Abstract
1. We have studied the oscillatory activity of single neurons (91 recorded extracellularly and 76 intracellularly) in the primary visual cortex of cats and kittens to characterize its origins and its stimulus dependency. A new method for the detection of oscillations was developed in order to maximize the range of detectable frequencies in both types of recordings. Three types of activity were examined: spontaneous background activity, responses to intracellular current steps and visual responses. 2. During spontaneous activity, persistent oscillatory activity was very rare in both types of recordings. However, when intracellular records were made using KCl-filled micropipettes, spontaneous activity appeared rhythmic and contained repeated depolarizing events at a variety of frequencies, suggestive of tonic periodic inhibitory input normally masked at resting potential. 3. Patterns of firing activity in response to intracellular current steps allowed us to classify neurons as regular spiking, intrinsically bursting, and fast-spiking types, as described in vitro. In the case of rhythmically firing cells, the spike frequency increased with the amount of injected current. Subthreshold current-induced oscillations were rarely observed (2 out of 76 cells). 4. Visual stimulation elicited oscillations in one-third of the neurons (55 out of 167), predominantly in the 7-20 Hz frequency range in 93% of the cases. Rhythmicity was observed in both simple and complex cells, and appeared to be more prominent at 5 and 6 weeks of age. 5. Intracellular recordings in bridge mode and voltage clamp revealed that visually evoked oscillations were driven by synaptic activity and did not depend primarily on the intrinsic properties of recorded neurons. Hyperpolarizing the membrane led to an increase in the size of the rhythmic depolarizing events without a change in frequency. In voltage-clamped cells, current