Abstract
1. Whole-cell patch clamp recordings were obtained from sixty-five rat supraoptic nucleus (SON) neurones in brain slices to investigate ionic mechanisms underlying depolarizing after-potentials (DAPs). When cells were voltage clamped around -58 mV, slow inward currents mediating DAPs (IDAP), evoked by three brief depolarizing pulses, had a peak of 17 +/- 1 pA (mean +/- S.E.M.) and lasted for 2.8 +/- 0.1 s. 2. No significant differences in the amplitude and duration were observed when one to three preceding depolarizing pulses were applied, although there was a tendency for twin pulses to evoke larger IDAP than a single pulse. The IDAP was absent when membrane potentials were more negative than -70 mV. In the range -70 to -50 mV, IDAP amplitudes and durations increased as the membrane became more depolarized, with an activation threshold of -65.7 +/- 0.7 mV. 3. IDAP with normal amplitude and duration could be evoked during the decay of a preceding IDAP. As frequencies of depolarizing pulses rose from 2 to 20 Hz, the times to peak IDAP amplitude were reduced but the amplitudes and durations did not change. 4. A consistent reduction in membrane conductance during the IDAP was observed in all SON neurones tested, and averaged 34.6 +/- 3.3%. Small hyperpolarizing pulses used to measure membrane conductances appeared not to disturb major ionic mechanisms underlying IDAP, since the slope and duration of IDAP with and without test pulses were similar. 5. The IDAP had an averaged reversal potential of -87.4 +/- 1.6 mV, which was close to the K+ equilibrium potential. An elevation in [K+]o reduced or abolished the IDAP, and shifted its