Abstract
1. We have examined the clearance of synaptically released acetylcholine in the otic ganglion when acetylcholinesterase was blocked with eserine. 2. Intracellular recordings were made from otic ganglion neurones, in vitro. The decay of the excitatory postsynaptic potential (EPSP), in response to stimulation of afferent fibres, was greatly prolonged in the presence of eserine. Low frequency (0.05-4 Hz) repetitive synaptic stimulation led to a slow depolarization of the postsynaptic cell that persisted throughout the period of stimulation. This slow depolarization was blocked by the nicotinic antagonists mecamylamine (100 microM) or (+)tubocurarine (100 microM), but was unaffected by atropine (1 microM), indicating that the response was due to the activation of nicotinic receptors. 3. Following 2 Hz synaptic stimulation (30 s), the rate of rise of the slow depolarization had a time constant of 3.1 +/- 0.4 s and a peak amplitude of 12 +/- 1 mV. Upon cessation of stimulation, the depolarization decayed to resting levels with a time constant of 18.3 +/- 1.5 s (n = 23). At increasing stimulation frequencies the rate of rise of the depolarization increased. Lowering the probability of release, by adding cadmium to the perfusing solution or by lowering extracellular calcium, slowed the rise time of the response. 4. Both the onset and decay kinetics of the slow depolarization had a low temperature sensitivity, indicating that they reflect diffusional processes. 5. Repetitive stimulation (2 Hz) of the afferent nerve supplying the ganglion, in the presence of eserine, also caused a slow depolarization in cell in which we could not demonstrate a synaptic input. This indicates that synaptically released acetylcholine can spill over onto nearby neurones. 6. We conclude that at parasympathetic synapses, under physiological conditions, transmitter action is terminated by the enzymatic degradation of acetylcholine. When acetylcholinesterase is blocked, acetylcholine accumulates because its removal by diffusion is slow.
Full text
PDF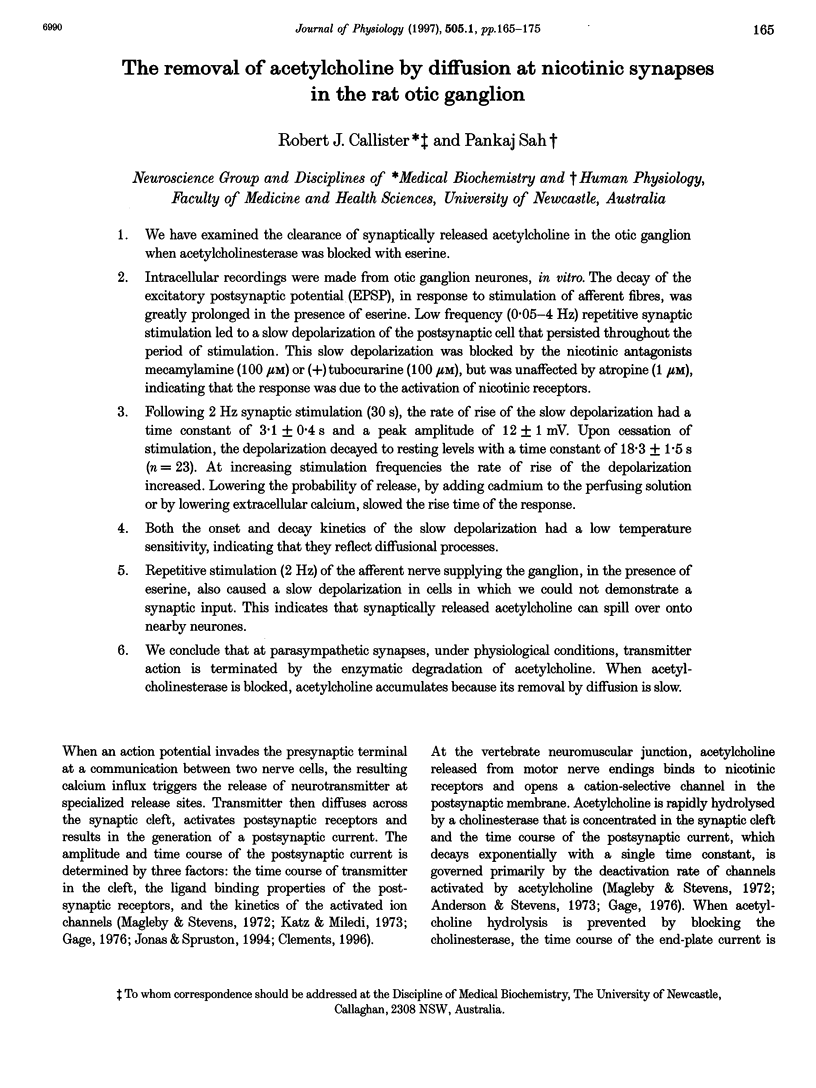
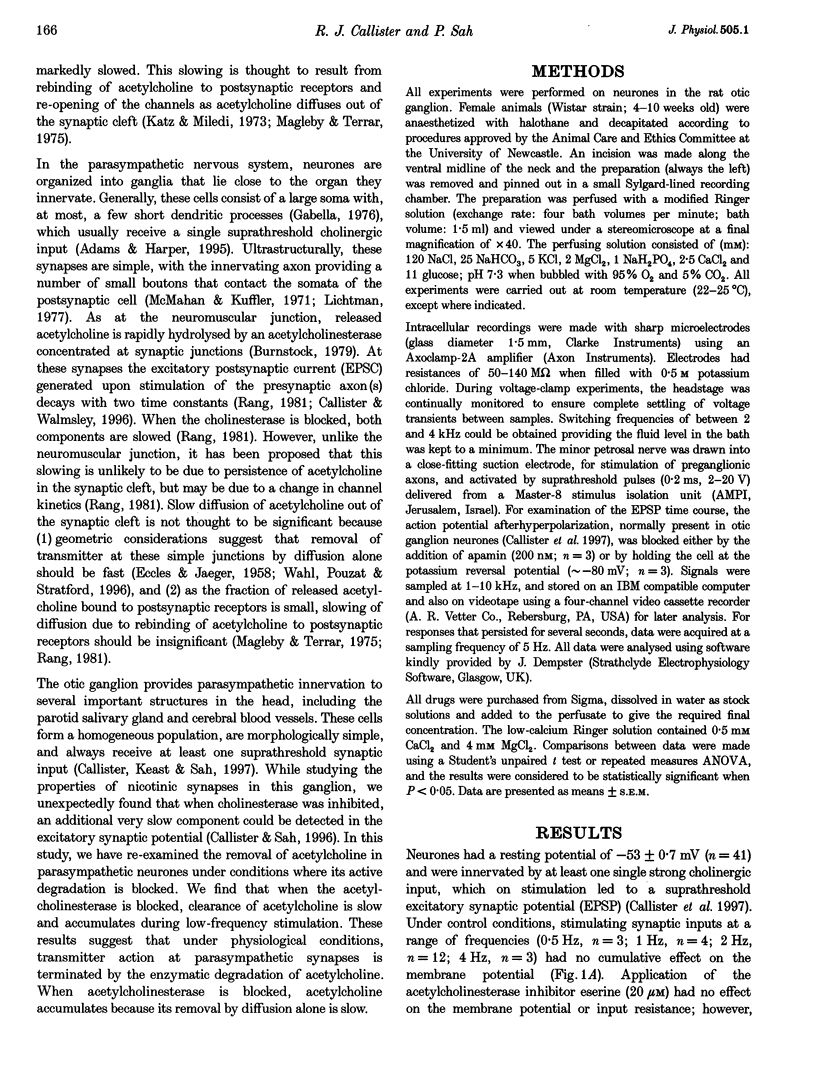
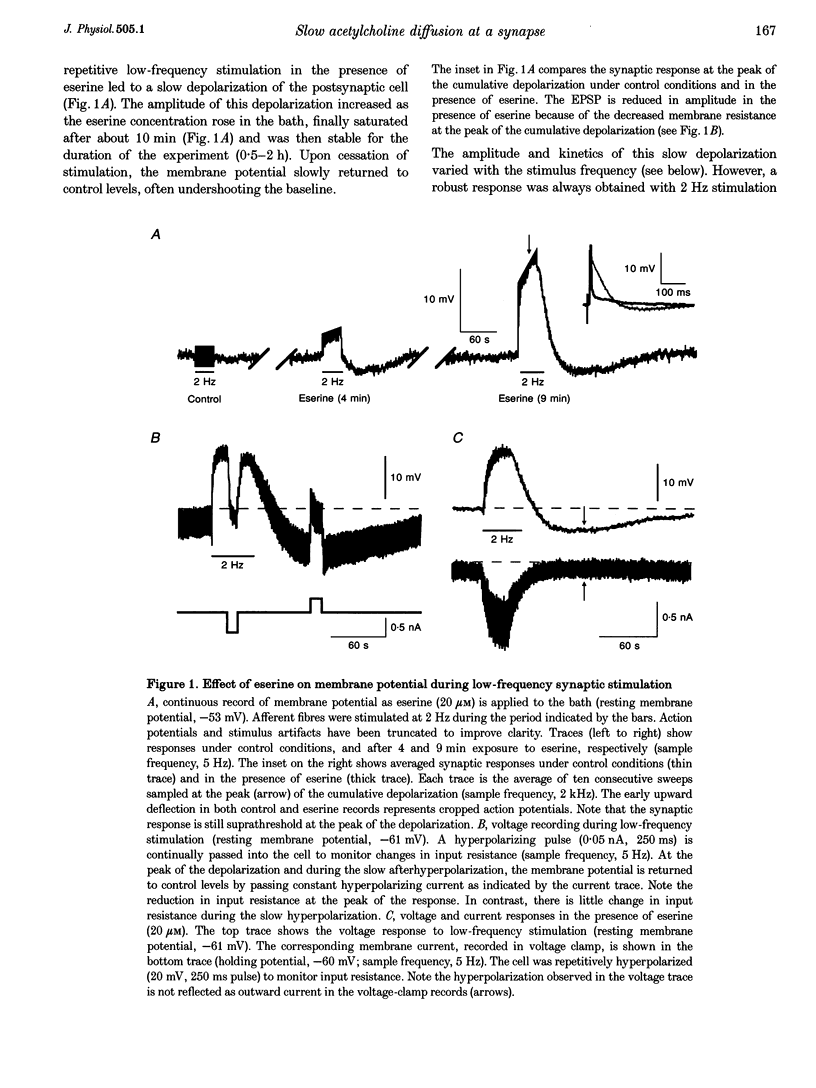
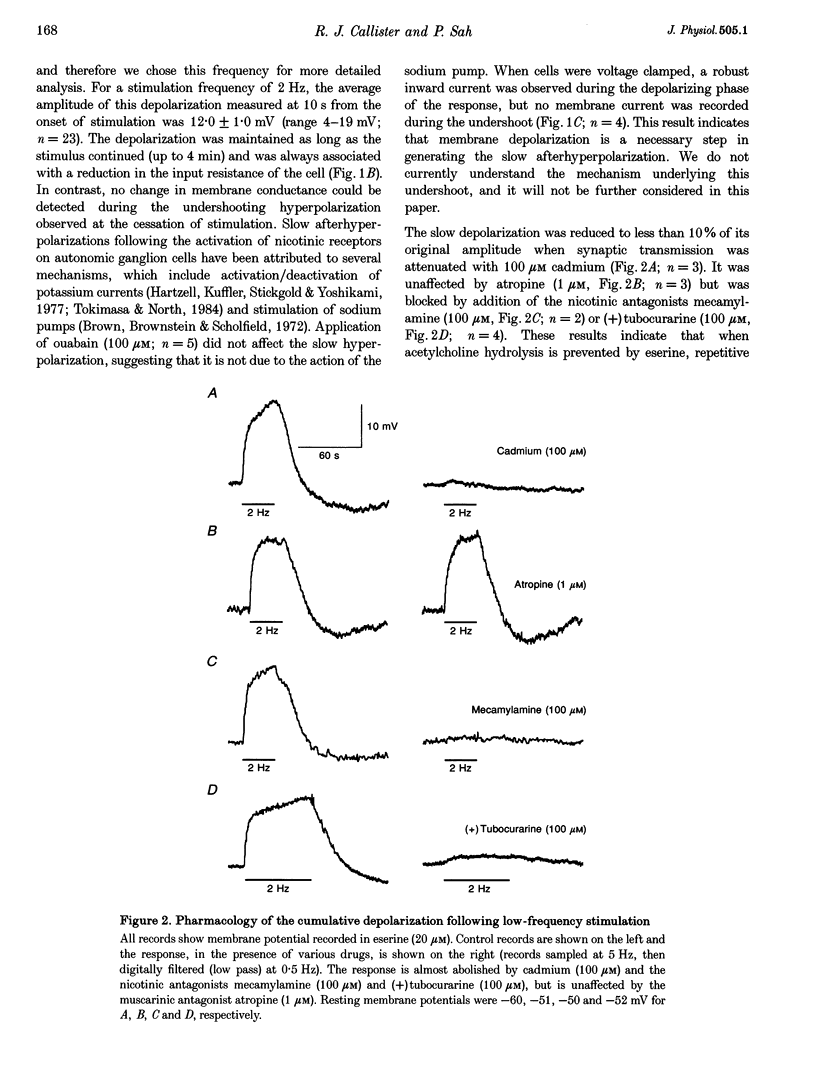
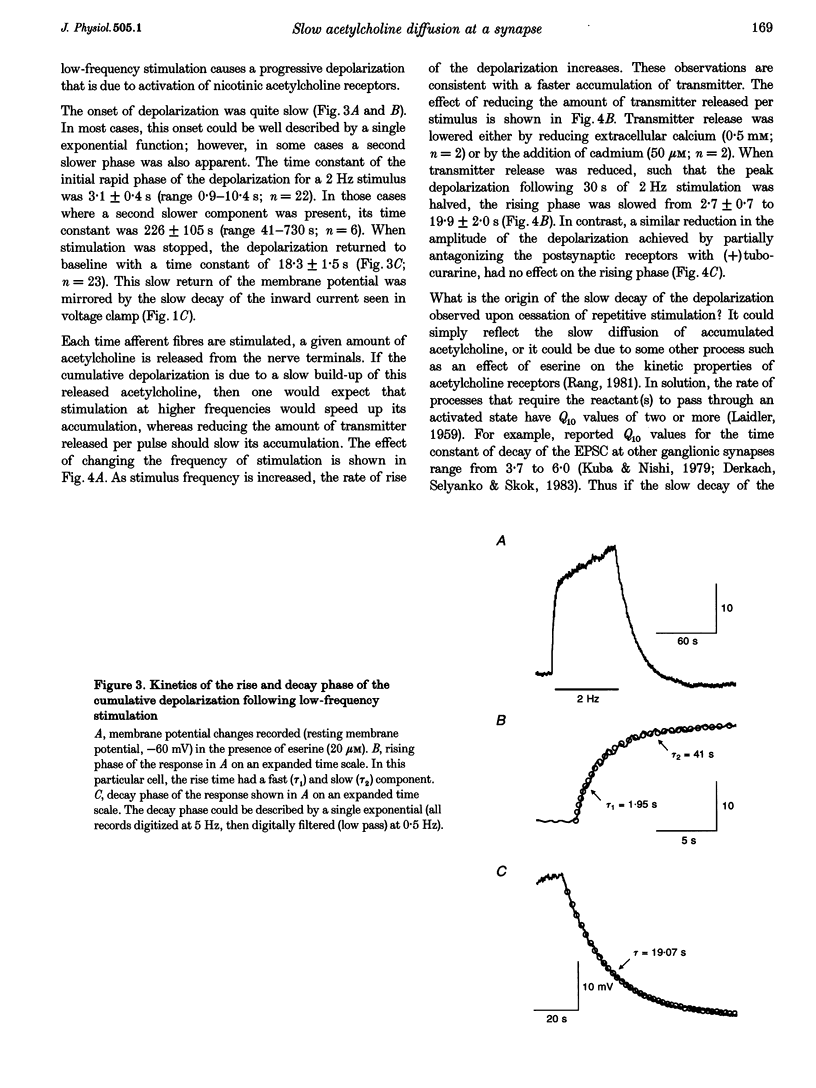
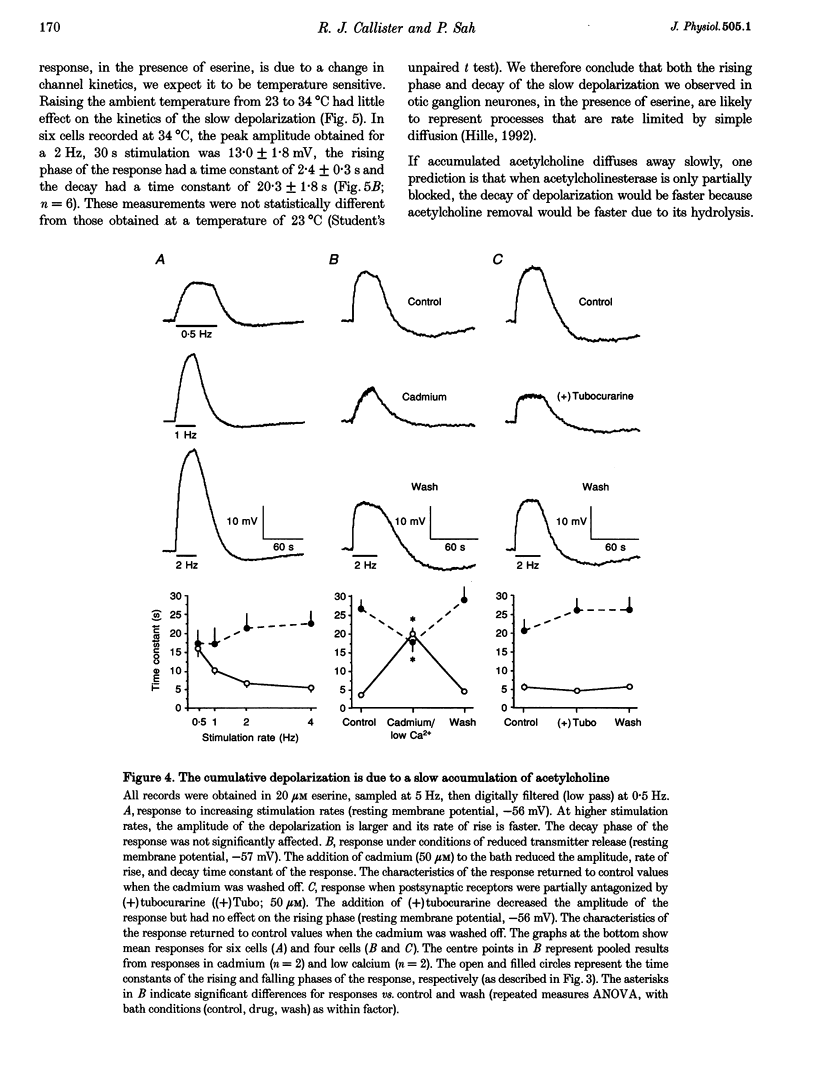
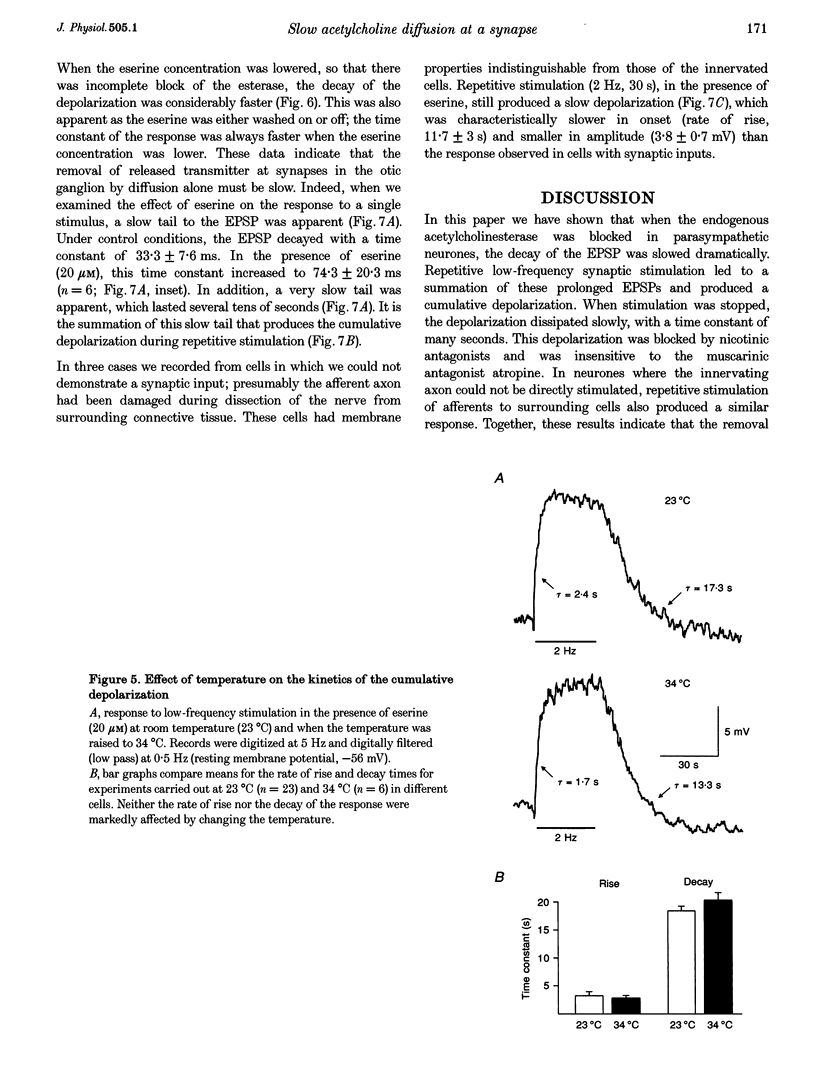
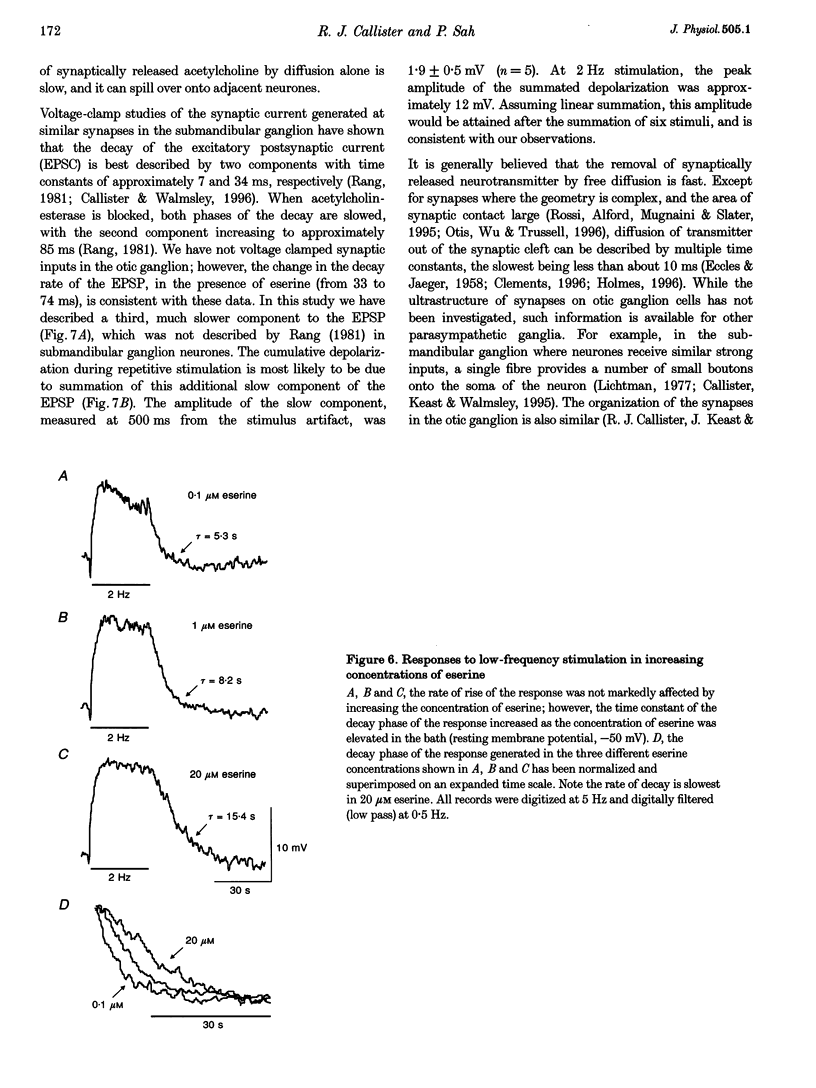
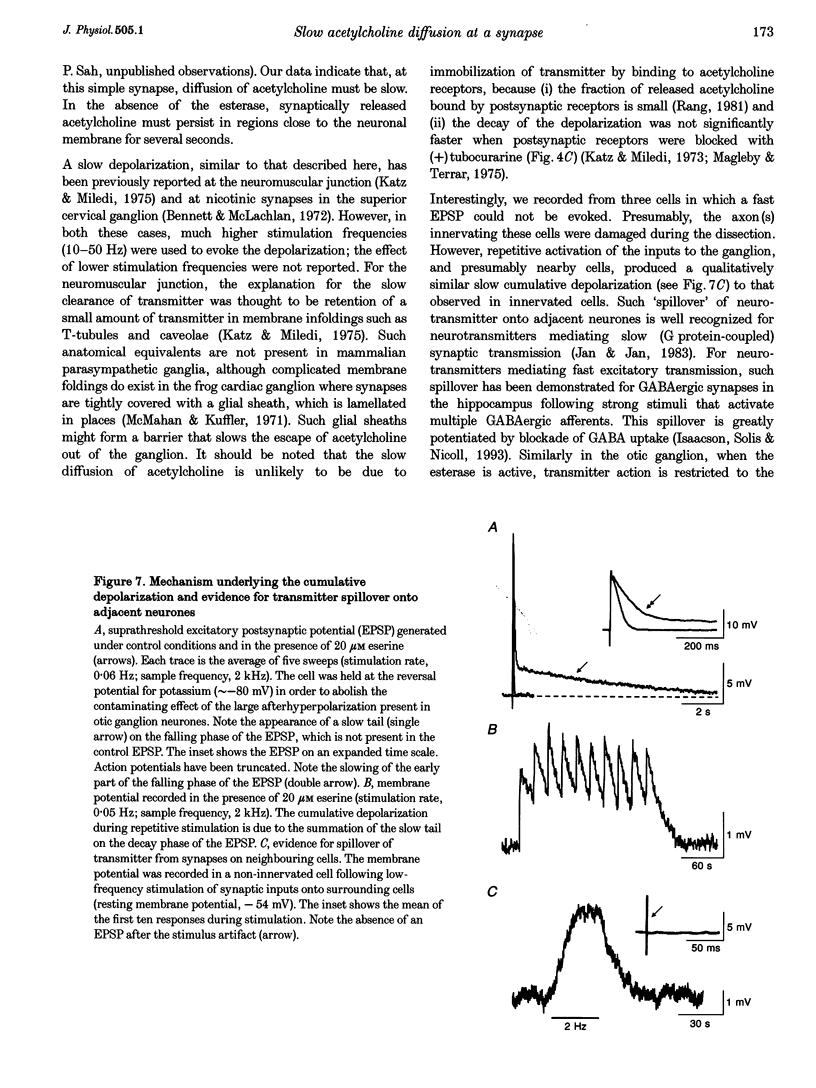
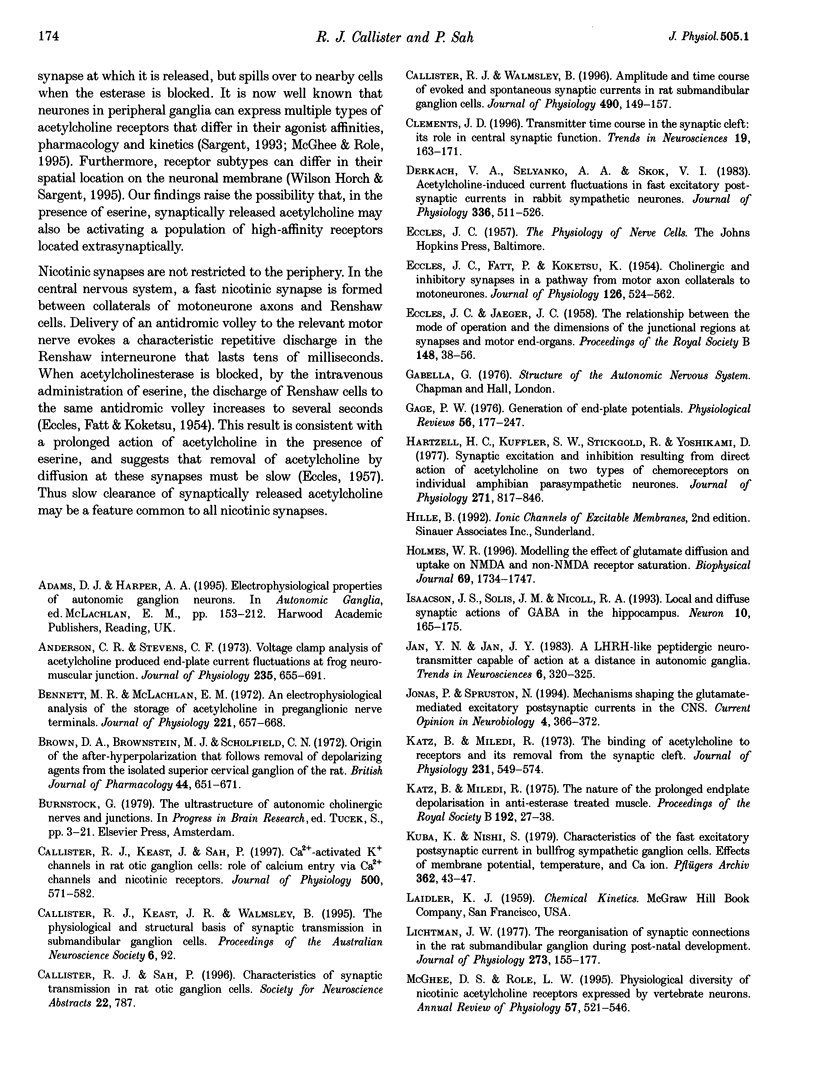