responses showed large oscillations at the same frequency as in bridge mode, independently of the actual value of the holding potential. 6. In fourteen intracellularly recorded neurons, oscillations consisted of excitatory events that could be superimposed on a depolarizing or a hyperpolarizing slow wave. In two other neurons, visual responses consisted of excitatory and inhibitory events, alternating with a constant phase shift. 7. Drifting bars were much more efficient in evoking oscillatory responses than flashed bars. Except in three cells, the frequency of the oscillation did not depend on the physical characteristics of the stimulus that were tested (contrast, orientation, direction, ocularity and position in the receptive field). No significant correlation was found between the intensity of the visual response and the strength of the rhythmic component. 8. Although it cannot be excluded that the dominant frequency of oscillations might be related to the type of anaesthetics used, no correlation was found between local EEG and the oscillatory activity elicited by visual stimulation. 9. We conclude that the oscillations observed in the present work are generated by synaptic activity. It is likely that they represent an important mode of transmission in sensory processing, resulting from periodic packets of synchronized activity propagated across recurrent circuits. Their relevance to perceptual binding is further discussed.
Full text
PDF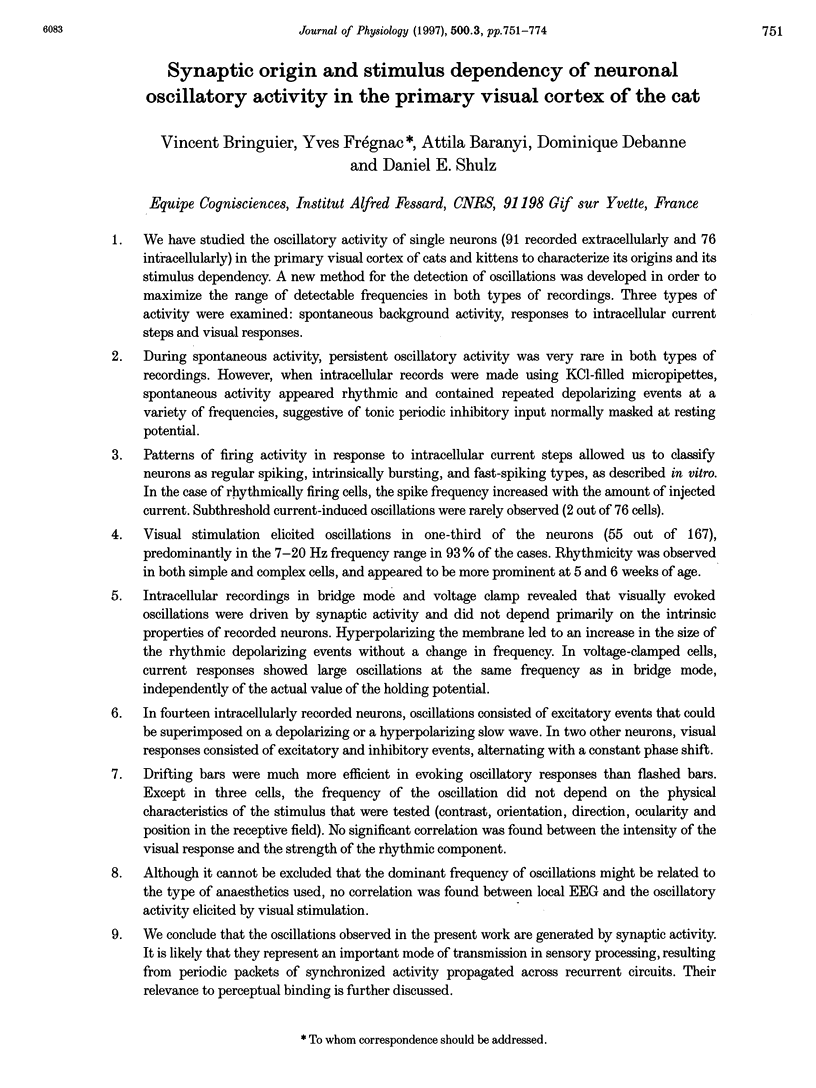
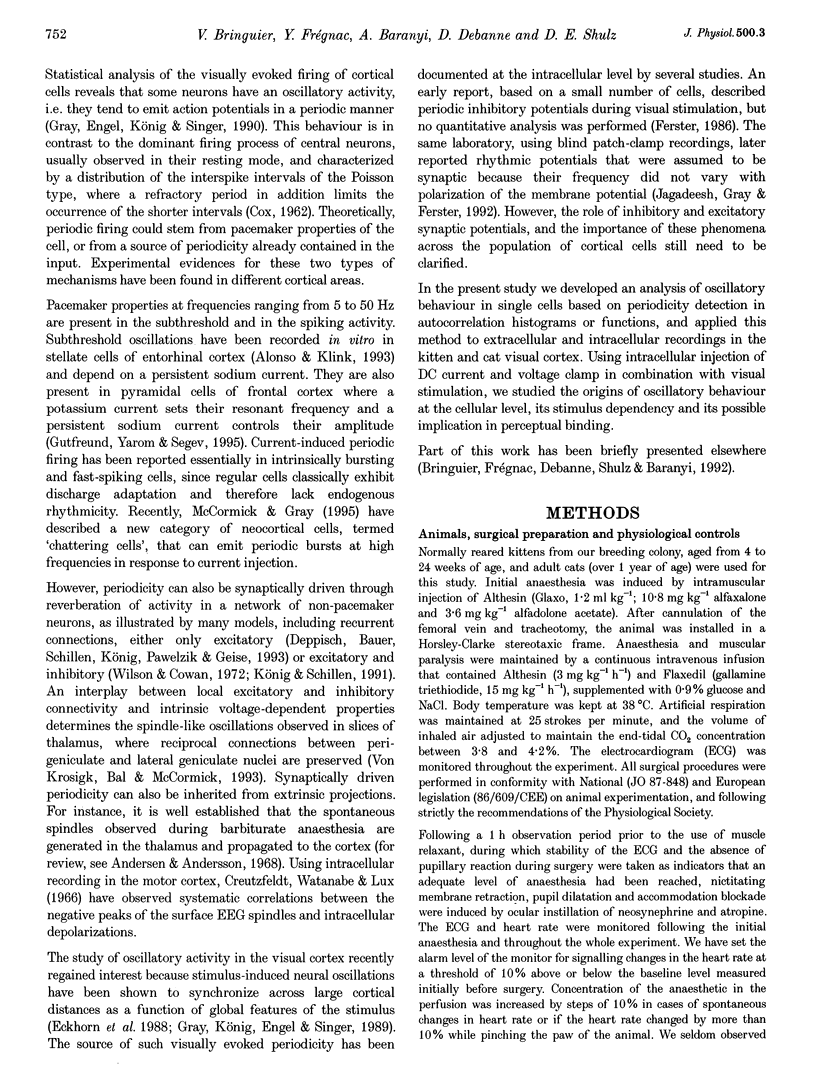
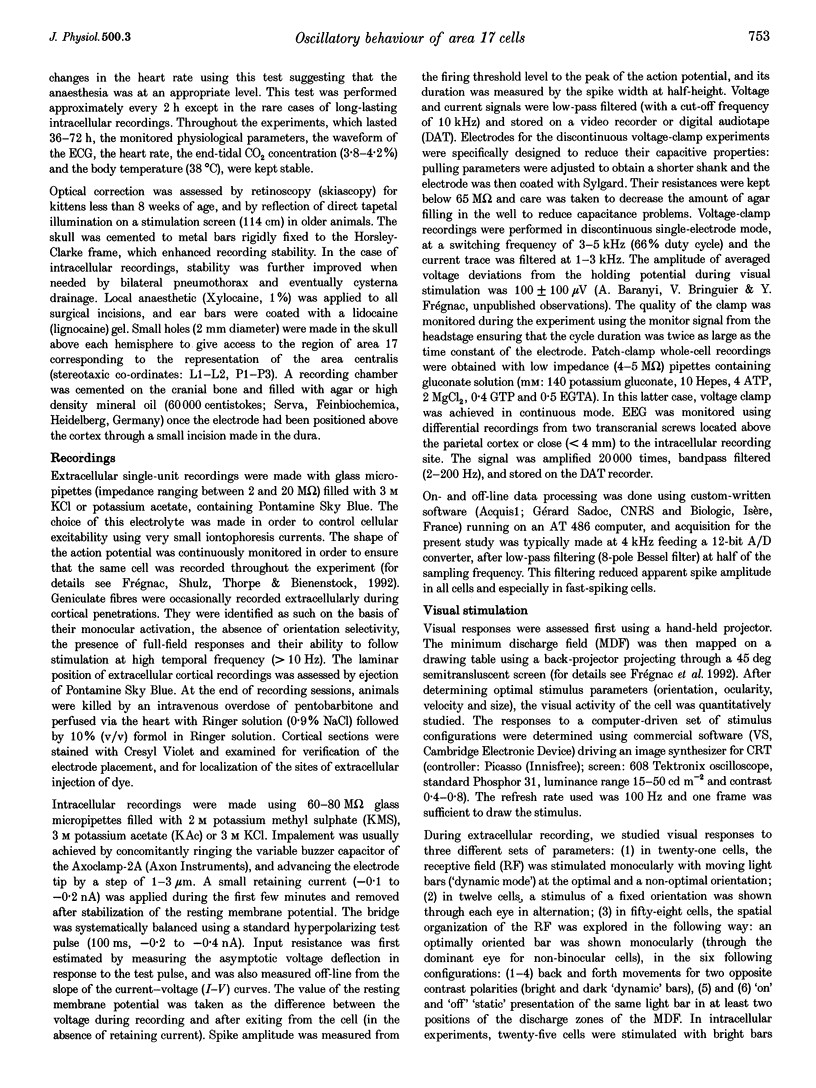
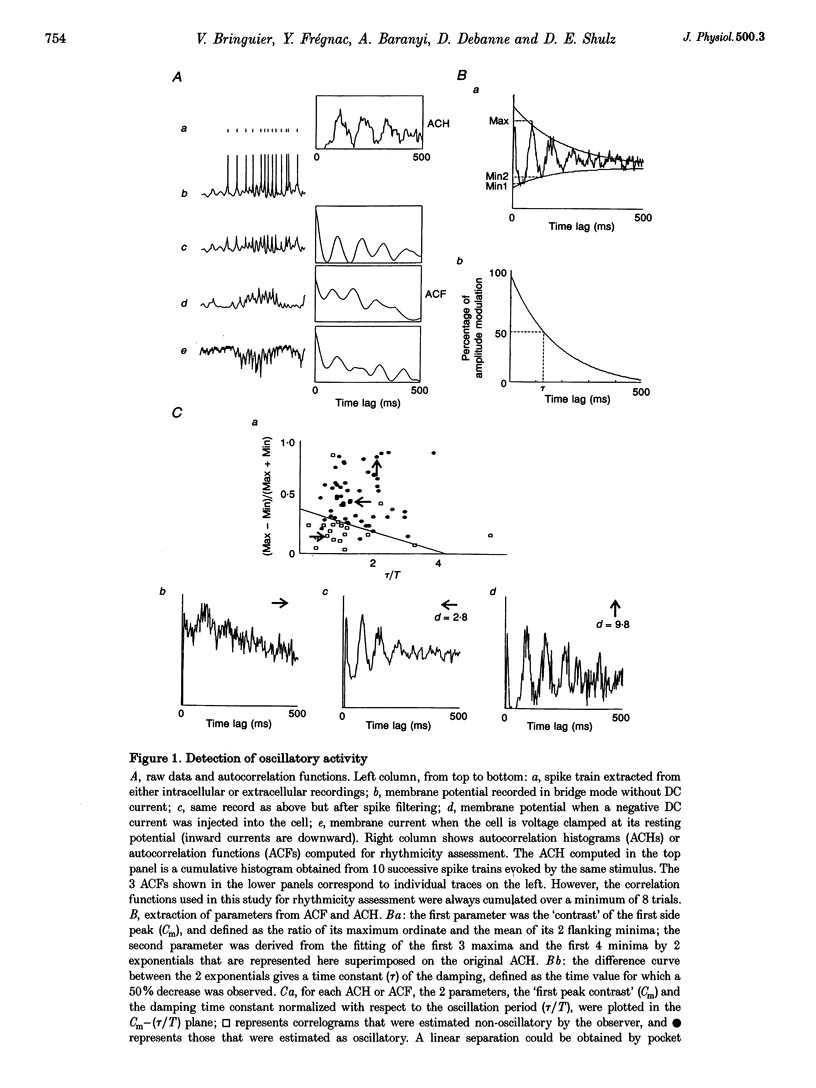
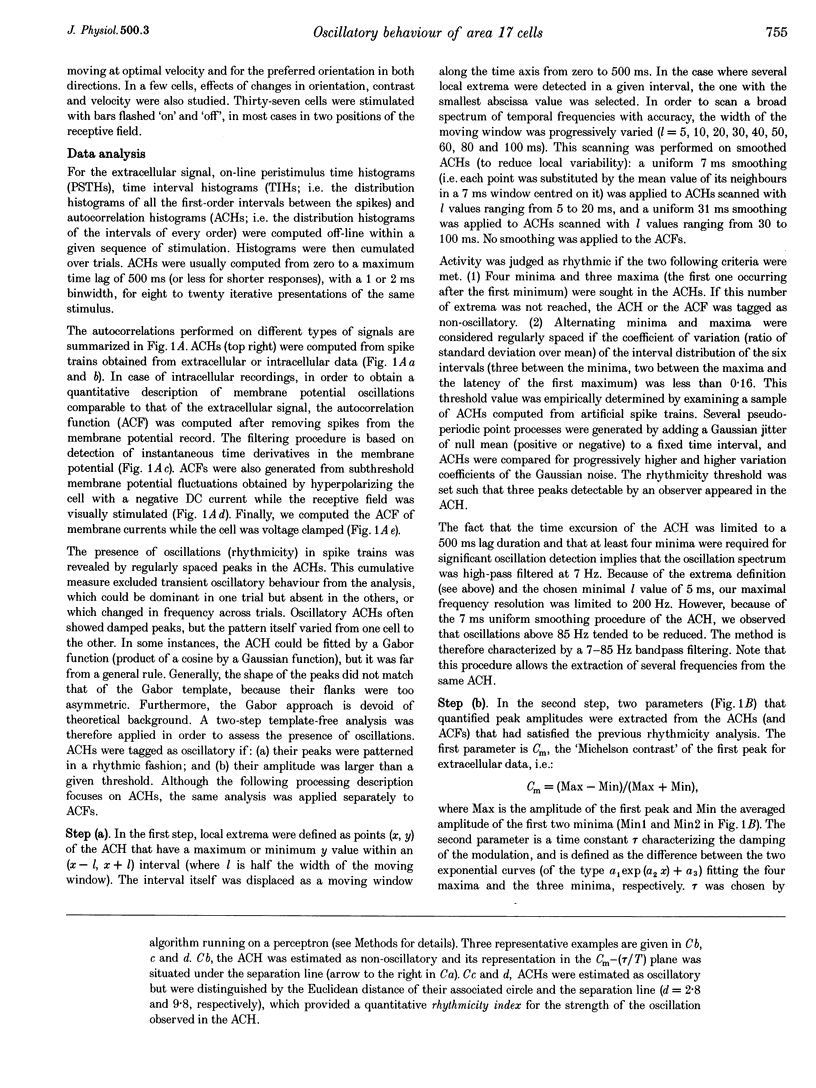
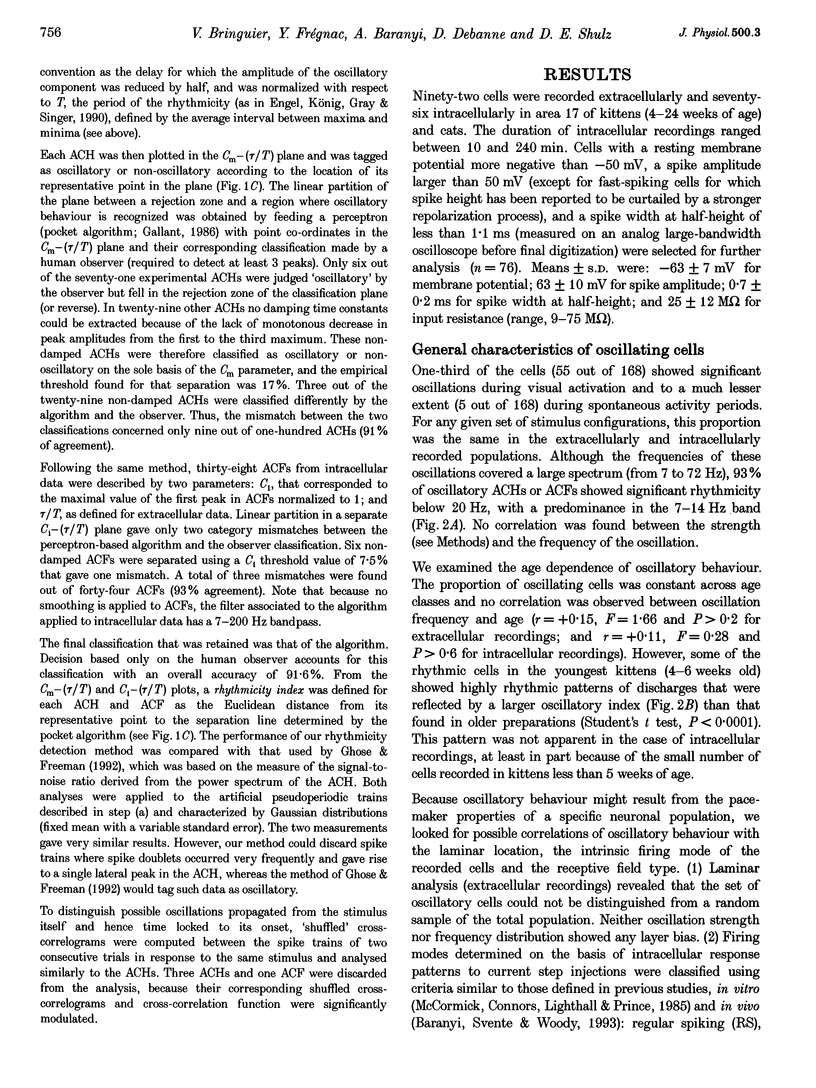
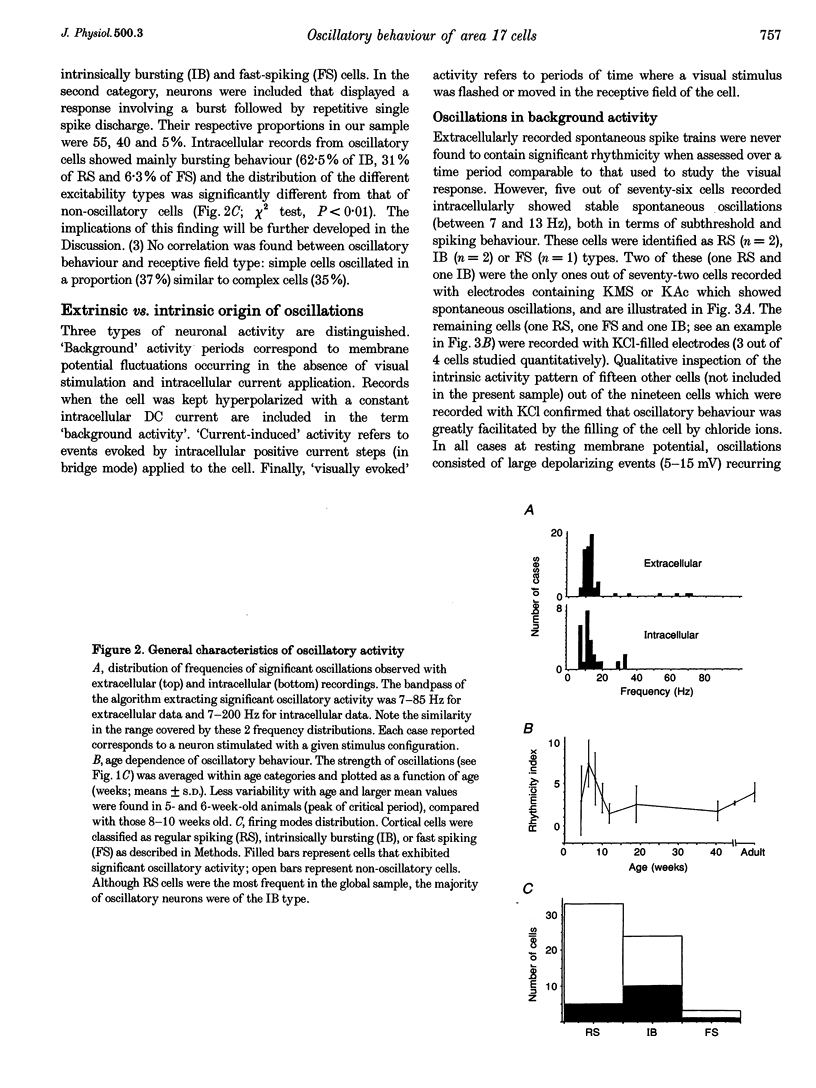
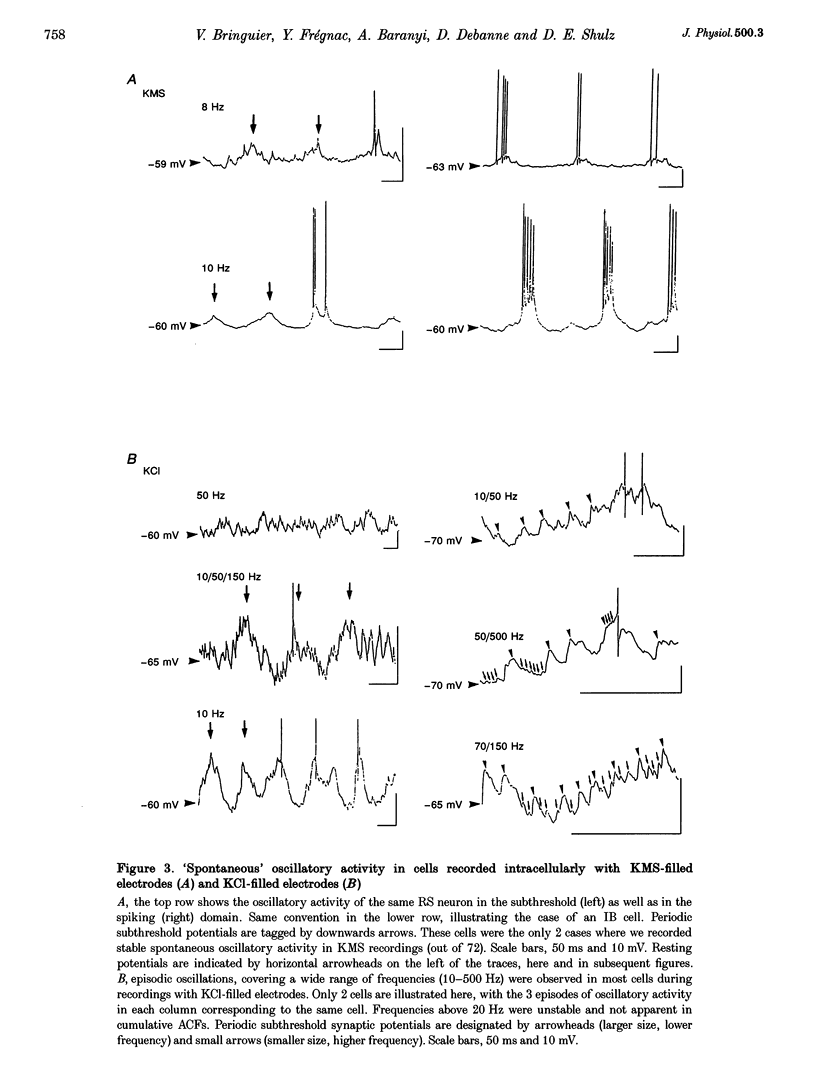
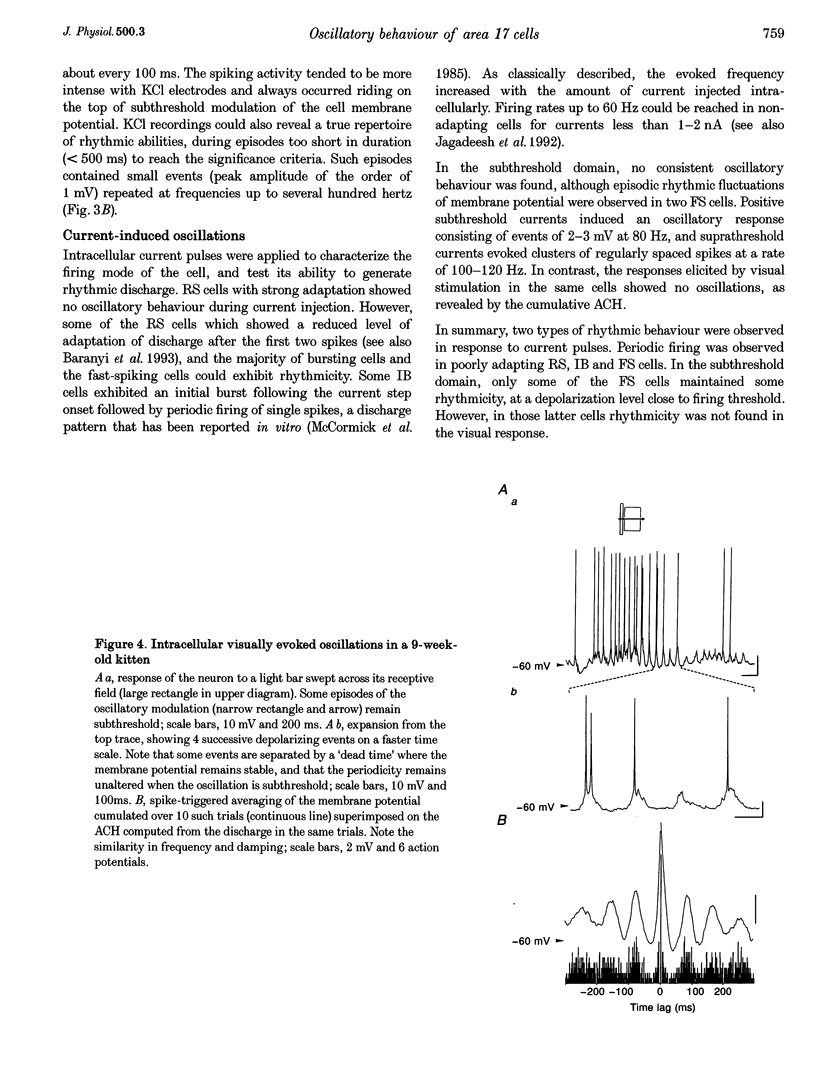
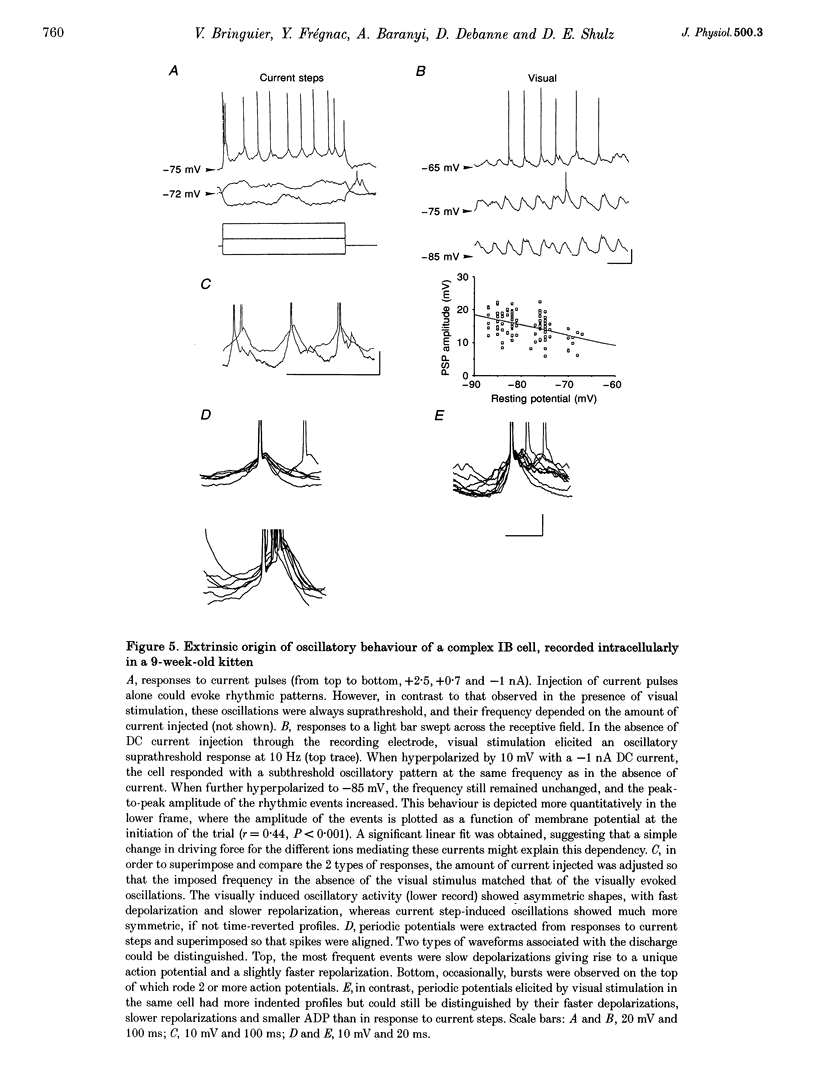
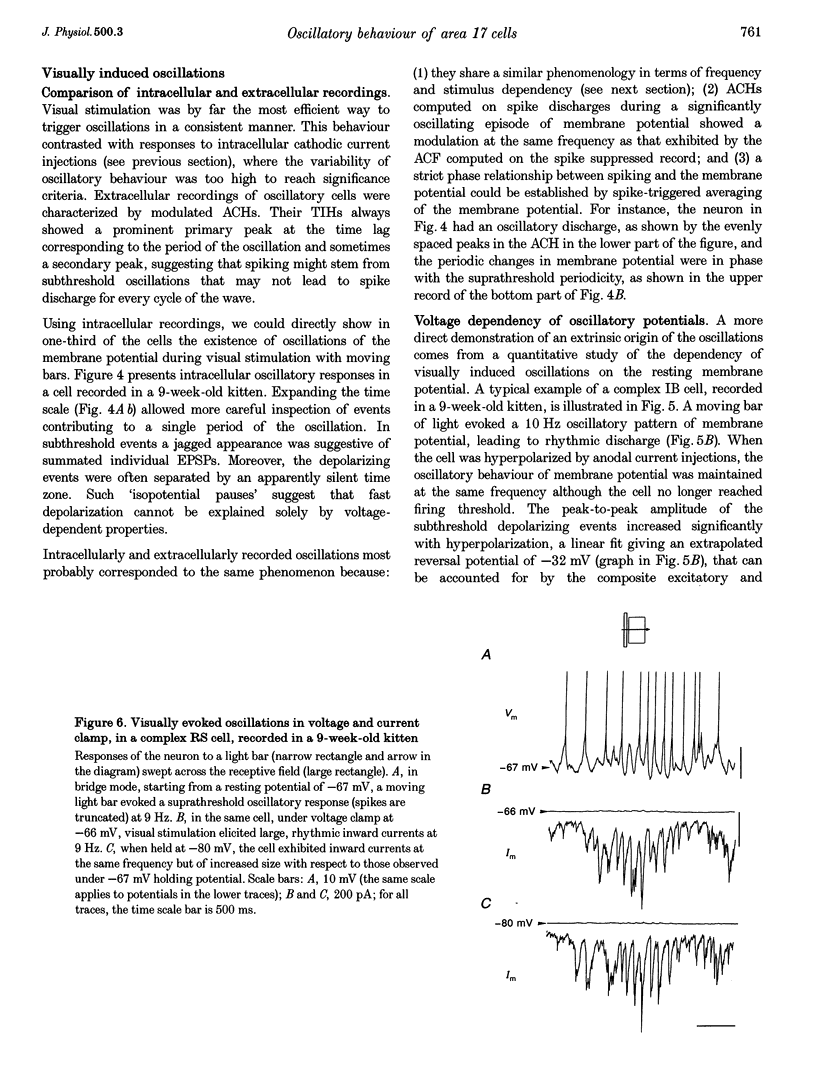
