Abstract
1. Total RNA isolated from embryonic chick paravertebral sympathetic ganglia was used in a reverse transcription-polymerase chain reaction (RT-PCR) assay with a pair of degenerate oligonucleotide primers deduced from conserved regions of mammalian glycine receptor alpha-subunits. Three classes of cDNA were identified which encode portions of the chicken homologues of the mammalian glycine receptor alpha 1, alpha 2 and alpha 3 subunits. 2. The presence of functional glycine receptors was investigated in the whole-cell configuration of the patch-clamp technique in neurons dissociated from the ganglia and kept in culture for 7-8 days. In cells voltage clamped to -70 mV, glycine consistently induced inward currents in a concentration-dependent manner and elicited half-maximal peak current amplitudes at 43 microM. 3. The steady-state current-voltage relation for glycine-induced currents was linear between +80 and -60 mV, but showed outward rectification at more hyperpolarized potentials. Reversal potentials of these currents shifted with changes in intracellular chloride concentrations and matched the calculated Nernst potentials for chloride. 4. beta-Alanine and taurine were significantly less potent than glycine in triggering inward currents, with half-maximal responses at 79 and 86 microM, respectively. At maximally active concentrations, beta-alanine-evoked currents were identical in amplitude to those induced by glycine. Taurine-evoked currents, in contrast, never reached the same amplitude as glycine-induced currents. 5. The classical glycine receptor antagonist strychnine reversibly reduced glycine-induced currents, with half-maximal inhibition occurring at 62 nM. Two more recently characterized glycine receptor antagonists, isonipecotic acid (half-maximal inhibition at 2 mM) and 7-trifluoromethyl-4-hydroxyquinoline-3-carboxylic acid (half-maximal inhibition at 67 microM), also blocked