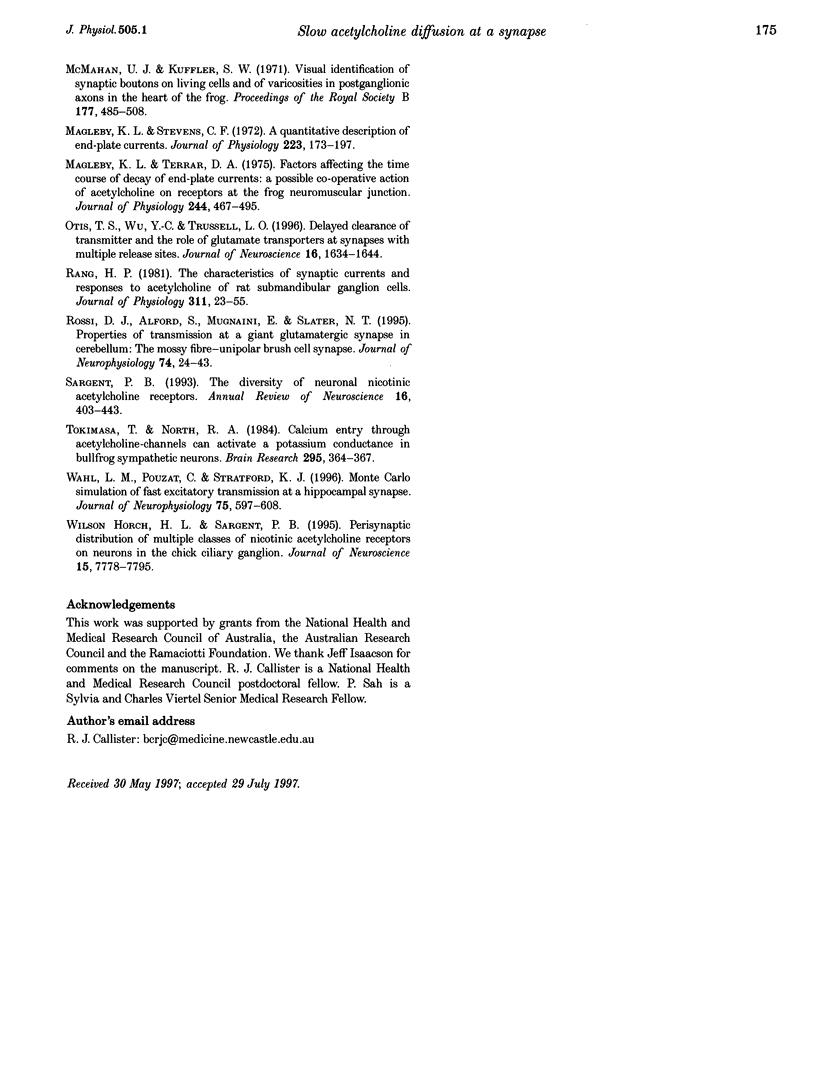
Selected References
These references are in PubMed. This may not be the complete list of references from this article.
- Anderson C. R., Stevens C. F. Voltage clamp analysis of acetylcholine produced end-plate current fluctuations at frog neuromuscular junction. J Physiol. 1973 Dec;235(3):655–691. doi: 10.1113/jphysiol.1973.sp010410. [DOI] [PMC free article] [PubMed] [Google Scholar]
- Bennett M. R., McLachlan E. M. An electrophysiological analysis of the storage of acetylcholine in preganglionic nerve terminals. J Physiol. 1972 Mar;221(3):657–668. doi: 10.1113/jphysiol.1972.sp009774. [DOI] [PMC free article] [PubMed] [Google Scholar]
- Brown D. A., Brownstein M. J., Scholfield C. N. Origin of the after-hyperpolarization that follows removal of depolarizing agents from the isolated superior cervical ganglion of the rat. Br J Pharmacol. 1972 Apr;44(4):651–671. doi: 10.1111/j.1476-5381.1972.tb07305.x. [DOI] [PMC free article] [PubMed] [Google Scholar]
- Burnstock G. The ultrastructure of autonomic cholinergic nerves and junctions. Prog Brain Res. 1979;49:3–21. doi: 10.1016/S0079-6123(08)64618-X. [DOI] [PubMed] [Google Scholar]
