Abstract
1. Purkinje cells (PCs) from guinea-pig cerebellar slices were physiologically characterized using intracellular techniques. Extracellular caesium ions were used to linearize the membrane properties of PCs near the resting potential. Under these conditions the average input resistance, RN, was 29 M omega, the average system time constant, tau 0, was 82 ms and the average cable length, LN, was 0.59. 2. Three PCs were fully reconstructed following physiological measurements and staining with horseradish peroxidase. Assuming that each spine has an area of 1 micron 2 and that the spine density over the spiny dendrites is ten spines per micrometre length, the total membrane area of each PC is approximately 150,000 microns 2, of which approximately 100,000 microns 2 is in the spines. 3. Detailed passive cable and compartmental models were built for each of the three reconstructed PCs. Computational methods were devised to incorporate globally the huge number of spines into these models. In all three cells the models predict that the specific membrane resistivity, Rm, of the soma is much lower than the dendritic Rm (approximately 500 and approximately 100,000 omega cm2 respectively). The specific membrane capacitance, Cm, is estimated to be 1.5-2 muF cm-2 and the specific cytoplasm resistivity, Ri, is 250 omega cm. 4. The average cable length of the dendrites according to the model is 0.13 lambda, suggesting that under caesium conditions PCs are electrically very compact. Brief somatic spikes, however, are expected to attenuate 30-fold when spreading passively into the dendritic terminals. A