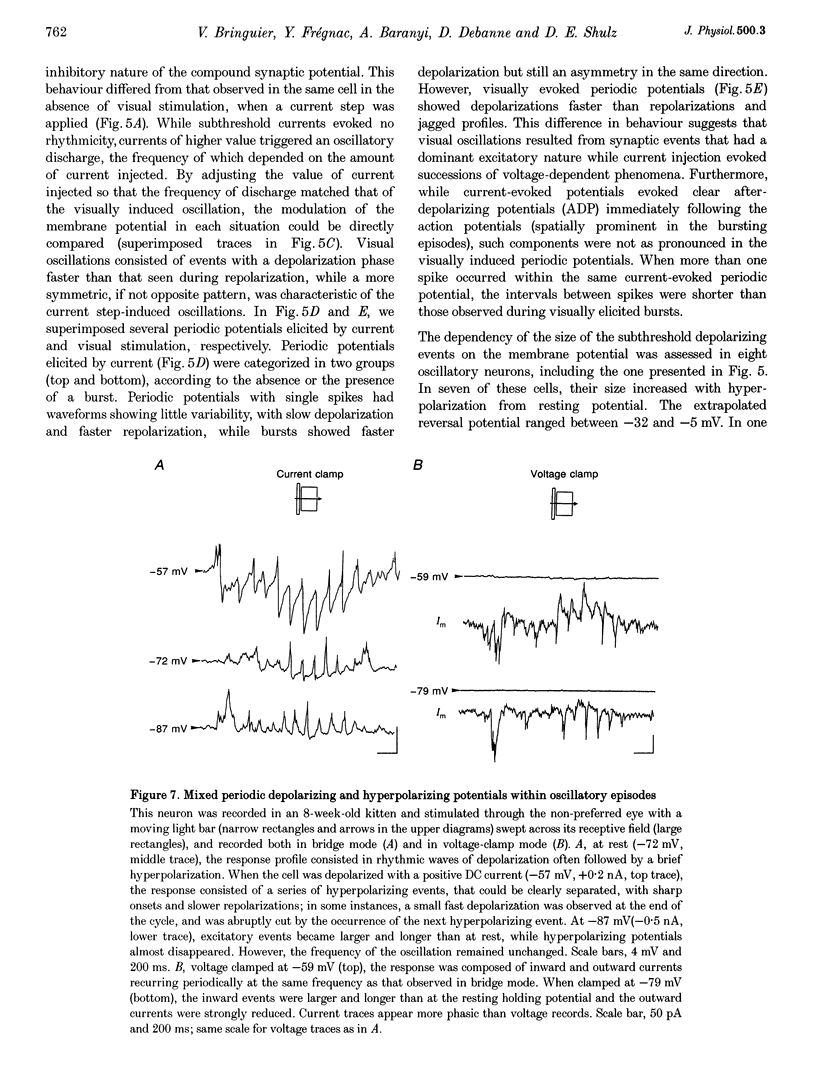
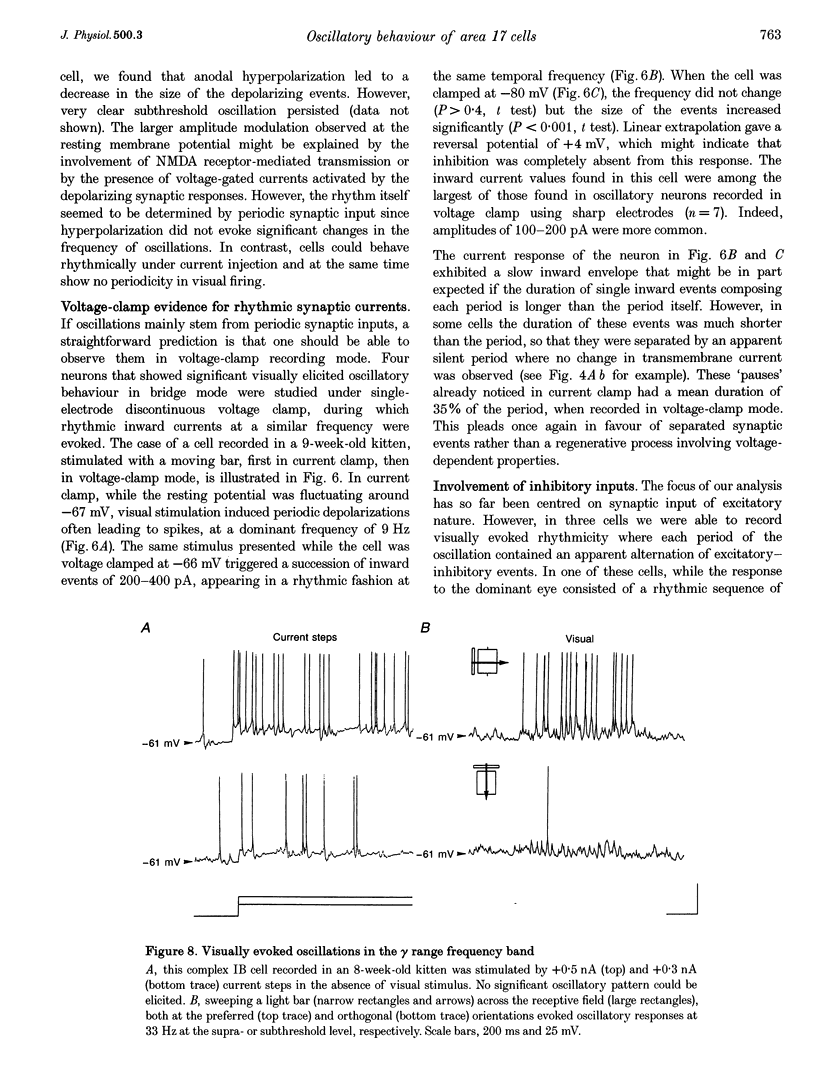
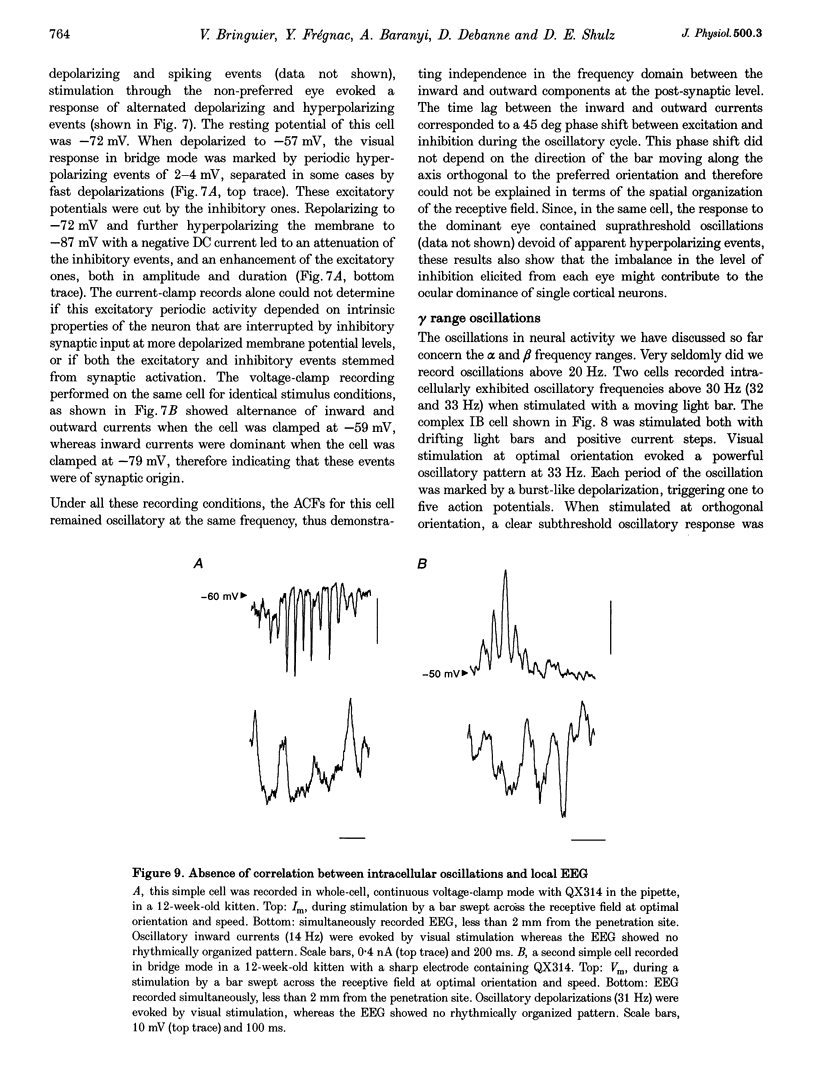
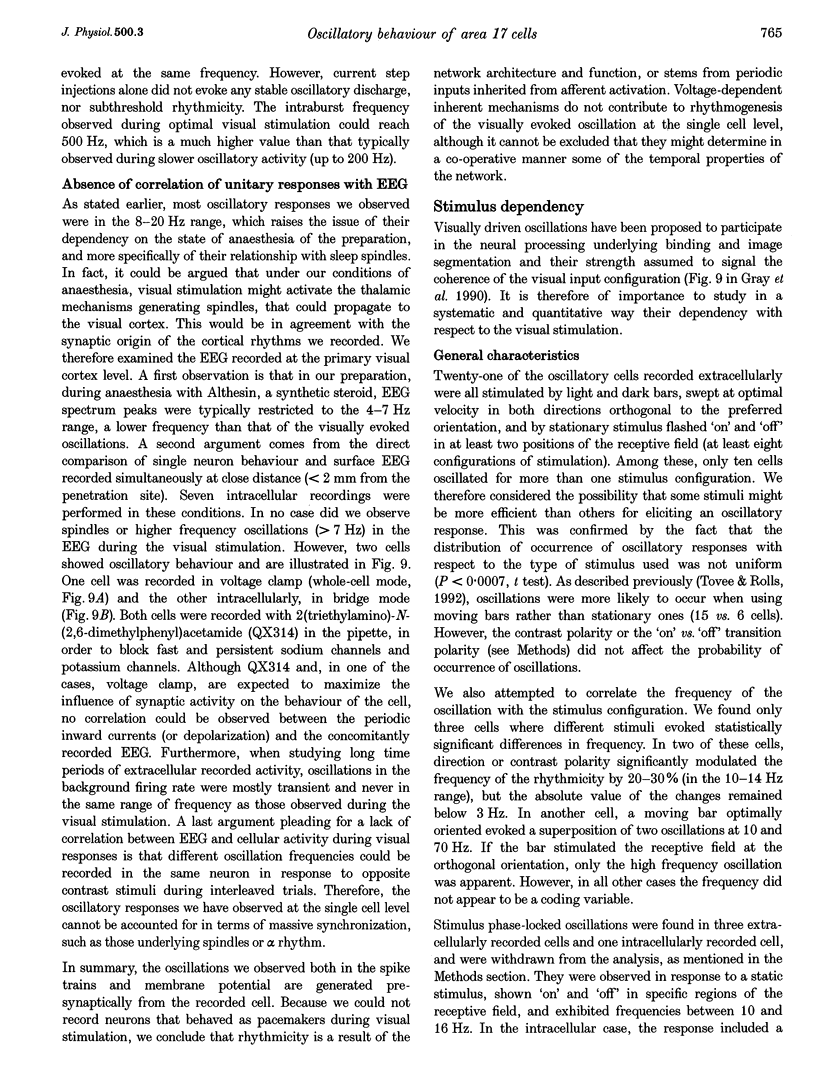
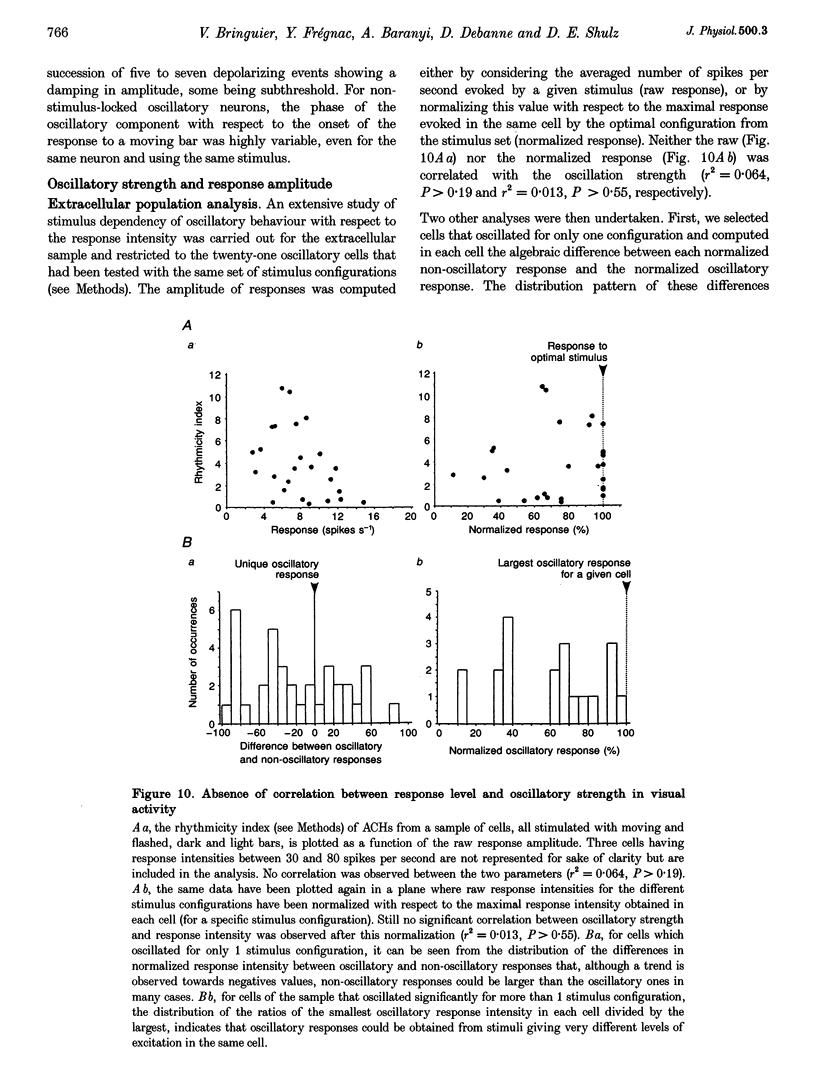
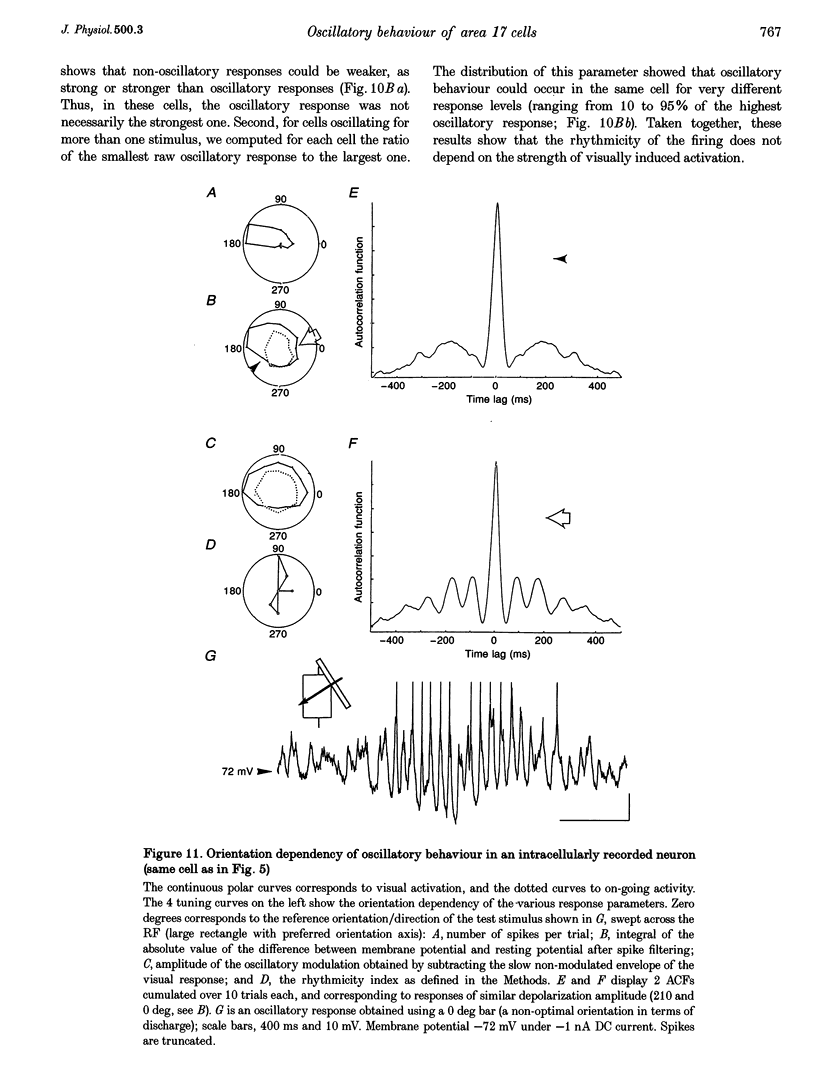
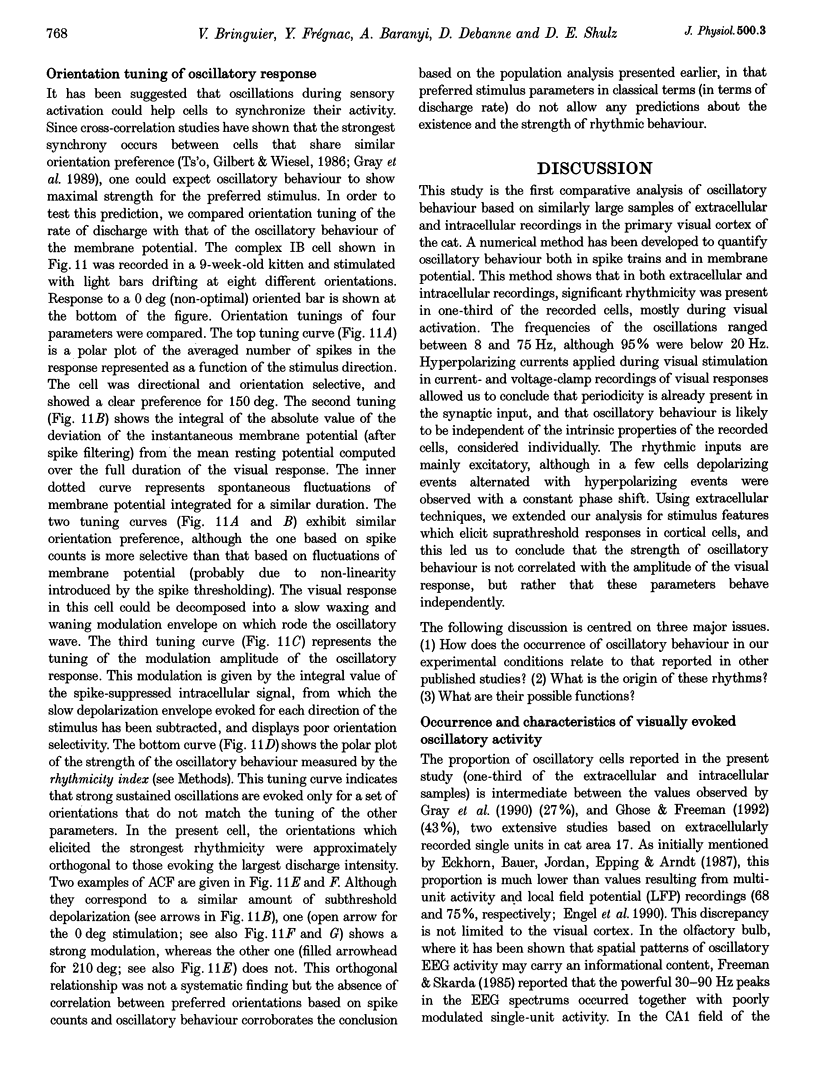
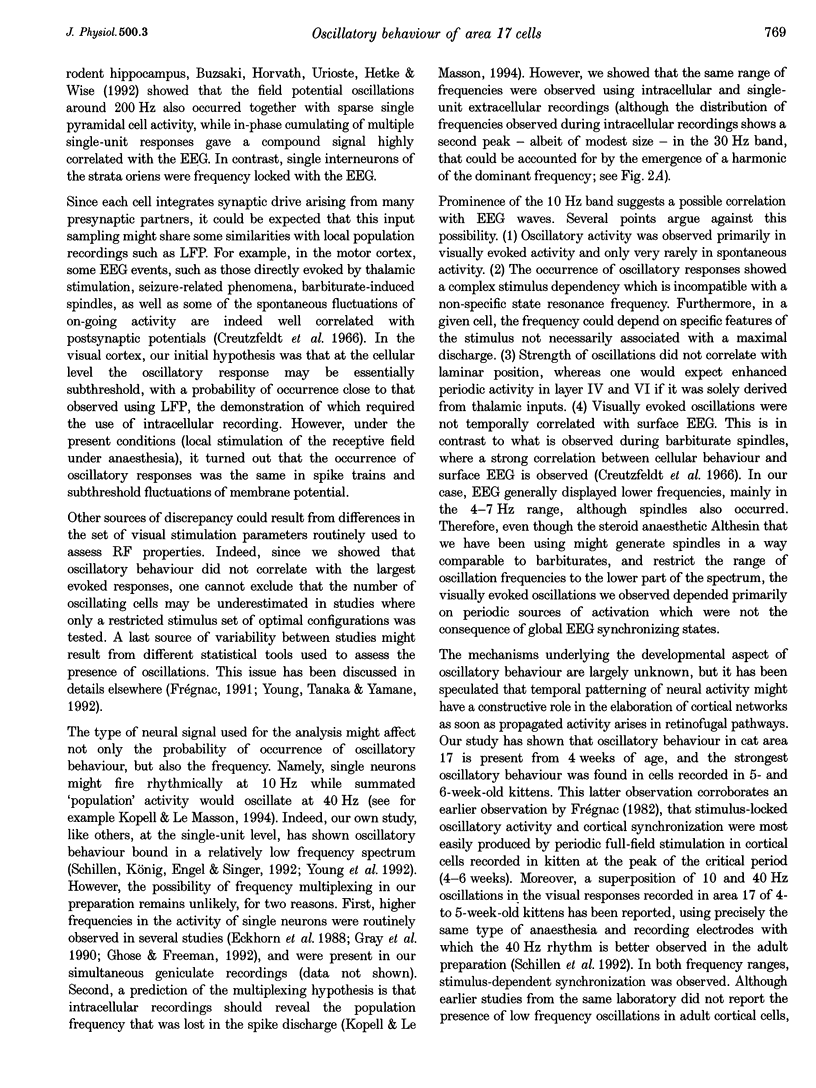
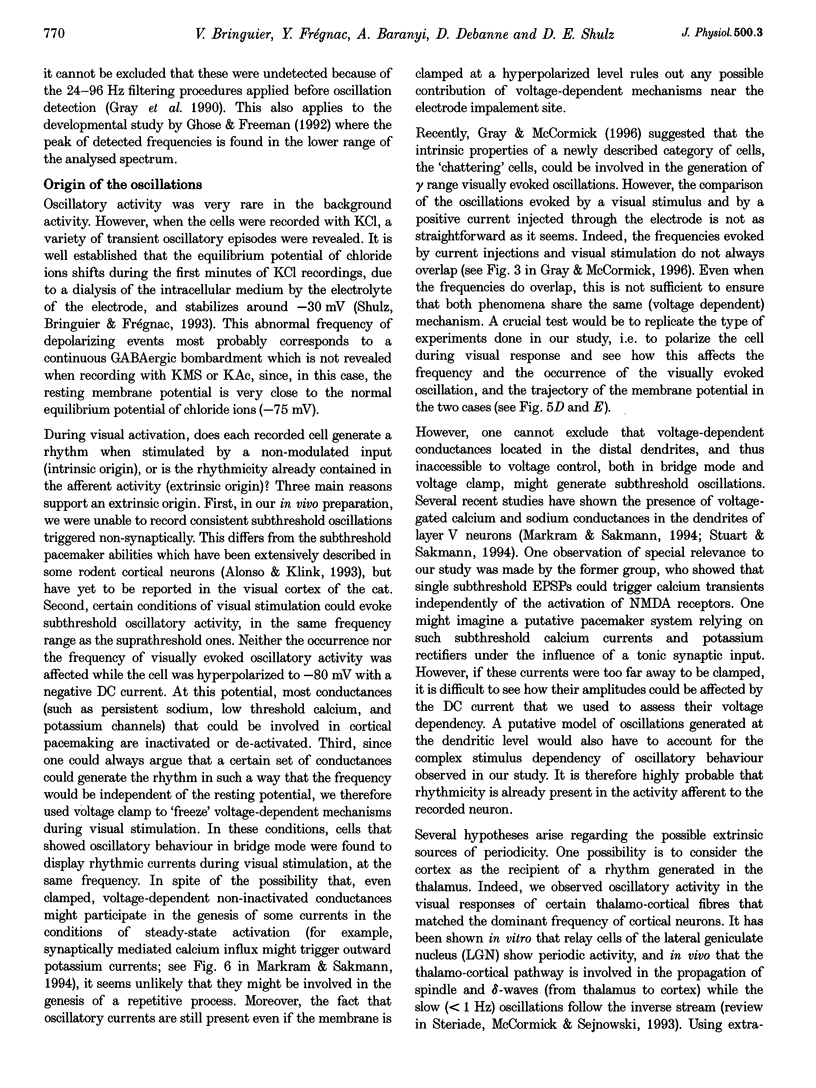
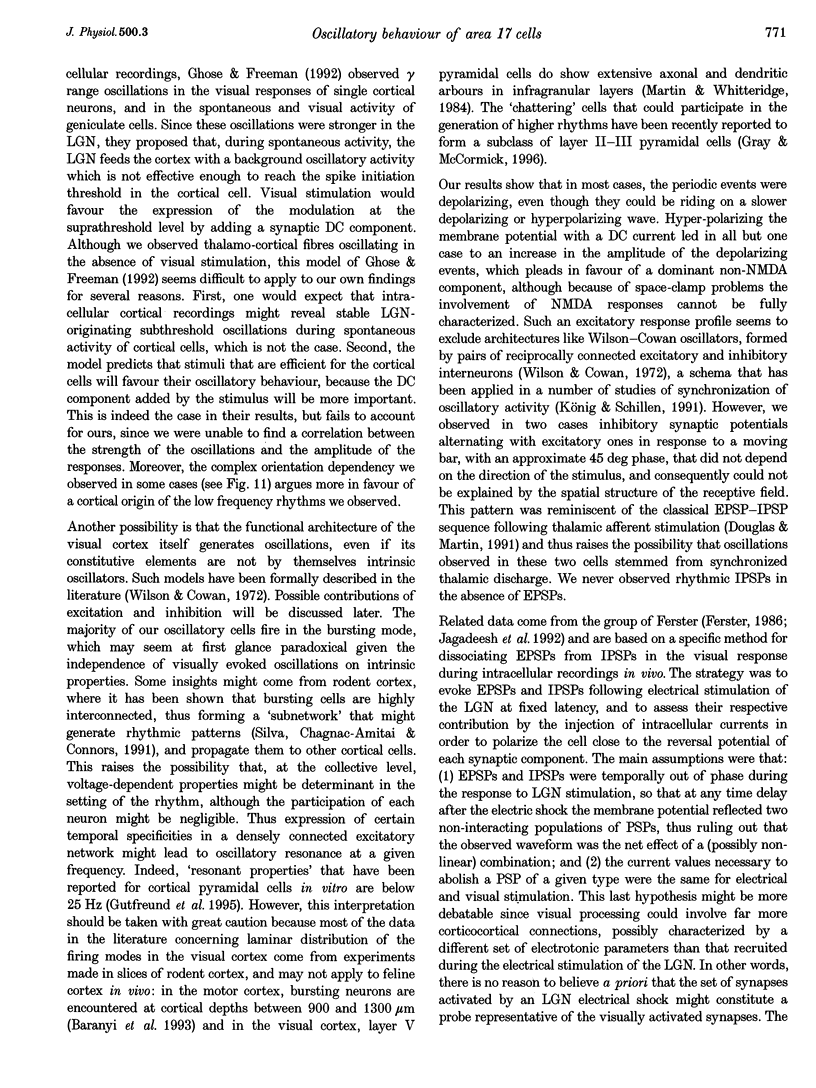
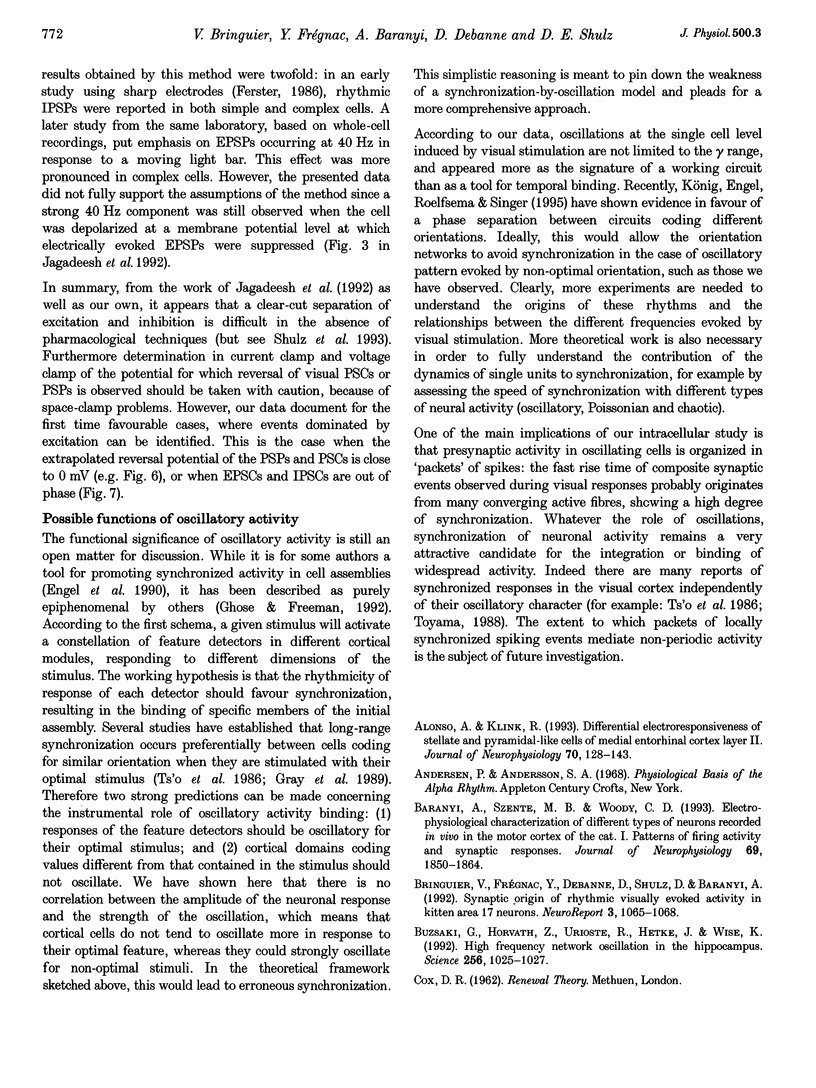
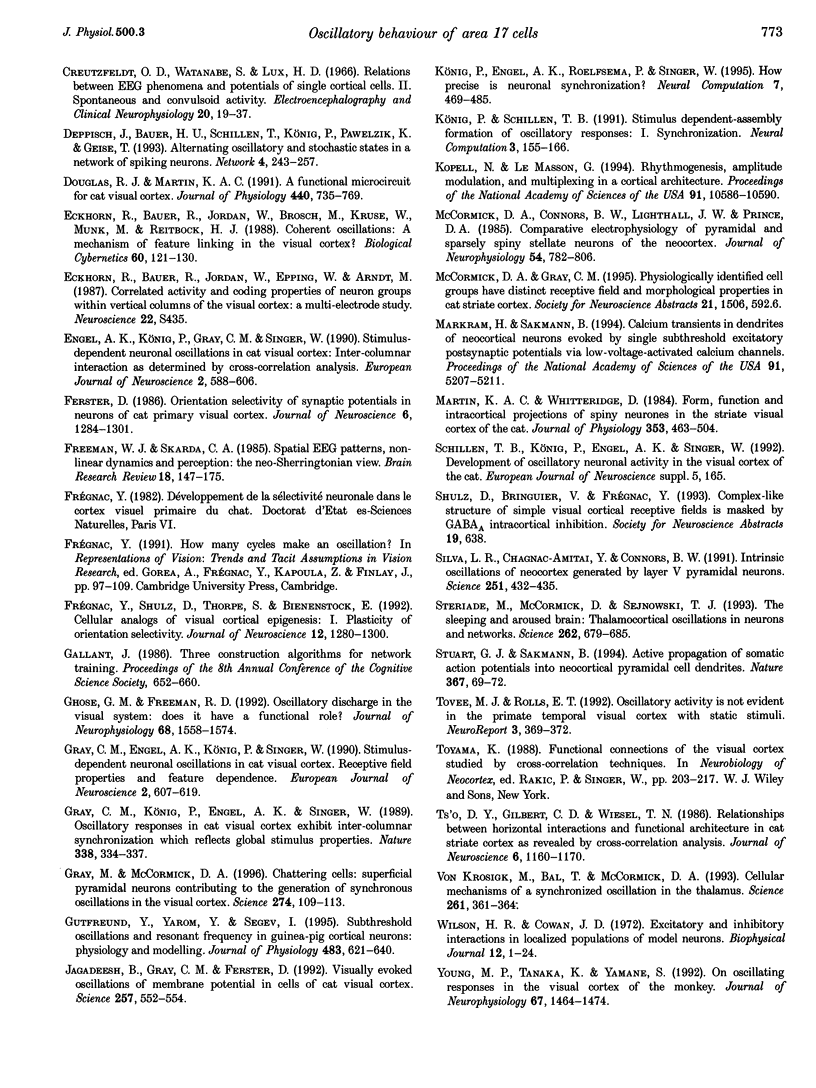
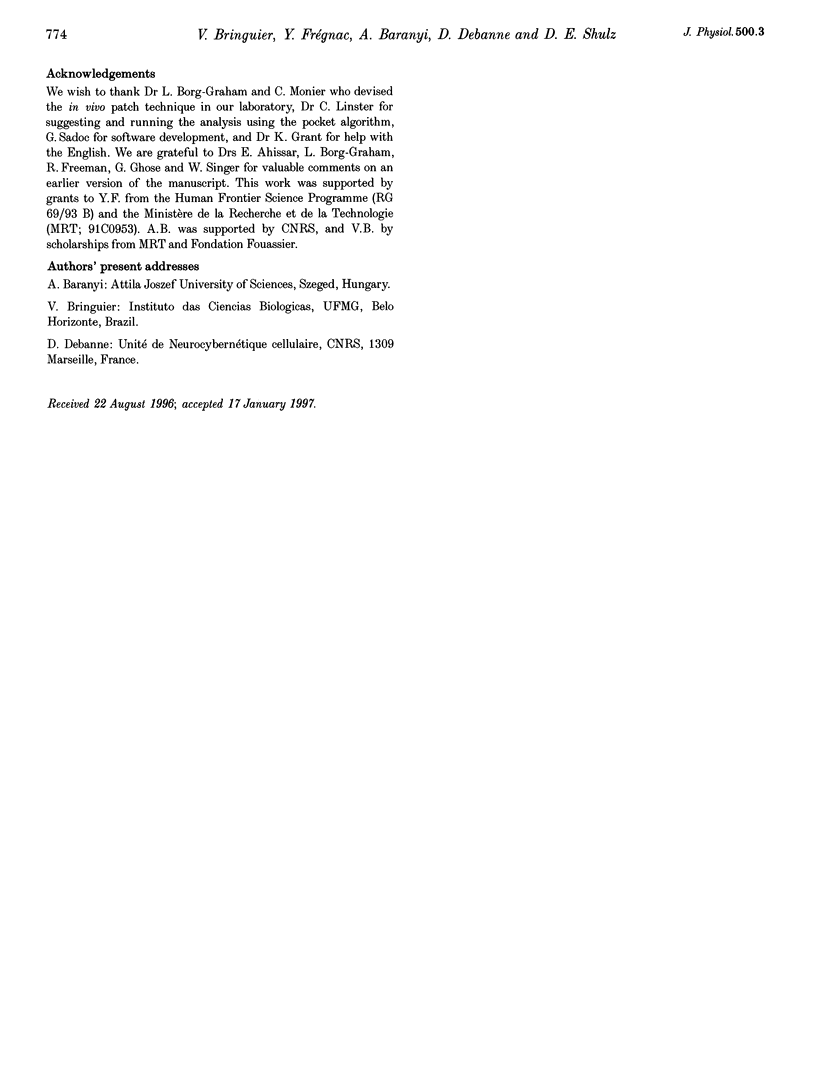
Selected References
These references are in PubMed. This may not be the complete list of references from this article.