- Callister R. J., Keast J. R., Sah P. Ca(2+)-activated K+ channels in rat otic ganglion cells: role of Ca2+ entry via Ca2+ channels and nicotinic receptors. J Physiol. 1997 May 1;500(Pt 3):571–582. doi: 10.1113/jphysiol.1997.sp022043. [DOI] [PMC free article] [PubMed] [Google Scholar]
- Callister R. J., Walmsley B. Amplitude and time course of evoked and spontaneous synaptic currents in rat submandibular ganglion cells. J Physiol. 1996 Jan 1;490(Pt 1):149–157. doi: 10.1113/jphysiol.1996.sp021132. [DOI] [PMC free article] [PubMed] [Google Scholar]
- Clements J. D. Transmitter timecourse in the synaptic cleft: its role in central synaptic function. Trends Neurosci. 1996 May;19(5):163–171. doi: 10.1016/s0166-2236(96)10024-2. [DOI] [PubMed] [Google Scholar]
- Derkach V. A., Selyanko A. A., Skok V. I. Acetylcholine-induced current fluctuations and fast excitatory post-synaptic currents in rabbit sympathetic neurones. J Physiol. 1983 Mar;336:511–526. doi: 10.1113/jphysiol.1983.sp014595. [DOI] [PMC free article] [PubMed] [Google Scholar]
- ECCLES J. C., FATT P., KOKETSU K. Cholinergic and inhibitory synapses in a pathway from motor-axon collaterals to motoneurones. J Physiol. 1954 Dec 10;126(3):524–562. doi: 10.1113/jphysiol.1954.sp005226. [DOI] [PMC free article] [PubMed] [Google Scholar]
