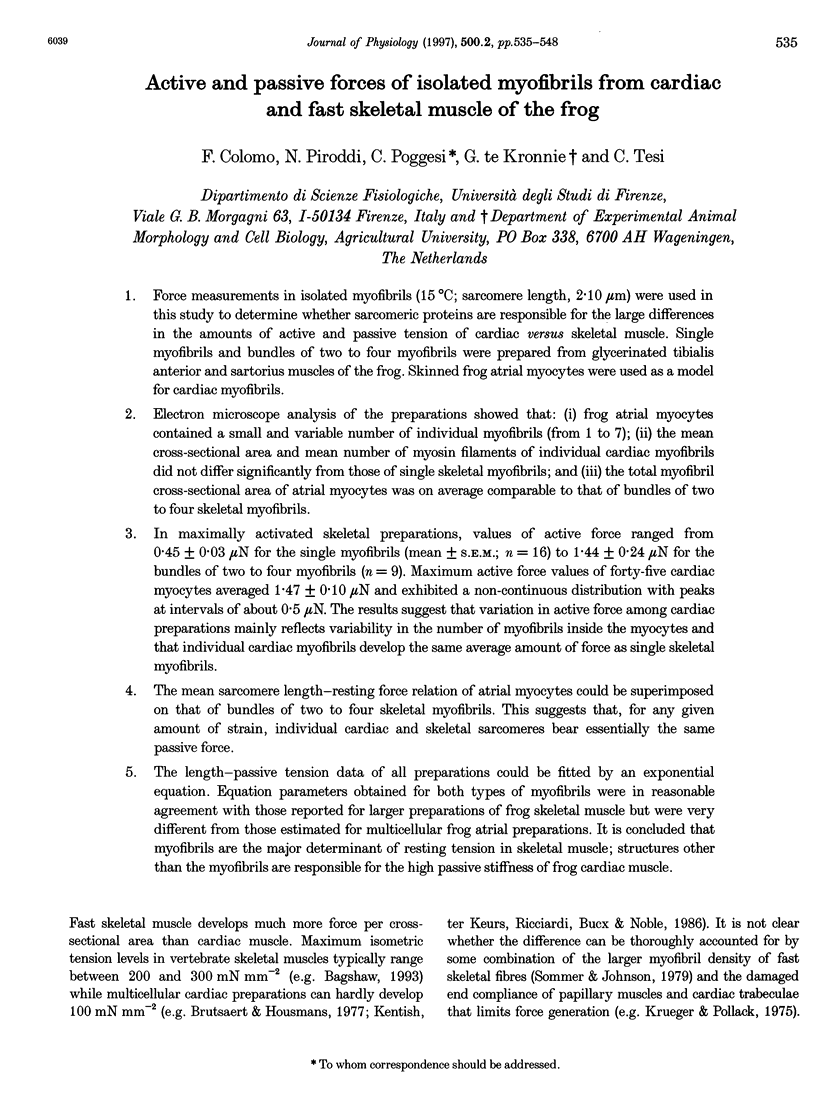
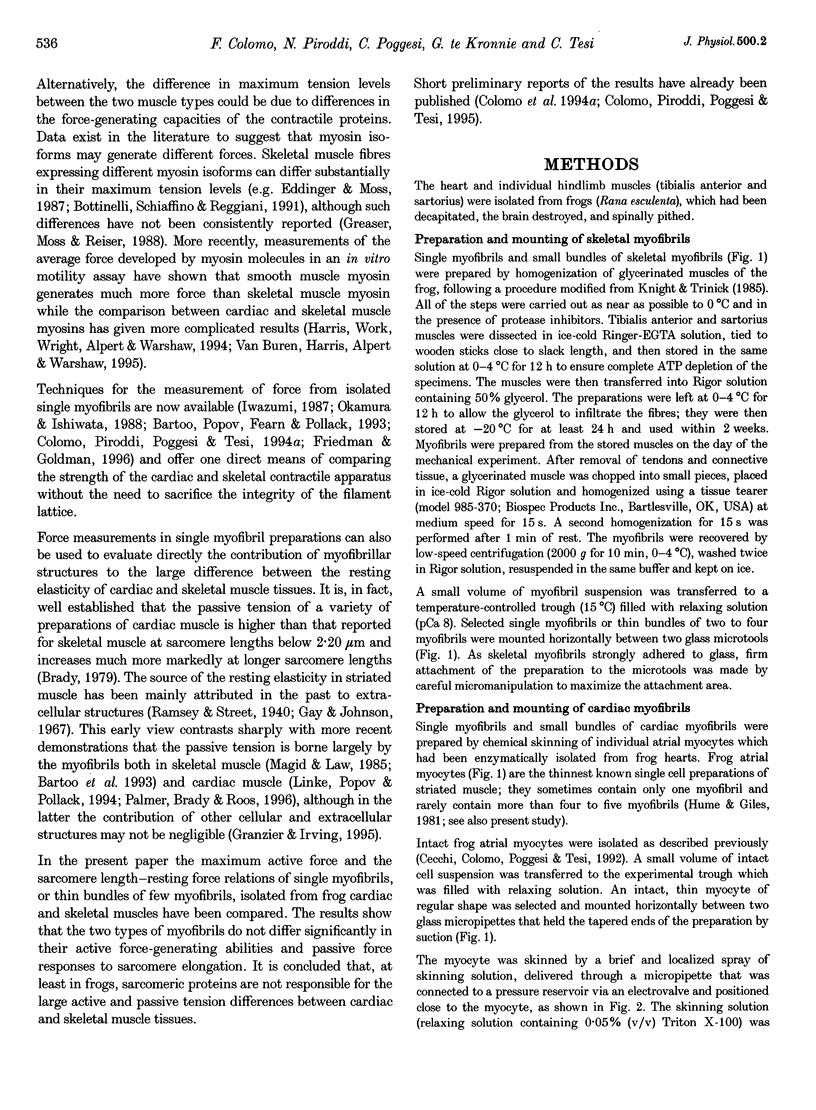
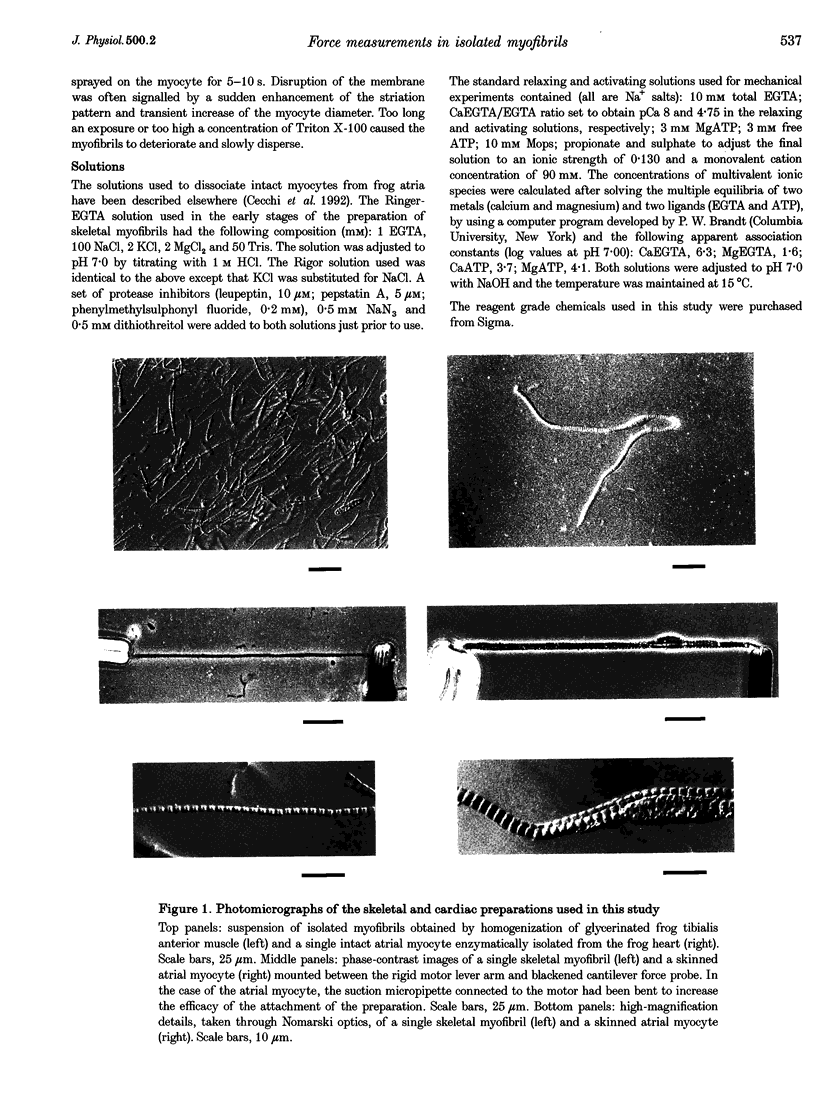
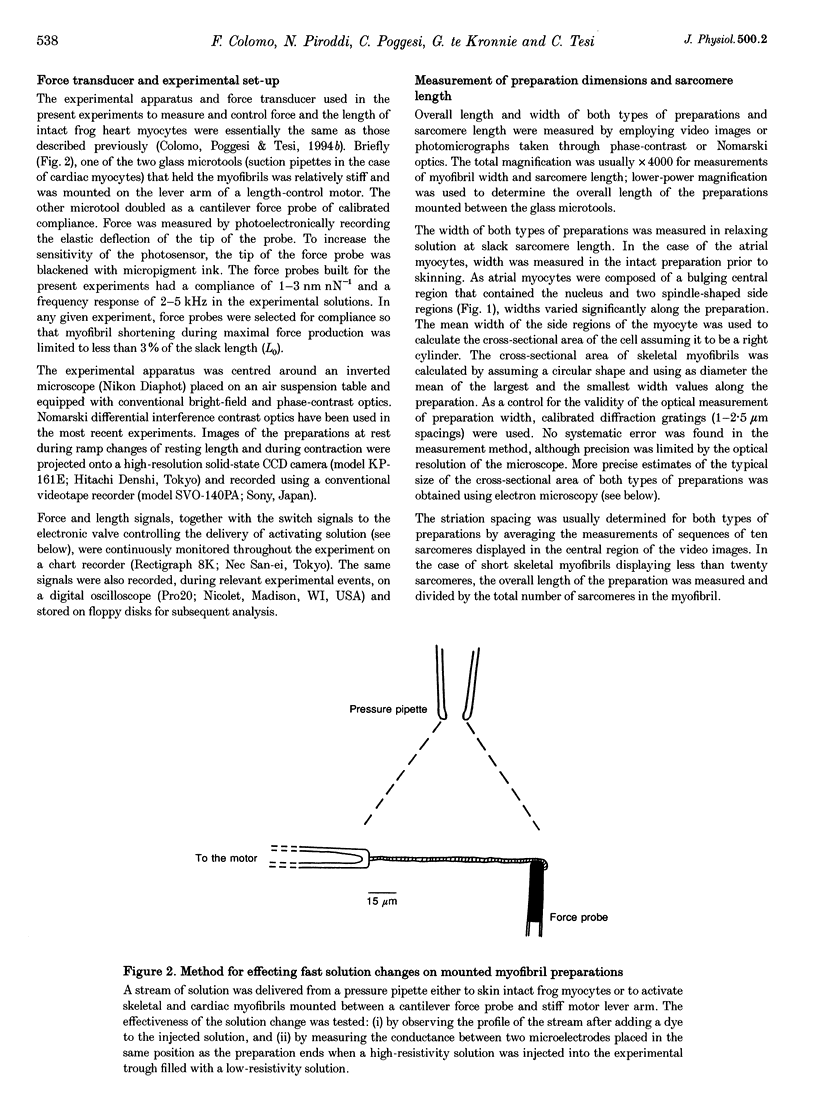
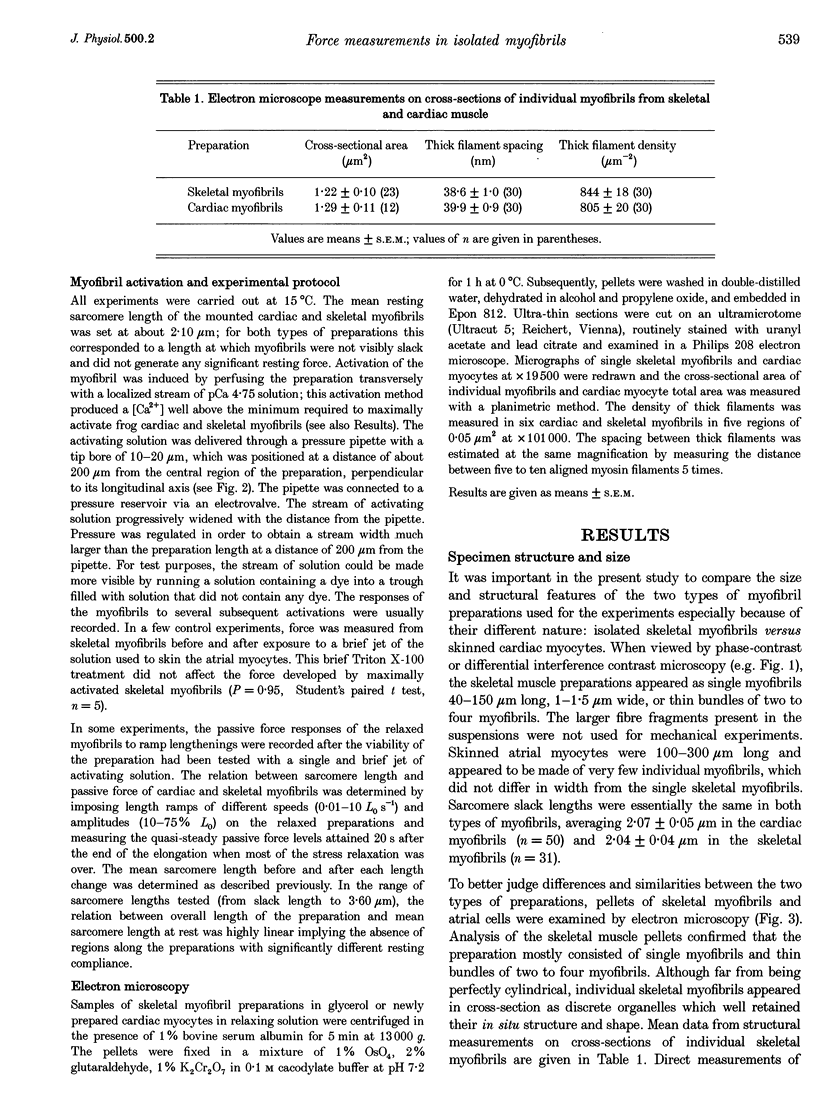
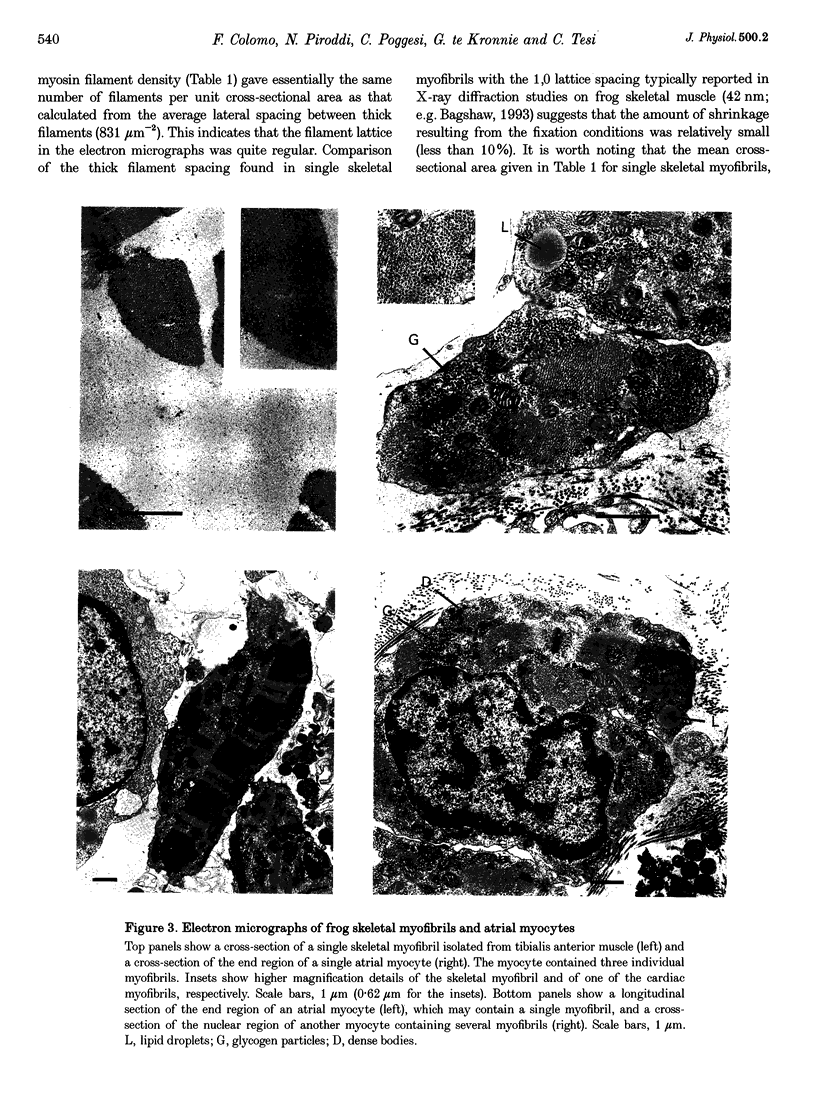
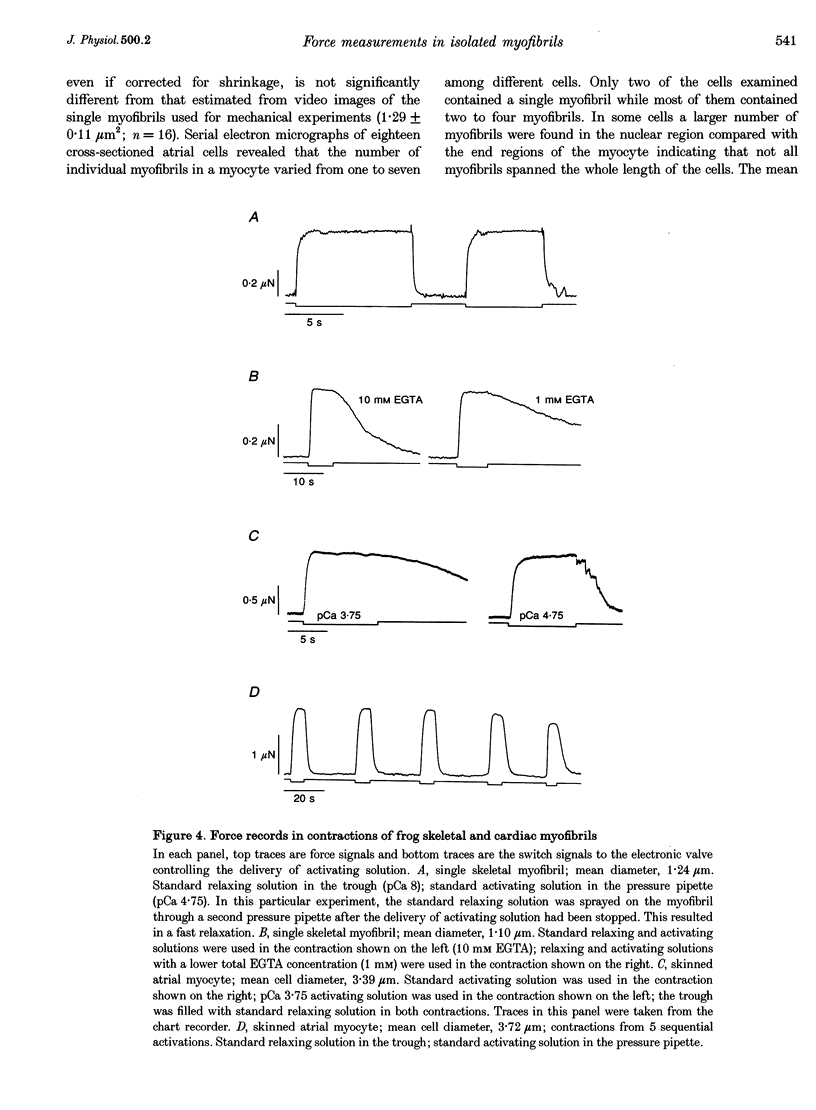
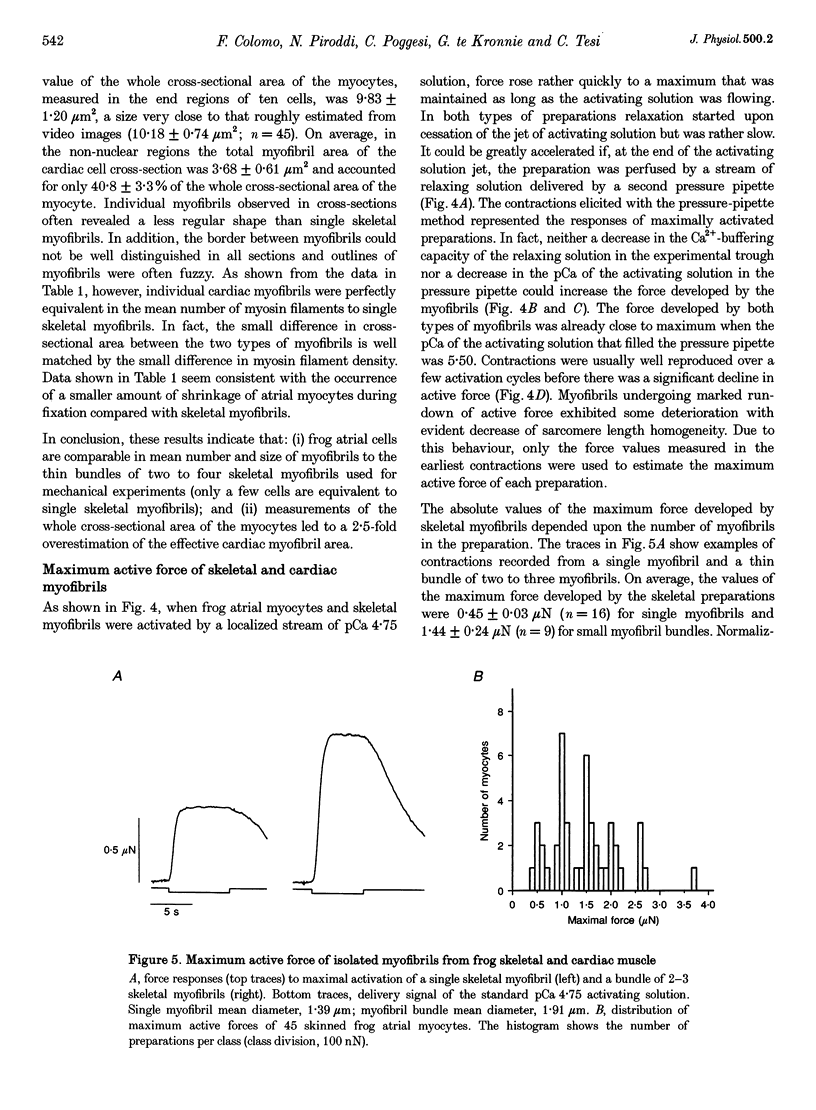
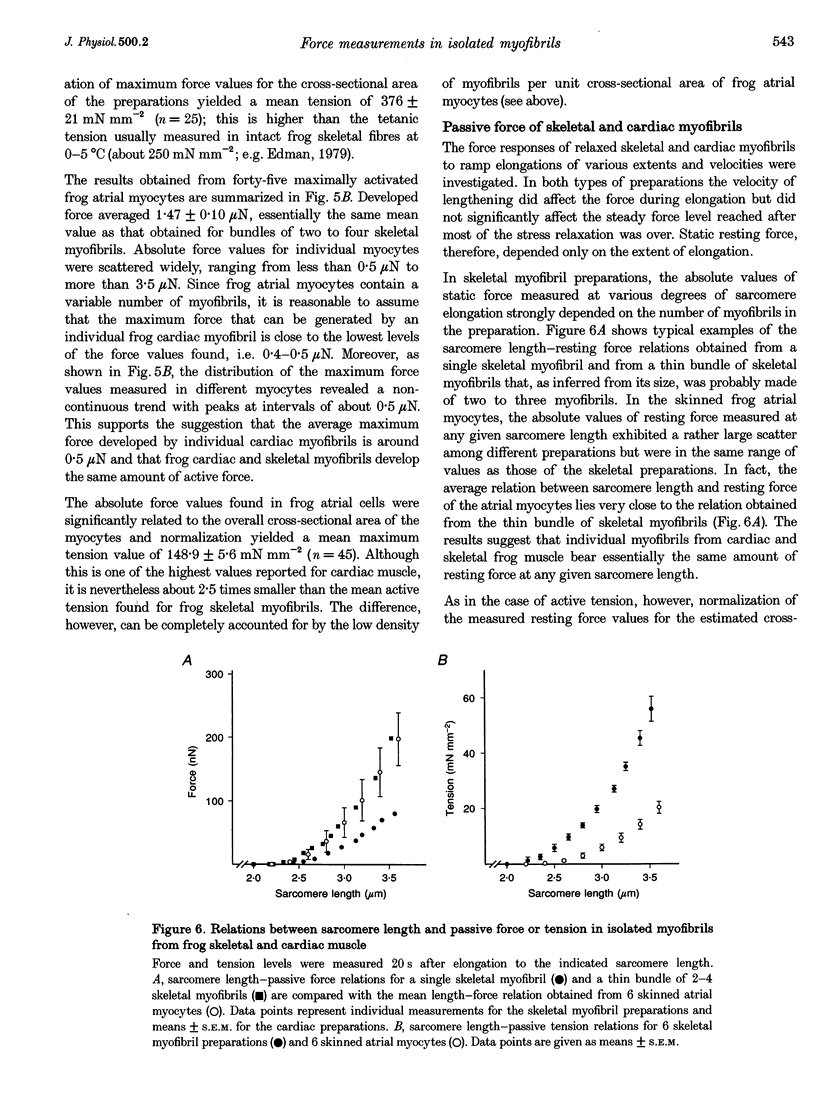
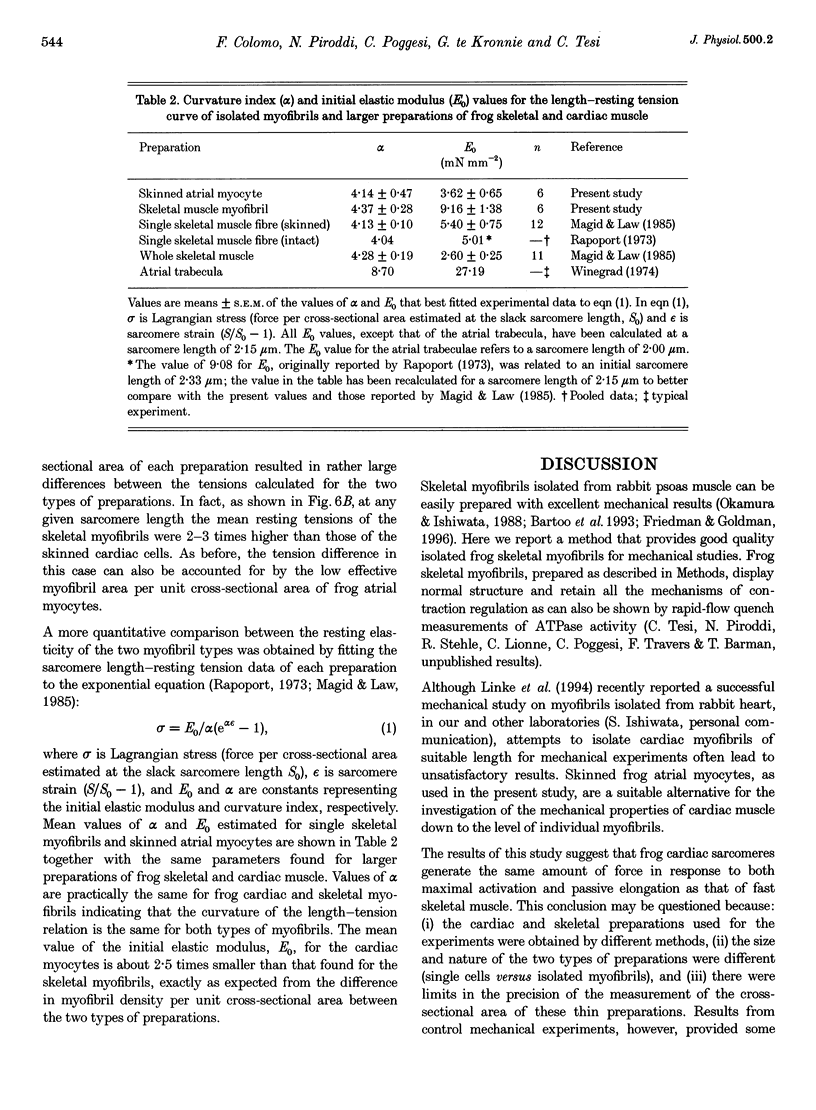
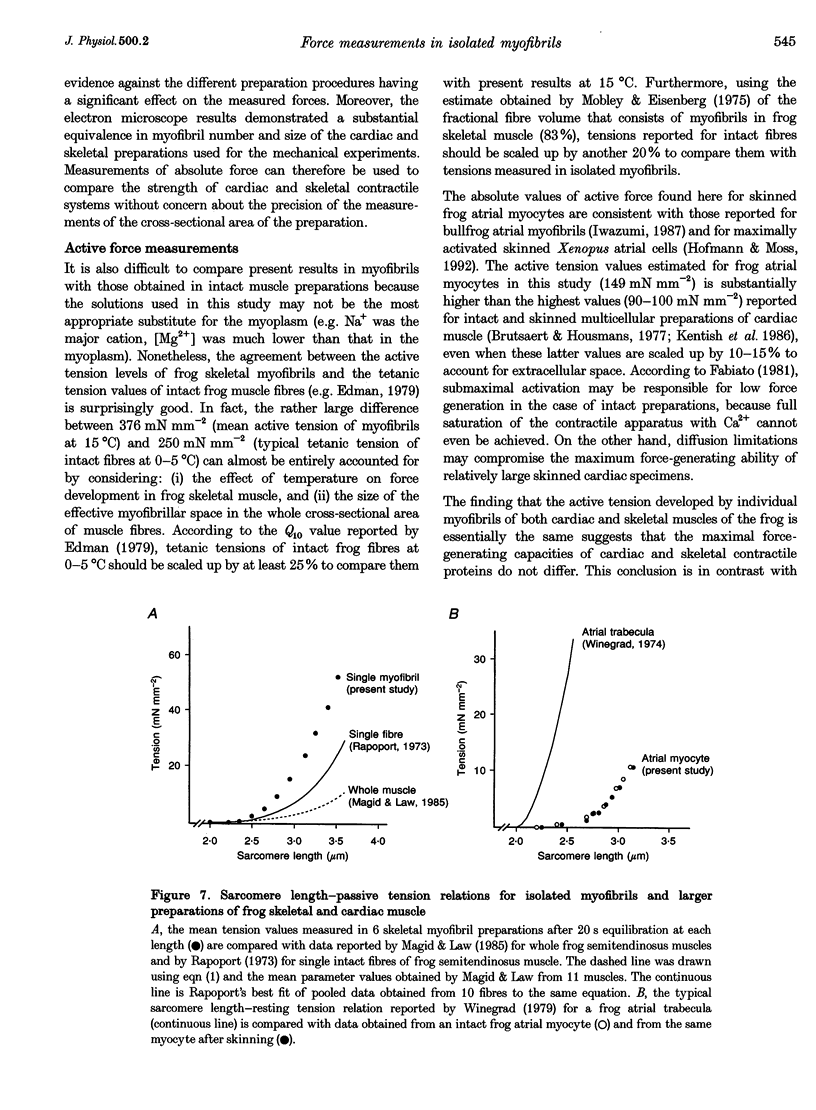
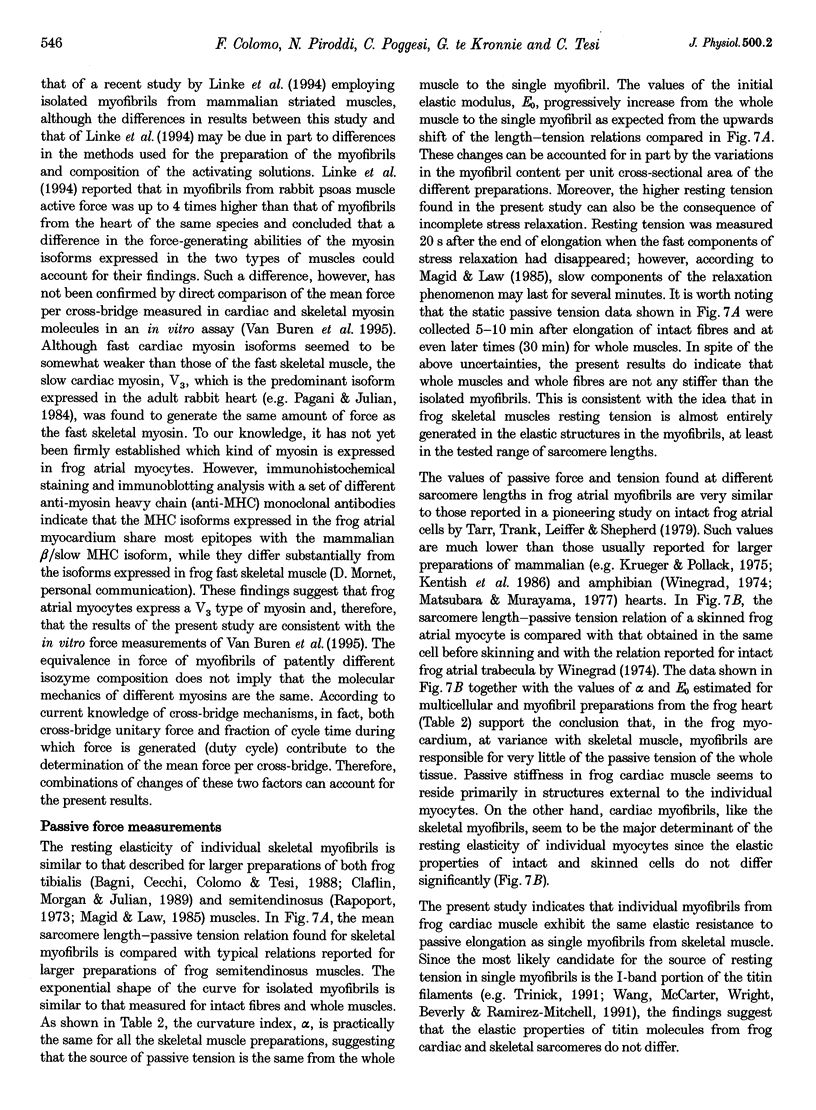
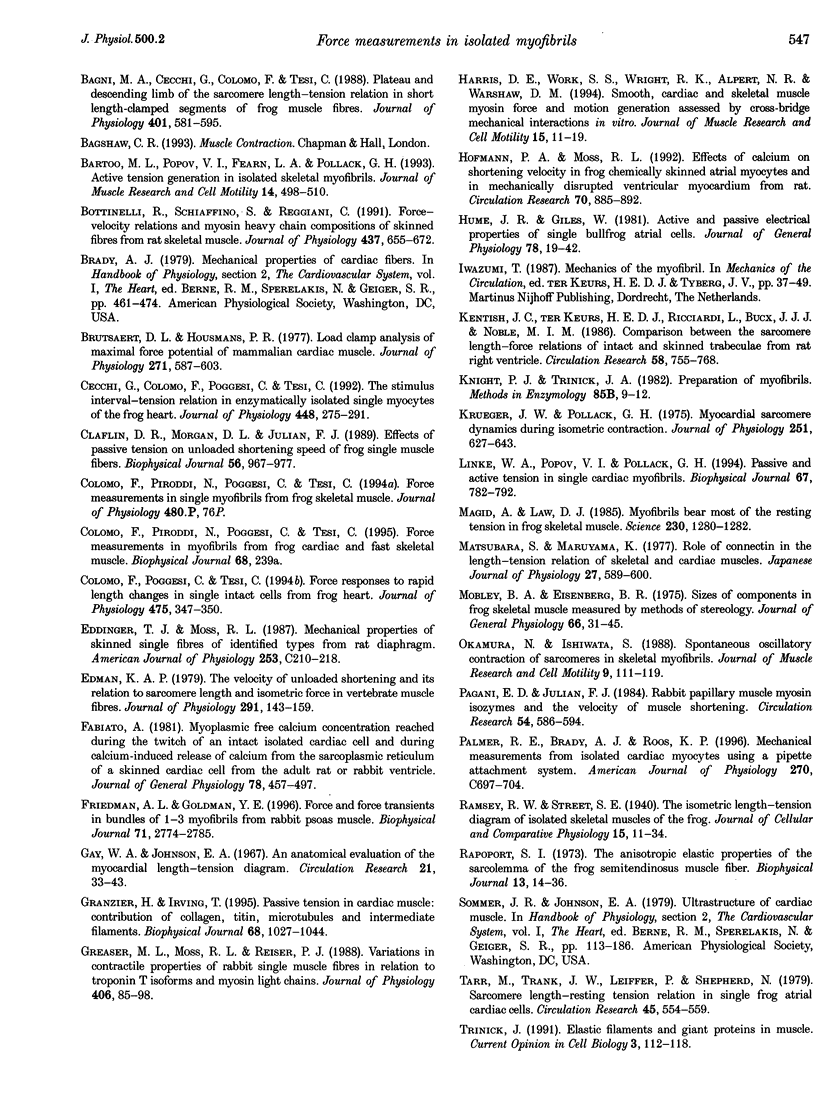
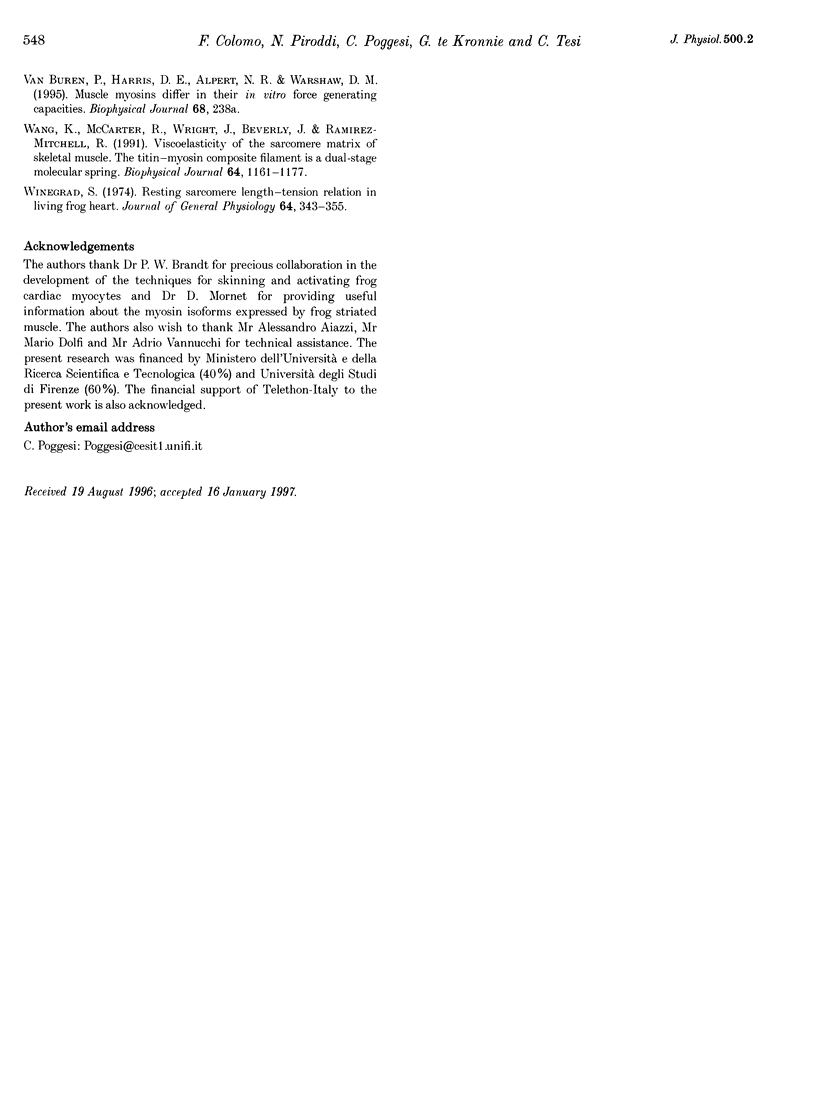
Images in this article
Selected References
These references are in PubMed. This may not be the complete list of references from this article.
- Bagni M. A., Cecchi G., Colomo F., Tesi C. Plateau and descending limb of the sarcomere length-tension relation in short length-clamped segments of frog muscle fibres. J Physiol. 1988 Jul;401:581–595. doi: 10.1113/jphysiol.1988.sp017181. [DOI] [PMC free article] [PubMed] [Google Scholar]