reversal potential toward more positive levels. Perifusion of slices with 7.5-10 mM TEA, a K+ channel blocker, reversibly suppressed the IDAP. 6. Both Na+ and Ca2+ currents failed to induce an IDAP-like current during perifusion of slices with media containing high [K+]o or TEA. However, the IDAP was abolished by replacing external Ca2+ with Co2+, or replacing 82% of external Na+ with choline or Li+. Perifusion of slices with media containing 1-2 microM TTX also reduced IDAP by 55.5 +/- 9.0%. 7. These results suggest that the generation of DAPs in SON neurones mainly involves a reduction in outward K+ current(s), which probably has little or no inactivation and can be inhibited by [Ca2+]i transients, due to Ca2+ influx during action potentials and Ca2+ release from internal stores. Na+ influx might provide a permissive influence for Ca(2+)-induced reduction of K+ conductances and/or help to raise [Ca2+]i via reverse-mode Ca(2+)-Na+ exchange. Other conductances, making minor contributions to the IDAP, may also be involved.
Full text
PDF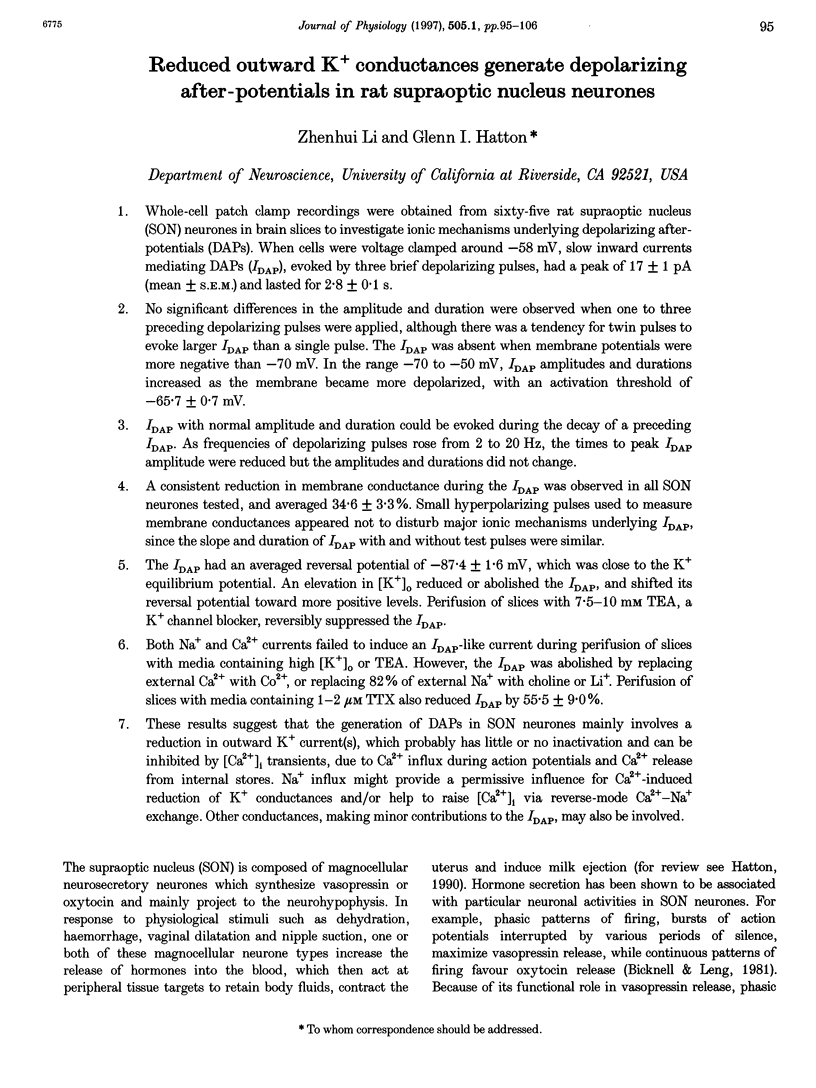