- ECCLES J. C., JAEGER J. C. The relationship between the mode of operation and the dimensions of the junctional regions at synapses and motor end-organs. Proc R Soc Lond B Biol Sci. 1958 Jan 1;148(930):38–56. doi: 10.1098/rspb.1958.0003. [DOI] [PubMed] [Google Scholar]
- Gage P. W. Generation of end-plate potentials. Physiol Rev. 1976 Jan;56(1):177–247. doi: 10.1152/physrev.1976.56.1.177. [DOI] [PubMed] [Google Scholar]
- Hartzell H. C., Kuffler S. W., Stickgold R., Yoshikami D. Synaptic excitation and inhibition resulting from direct action of acetylcholine on two types of chemoreceptors on individual amphibian parasympathetic neurones. J Physiol. 1977 Oct;271(3):817–846. doi: 10.1113/jphysiol.1977.sp012027. [DOI] [PMC free article] [PubMed] [Google Scholar]
- Holmes W. R. Modeling the effect of glutamate diffusion and uptake on NMDA and non-NMDA receptor saturation. Biophys J. 1995 Nov;69(5):1734–1747. doi: 10.1016/S0006-3495(95)80043-3. [DOI] [PMC free article] [PubMed] [Google Scholar]
- Horch H. L., Sargent P. B. Perisynaptic surface distribution of multiple classes of nicotinic acetylcholine receptors on neurons in the chicken ciliary ganglion. J Neurosci. 1995 Dec;15(12):7778–7795. doi: 10.1523/JNEUROSCI.15-12-07778.1995. [DOI] [PMC free article] [PubMed] [Google Scholar]