- Bartoo M. L., Popov V. I., Fearn L. A., Pollack G. H. Active tension generation in isolated skeletal myofibrils. J Muscle Res Cell Motil. 1993 Oct;14(5):498–510. doi: 10.1007/BF00297212. [DOI] [PubMed] [Google Scholar]
- Bottinelli R., Schiaffino S., Reggiani C. Force-velocity relations and myosin heavy chain isoform compositions of skinned fibres from rat skeletal muscle. J Physiol. 1991 Jun;437:655–672. doi: 10.1113/jphysiol.1991.sp018617. [DOI] [PMC free article] [PubMed] [Google Scholar]
- Brutsaert D. L., Housmans P. R. Load clamp analysis of maximal force potential of mammalian cardiac muscle. J Physiol. 1977 Oct;271(3):587–603. doi: 10.1113/jphysiol.1977.sp012016. [DOI] [PMC free article] [PubMed] [Google Scholar]
- Cecchi G., Colomo F., Poggesi C., Tesi C. The stimulus interval-tension relation in enzymatically isolated single myocytes of the frog heart. J Physiol. 1992 Mar;448:275–291. doi: 10.1113/jphysiol.1992.sp019041. [DOI] [PMC free article] [PubMed] [Google Scholar]
- Claflin D. R., Morgan D. L., Julian F. J. Effects of passive tension on unloaded shortening speed of frog single muscle fibers. Biophys J. 1989 Nov;56(5):967–977. doi: 10.1016/S0006-3495(89)82742-0. [DOI] [PMC free article] [PubMed] [Google Scholar]
- Colomo F., Poggesi C., Tesi C. Force responses to rapid length changes in single intact cells from frog heart. J Physiol. 1994 Mar 1;475(2):347–350. doi: 10.1113/jphysiol.1994.sp020075. [DOI] [PMC free article] [PubMed] [Google Scholar]
- Eddinger T. J., Moss R. L. Mechanical properties of skinned single fibers of identified types from rat diaphragm. Am J Physiol. 1987 Aug;253(2 Pt 1):C210–C218. doi: 10.1152/ajpcell.1987.253.2.C210. [DOI] [PubMed] [Google Scholar]
- Edman K. A. The velocity of unloaded shortening and its relation to sarcomere length and isometric force in vertebrate muscle fibres. J Physiol. 1979 Jun;291:143–159. doi: 10.1113/jphysiol.1979.sp012804. [DOI] [PMC free article] [PubMed] [Google Scholar]
- Fabiato A. Myoplasmic free calcium concentration reached during the twitch of an intact isolated cardiac cell and during calcium-induced release of calcium from the sarcoplasmic reticulum of a skinned cardiac cell from the adult rat or rabbit ventricle. J Gen Physiol. 