Abstract
1. Cardiac sarcomere stiffness was investigated during diastole in eighteen trabeculae dissected from the right ventricle of rat heart. The trabeculae were stimulated at 0.5 Hz, in a modified Krebs-Henseleit solution (pH, 7.4; 25 degrees C). Sarcomere length (SL) was measured using high resolution (+/-2 nm) laser diffraction techniques. Force (F) was measured with a silicon strain gauge. 2. SL increased exponentially (amplitude, 25 +/- 9 nm; n = 15) throughout diastole. This increase occurred even at slack SL, showing that this phenomenon was due to an internal expansion. The majority of the muscles showed discrete spontaneous fluctuations of SL (amplitude < 20 nm) starting approximately 1 s after the end of the twitch. 3. The intracellular free Ca2+ concentration ([Ca2+]i) was measured from the fluorescence of microinjected fura-2 salt in seven trabeculae under the same experimental conditions. [Ca2+]i continuously declined (from 240 to 90 nM) during diastole following a monoexponential time course (time constant, 210-325 ms). 4. The stiffness of the sarcomere was evaluated at 10, 30, 50, 70 and 90% of diastole using bursts (30 ms) of 500 Hz sinusoidal perturbations of muscle length (amplitude of SL oscillations < 30 nm). At 1 nM external Ca2+ concentration ([Ca2+]o), the average stiffness modulus (Mod) increased from 9.3 +/- 0.6 to 12 +/- 0.6 nN mm-2 micron-1 (n = 18; P < 0.05), while the average phase shift (phi) between F and SL signals decreased from 84 +/- 3 to 73 +/- 