glycine-evoked currents in a reversible manner. The chloride channel blocker picrotoxin reduced glycine-evoked currents, with half-maximal effects at 348 microM. Inhibition by the glycine receptor channel blocker cyanotriphenylborate was half-maximal at 4 microM. 6. Apart from evoking inward currents, glycine occasionally triggered short (< 100 ms) spike-like currents which were abolished by hexamethonium and thus reflected synaptic release of endogenous acetylcholine. In addition, glycine caused Ca(2+)-dependent and tetrodotoxin-sensitive tritium overflow from neurons previously labelled with [3H]noradrenaline. This stimulatory action of glycine was reduced in the presence of strychnine and after treatment with the chloride uptake inhibitor furosemide (frusemide). 7. In 65% of neurons loaded with the Ca2+ indicator fura-2 acetoxymethyl ester, glycine increased the ratio of the fluorescence signal obtained with excitation wavelengths of 340 and 380 nm, respectively, which indicates a rise in intracellular Ca2+ concentration. 8. The results show that sympathetic neurons contain transcripts for different glycine receptor alpha-subunits and carry functional heteromeric glycine receptors which depolarize the majority of neurons to trigger transmitter release.
Full text
PDF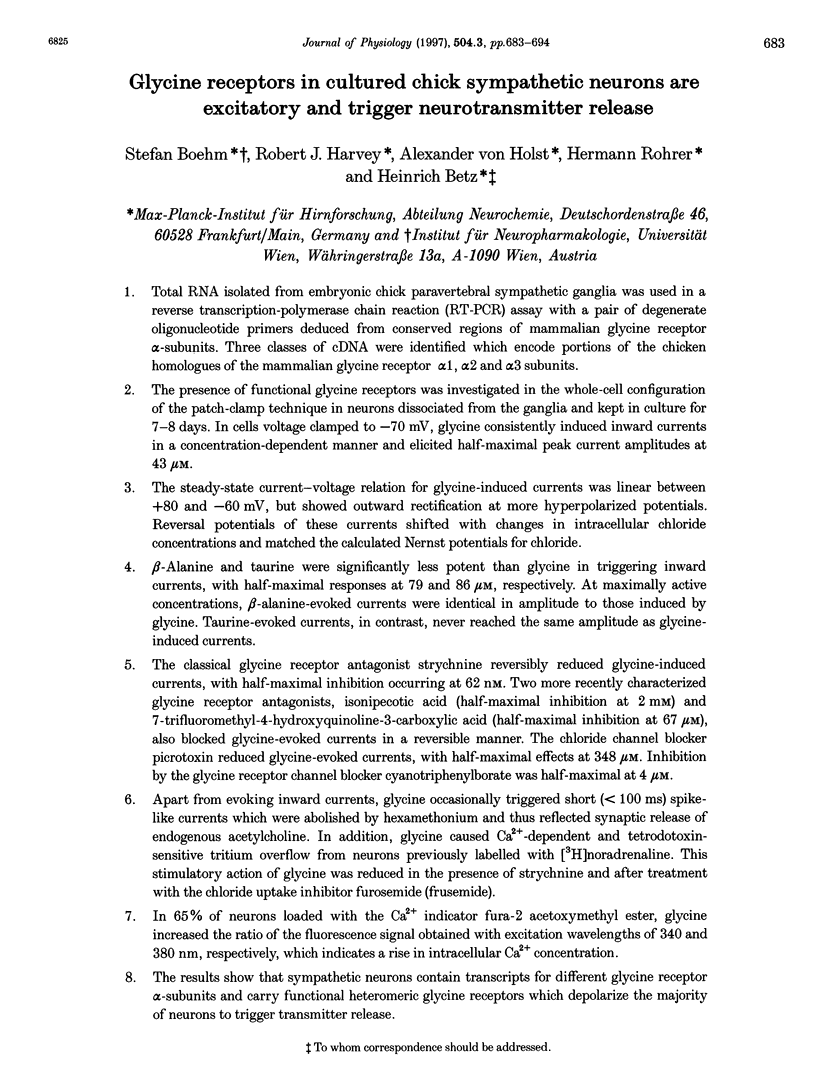
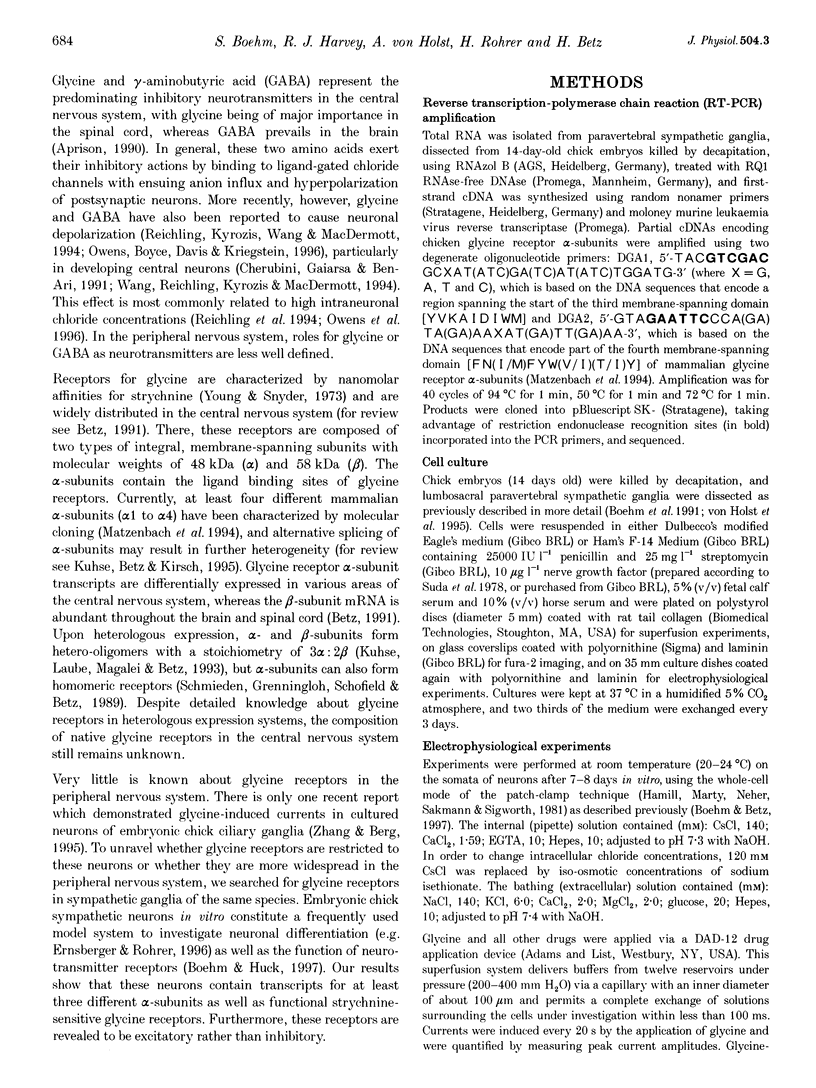
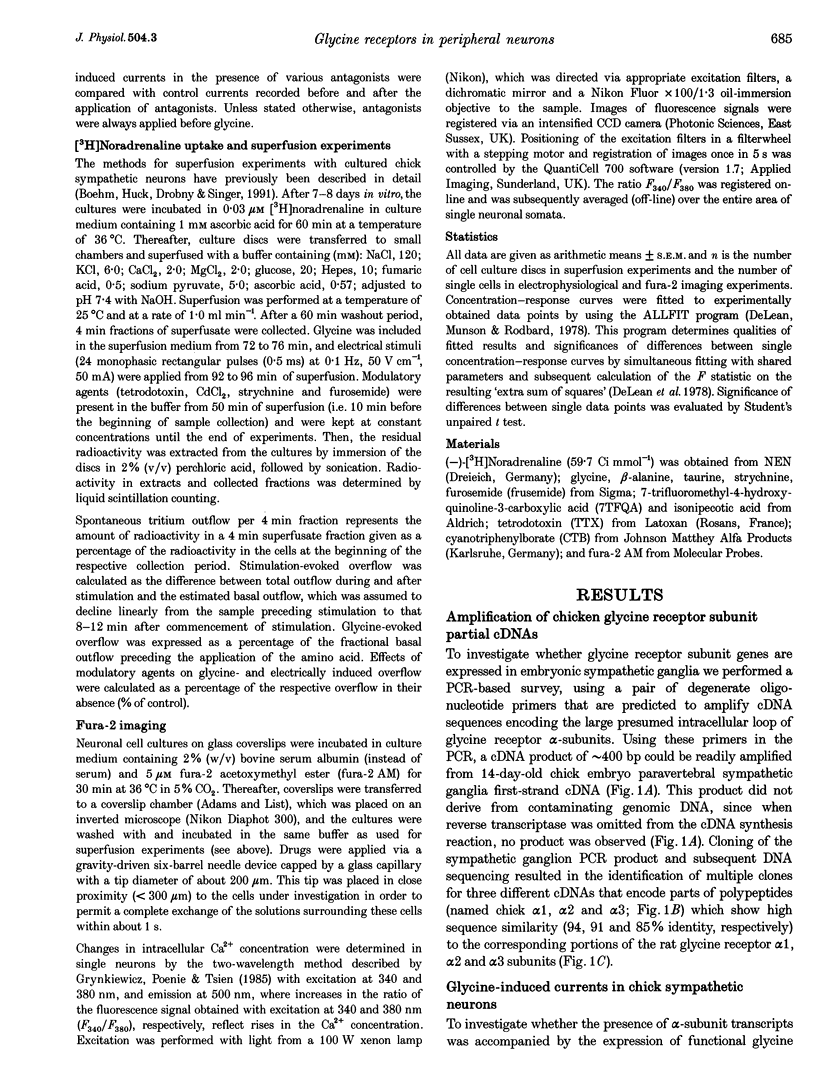
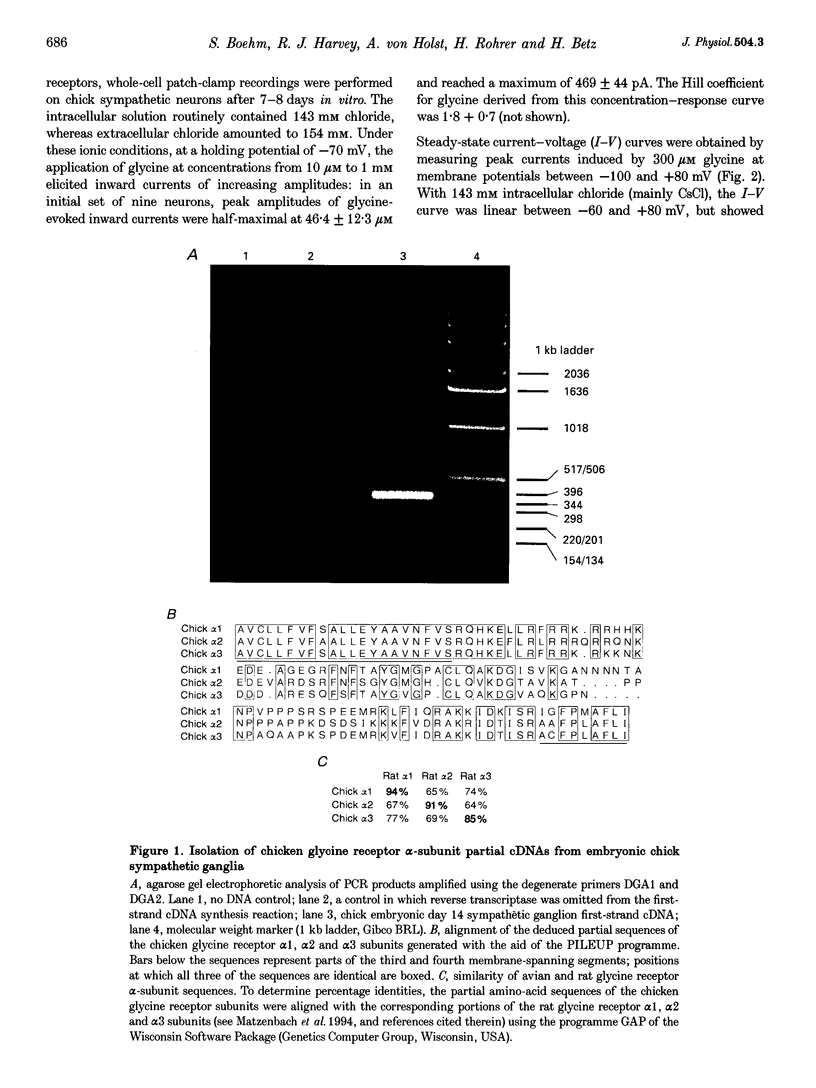
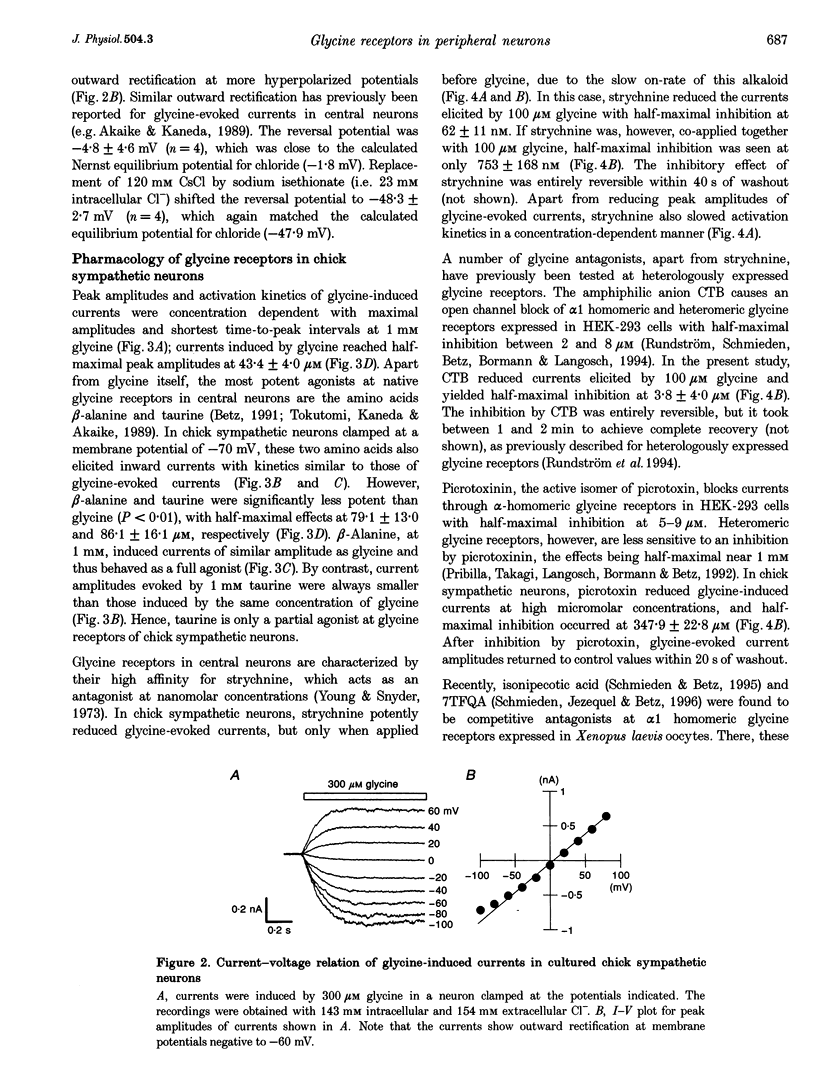
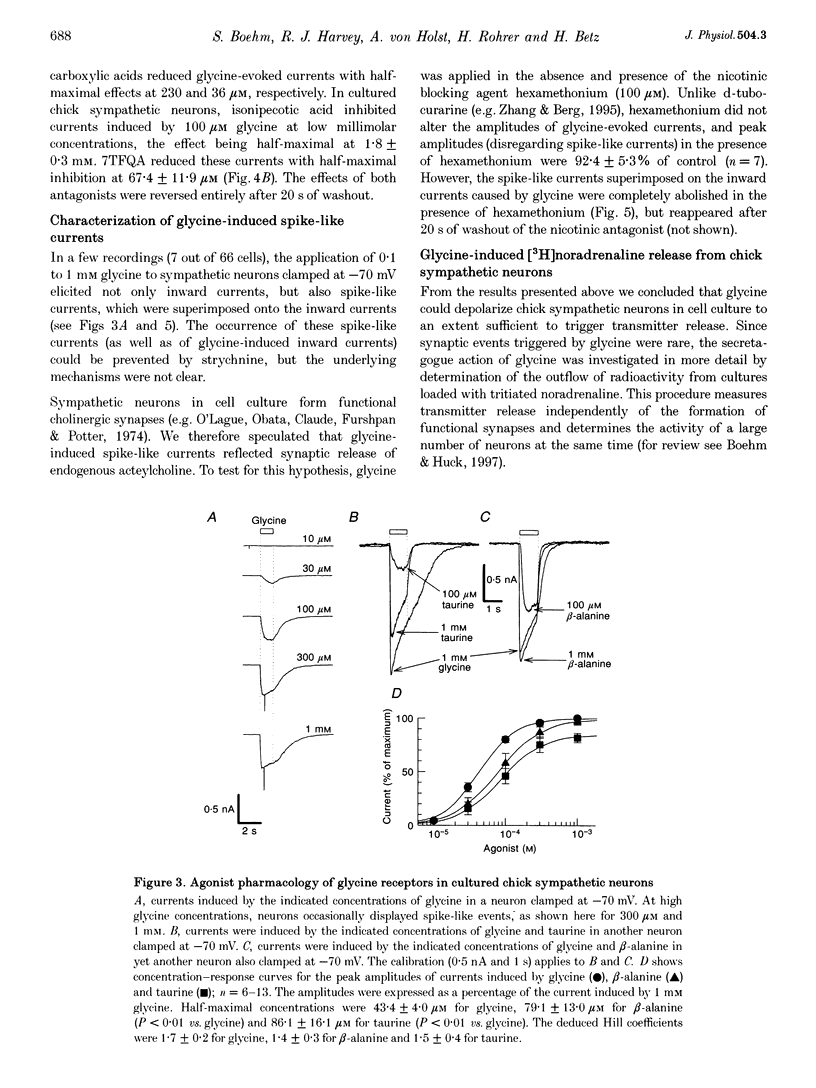
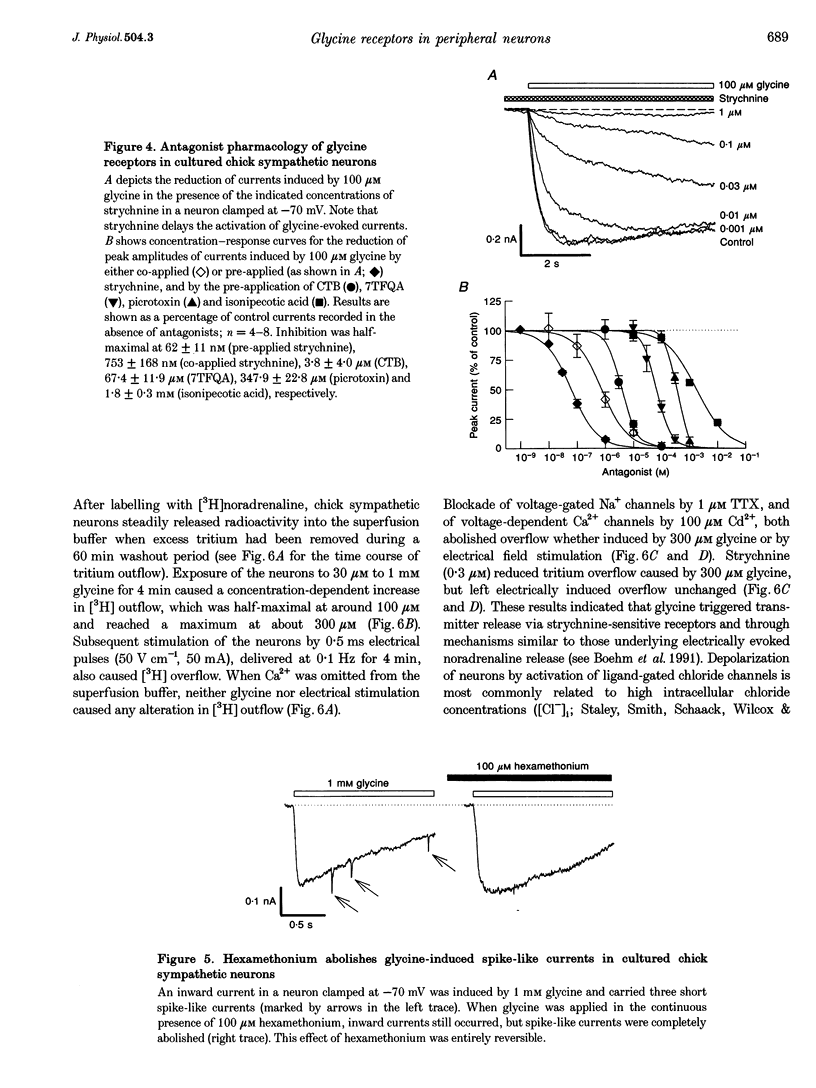
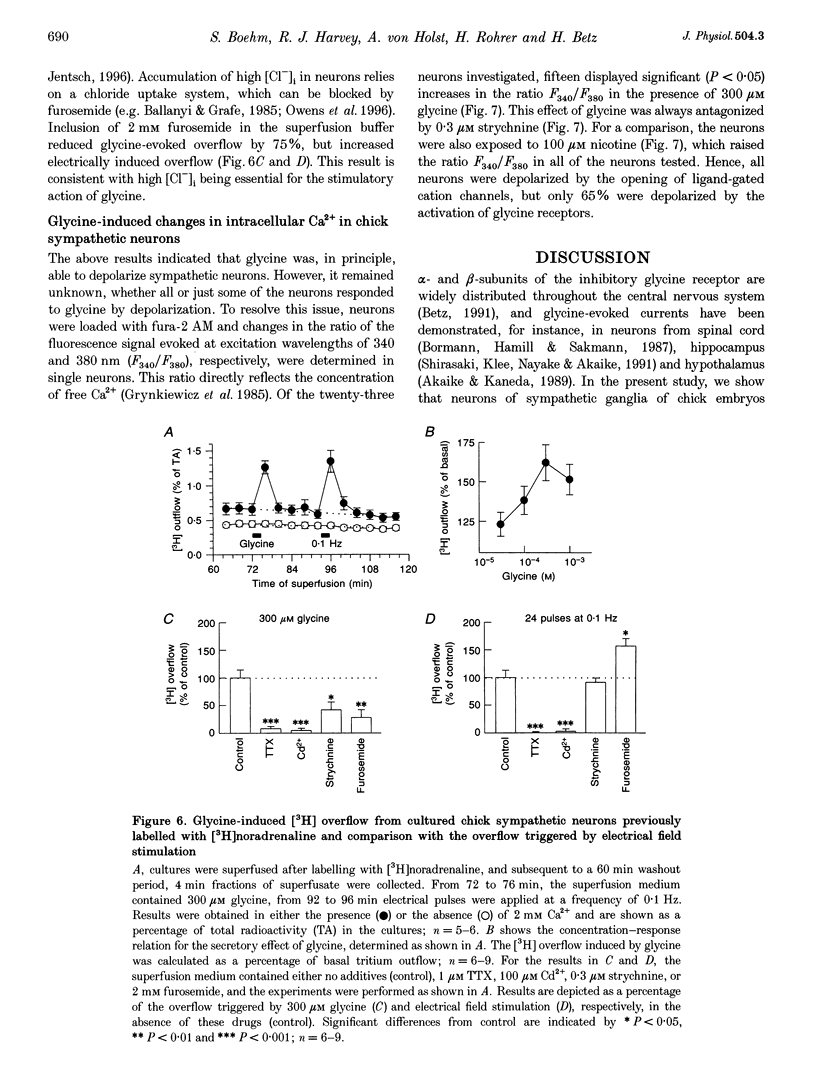
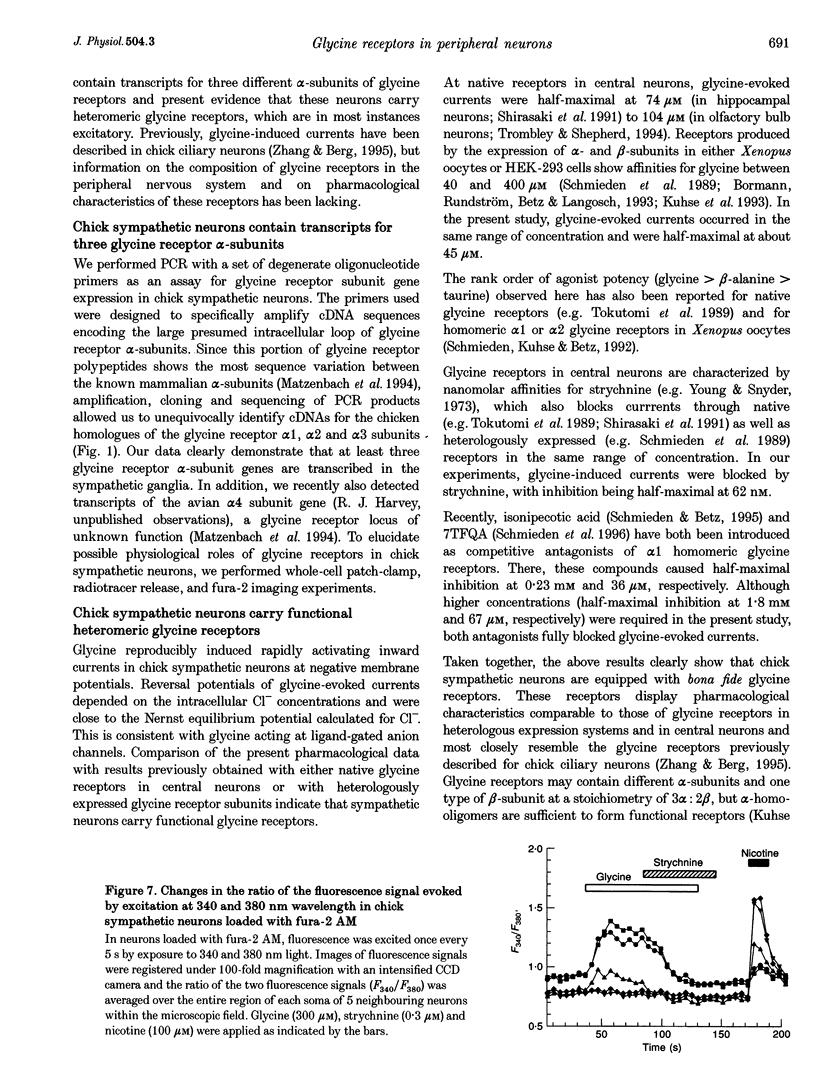
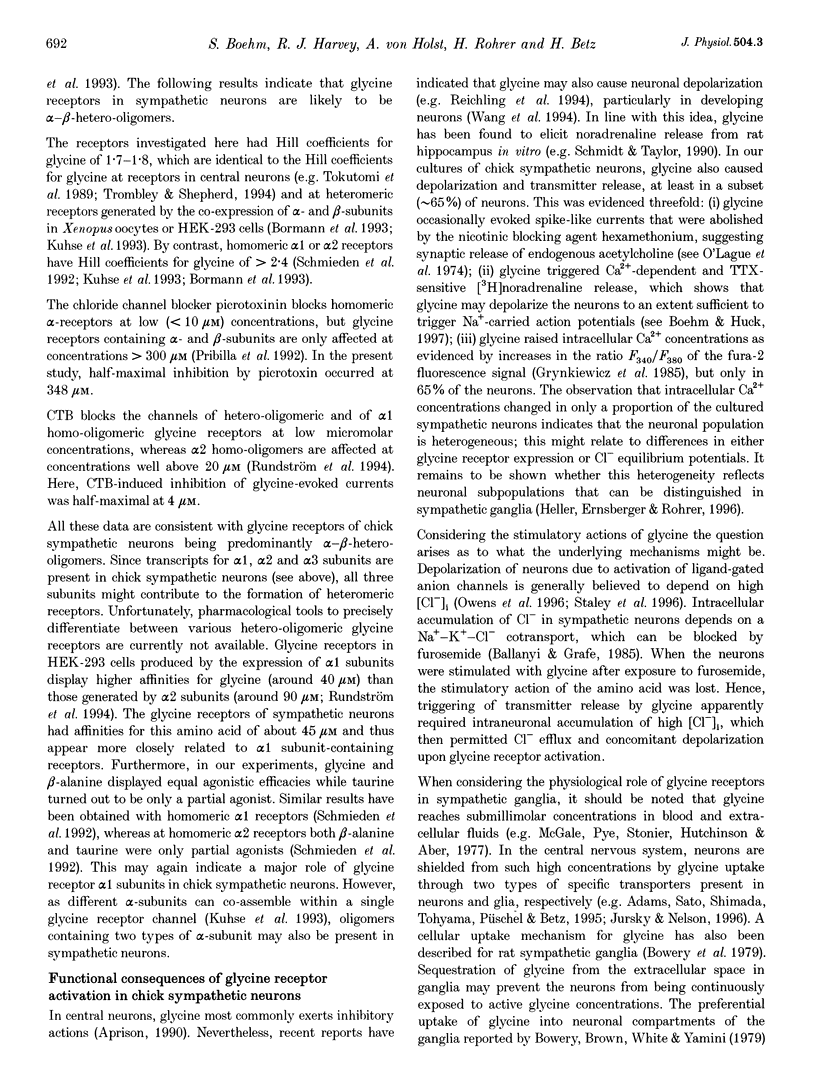
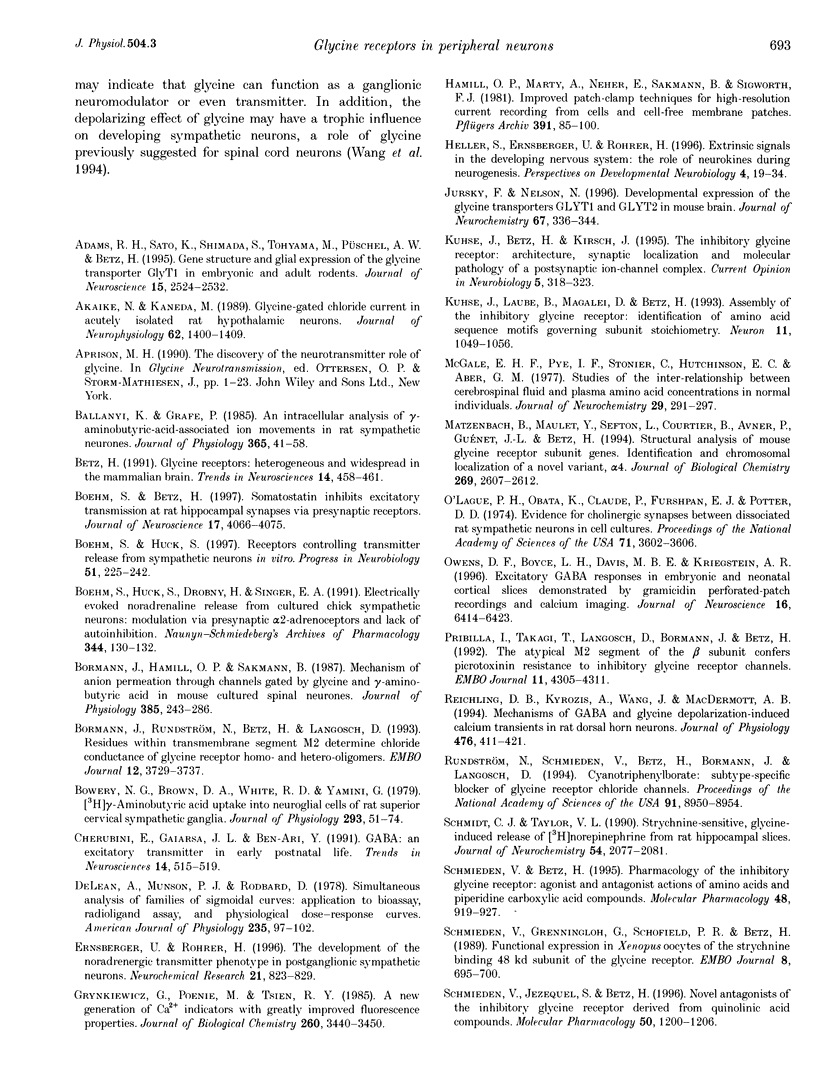
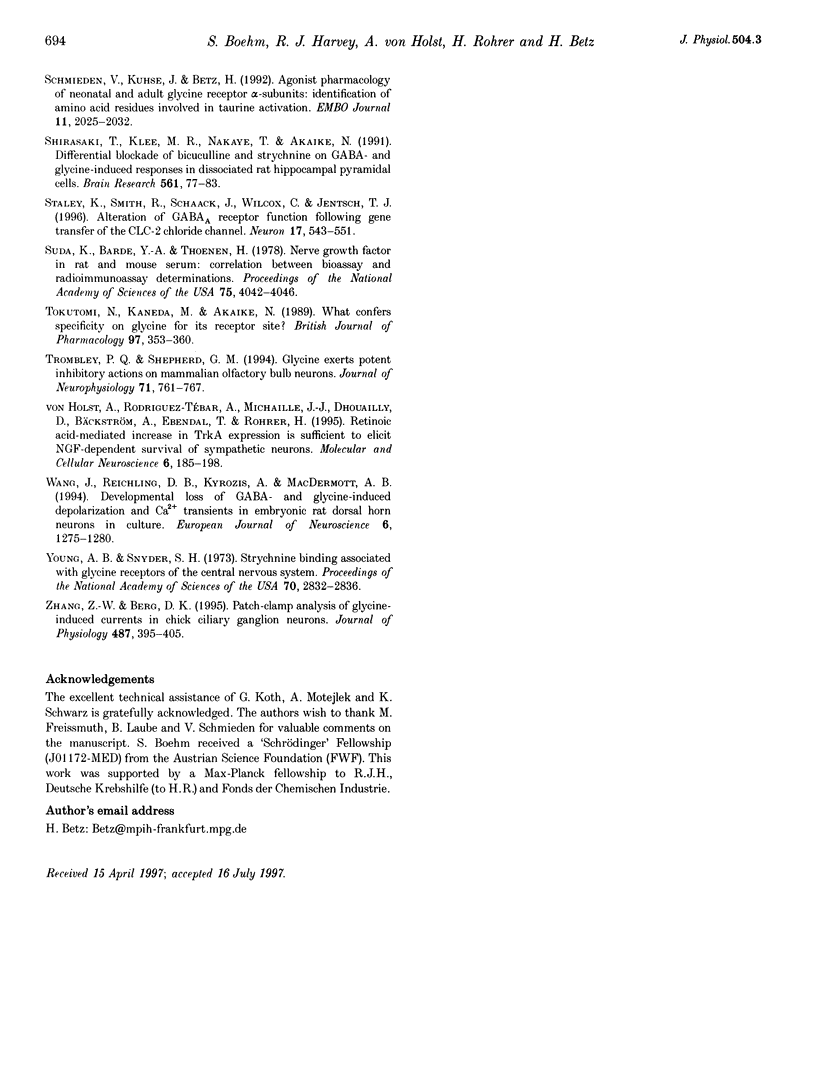
Images in this article
Selected References
These references are in PubMed. This may not be the complete list of references from this article.