1981 Nov;78(5):457–497. doi: 10.1085/jgp.78.5.457. [DOI] [PMC free article] [PubMed] [Google Scholar]
- Friedman A. L., Goldman Y. E. Mechanical characterization of skeletal muscle myofibrils. Biophys J. 1996 Nov;71(5):2774–2785. doi: 10.1016/S0006-3495(96)79470-5. [DOI] [PMC free article] [PubMed] [Google Scholar]
- Gay W. A., Jr, Johnson E. A. An anatomical evaluation of the myocardial length-tension diagram. Circ Res. 1967 Jul;21(1):33–43. doi: 10.1161/01.res.21.1.33. [DOI] [PubMed] [Google Scholar]
- Granzier H. L., Irving T. C. Passive tension in cardiac muscle: contribution of collagen, titin, microtubules, and intermediate filaments. Biophys J. 1995 Mar;68(3):1027–1044. doi: 10.1016/S0006-3495(95)80278-X. [DOI] [PMC free article] [PubMed] [Google Scholar]
- Greaser M. L., Moss R. L., Reiser P. J. Variations in contractile properties of rabbit single muscle fibres in relation to troponin T isoforms and myosin light chains. J Physiol. 1988 Dec;406:85–98. doi: 10.1113/jphysiol.1988.sp017370. [DOI] [PMC free article] [PubMed] [Google Scholar]