4 deg (n = 18; P < 0.05) between 10 and 90% during diastole. The increase in Mod and the decrease in phi reversed when spontaneous activity occurred. When [Ca2+]o was raised to 2 mM, the stiffness time course reversed approximately 450 ms earlier, simultaneously with the occurrence of spontaneous activity. 5. Our results show that diastole is only an apparent steady state and suggest that the structural system responsible for the viscoelastic properties of the sarcomere is regulated by [Ca2+]i in the submicromolar range. Different possible origins of the dynamic changes in viscoelasticity during diastole are discussed.
Full text
PDF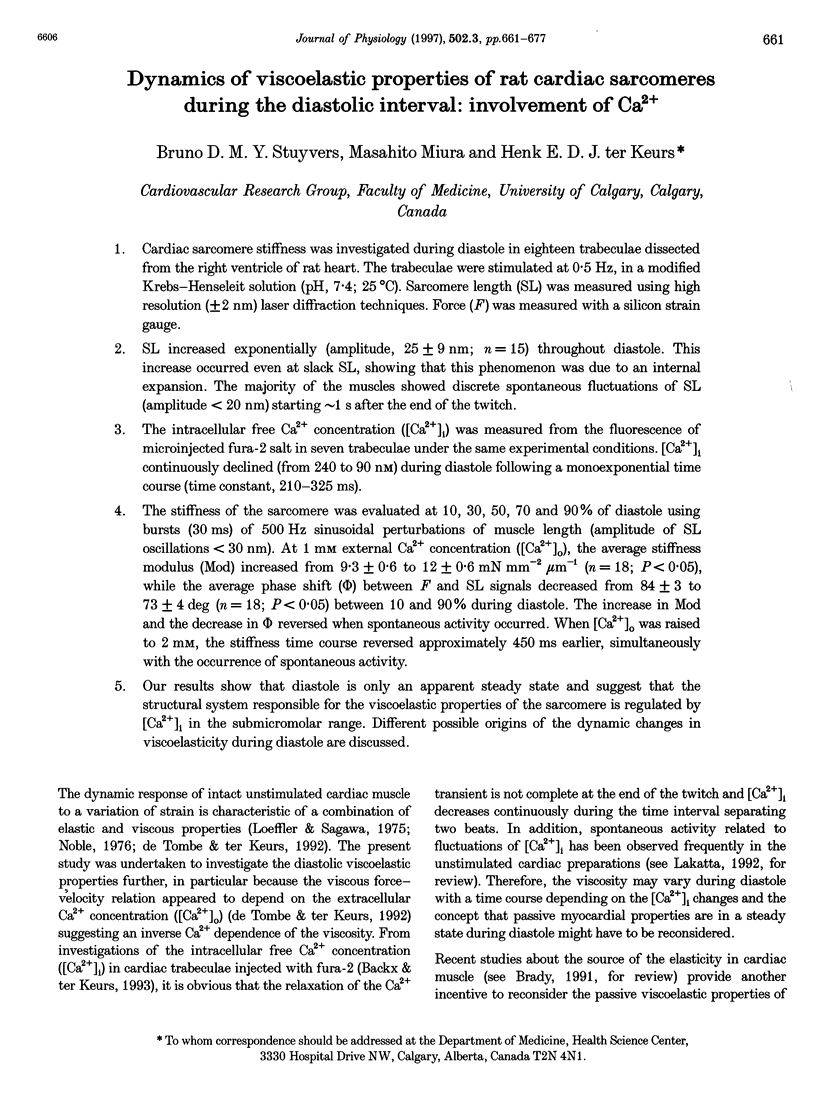
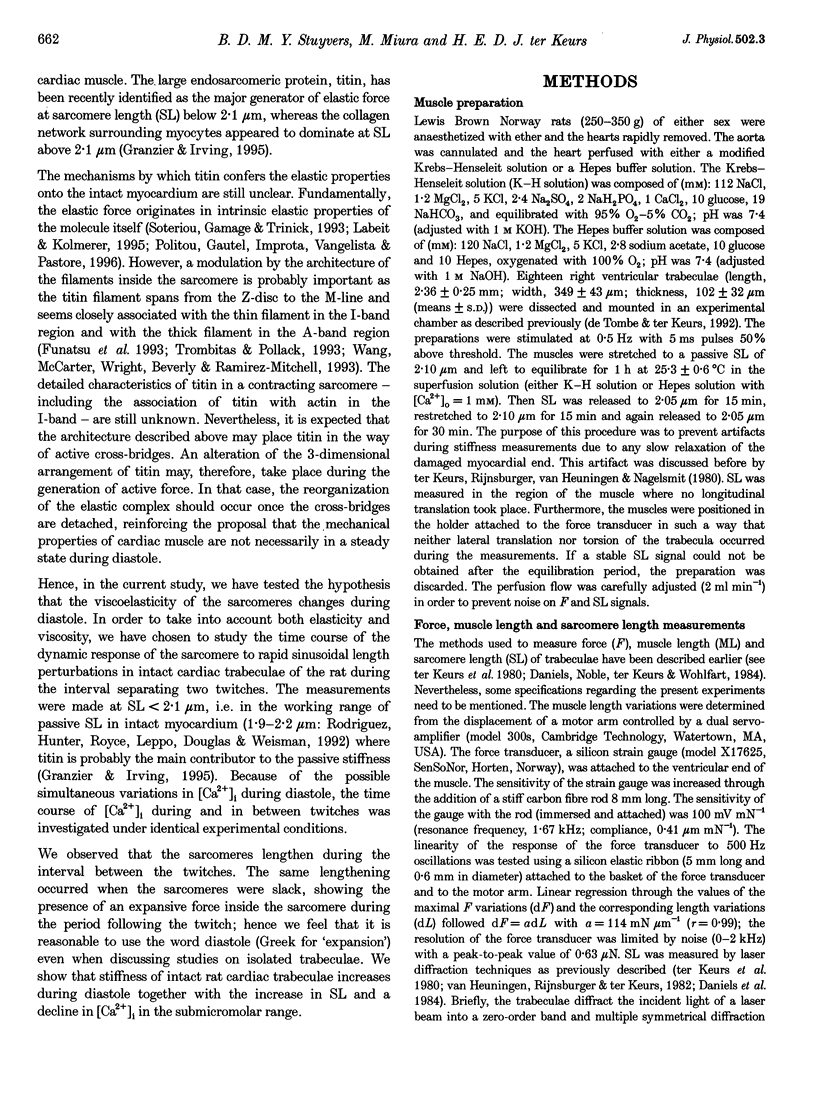
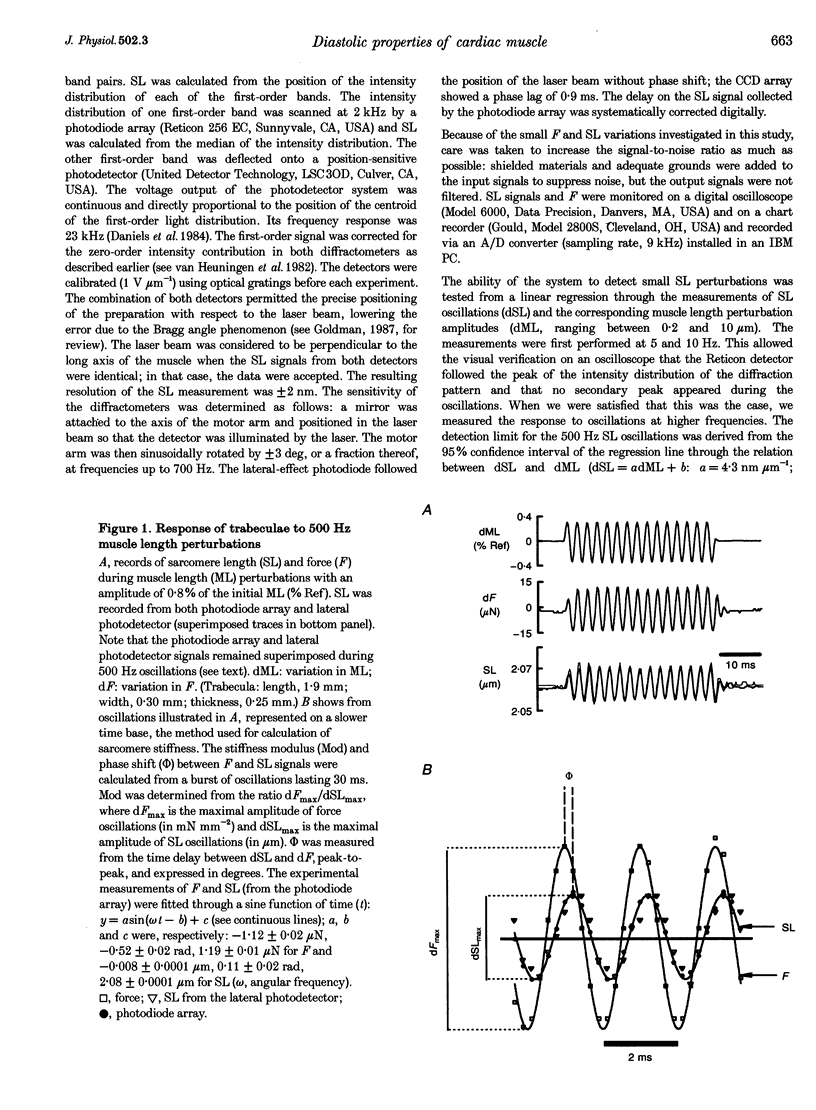
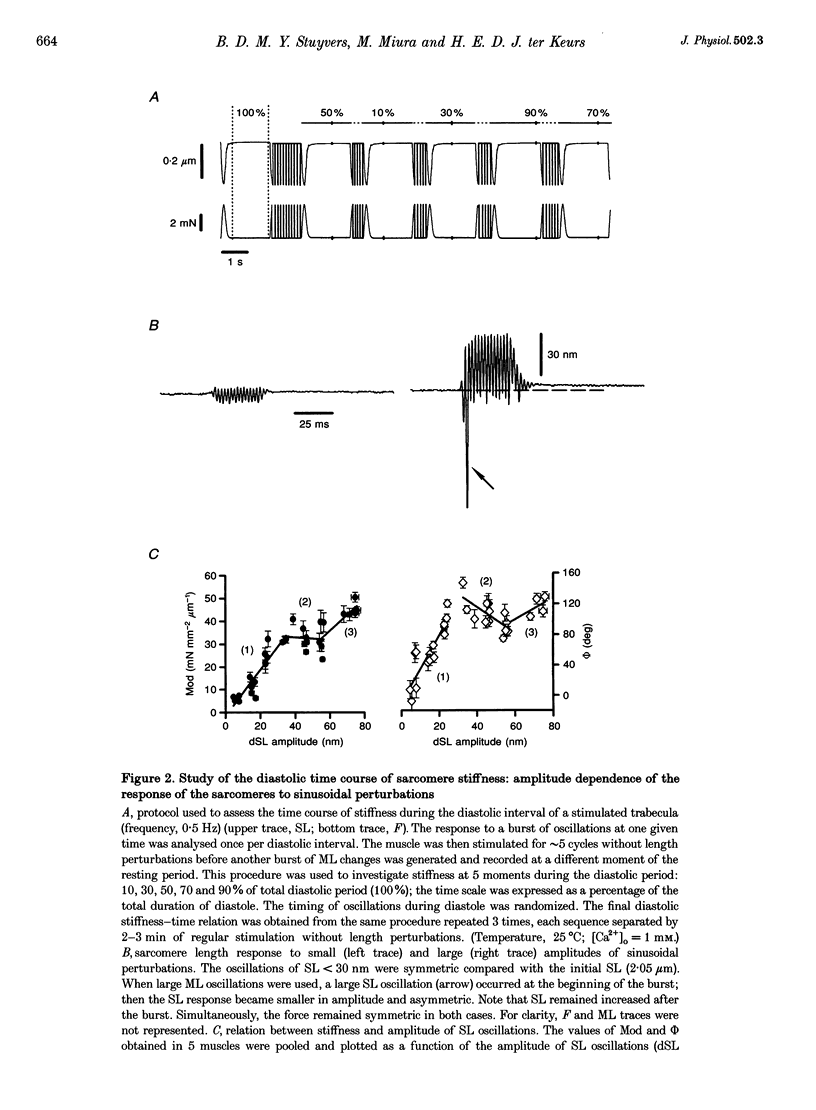
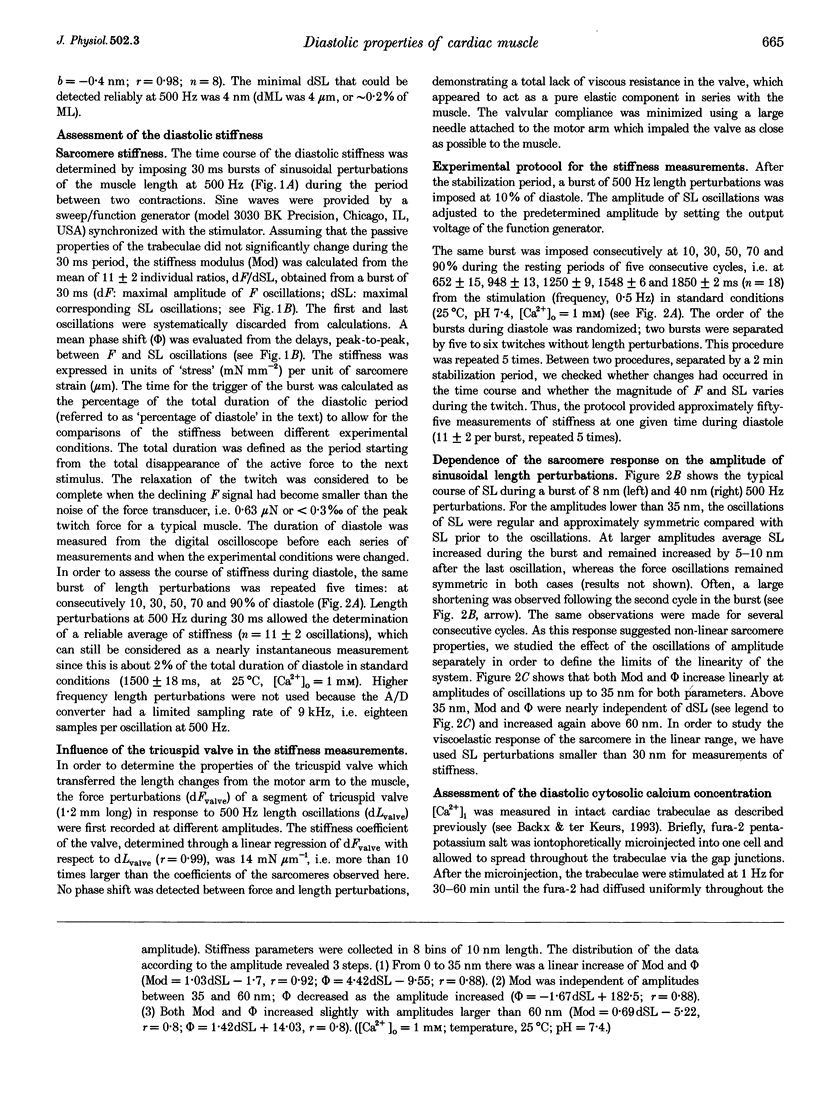
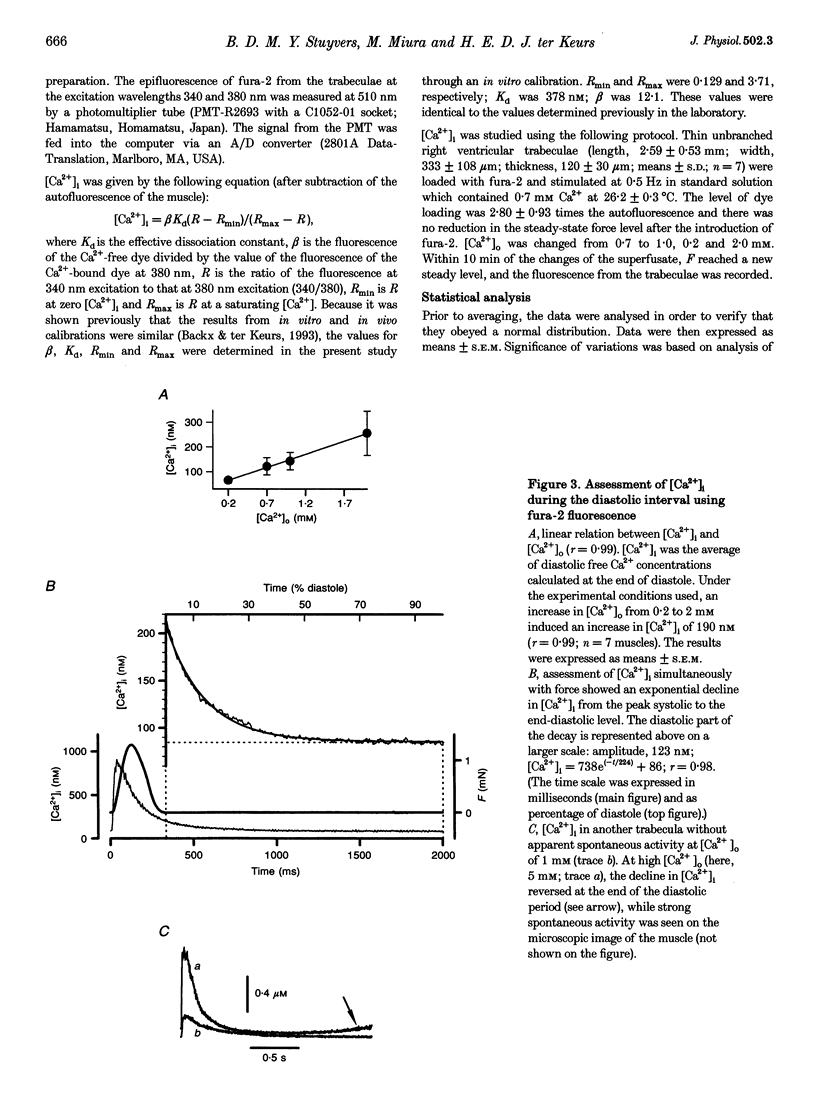
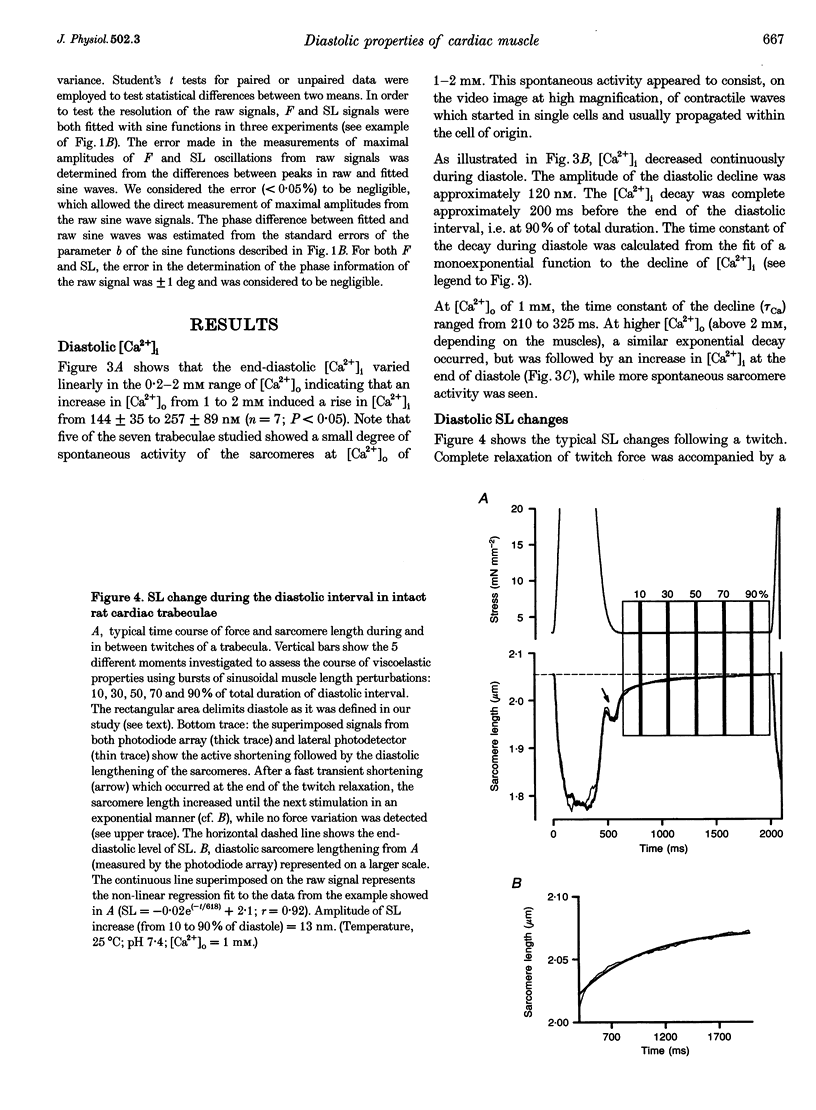
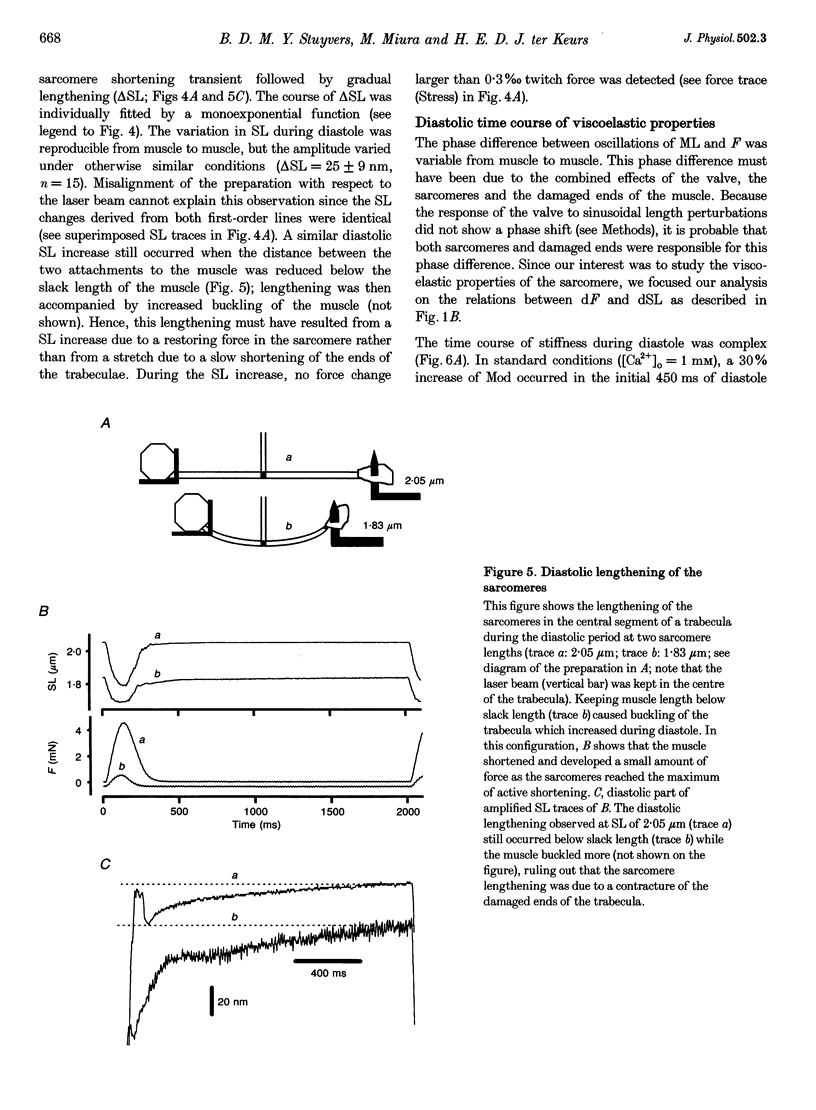
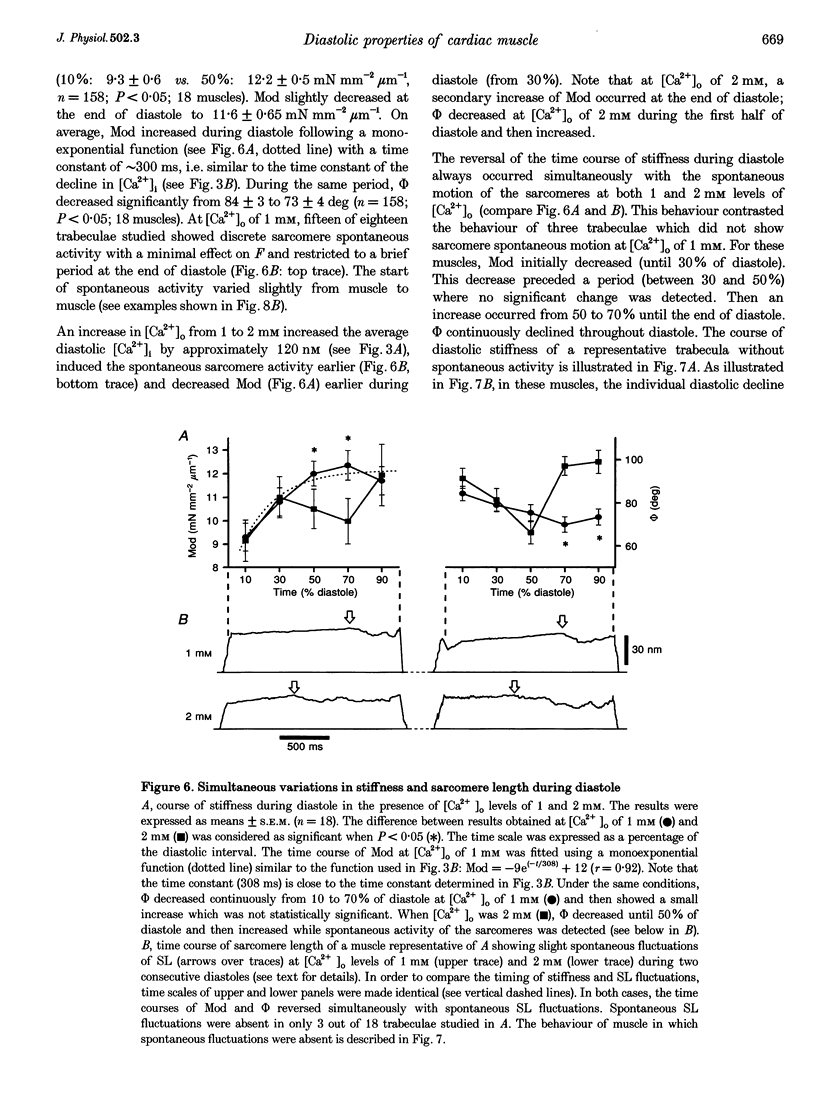
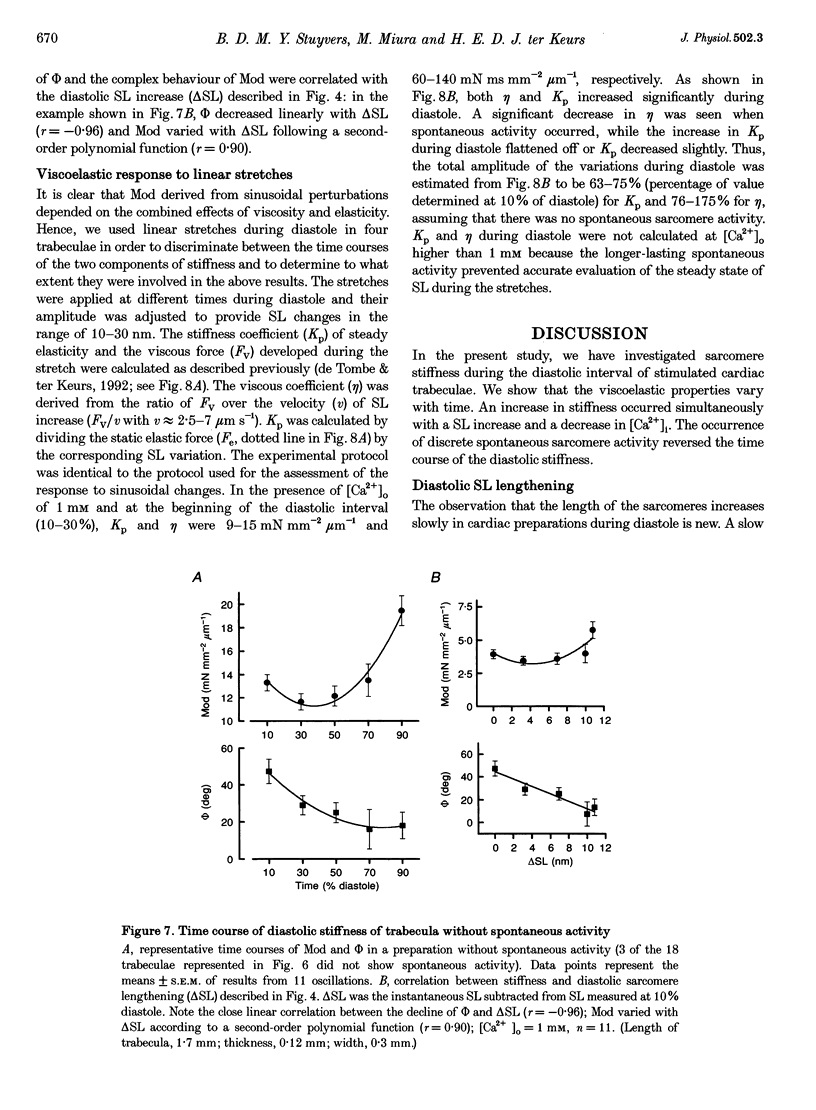
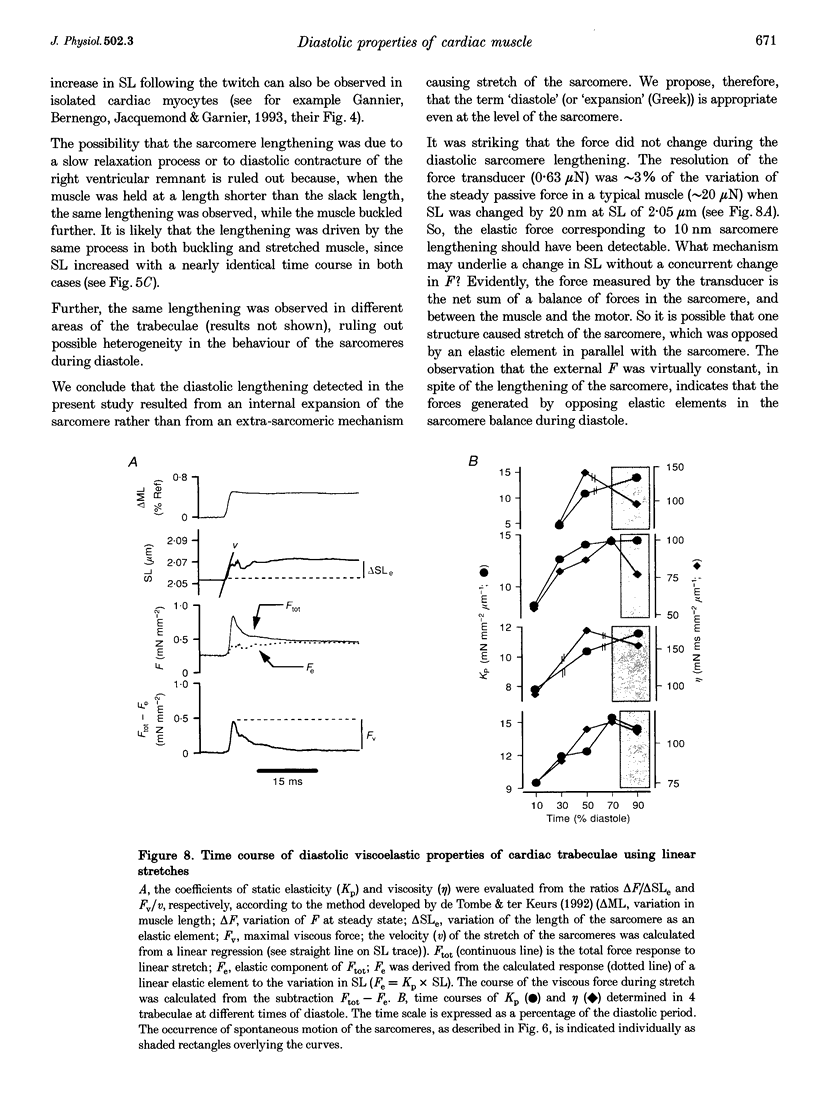
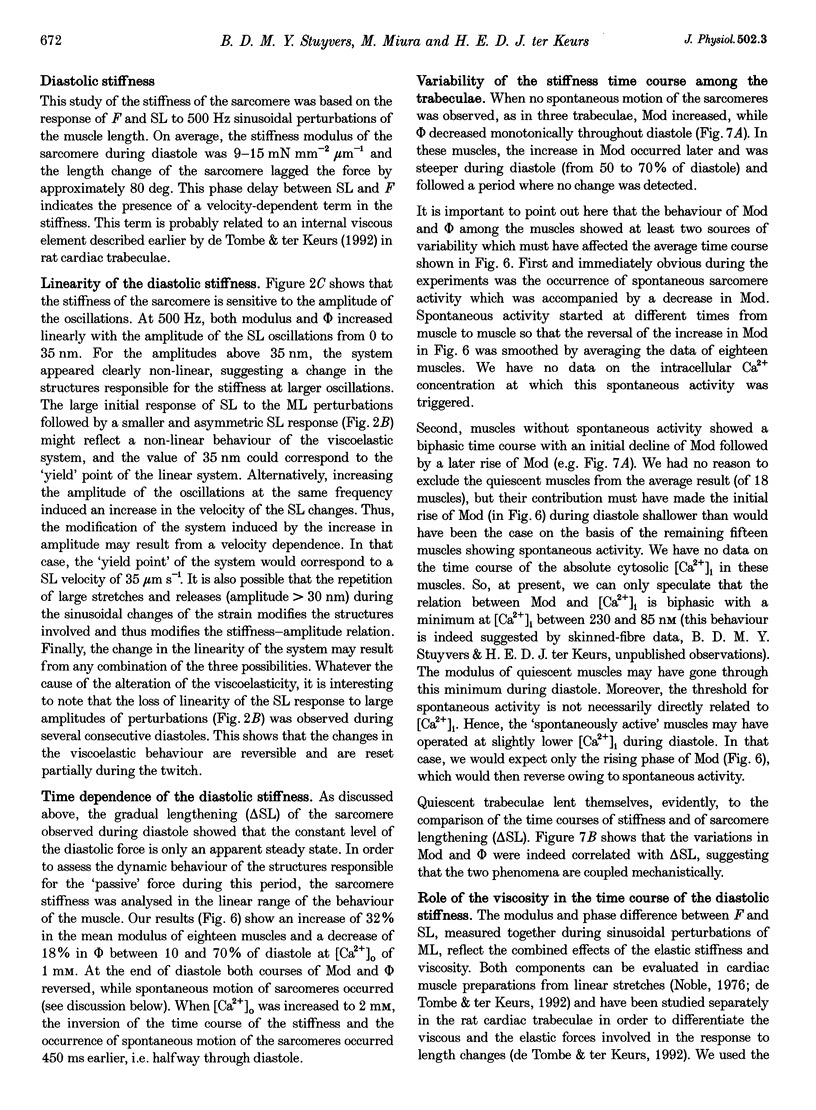
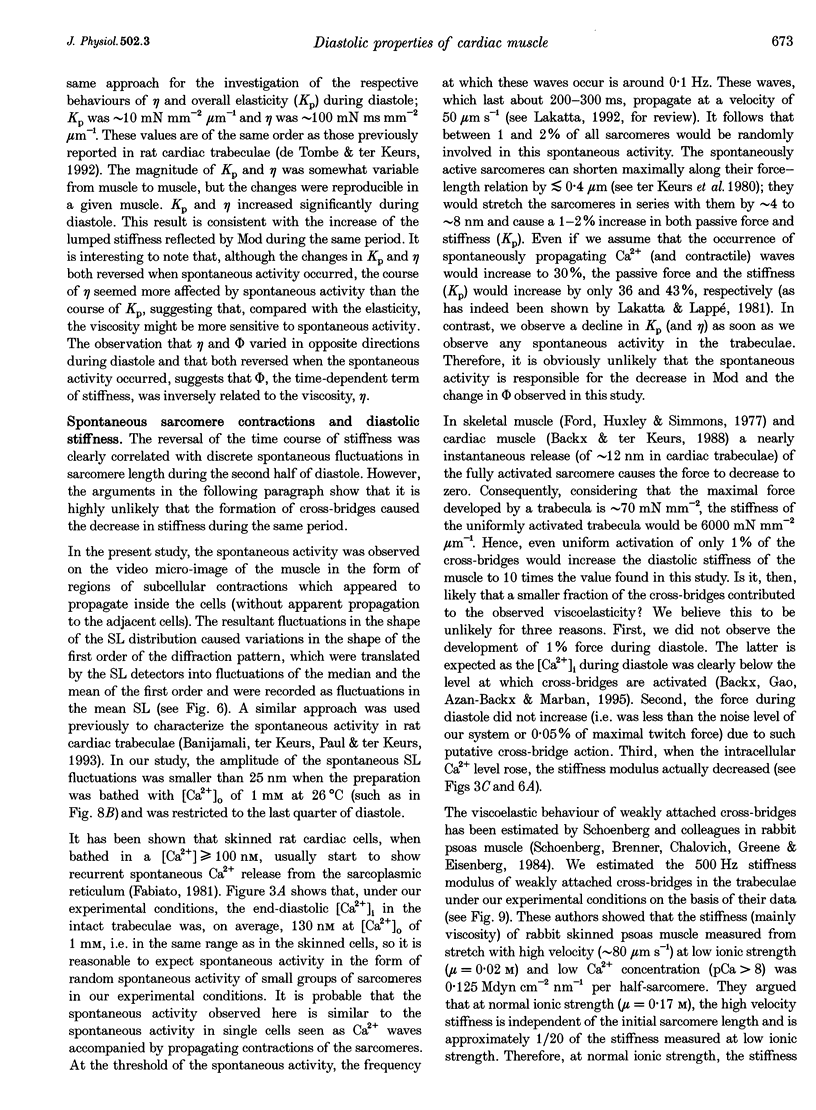
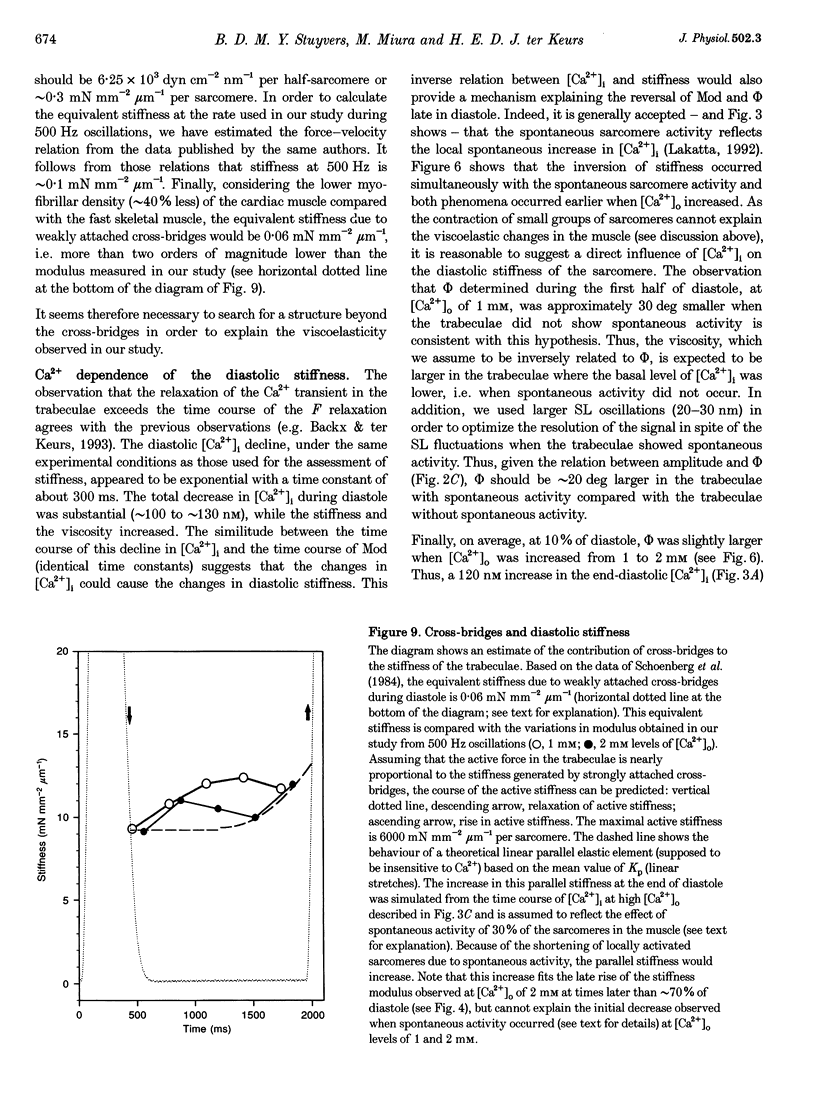
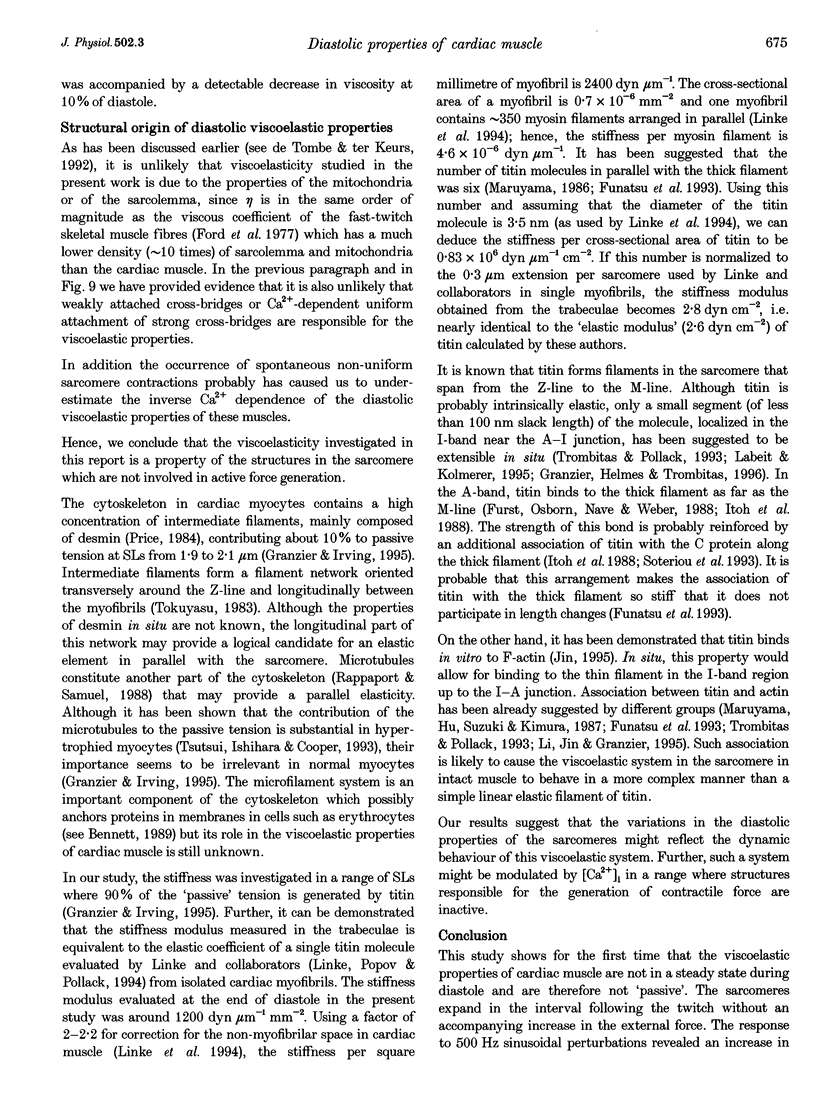
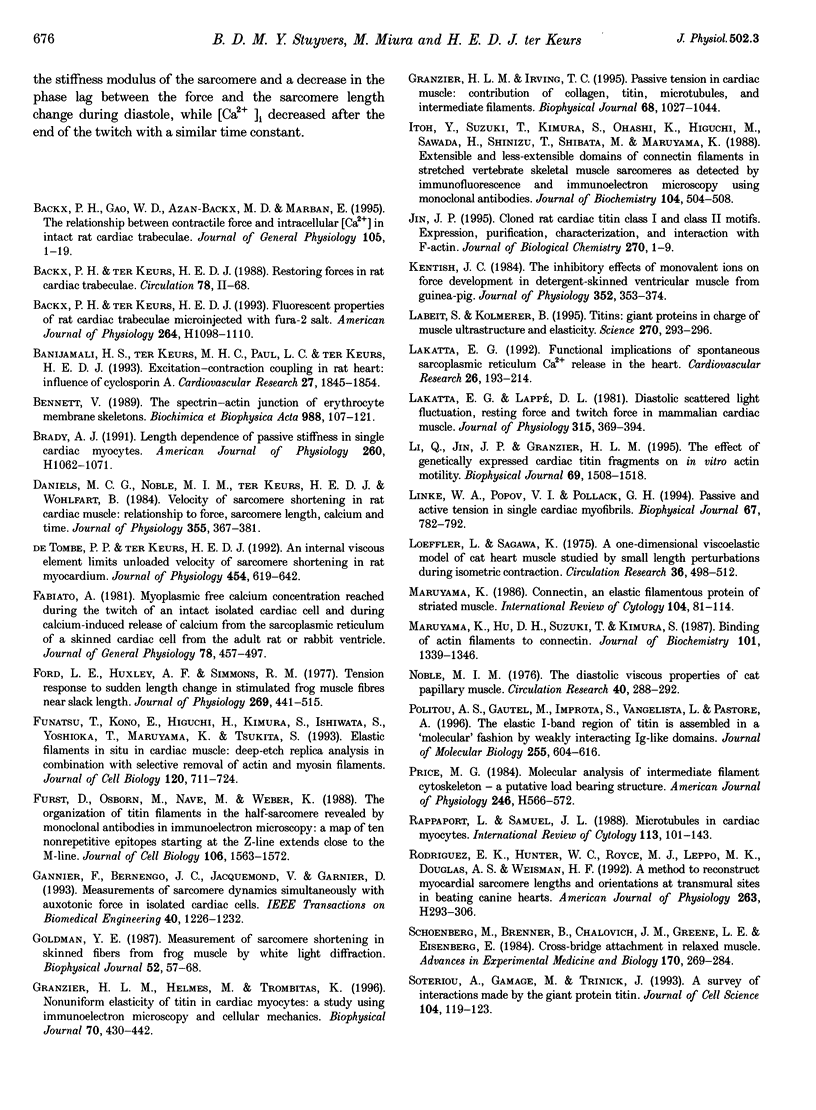
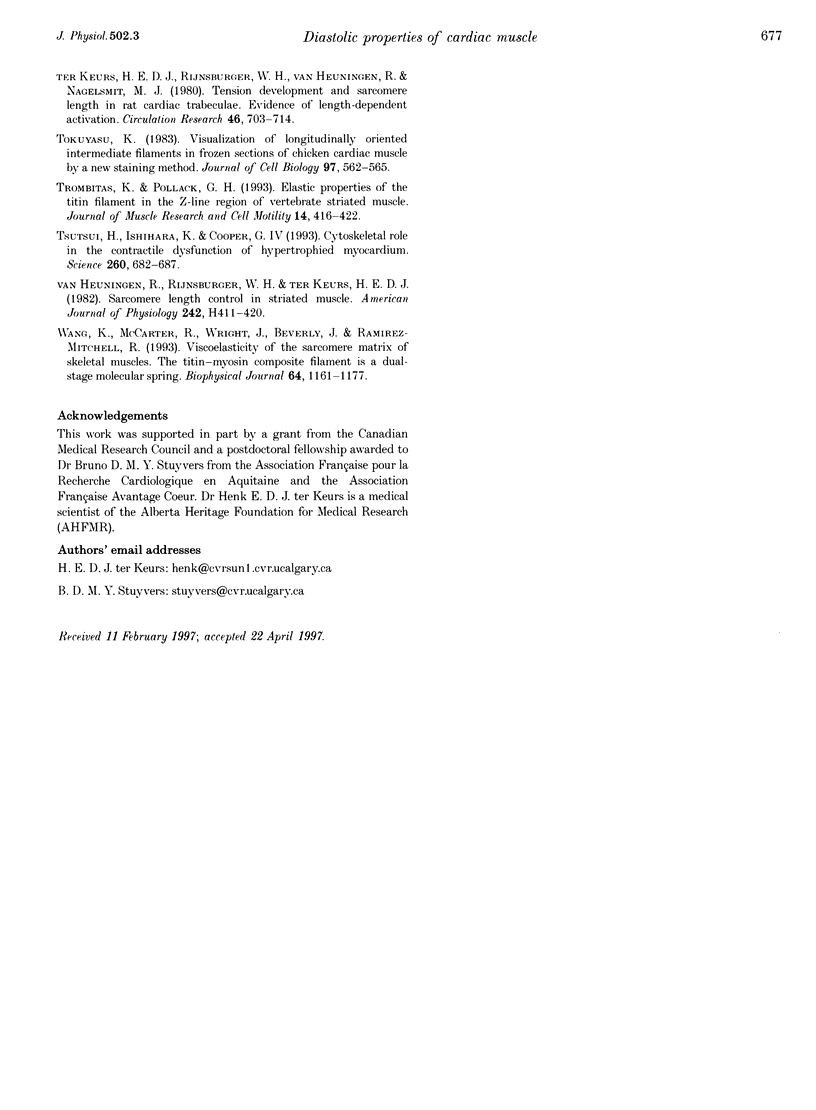
Images in this article
Selected References
These references are in PubMed. This may not be the complete list of references from this article.