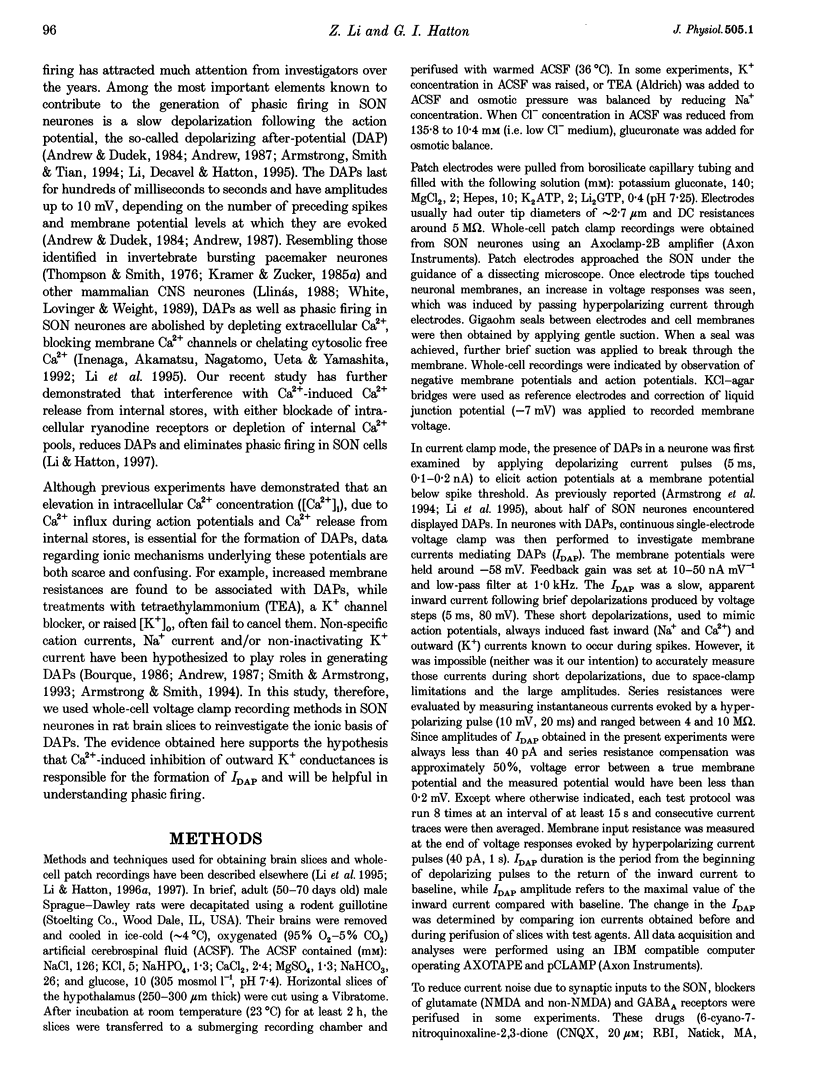
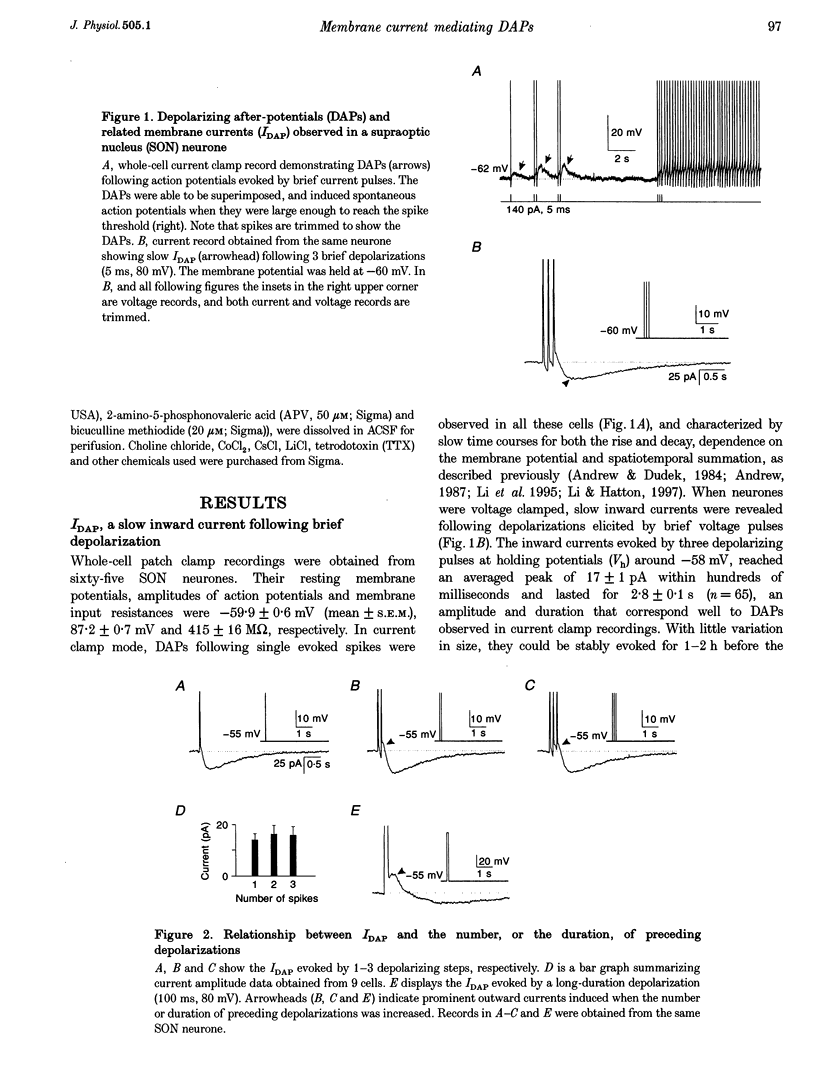
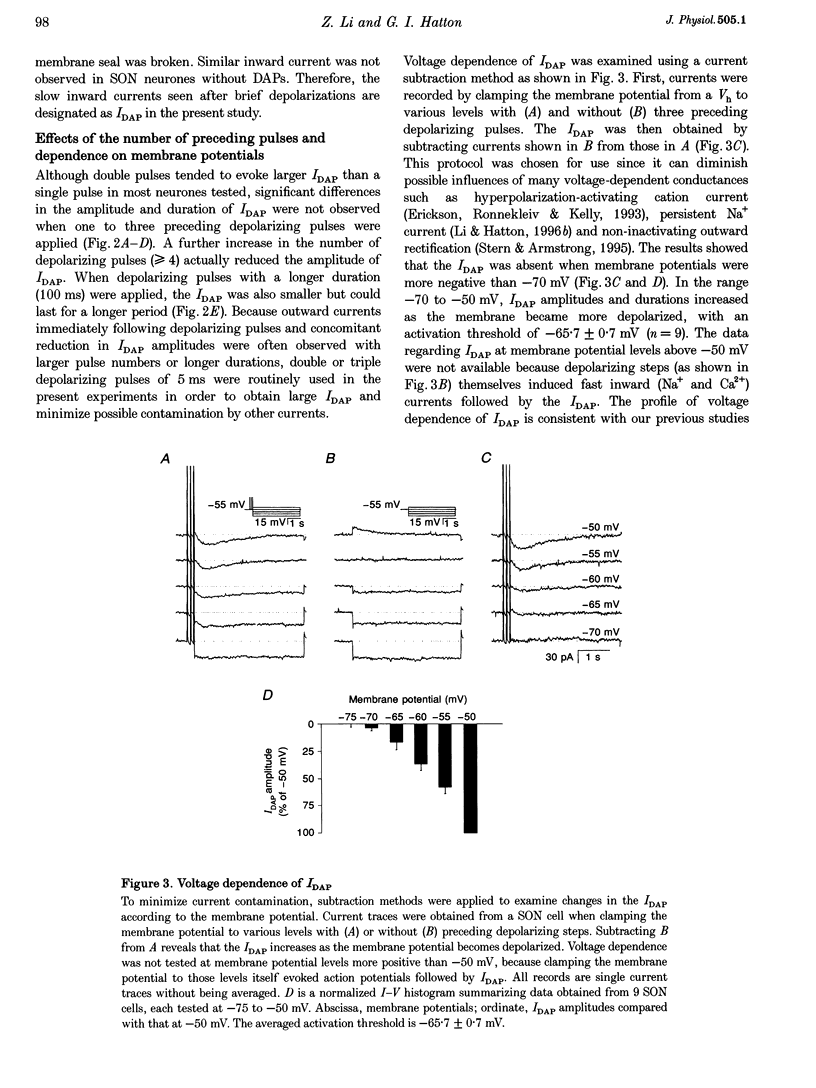
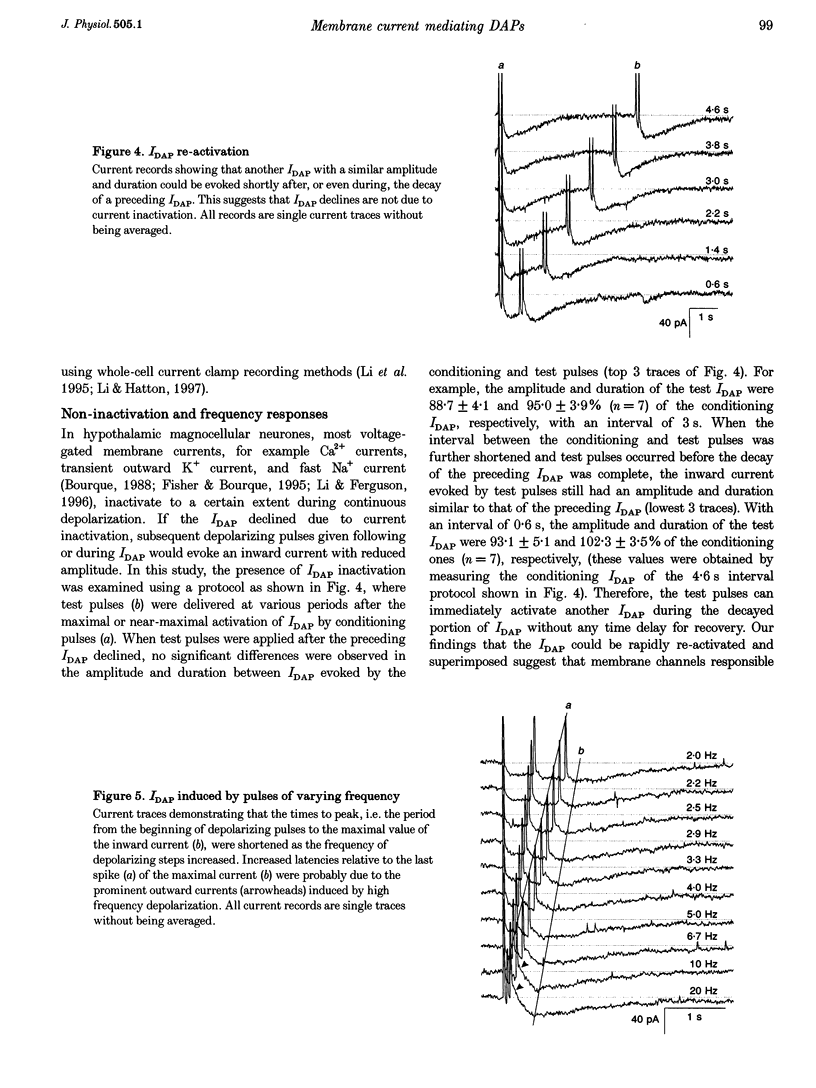
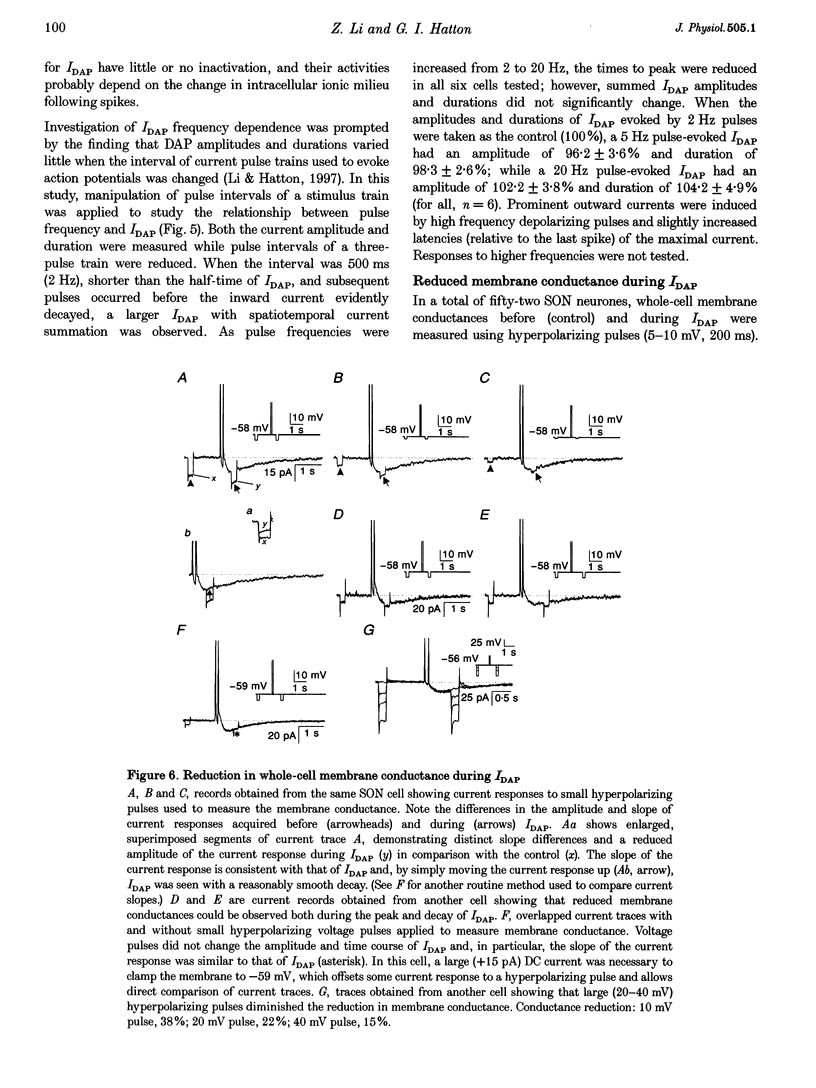
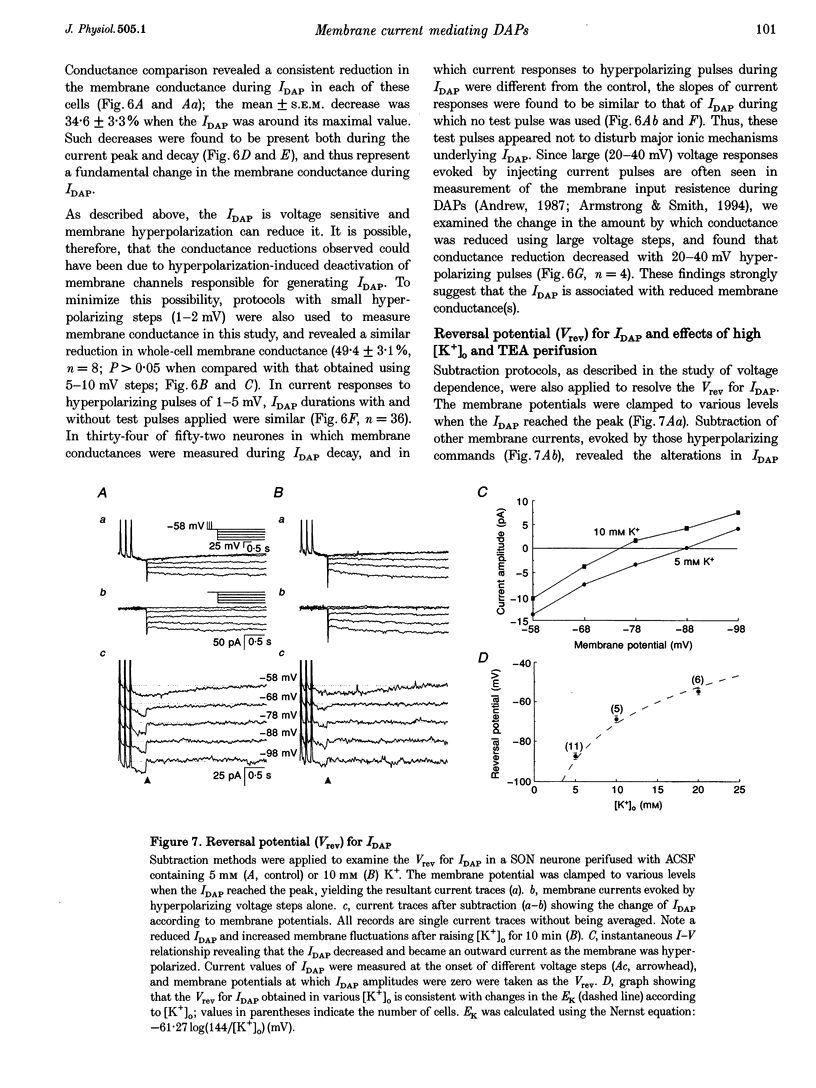
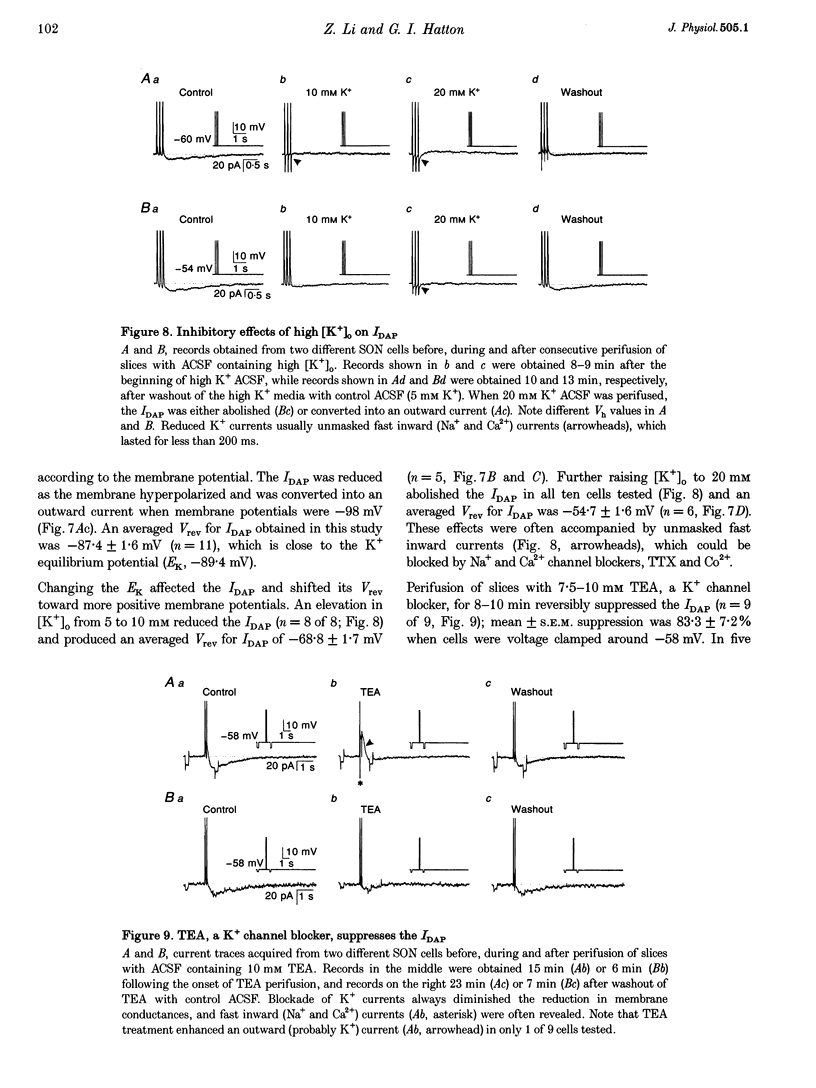
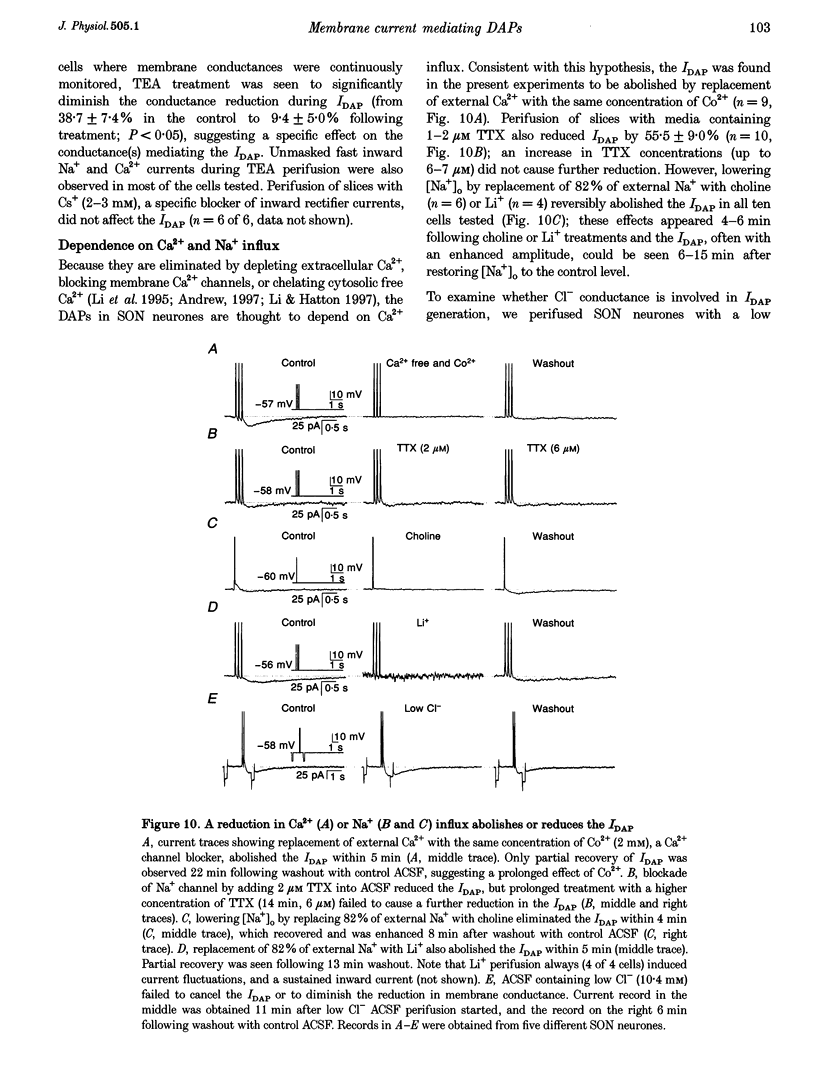
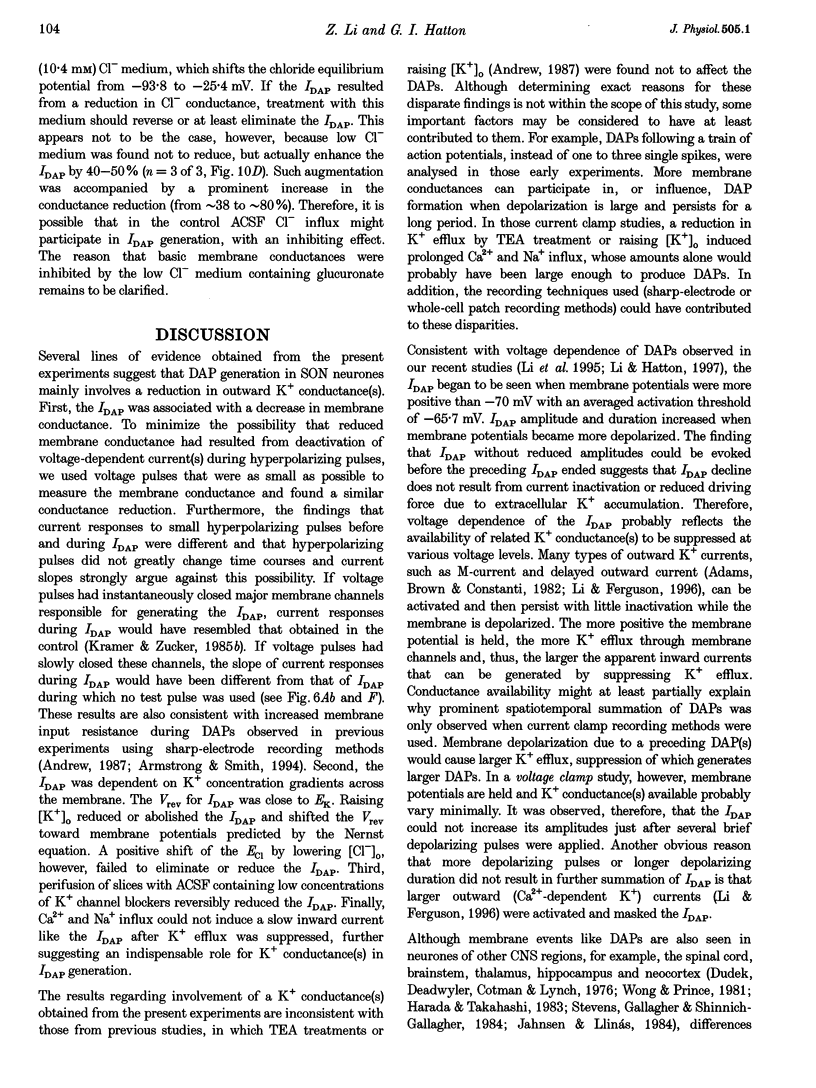
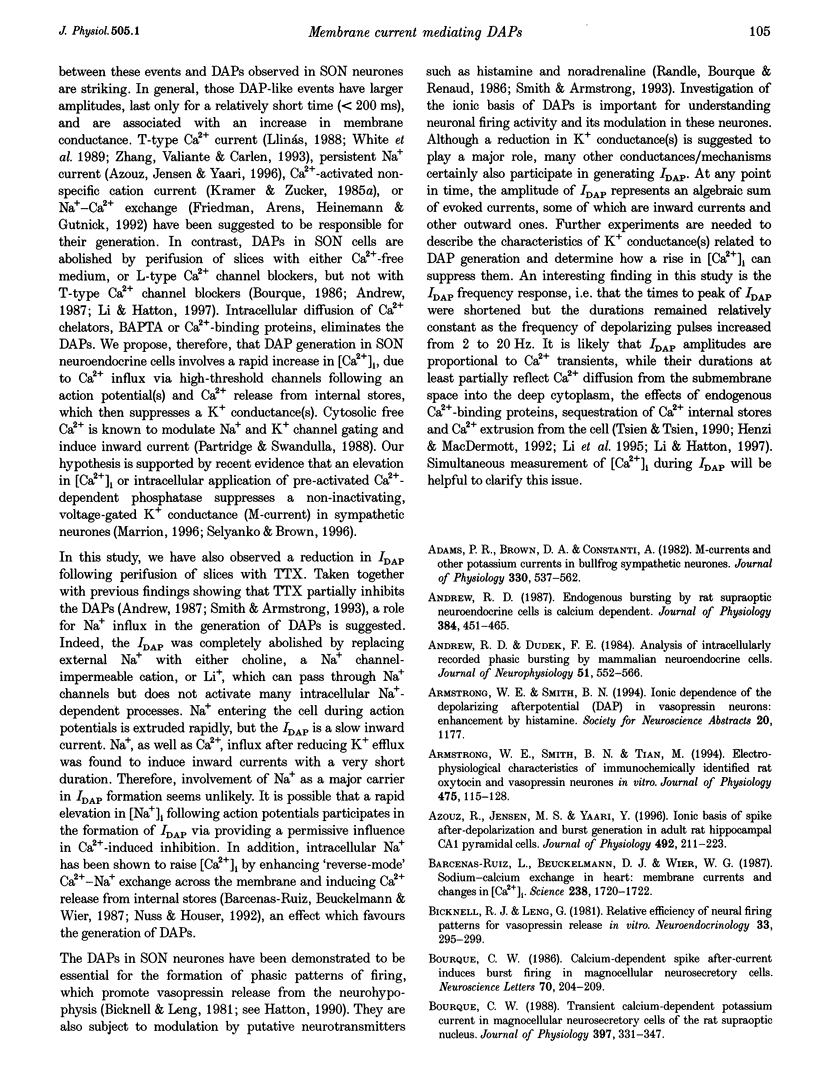
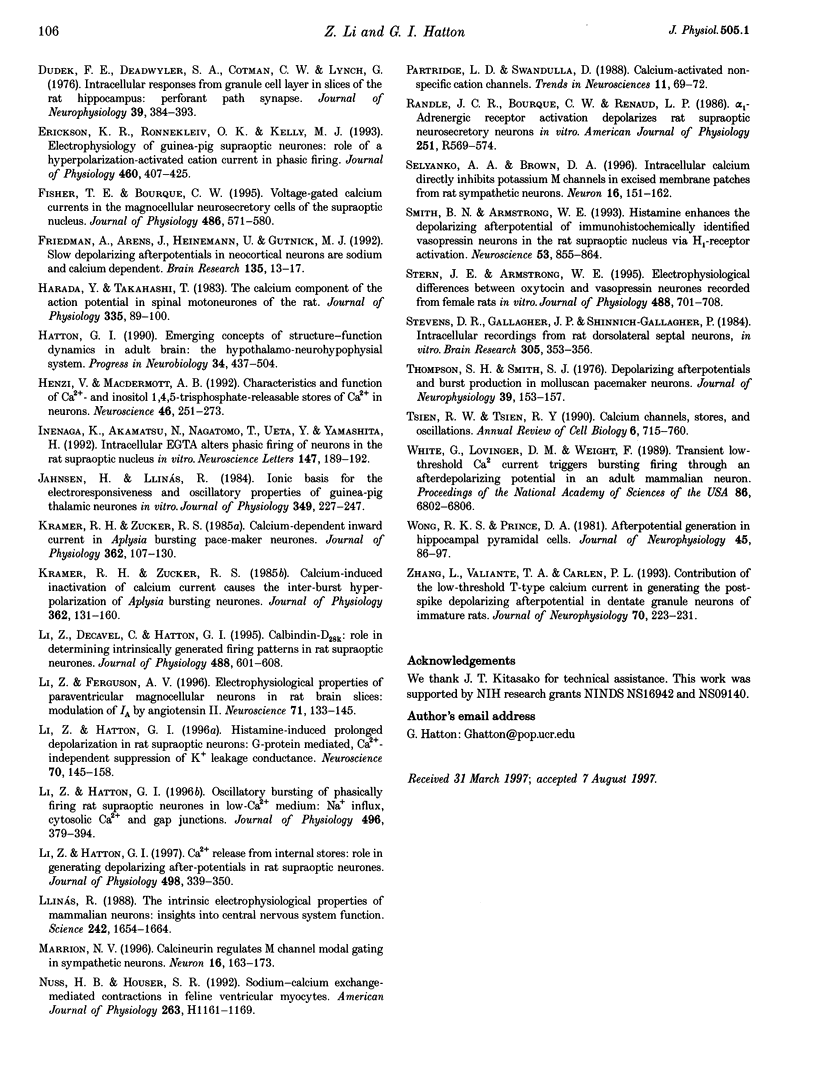
Selected References
These references are in PubMed. This may not be the complete list of references from this article.