- Harris D. E., Work S. S., Wright R. K., Alpert N. R., Warshaw D. M. Smooth, cardiac and skeletal muscle myosin force and motion generation assessed by cross-bridge mechanical interactions in vitro. J Muscle Res Cell Motil. 1994 Feb;15(1):11–19. doi: 10.1007/BF00123828. [DOI] [PubMed] [Google Scholar]
- Hofmann P. A., Moss R. L. Effects of calcium on shortening velocity in frog chemically skinned atrial myocytes and in mechanically disrupted ventricular myocardium from rat. Circ Res. 1992 May;70(5):885–892. doi: 10.1161/01.res.70.5.885. [DOI] [PubMed] [Google Scholar]
- Hume J. R., Giles W. Active and passive electrical properties of single bullfrog atrial cells. J Gen Physiol. 1981 Jul;78(1):19–42. doi: 10.1085/jgp.78.1.19. [DOI] [PMC free article] [PubMed] [Google Scholar]
- Kentish J. C., ter Keurs H. E., Ricciardi L., Bucx J. J., Noble M. I. Comparison between the sarcomere length-force relations of intact and skinned trabeculae from rat right ventricle. Influence of calcium concentrations on these relations. Circ Res. 1986 Jun;58(6):755–768. doi: 10.1161/01.res.58.6.755. [DOI] [PubMed] [Google Scholar]