- Isaacson J. S., Solís J. M., Nicoll R. A. Local and diffuse synaptic actions of GABA in the hippocampus. Neuron. 1993 Feb;10(2):165–175. doi: 10.1016/0896-6273(93)90308-e. [DOI] [PubMed] [Google Scholar]
- Jonas P., Spruston N. Mechanisms shaping glutamate-mediated excitatory postsynaptic currents in the CNS. Curr Opin Neurobiol. 1994 Jun;4(3):366–372. doi: 10.1016/0959-4388(94)90098-1. [DOI] [PubMed] [Google Scholar]
- Katz B., Miledi R. The binding of acetylcholine to receptors and its removal from the synaptic cleft. J Physiol. 1973 Jun;231(3):549–574. doi: 10.1113/jphysiol.1973.sp010248. [DOI] [PMC free article] [PubMed] [Google Scholar]
- Katz B., Miledi R. The nature of the prolonged endplate depolarization in anti-esterase treated muscle. Proc R Soc Lond B Biol Sci. 1975 Dec 31;192(1106):27–38. doi: 10.1098/rspb.1975.0149. [DOI] [PubMed] [Google Scholar]
- Lichtman J. W. The reorganization of synaptic connexions in the rat submandibular ganglion during post-natal development. J Physiol. 1977 Dec;273(1):155–177. doi: 10.1113/jphysiol.1977.sp012087. [DOI] [PMC free article] [PubMed] [Google Scholar]