- Alonso A., Klink R. Differential electroresponsiveness of stellate and pyramidal-like cells of medial entorhinal cortex layer II. J Neurophysiol. 1993 Jul;70(1):128–143. doi: 10.1152/jn.1993.70.1.128. [DOI] [PubMed] [Google Scholar]
- Baranyi A., Szente M. B., Woody C. D. Electrophysiological characterization of different types of neurons recorded in vivo in the motor cortex of the cat. I. Patterns of firing activity and synaptic responses. J Neurophysiol. 1993 Jun;69(6):1850–1864. doi: 10.1152/jn.1993.69.6.1850. [DOI] [PubMed] [Google Scholar]
- Bringuier V., Fregnac Y., Debanne D., Shulz D., Baranyi A. Synaptic origin of rhythmic visually evoked activity in kitten area 17 neurones. Neuroreport. 1992 Dec;3(12):1065–1068. doi: 10.1097/00001756-199212000-00008. [DOI] [PubMed] [Google Scholar]
- Buzsáki G., Horváth Z., Urioste R., Hetke J., Wise K. High-frequency network oscillation in the hippocampus. Science. 1992 May 15;256(5059):1025–1027. doi: 10.1126/science.1589772. [DOI] [PubMed] [Google Scholar]
- Creutzfeldt O. D., Watanabe S., Lux H. D. Relations between EEG phenomena and potentials of single cortical cells. II. Spontaneous and convulsoid activity. Electroencephalogr Clin Neurophysiol. 1966 Jan;20(1):19–37. doi: 10.1016/0013-4694(66)90137-4. [DOI] [PubMed] [Google Scholar]
- Douglas R. J., Martin K. A. A functional microcircuit for cat visual cortex. J Physiol. 1991;440:735–769. doi: 10.1113/jphysiol.1991.sp018733. [DOI] [PMC free article] [PubMed] [Google Scholar]
- Eckhorn R., Bauer R., Jordan W., Brosch M., Kruse W., Munk M., Reitboeck H. J. Coherent oscillations: a mechanism of feature linking in the visual cortex? Multiple electrode and correlation analyses in the cat. Biol Cybern. 1988;60(2):121–130. doi: 10.1007/BF00202899. [DOI] [PubMed] [Google Scholar]
- Engel Andreas K., König Peter, Gray Charles M., Singer Wolf. Stimulus-Dependent Neuronal Oscillations in Cat Visual Cortex: Inter-Columnar Interaction as Determined by Cross-Correlation Analysis. Eur J Neurosci. 1990;2(7):588–606. doi: 10.1111/j.1460-9568.1990.tb00449.x. [DOI] [PubMed] [Google Scholar]
- Ferster D. Orientation selectivity of synaptic potentials in neurons of cat primary visual cortex. J Neurosci. 1986 May;6(5):1284–1301. doi: 10.1523/JNEUROSCI.06-05-01284.1986. [DOI] [PMC free article] [PubMed] [Google Scholar]
- Freeman W. J., Skarda C. A. Spatial EEG patterns, non-linear dynamics and perception: the neo-Sherringtonian view. Brain Res. 1985 Dec;357(3):147–175. doi: 10.1016/0165-0173(85)90022-0. [DOI] [PubMed] [Google Scholar]
- Frégnac Y., Shulz D., Thorpe S., Bienenstock E. Cellular analogs of visual cortical epigenesis. I. Plasticity of orientation selectivity. J Neurosci. 1992 Apr;12(4):1280–1300. doi: 10.1523/JNEUROSCI.12-04-01280.1992. [DOI] [PMC free article] [PubMed] [Google Scholar]
- Ghose G. M., Freeman R. D. Oscillatory discharge in the visual system: does it have a functional role? J Neurophysiol. 1992 Nov;68(5):1558–1574. doi: 10.1152/jn.1992.68.5.1558. [DOI] [PubMed] [Google Scholar]
- Gray C. M., König P., Engel A. K., Singer W. Oscillatory responses in cat visual cortex exhibit inter-columnar synchronization which reflects global stimulus properties. Nature. 1989 Mar 23;338(6213):334–337. doi: 10.1038/338334a0. [DOI] [PubMed] [Google Scholar]
- Gray C. M., McCormick D. A. Chattering cells: superficial pyramidal neurons contributing to the generation of synchronous oscillations in the visual cortex. Science. 1996 Oct 4;274(5284):109–113. doi: 10.1126/science.274.5284.109. [DOI] [PubMed] [Google Scholar]
- Gray Charles M., Engel Andreas K., König Peter, Singer Wolf. Stimulus-Dependent Neuronal Oscillations in Cat Visual Cortex: Receptive Field Properties and Feature Dependence. Eur J Neurosci. 1990;2(7):607–619. doi: 10.1111/j.1460-9568.1990.tb00450.x. [DOI] [PubMed] [Google Scholar]
- Gutfreund Y., yarom Y., Segev I. Subthreshold oscillations and resonant frequency in guinea-pig cortical neurons: physiology and modelling. J Physiol. 1995 Mar 15;483(Pt 3):621–640. doi: 10.1113/jphysiol.1995.sp020611. [DOI] [PMC free article] [PubMed] [Google Scholar]
- Jagadeesh B., Gray C. M., Ferster D. Visually evoked oscillations of membrane potential in cells of cat visual cortex. Science. 1992 Jul 24;257(5069):552–554. doi: 10.1126/science.1636094. [DOI] [PubMed] [Google Scholar]
- Kopell N., LeMasson G. Rhythmogenesis, amplitude modulation, and multiplexing in a cortical architecture. Proc Natl Acad Sci U S A. 1994 Oct 25;91(22):10586–10590. doi: 10.1073/pnas.91.22.10586. [DOI] [PMC free article] [PubMed] [Google Scholar]
- König P., Engel A. K., Roelfsema P. R., Singer W. How precise is neuronal synchronization? Neural Comput. 1995 May;7(3):469–485. doi: 10.1162/neco.1995.7.3.469. [DOI] [PubMed] [Google Scholar]
- Markram H., Sakmann B. Calcium transients in dendrites of neocortical neurons evoked by single subthreshold excitatory postsynaptic potentials via low-voltage-activated calcium channels. Proc Natl Acad Sci U S A. 1994 May 24;91(11):5207–5211. doi: 10.1073/pnas.91.11.5207. [DOI] [PMC free article] [PubMed] [Google Scholar]
- Martin K. A., Whitteridge D. Form, function and intracortical projections of spiny neurones in the striate visual cortex of the cat. J Physiol. 1984 Aug;353:463–504. doi: 10.1113/jphysiol.1984.sp015347. [DOI] [PMC free article] [PubMed] [Google Scholar]
- McCormick D. A., Connors B. W., Lighthall J. W., Prince D. A. Comparative electrophysiology of pyramidal and sparsely spiny stellate neurons of the neocortex. J Neurophysiol. 1985 Oct;54(4):782–806. doi: 10.1152/jn.1985.54.4.782. [DOI] [PubMed] [Google Scholar]
- Silva L. R., Amitai Y., Connors B. W. Intrinsic oscillations of neocortex generated by layer 5 pyramidal neurons. Science. 1991 Jan 25;251(4992):432–435. doi: 10.1126/science.1824881. [DOI] [PubMed] [Google Scholar]
- Steriade M., McCormick D. A., Sejnowski T. J. Thalamocortical oscillations in the sleeping and aroused brain. Science. 1993 Oct 29;262(5134):679–685. doi: 10.1126/science.8235588. [DOI] [PubMed] [Google Scholar]
- Stuart G. J., Sakmann B. Active propagation of somatic action potentials into neocortical pyramidal cell dendrites. Nature. 1994 Jan 6;367(6458):69–72. doi: 10.1038/367069a0. [DOI] [PubMed] [Google Scholar]
- Tovee M. J., Rolls E. T. Oscillatory activity is not evident in the primate temporal visual cortex with static stimuli. Neuroreport. 1992 Apr;3(4):369–372. doi: 10.1097/00001756-199204000-00020. [DOI] [PubMed] [Google Scholar]
- Ts'o D. Y., Gilbert C. D., Wiesel T. N. Relationships between horizontal interactions and functional architecture in cat striate cortex as revealed by cross-correlation analysis. J Neurosci. 1986 Apr;6(4):1160–1170. doi: 10.1523/JNEUROSCI.06-04-01160.1986. [DOI] [PMC free article] [PubMed] [Google Scholar]
- Wilson H. R., Cowan J. D. Excitatory and inhibitory interactions in localized populations of model neurons. Biophys J. 1972 Jan;12(1):1–24. doi: 10.1016/S0006-3495(72)86068-5. [DOI] [PMC free article] [PubMed] [Google Scholar]
- Young M. P., Tanaka K., Yamane S. On oscillating neuronal responses in the visual cortex of the monkey. J Neurophysiol. 1992 Jun;67(6):1464–1474. doi: 10.1152/jn.1992.67.6.1464. [DOI] [PubMed] [Google Scholar]
- von Krosigk M., Bal T., McCormick D. A. Cellular mechanisms of a synchronized oscillation in the thalamus. Science. 1993 Jul 16;261(5119):361–364. doi: 10.1126/science.8392750. [DOI] [PubMed] [Google Scholar]