- Knight P. J., Trinick J. A. Preparation of myofibrils. Methods Enzymol. 1982;85(Pt B):9–12. doi: 10.1016/0076-6879(82)85004-0. [DOI] [PubMed] [Google Scholar]
- Krueger J. W., Pollack G. H. Myocardial sarcomere dynamics during isometric contraction. J Physiol. 1975 Oct;251(3):627–643. doi: 10.1113/jphysiol.1975.sp011112. [DOI] [PMC free article] [PubMed] [Google Scholar]
- Linke W. A., Popov V. I., Pollack G. H. Passive and active tension in single cardiac myofibrils. Biophys J. 1994 Aug;67(2):782–792. doi: 10.1016/S0006-3495(94)80538-7. [DOI] [PMC free article] [PubMed] [Google Scholar]
- Magid A., Law D. J. Myofibrils bear most of the resting tension in frog skeletal muscle. Science. 1985 Dec 13;230(4731):1280–1282. doi: 10.1126/science.4071053. [DOI] [PubMed] [Google Scholar]
- Matsubara S., Maruyama K. Role of connectin in the length-tension relation of skeletal and cardiac muscles. Jpn J Physiol. 1977;27(5):589–600. doi: 10.2170/jjphysiol.27.589. [DOI] [PubMed] [Google Scholar]