- Backx P. H., Gao W. D., Azan-Backx M. D., Marban E. The relationship between contractile force and intracellular [Ca2+] in intact rat cardiac trabeculae. J Gen Physiol. 1995 Jan;105(1):1–19. doi: 10.1085/jgp.105.1.1. [DOI] [PMC free article] [PubMed] [Google Scholar]
- Backx P. H., Ter Keurs H. E. Fluorescent properties of rat cardiac trabeculae microinjected with fura-2 salt. Am J Physiol. 1993 Apr;264(4 Pt 2):H1098–H1110. doi: 10.1152/ajpheart.1993.264.4.H1098. [DOI] [PubMed] [Google Scholar]
- Banijamali H. S., ter Keurs M. H., Paul L. C., ter Keurs H. E. Excitation-contraction coupling in rat heart: influence of cyclosporin A. Cardiovasc Res. 1993 Oct;27(10):1845–1854. doi: 10.1093/cvr/27.10.1845. [DOI] [PubMed] [Google Scholar]
- Bennett V. The spectrin-actin junction of erythrocyte membrane skeletons. Biochim Biophys Acta. 1989 Jan 18;988(1):107–121. doi: 10.1016/0304-4157(89)90006-3. [DOI] [PubMed] [Google Scholar]
- Brady A. J. Length dependence of passive stiffness in single cardiac myocytes. Am J Physiol. 1991 Apr;260(4 Pt 2):H1062–H1071. doi: 10.1152/ajpheart.1991.260.4.H1062. [DOI] [PubMed] [Google Scholar]
- Daniels M., Noble M. I., ter Keurs H. E., Wohlfart B. Velocity of sarcomere shortening in rat cardiac muscle: relationship to force, sarcomere length, calcium and time. J Physiol. 1984 Oct;355:367–381. doi: 10.1113/jphysiol.1984.sp015424. [DOI] [PMC free article] [PubMed] [Google Scholar]