- Magleby K. L., Stevens C. F. A quantitative description of end-plate currents. J Physiol. 1972 May;223(1):173–197. doi: 10.1113/jphysiol.1972.sp009840. [DOI] [PMC free article] [PubMed] [Google Scholar]
- Magleby K. L., Terrar D. A. Factors affecting the time course of decay of end-plate currents: a possible cooperative action of acetylcholine on receptors at the frog neuromuscular junction. J Physiol. 1975 Jan;244(2):467–495. doi: 10.1113/jphysiol.1975.sp010808. [DOI] [PMC free article] [PubMed] [Google Scholar]
- McGehee D. S., Role L. W. Physiological diversity of nicotinic acetylcholine receptors expressed by vertebrate neurons. Annu Rev Physiol. 1995;57:521–546. doi: 10.1146/annurev.ph.57.030195.002513. [DOI] [PubMed] [Google Scholar]
- McMahan U. J., Kuffler S. W. Visual identification of synaptic boutons on living ganglion cells and of varicosities in postganglionic axons in the heart of the frog. Proc R Soc Lond B Biol Sci. 1971 Apr 27;177(1049):485–508. doi: 10.1098/rspb.1971.0044. [DOI] [PubMed] [Google Scholar]
- Otis T. S., Wu Y. C., Trussell L. O. Delayed clearance of transmitter and the role of glutamate transporters at synapses with multiple release sites. J Neurosci. 1996 Mar 1;16(5):1634–1644. doi: 10.1523/JNEUROSCI.16-05-01634.1996. [DOI] [PMC free article] [PubMed] [Google Scholar]