- Adams P. R., Brown D. A., Constanti A. M-currents and other potassium currents in bullfrog sympathetic neurones. J Physiol. 1982 Sep;330:537–572. doi: 10.1113/jphysiol.1982.sp014357. [DOI] [PMC free article] [PubMed] [Google Scholar]
- Andrew R. D., Dudek F. E. Analysis of intracellularly recorded phasic bursting by mammalian neuroendocrine cells. J Neurophysiol. 1984 Mar;51(3):552–566. doi: 10.1152/jn.1984.51.3.552. [DOI] [PubMed] [Google Scholar]
- Andrew R. D. Endogenous bursting by rat supraoptic neuroendocrine cells is calcium dependent. J Physiol. 1987 Mar;384:451–465. doi: 10.1113/jphysiol.1987.sp016463. [DOI] [PMC free article] [PubMed] [Google Scholar]
- Armstrong W. E., Smith B. N., Tian M. Electrophysiological characteristics of immunochemically identified rat oxytocin and vasopressin neurones in vitro. J Physiol. 1994 Feb 15;475(1):115–128. doi: 10.1113/jphysiol.1994.sp020053. [DOI] [PMC free article] [PubMed] [Google Scholar]
- Azouz R., Jensen M. S., Yaari Y. Ionic basis of spike after-depolarization and burst generation in adult rat hippocampal CA1 pyramidal cells. J Physiol. 1996 Apr 1;492(Pt 1):211–223. doi: 10.1113/jphysiol.1996.sp021302. [DOI] [PMC free article] [PubMed] [Google Scholar]
- Barcenas-Ruiz L., Beuckelmann D. J., Wier W. G. Sodium-calcium exchange in heart: membrane currents and changes in [Ca2+]i. Science. 1987 Dec 18;238(4834):1720–1722. doi: 10.1126/science.3686010. [DOI] [PubMed] [Google Scholar]
- Bicknell R. J., Leng G. Relative efficiency of neural firing patterns for vasopressin release in vitro. Neuroendocrinology. 1981 Nov;33(5):295–299. doi: 10.1159/000123248. [DOI] [PubMed] [Google Scholar]
- Bourque C. W. Calcium-dependent spike after-current induces burst firing in magnocellular neurosecretory cells. Neurosci Lett. 1986 Oct 8;70(2):204–209. doi: 10.1016/0304-3940(86)90464-7. [DOI] [PubMed] [Google Scholar]
- Bourque C. W. Transient calcium-dependent potassium current in magnocellular neurosecretory cells of the rat supraoptic nucleus. J Physiol. 1988 Mar;397:331–347. doi: 10.1113/jphysiol.1988.sp017004. [DOI] [PMC free article] [PubMed] [Google Scholar]
- Dudek F. E., Deadwyler S. A., Cotman C. W., Lynch G. Intracellular responses from granule cell layer in slices of rat hippocampus: perforant path synapse. J Neurophysiol. 1976 Mar;39(2):384–393. doi: 10.1152/jn.1976.39.2.384. [DOI] [PubMed] [Google Scholar]
- Erickson K. R., Ronnekleiv O. K., Kelly M. J. Electrophysiology of guinea-pig supraoptic neurones: role of a hyperpolarization-activated cation current in phasic firing. J Physiol. 1993 Jan;460:407–425. doi: 10.1113/jphysiol.1993.sp019478. [DOI] [PMC free article] [PubMed] [Google Scholar]
- Fisher T. E., Bourque C. W. Voltage-gated calcium currents in the magnocellular neurosecretory cells of the rat supraoptic nucleus. J Physiol. 1995 Aug 1;486(Pt 3):571–580. doi: 10.1113/jphysiol.1995.sp020835. [DOI] [PMC free article] [PubMed] [Google Scholar]
- Friedman A., Arens J., Heinemann U., Gutnick M. J. Slow depolarizing afterpotentials in neocortical neurons are sodium and calcium dependent. Neurosci Lett. 1992 Jan 20;135(1):13–17. doi: 10.1016/0304-3940(92)90125-q. [DOI] [PubMed] [Google Scholar]
- Harada Y., Takahashi T. The calcium component of the action potential in spinal motoneurones of the rat. J Physiol. 1983 Feb;335:89–100. doi: 10.1113/jphysiol.1983.sp014521. [DOI] [PMC free article] [PubMed] [Google Scholar]
- Hatton G. I. Emerging concepts of structure-function dynamics in adult brain: the hypothalamo-neurohypophysial system. Prog Neurobiol. 1990;34(6):437–504. doi: 10.1016/0301-0082(90)90017-b. [DOI] [PubMed] [Google Scholar]
- Henzi V., MacDermott A. B. Characteristics and function of Ca(2+)- and inositol 1,4,5-trisphosphate-releasable stores of Ca2+ in neurons. Neuroscience. 1992;46(2):251–273. doi: 10.1016/0306-4522(92)90049-8. [DOI] [PubMed] [Google Scholar]
- Inenaga K., Akamatsu N., Nagatomo T., Ueta Y., Yamashita H. Intracellular EGTA alters phasic firing of neurons in the rat supraoptic nucleus in vitro. Neurosci Lett. 1992 Dec 7;147(2):189–192. doi: 10.1016/0304-3940(92)90592-u. [DOI] [PubMed] [Google Scholar]
- Jahnsen H., Llinás R. Ionic basis for the electro-responsiveness and oscillatory properties of guinea-pig thalamic neurones in vitro. J Physiol. 1984 Apr;349:227–247. doi: 10.1113/jphysiol.1984.sp015154. [DOI] [PMC free article] [PubMed] [Google Scholar]
- Kramer R. H., Zucker R. S. Calcium-dependent inward current in Aplysia bursting pace-maker neurones. J Physiol. 1985 May;362:107–130. doi: 10.1113/jphysiol.1985.sp015666. [DOI] [PMC free article] [PubMed] [Google Scholar]