- Fabiato A. Myoplasmic free calcium concentration reached during the twitch of an intact isolated cardiac cell and during calcium-induced release of calcium from the sarcoplasmic reticulum of a skinned cardiac cell from the adult rat or rabbit ventricle. J Gen Physiol. 1981 Nov;78(5):457–497. doi: 10.1085/jgp.78.5.457. [DOI] [PMC free article] [PubMed] [Google Scholar]
- Ford L. E., Huxley A. F., Simmons R. M. Tension responses to sudden length change in stimulated frog muscle fibres near slack length. J Physiol. 1977 Jul;269(2):441–515. doi: 10.1113/jphysiol.1977.sp011911. [DOI] [PMC free article] [PubMed] [Google Scholar]
- Funatsu T., Kono E., Higuchi H., Kimura S., Ishiwata S., Yoshioka T., Maruyama K., Tsukita S. Elastic filaments in situ in cardiac muscle: deep-etch replica analysis in combination with selective removal of actin and myosin filaments. J Cell Biol. 1993 Feb;120(3):711–724. doi: 10.1083/jcb.120.3.711. [DOI] [PMC free article] [PubMed] [Google Scholar]
- Fürst D. O., Osborn M., Nave R., Weber K. The organization of titin filaments in the half-sarcomere revealed by monoclonal antibodies in immunoelectron microscopy: a map of ten nonrepetitive epitopes starting at the Z line extends close to the M line. J Cell Biol. 1988 May;106(5):1563–1572. doi: 10.1083/jcb.106.5.1563. [DOI] [PMC free article] [PubMed] [Google Scholar]
- Gannier F., Bernengo J. C., Jacquemond V., Garnier D. Measurements of sarcomere dynamics simultaneously with auxotonic force in isolated cardiac cells. IEEE Trans Biomed Eng. 1993 Dec;40(12):1226–1232. doi: 10.1109/10.250578. [DOI] [PubMed] [Google Scholar]