- Mobley B. A., Eisenberg B. R. Sizes of components in frog skeletal muscle measured by methods of stereology. J Gen Physiol. 1975 Jul;66(1):31–45. doi: 10.1085/jgp.66.1.31. [DOI] [PMC free article] [PubMed] [Google Scholar]
- Okamura N., Ishiwata S. Spontaneous oscillatory contraction of sarcomeres in skeletal myofibrils. J Muscle Res Cell Motil. 1988 Apr;9(2):111–119. doi: 10.1007/BF01773733. [DOI] [PubMed] [Google Scholar]
- Pagani E. D., Julian F. J. Rabbit papillary muscle myosin isozymes and the velocity of muscle shortening. Circ Res. 1984 May;54(5):586–594. doi: 10.1161/01.res.54.5.586. [DOI] [PubMed] [Google Scholar]
- Palmer R. E., Brady A. J., Roos K. P. Mechanical measurements from isolated cardiac myocytes using a pipette attachment system. Am J Physiol. 1996 Feb;270(2 Pt 1):C697–C704. doi: 10.1152/ajpcell.1996.270.2.C697. [DOI] [PubMed] [Google Scholar]
- Rapoport S. I. The anisotropic elastic properties of the sarcolemma of the frog semitendinosus muscle fiber. Biophys J. 1973 Jan;13(1):14–36. doi: 10.1016/S0006-3495(73)85967-3. [DOI] [PMC free article] [PubMed] [Google Scholar]
- Tarr M., Trank J. W., Leiffer P., Shepherd N. Sarcomere length-resting tension relation in single frog atrial cardiac cells. Circ Res. 1979 Oct;45(4):554–559. doi: 10.1161/01.res.45.4.554. [DOI] [PubMed] [Google Scholar]
- Trinick J. Elastic filaments and giant proteins in muscle. Curr Opin Cell Biol. 1991 Feb;3(1):112–119. doi: 10.1016/0955-0674(91)90173-v. [DOI] [PubMed] [Google Scholar]
- Wang K., McCarter R., Wright J., Beverly J., Ramirez-Mitchell R. Viscoelasticity of the sarcomere matrix of skeletal muscles. The titin-myosin composite filament is a dual-stage molecular spring. Biophys J. 1993 Apr;64(4):1161–1177. doi: 10.1016/S0006-3495(93)81482-6. [DOI] [PMC free article] [PubMed] [Google Scholar]
- Winegrad S. Resting sarcomere length-tension relation in living frog heart. J Gen Physiol. 1974 Sep;64(3):343–355. doi: 10.1085/jgp.64.3.343. [DOI] [PMC free article] [PubMed] [Google Scholar]