- Rang H. P. The characteristics of synaptic currents and responses to acetylcholine of rat submandibular ganglion cells. J Physiol. 1981 Feb;311:23–55. doi: 10.1113/jphysiol.1981.sp013571. [DOI] [PMC free article] [PubMed] [Google Scholar]
- Rossi D. J., Alford S., Mugnaini E., Slater N. T. Properties of transmission at a giant glutamatergic synapse in cerebellum: the mossy fiber-unipolar brush cell synapse. J Neurophysiol. 1995 Jul;74(1):24–42. doi: 10.1152/jn.1995.74.1.24. [DOI] [PubMed] [Google Scholar]
- Sargent P. B. The diversity of neuronal nicotinic acetylcholine receptors. Annu Rev Neurosci. 1993;16:403–443. doi: 10.1146/annurev.ne.16.030193.002155. [DOI] [PubMed] [Google Scholar]
- Tokimasa T., North R. A. Calcium entry through acetylcholine-channels can activate potassium conductance in bullfrog sympathetic neurons. Brain Res. 1984 Mar 19;295(2):364–367. doi: 10.1016/0006-8993(84)90987-9. [DOI] [PubMed] [Google Scholar]
- Wahl L. M., Pouzat C., Stratford K. J. Monte Carlo simulation of fast excitatory synaptic transmission at a hippocampal synapse. J Neurophysiol. 1996 Feb;75(2):597–608. doi: 10.1152/jn.1996.75.2.597. [DOI] [PubMed] [Google Scholar]