- Goldman Y. E. Measurement of sarcomere shortening in skinned fibers from frog muscle by white light diffraction. Biophys J. 1987 Jul;52(1):57–68. doi: 10.1016/S0006-3495(87)83188-0. [DOI] [PMC free article] [PubMed] [Google Scholar]
- Granzier H. L., Irving T. C. Passive tension in cardiac muscle: contribution of collagen, titin, microtubules, and intermediate filaments. Biophys J. 1995 Mar;68(3):1027–1044. doi: 10.1016/S0006-3495(95)80278-X. [DOI] [PMC free article] [PubMed] [Google Scholar]
- Granzier H., Helmes M., Trombitás K. Nonuniform elasticity of titin in cardiac myocytes: a study using immunoelectron microscopy and cellular mechanics. Biophys J. 1996 Jan;70(1):430–442. doi: 10.1016/S0006-3495(96)79586-3. [DOI] [PMC free article] [PubMed] [Google Scholar]
- Itoh Y., Suzuki T., Kimura S., Ohashi K., Higuchi H., Sawada H., Shimizu T., Shibata M., Maruyama K. Extensible and less-extensible domains of connectin filaments in stretched vertebrate skeletal muscle sarcomeres as detected by immunofluorescence and immunoelectron microscopy using monoclonal antibodies. J Biochem. 1988 Oct;104(4):504–508. doi: 10.1093/oxfordjournals.jbchem.a122499. [DOI] [PubMed] [Google Scholar]
- Kentish J. C. The inhibitory effects of monovalent ions on force development in detergent-skinned ventricular muscle from guinea-pig. J Physiol. 1984 Jul;352:353–374. doi: 10.1113/jphysiol.1984.sp015296. [DOI] [PMC free article] [PubMed] [Google Scholar]