- Adams R. H., Sato K., Shimada S., Tohyama M., Püschel A. W., Betz H. Gene structure and glial expression of the glycine transporter GlyT1 in embryonic and adult rodents. J Neurosci. 1995 Mar;15(3 Pt 2):2524–2532. doi: 10.1523/JNEUROSCI.15-03-02524.1995. [DOI] [PMC free article] [PubMed] [Google Scholar]
- Akaike N., Kaneda M. Glycine-gated chloride current in acutely isolated rat hypothalamic neurons. J Neurophysiol. 1989 Dec;62(6):1400–1409. doi: 10.1152/jn.1989.62.6.1400. [DOI] [PubMed] [Google Scholar]
- Ballanyi K., Grafe P. An intracellular analysis of gamma-aminobutyric-acid-associated ion movements in rat sympathetic neurones. J Physiol. 1985 Aug;365:41–58. doi: 10.1113/jphysiol.1985.sp015758. [DOI] [PMC free article] [PubMed] [Google Scholar]
- Betz H. Glycine receptors: heterogeneous and widespread in the mammalian brain. Trends Neurosci. 1991 Oct;14(10):458–461. doi: 10.1016/0166-2236(91)90045-v. [DOI] [PubMed] [Google Scholar]
- Boehm S., Betz H. Somatostatin inhibits excitatory transmission at rat hippocampal synapses via presynaptic receptors. J Neurosci. 1997 Jun 1;17(11):4066–4075. doi: 10.1523/JNEUROSCI.17-11-04066.1997. [DOI] [PMC free article] [PubMed] [Google Scholar]
- Boehm S., Huck S. Receptors controlling transmitter release from sympathetic neurons in vitro. Prog Neurobiol. 1997 Feb;51(3):225–242. doi: 10.1016/s0301-0082(96)00056-1. [DOI] [PubMed] [Google Scholar]
- Bormann J., Hamill O. P., Sakmann B. Mechanism of anion permeation through channels gated by glycine and gamma-aminobutyric acid in mouse cultured spinal neurones. J Physiol. 1987 Apr;385:243–286. doi: 10.1113/jphysiol.1987.sp016493. [DOI] [PMC free article] [PubMed] [Google Scholar]
- Bormann J., Rundström N., Betz H., Langosch D. Residues within transmembrane segment M2 determine chloride conductance of glycine receptor homo- and hetero-oligomers. EMBO J. 1993 Oct;12(10):3729–3737. doi: 10.1002/j.1460-2075.1993.tb06050.x. [DOI] [PMC free article] [PubMed] [Google Scholar]
- Bowery N. G., Brown D. A., White R. D., Yamini G. [3H]gamma-Aminobutyric acid uptake into neuroglial cells of rat superior cervical sympathetic ganglia. J Physiol. 1979 Aug;293:51–74. doi: 10.1113/jphysiol.1979.sp012878. [DOI] [PMC free article] [PubMed] [Google Scholar]
- Böhm S., Huck S., Drobny H., Singer E. A. Electrically evoked noradrenaline release from cultured chick sympathetic neurons: modulation via presynaptic alpha-adrenoceptors and lack of autoinhibition. Naunyn Schmiedebergs Arch Pharmacol. 1991 Jul;344(1):130–132. doi: 10.1007/BF00167393. [DOI] [PubMed] [Google Scholar]
- Cherubini E., Gaiarsa J. L., Ben-Ari Y. GABA: an excitatory transmitter in early postnatal life. Trends Neurosci. 1991 Dec;14(12):515–519. doi: 10.1016/0166-2236(91)90003-d. [DOI] [PubMed] [Google Scholar]
- DeLean A., Munson P. J., Rodbard D. Simultaneous analysis of families of sigmoidal curves: application to bioassay, radioligand assay, and physiological dose-response curves. Am J Physiol. 1978 Aug;235(2):E97–102. doi: 10.1152/ajpendo.1978.235.2.E97. [DOI] [PubMed] [Google Scholar]
- Ernsberger U., Rohrer H. The development of the noradrenergic transmitter phenotype in postganglionic sympathetic neurons. Neurochem Res. 1996 Jul;21(7):823–829. doi: 10.1007/BF02532306. [DOI] [PubMed] [Google Scholar]
- Grynkiewicz G., Poenie M., Tsien R. Y. A new generation of Ca2+ indicators with greatly improved fluorescence properties. J Biol Chem. 1985 Mar 25;260(6):3440–3450. [PubMed] [Google Scholar]
- Hamill O. P., Marty A., Neher E., Sakmann B., Sigworth F. J. Improved patch-clamp techniques for high-resolution current recording from cells and cell-free membrane patches. Pflugers Arch. 1981 Aug;391(2):85–100. doi: 10.1007/BF00656997. [DOI] [PubMed] [Google Scholar]
- Heller S., Ernsberger U., Rohrer H. Extrinsic signals in the developing nervous system: the role of neurokines during neurogenesis. Perspect Dev Neurobiol. 1996;4(1):19–34. [PubMed] [Google Scholar]
- Jursky F., Nelson N. Developmental expression of the glycine transporters GLYT1 and GLYT2 in mouse brain. J Neurochem. 1996 Jul;67(1):336–344. doi: 10.1046/j.1471-4159.1996.67010336.x. [DOI] [PubMed] [Google Scholar]
- Kuhse J., Betz H., Kirsch J. The inhibitory glycine receptor: architecture, synaptic localization and molecular pathology of a postsynaptic ion-channel complex. Curr Opin Neurobiol. 1995 Jun;5(3):318–323. doi: 10.1016/0959-4388(95)80044-1. [DOI] [PubMed] [Google Scholar]
- Kuhse J., Laube B., Magalei D., Betz H. Assembly of the inhibitory glycine receptor: identification of amino acid sequence motifs governing subunit stoichiometry. Neuron. 1993 Dec;11(6):1049–1056. doi: 10.1016/0896-6273(93)90218-g. [DOI] [PubMed] [Google Scholar]
- Matzenbach B., Maulet Y., Sefton L., Courtier B., Avner P., Guénet J. L., Betz H. Structural analysis of mouse glycine receptor alpha subunit genes. Identification and chromosomal localization of a novel variant. J Biol Chem. 1994 Jan 28;269(4):2607–2612. [PubMed] [Google Scholar]
- McGale E. H., Pye I. F., Stonier C., Hutchinson E. C., Aber G. M. Studies of the inter-relationship between cerebrospinal fluid and plasma amino acid concentrations in normal individuals. J Neurochem. 1977 Aug;29(2):291–297. doi: 10.1111/j.1471-4159.1977.tb09621.x. [DOI] [PubMed] [Google Scholar]