- Kramer R. H., Zucker R. S. Calcium-induced inactivation of calcium current causes the inter-burst hyperpolarization of Aplysia bursting neurones. J Physiol. 1985 May;362:131–160. doi: 10.1113/jphysiol.1985.sp015667. [DOI] [PMC free article] [PubMed] [Google Scholar]
- Li Z., Decavel C., Hatton G. I. Calbindin-D28k: role in determining intrinsically generated firing patterns in rat supraoptic neurones. J Physiol. 1995 Nov 1;488(Pt 3):601–608. doi: 10.1113/jphysiol.1995.sp020993. [DOI] [PMC free article] [PubMed] [Google Scholar]
- Li Z., Ferguson A. V. Electrophysiological properties of paraventricular magnocellular neurons in rat brain slices: modulation of IA by angiotensin II. Neuroscience. 1996 Mar;71(1):133–145. doi: 10.1016/0306-4522(95)00434-3. [DOI] [PubMed] [Google Scholar]
- Li Z., Hatton G. I. Ca2+ release from internal stores: role in generating depolarizing after-potentials in rat supraoptic neurones. J Physiol. 1997 Jan 15;498(Pt 2):339–350. doi: 10.1113/jphysiol.1997.sp021862. [DOI] [PMC free article] [PubMed] [Google Scholar]
- Li Z., Hatton G. I. Histamine-induced prolonged depolarization in rat supraoptic neurons: G-protein-mediated, Ca(2+)-independent suppression of K+ leakage conductance. Neuroscience. 1996 Jan;70(1):145–158. doi: 10.1016/0306-4522(95)00373-q. [DOI] [PubMed] [Google Scholar]
- Li Z., Hatton G. I. Oscillatory bursting of phasically firing rat supraoptic neurones in low-Ca2+ medium: Na+ influx, cytosolic Ca2+ and gap junctions. J Physiol. 1996 Oct 15;496(Pt 2):379–394. doi: 10.1113/jphysiol.1996.sp021692. [DOI] [PMC free article] [PubMed] [Google Scholar]
- Marrion N. V. Calcineurin regulates M channel modal gating in sympathetic neurons. Neuron. 1996 Jan;16(1):163–173. doi: 10.1016/s0896-6273(00)80033-1. [DOI] [PubMed] [Google Scholar]
- Partridge L. D., Swandulla D. Calcium-activated non-specific cation channels. Trends Neurosci. 1988 Feb;11(2):69–72. doi: 10.1016/0166-2236(88)90167-1. [DOI] [PubMed] [Google Scholar]
- Randle J. C., Bourque C. W., Renaud L. P. Alpha 1-adrenergic receptor activation depolarizes rat supraoptic neurosecretory neurons in vitro. Am J Physiol. 1986 Sep;251(3 Pt 2):R569–R574. doi: 10.1152/ajpregu.1986.251.3.R569. [DOI] [PubMed] [Google Scholar]
- Selyanko A. A., Brown D. A. Intracellular calcium directly inhibits potassium M channels in excised membrane patches from rat sympathetic neurons. Neuron. 1996 Jan;16(1):151–162. doi: 10.1016/s0896-6273(00)80032-x. [DOI] [PubMed] [Google Scholar]
- Smith B. N., Armstrong W. E. Histamine enhances the depolarizing afterpotential of immunohistochemically identified vasopressin neurons in the rat supraoptic nucleus via H1-receptor activation. Neuroscience. 1993 Apr;53(3):855–864. doi: 10.1016/0306-4522(93)90630-x. [DOI] [PubMed] [Google Scholar]
- Stern J. E., Armstrong W. E. Electrophysiological differences between oxytocin and vasopressin neurones recorded from female rats in vitro. J Physiol. 1995 Nov 1;488(Pt 3):701–708. doi: 10.1113/jphysiol.1995.sp021001. [DOI] [PMC free article] [PubMed] [Google Scholar]
- Stevens D. R., Gallagher J. P., Shinnick-Gallagher P. Intracellular recordings from rat dorsolateral septal neurons, in vitro. Brain Res. 1984 Jul 9;305(2):353–356. doi: 10.1016/0006-8993(84)90441-4. [DOI] [PubMed] [Google Scholar]
- Thompson S. H., Smith S. J. Depolarizing afterpotentials and burst production in molluscan pacemaker neurons. J Neurophysiol. 1976 Jan;39(1):153–161. doi: 10.1152/jn.1976.39.1.153. [DOI] [PubMed] [Google Scholar]
- Tsien R. W., Tsien R. Y. Calcium channels, stores, and oscillations. Annu Rev Cell Biol. 1990;6:715–760. doi: 10.1146/annurev.cb.06.110190.003435. [DOI] [PubMed] [Google Scholar]
- White G., Lovinger D. M., Weight F. F. Transient low-threshold Ca2+ current triggers burst firing through an afterdepolarizing potential in an adult mammalian neuron. Proc Natl Acad Sci U S A. 1989 Sep;86(17):6802–6806. doi: 10.1073/pnas.86.17.6802. [DOI] [PMC free article] [PubMed] [Google Scholar]
- Wong R. K., Prince D. A. Afterpotential generation in hippocampal pyramidal cells. J Neurophysiol. 1981 Jan;45(1):86–97. doi: 10.1152/jn.1981.45.1.86. [DOI] [PubMed] [Google Scholar]
- Zhang L., Valiante T. A., Carlen P. L. Contribution of the low-threshold T-type calcium current in generating the post-spike depolarizing afterpotential in dentate granule neurons of immature rats. J Neurophysiol. 1993 Jul;70(1):223–231. doi: 10.1152/jn.1993.70.1.223. [DOI] [PubMed] [Google Scholar]