- Labeit S., Kolmerer B. Titins: giant proteins in charge of muscle ultrastructure and elasticity. Science. 1995 Oct 13;270(5234):293–296. doi: 10.1126/science.270.5234.293. [DOI] [PubMed] [Google Scholar]
- Lakatta E. G. Functional implications of spontaneous sarcoplasmic reticulum Ca2+ release in the heart. Cardiovasc Res. 1992 Mar;26(3):193–214. doi: 10.1093/cvr/26.3.193. [DOI] [PubMed] [Google Scholar]
- Lakatta E. G., Lappé D. L. Diastolic scattered light fluctuation, resting force and twitch force in mammalian cardiac muscle. J Physiol. 1981 Jun;315:369–394. doi: 10.1113/jphysiol.1981.sp013753. [DOI] [PMC free article] [PubMed] [Google Scholar]
- Li Q., Jin J. P., Granzier H. L. The effect of genetically expressed cardiac titin fragments on in vitro actin motility. Biophys J. 1995 Oct;69(4):1508–1518. doi: 10.1016/S0006-3495(95)80021-4. [DOI] [PMC free article] [PubMed] [Google Scholar]
- Linke W. A., Popov V. I., Pollack G. H. Passive and active tension in single cardiac myofibrils. Biophys J. 1994 Aug;67(2):782–792. doi: 10.1016/S0006-3495(94)80538-7. [DOI] [PMC free article] [PubMed] [Google Scholar]
- Loeffler L., 3rd, Sagawa K. A one-dimensional viscoelastic model of cat heart muscle studied by small length perturbations during isometric contraction. Circ Res. 1975 Apr;36(4):498–512. doi: 10.1161/01.res.36.4.498. [DOI] [PubMed] [Google Scholar]
- Maruyama K. Connectin, an elastic filamentous protein of striated muscle. Int Rev Cytol. 1986;104:81–114. doi: 10.1016/s0074-7696(08)61924-5. [DOI] [PubMed] [Google Scholar]