- O'Lague P. H., Obata K., Claude P., Furshpan E. J., Potter D. D. Evidence for cholinergic synapses between dissociated rat sympathetic neurons in cell culture. Proc Natl Acad Sci U S A. 1974 Sep;71(9):3602–3606. doi: 10.1073/pnas.71.9.3602. [DOI] [PMC free article] [PubMed] [Google Scholar]
- Owens D. F., Boyce L. H., Davis M. B., Kriegstein A. R. Excitatory GABA responses in embryonic and neonatal cortical slices demonstrated by gramicidin perforated-patch recordings and calcium imaging. J Neurosci. 1996 Oct 15;16(20):6414–6423. doi: 10.1523/JNEUROSCI.16-20-06414.1996. [DOI] [PMC free article] [PubMed] [Google Scholar]
- Pribilla I., Takagi T., Langosch D., Bormann J., Betz H. The atypical M2 segment of the beta subunit confers picrotoxinin resistance to inhibitory glycine receptor channels. EMBO J. 1992 Dec;11(12):4305–4311. doi: 10.1002/j.1460-2075.1992.tb05529.x. [DOI] [PMC free article] [PubMed] [Google Scholar]
- Reichling D. B., Kyrozis A., Wang J., MacDermott A. B. Mechanisms of GABA and glycine depolarization-induced calcium transients in rat dorsal horn neurons. J Physiol. 1994 May 1;476(3):411–421. doi: 10.1113/jphysiol.1994.sp020142. [DOI] [PMC free article] [PubMed] [Google Scholar]
- Rundström N., Schmieden V., Betz H., Bormann J., Langosch D. Cyanotriphenylborate: subtype-specific blocker of glycine receptor chloride channels. Proc Natl Acad Sci U S A. 1994 Sep 13;91(19):8950–8954. doi: 10.1073/pnas.91.19.8950. [DOI] [PMC free article] [PubMed] [Google Scholar]
- Schmidt C. J., Taylor V. L. Strychnine-sensitive, glycine-induced release of [3H]norepinephrine from rat hippocampal slices. J Neurochem. 1990 Jun;54(6):2077–2081. doi: 10.1111/j.1471-4159.1990.tb04913.x. [DOI] [PubMed] [Google Scholar]
- Schmieden V., Betz H. Pharmacology of the inhibitory glycine receptor: agonist and antagonist actions of amino acids and piperidine carboxylic acid compounds. Mol Pharmacol. 1995 Nov;48(5):919–927. [PubMed] [Google Scholar]
- Schmieden V., Grenningloh G., Schofield P. R., Betz H. Functional expression in Xenopus oocytes of the strychnine binding 48 kd subunit of the glycine receptor. EMBO J. 1989 Mar;8(3):695–700. doi: 10.1002/j.1460-2075.1989.tb03428.x. [DOI] [PMC free article] [PubMed] [Google Scholar]
- Schmieden V., Jezequel S., Betz H. Novel antagonists of the inhibitory glycine receptor derived from quinolinic acid compounds. Mol Pharmacol. 1996 Nov;50(5):1200–1206. [PubMed] [Google Scholar]
- Schmieden V., Kuhse J., Betz H. Agonist pharmacology of neonatal and adult glycine receptor alpha subunits: identification of amino acid residues involved in taurine activation. EMBO J. 1992 Jun;11(6):2025–2032. doi: 10.1002/j.1460-2075.1992.tb05259.x. [DOI] [PMC free article] [PubMed] [Google Scholar]
- Shirasaki T., Klee M. R., Nakaye T., Akaike N. Differential blockade of bicuculline and strychnine on GABA- and glycine-induced responses in dissociated rat hippocampal pyramidal cells. Brain Res. 1991 Oct 4;561(1):77–83. doi: 10.1016/0006-8993(91)90751-g. [DOI] [PubMed] [Google Scholar]
- Staley K., Smith R., Schaack J., Wilcox C., Jentsch T. J. Alteration of GABAA receptor function following gene transfer of the CLC-2 chloride channel. Neuron. 1996 Sep;17(3):543–551. doi: 10.1016/s0896-6273(00)80186-5. [DOI] [PubMed] [Google Scholar]
- Suda K., Barde Y. A., Thoenen H. Nerve growth factor in mouse and rat serum: correlation between bioassay and radioimmunoassay determinations. Proc Natl Acad Sci U S A. 1978 Aug;75(8):4042–4046. doi: 10.1073/pnas.75.8.4042. [DOI] [PMC free article] [PubMed] [Google Scholar]
- Tokutomi N., Kaneda M., Akaike N. What confers specificity on glycine for its receptor site? Br J Pharmacol. 1989 Jun;97(2):353–360. doi: 10.1111/j.1476-5381.1989.tb11961.x. [DOI] [PMC free article] [PubMed] [Google Scholar]
- Trombley P. Q., Shepherd G. M. Glycine exerts potent inhibitory actions on mammalian olfactory bulb neurons. J Neurophysiol. 1994 Feb;71(2):761–767. doi: 10.1152/jn.1994.71.2.761. [DOI] [PubMed] [Google Scholar]
- Wang J., Reichling D. B., Kyrozis A., MacDermott A. B. Developmental loss of GABA- and glycine-induced depolarization and Ca2+ transients in embryonic rat dorsal horn neurons in culture. Eur J Neurosci. 1994 Aug 1;6(8):1275–1280. doi: 10.1111/j.1460-9568.1994.tb00317.x. [DOI] [PubMed] [Google Scholar]
- Young A. B., Snyder S. H. Strychnine binding associated with glycine receptors of the central nervous system. Proc Natl Acad Sci U S A. 1973 Oct;70(10):2832–2836. doi: 10.1073/pnas.70.10.2832. [DOI] [PMC free article] [PubMed] [Google Scholar]
- Zhang Z. W., Berg D. K. Patch-clamp analysis of glycine-induced currents in chick ciliary ganglion neurons. J Physiol. 1995 Sep 1;487(Pt 2):395–405. doi: 10.1113/jphysiol.1995.sp020888. [DOI] [PMC free article] [PubMed] [Google Scholar]
- von Holst A., Rodriguez-Tébar A., Michaille J. J., Dhouailly D., Bäckström A., Ebendal T., Rohrer H. Retinoic acid-mediated increase in TrkA expression is sufficient to elicit NGF-dependent survival of sympathetic neurons. Mol Cell Neurosci. 1995 Jun;6(3):185–198. doi: 10.1006/mcne.1995.1016. [DOI] [PubMed] [Google Scholar]