- Maruyama K., Hu D. H., Suzuki T., Kimura S. Binding of actin filaments to connectin. J Biochem. 1987 Jun;101(6):1339–1346. doi: 10.1093/oxfordjournals.jbchem.a122001. [DOI] [PubMed] [Google Scholar]
- Noble M. I. The diastolic viscous properties of cat papillary muscle. Circ Res. 1977 Mar;40(3):288–292. doi: 10.1161/01.res.40.3.288. [DOI] [PubMed] [Google Scholar]
- Politou A. S., Gautel M., Improta S., Vangelista L., Pastore A. The elastic I-band region of titin is assembled in a "modular" fashion by weakly interacting Ig-like domains. J Mol Biol. 1996 Feb 2;255(4):604–616. doi: 10.1006/jmbi.1996.0050. [DOI] [PubMed] [Google Scholar]
- Price M. G. Molecular analysis of intermediate filament cytoskeleton--a putative load-bearing structure. Am J Physiol. 1984 Apr;246(4 Pt 2):H566–H572. doi: 10.1152/ajpheart.1984.246.4.H566. [DOI] [PubMed] [Google Scholar]
- Rappaport L., Samuel J. L. Microtubules in cardiac myocytes. Int Rev Cytol. 1988;113:101–143. doi: 10.1016/s0074-7696(08)60847-5. [DOI] [PubMed] [Google Scholar]
- Rodriguez E. K., Hunter W. C., Royce M. J., Leppo M. K., Douglas A. S., Weisman H. F. A method to reconstruct myocardial sarcomere lengths and orientations at transmural sites in beating canine hearts. Am J Physiol. 1992 Jul;263(1 Pt 2):H293–H306. doi: 10.1152/ajpheart.1992.263.1.H293. [DOI] [PubMed] [Google Scholar]
- Schoenberg M., Brenner B., Chalovich J. M., Greene L. E., Eisenberg E. Cross-bridge attachment in relaxed muscle. Adv Exp Med Biol. 1984;170:269–284. doi: 10.1007/978-1-4684-4703-3_24. [DOI] [PubMed] [Google Scholar]
- Soteriou A., Gamage M., Trinick J. A survey of interactions made by the giant protein titin. J Cell Sci. 1993 Jan;104(Pt 1):119–123. doi: 10.1242/jcs.104.1.119. [DOI] [PubMed] [Google Scholar]
- Taussig R., Gilman A. G. Mammalian membrane-bound adenylyl cyclases. J Biol Chem. 1995 Jan 6;270(1):1–4. doi: 10.1074/jbc.270.1.1. [DOI] [PubMed] [Google Scholar]
- Tsutsui H., Ishihara K., Cooper G., 4th Cytoskeletal role in the contractile dysfunction of hypertrophied myocardium. Science. 1993 Apr 30;260(5108):682–687. doi: 10.1126/science.8097594. [DOI] [PubMed] [Google Scholar]
- Wang K., McCarter R., Wright J., Beverly J., Ramirez-Mitchell R. Viscoelasticity of the sarcomere matrix of skeletal muscles. The titin-myosin composite filament is a dual-stage molecular spring. Biophys J. 1993 Apr;64(4):1161–1177. doi: 10.1016/S0006-3495(93)81482-6. [DOI] [PMC free article] [PubMed] [Google Scholar]
- de Tombe P. P., ter Keurs H. E. An internal viscous element limits unloaded velocity of sarcomere shortening in rat myocardium. J Physiol. 1992 Aug;454:619–642. doi: 10.1113/jphysiol.1992.sp019283. [DOI] [PMC free article] [PubMed] [Google Scholar]
- ter Keurs H. E., Rijnsburger W. H., van Heuningen R., Nagelsmit M. J. Tension development and sarcomere length in rat cardiac trabeculae. Evidence of length-dependent activation. Circ Res. 1980 May;46(5):703–714. doi: 10.1161/01.res.46.5.703. [DOI] [PubMed] [Google Scholar]