simulated 200 Hz train of fast, 90 mV somatic spikes produced a smooth 12 mV steady depolarization at the dendritic terminals. 5. A transient synaptic conductance increase, with a 1 nS peak at 0.5 ms and a driving force of 60 mV, is expected to produce approximately 20 mV peak depolarization at the spine head membrane. This EPSP then attenuates between 200- and 900-fold into the soma. Approximately 800 randomly distributed and synchronously activated spiny inputs are required to fire the soma. 6. The passive model of the PC predicts a poor resolution of the spatio-temporal pattern of the parallel fibre input. An equally sized, randomly distributed group of approximately 1% of the parallel fibres, activated within a time window of a few milliseconds, would result in approximately the same composite EPSP at the soma.
Full text
PDF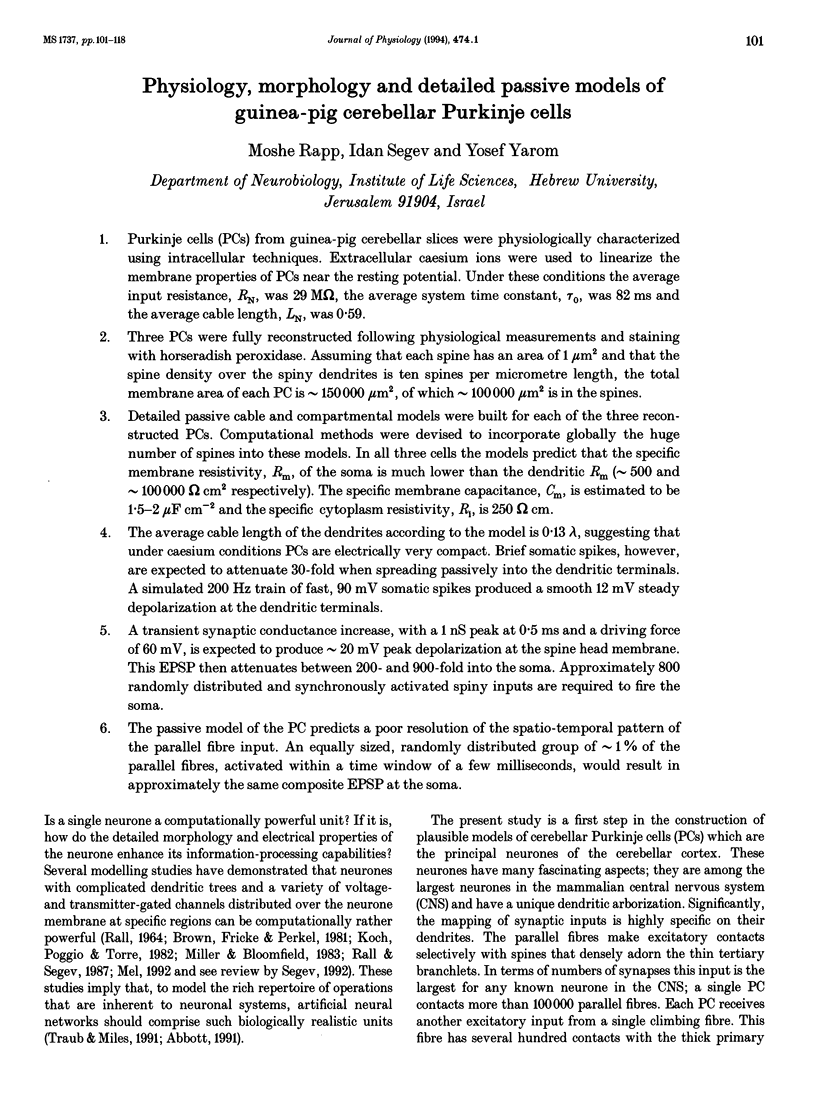
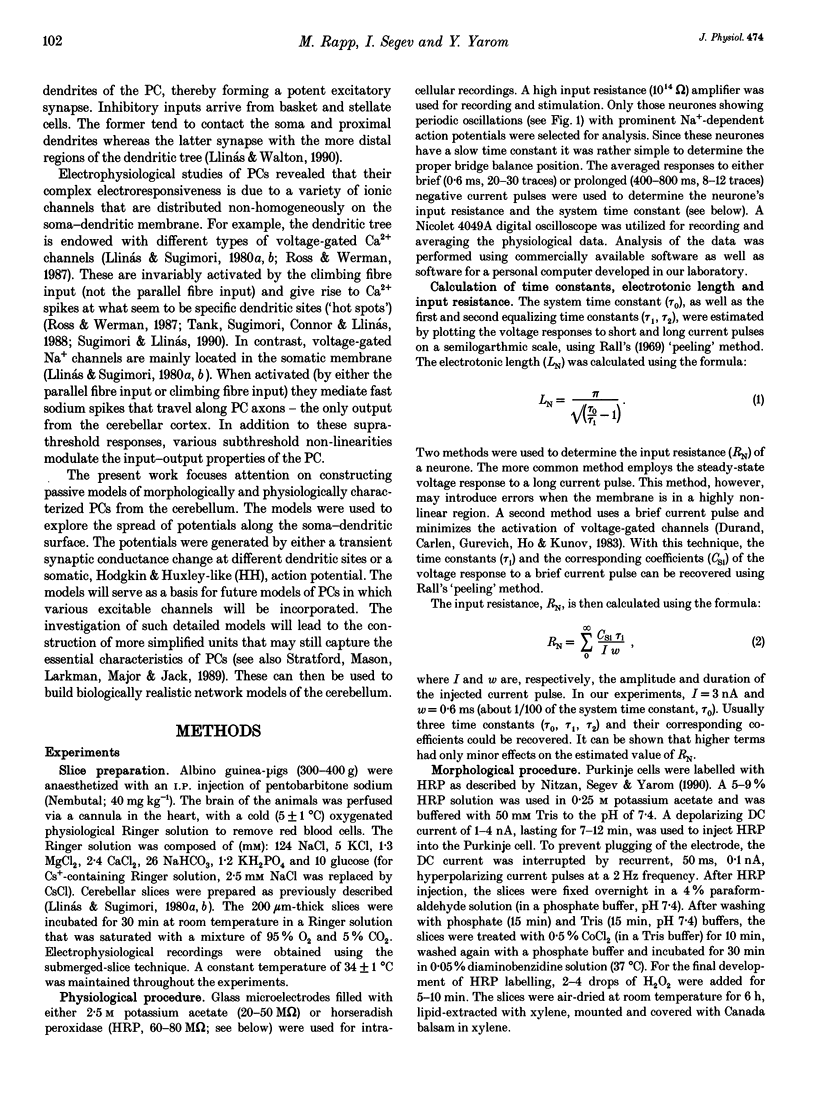
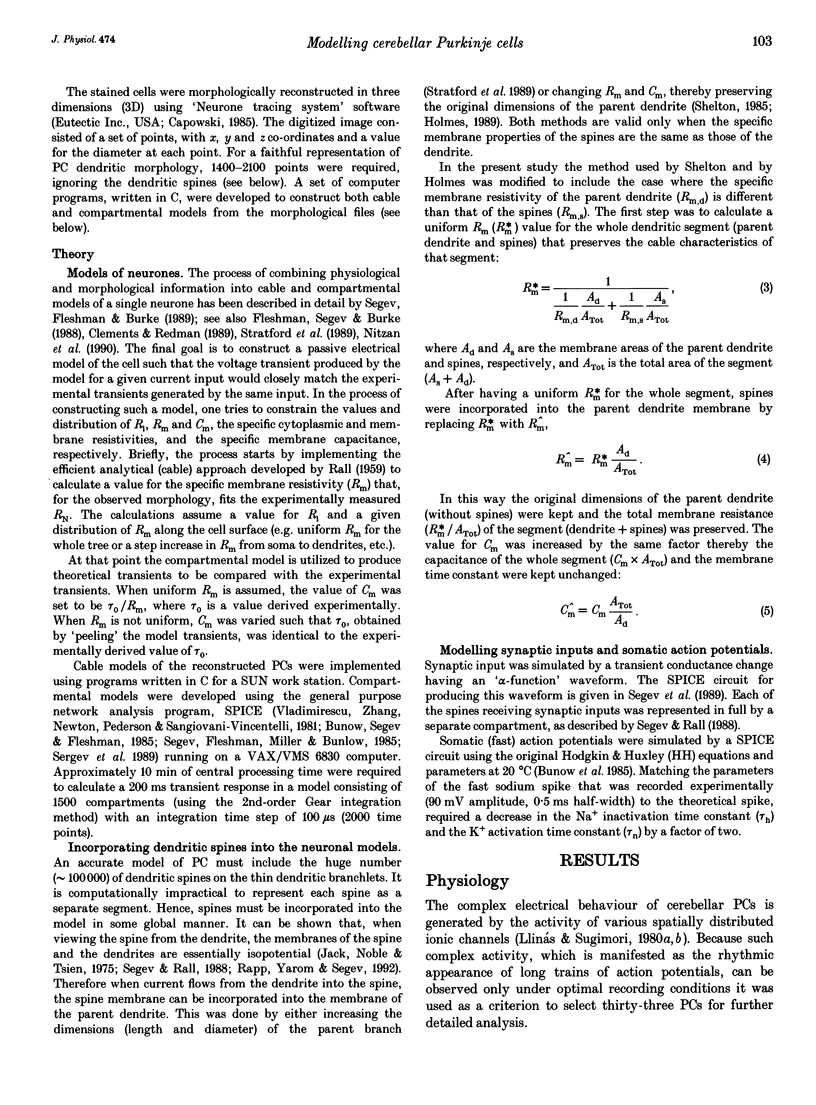
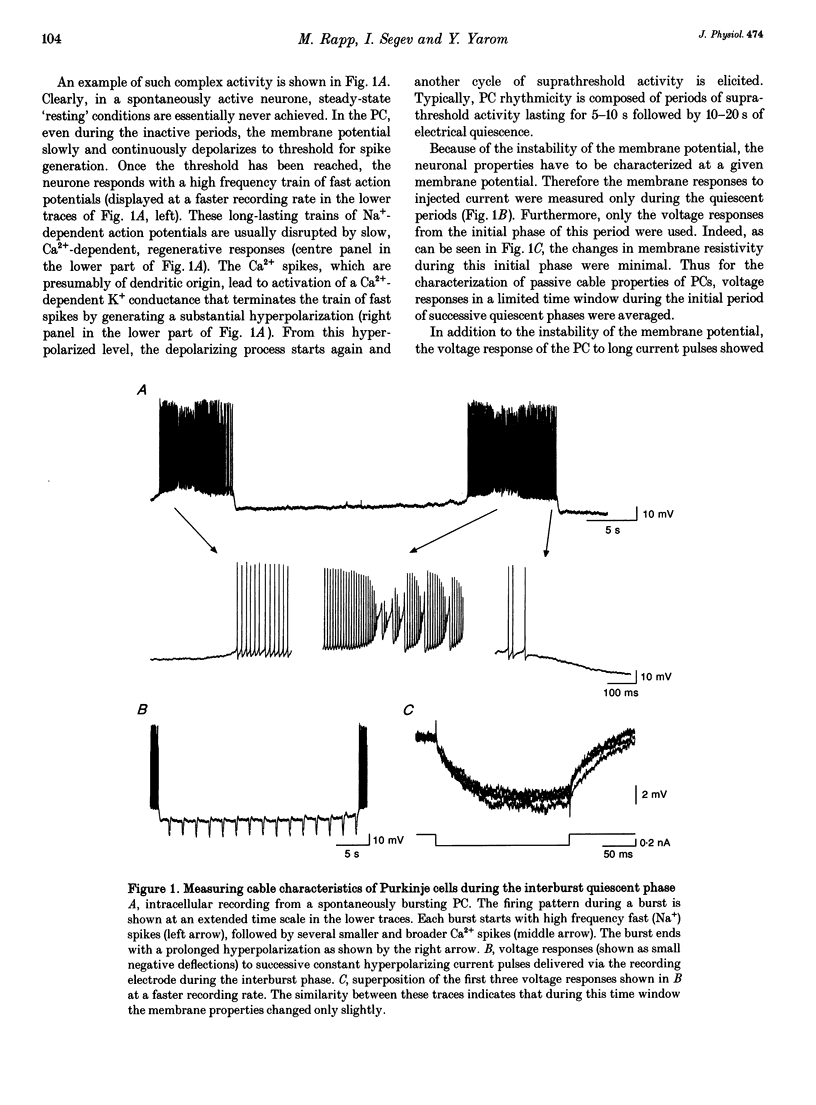
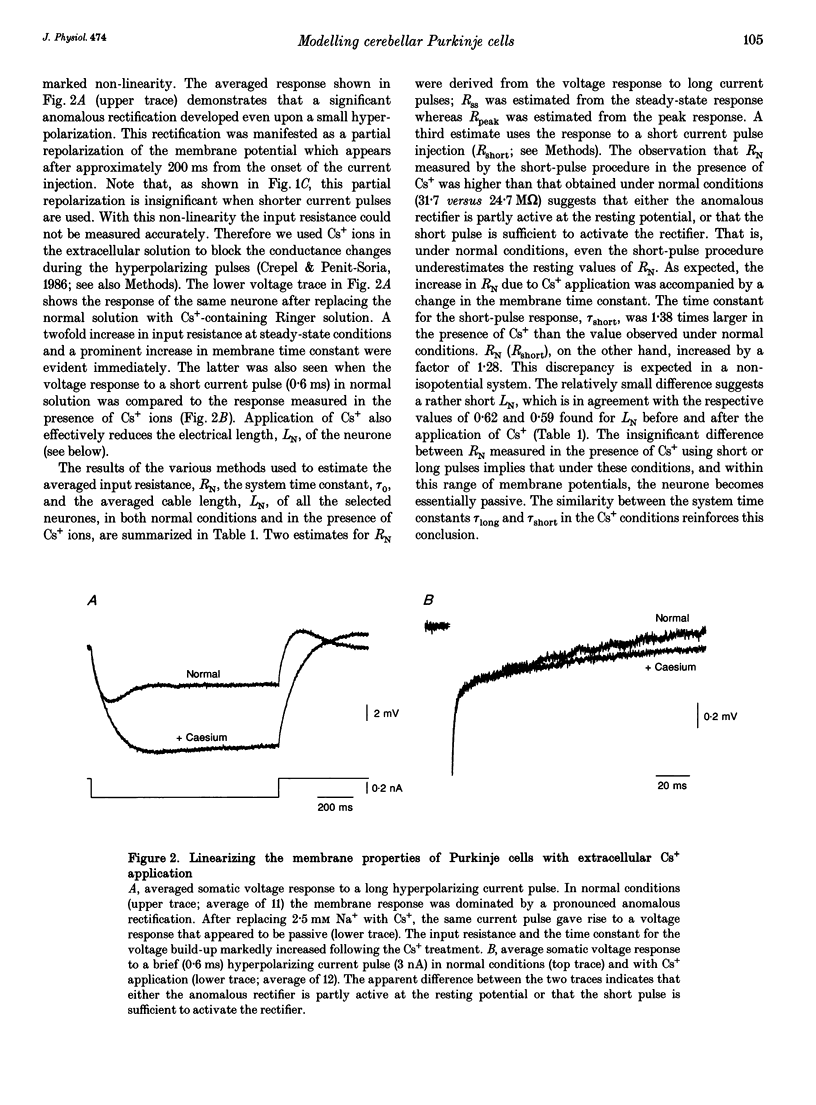
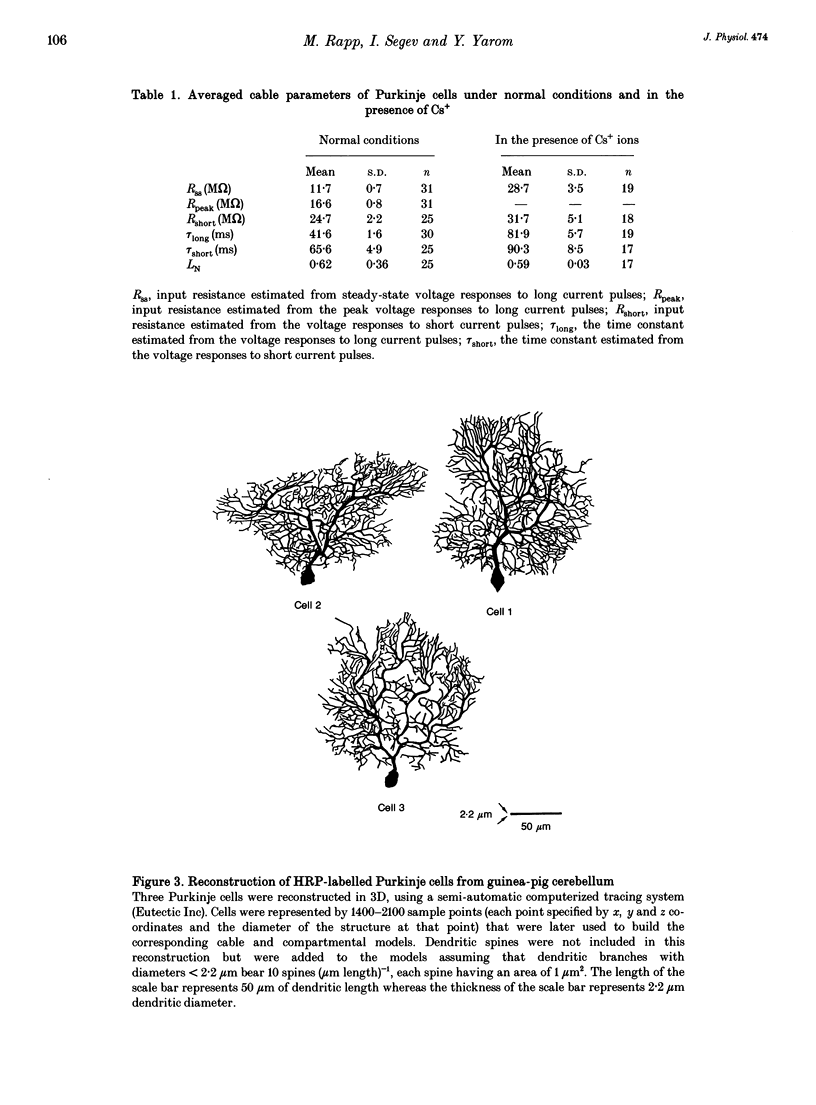
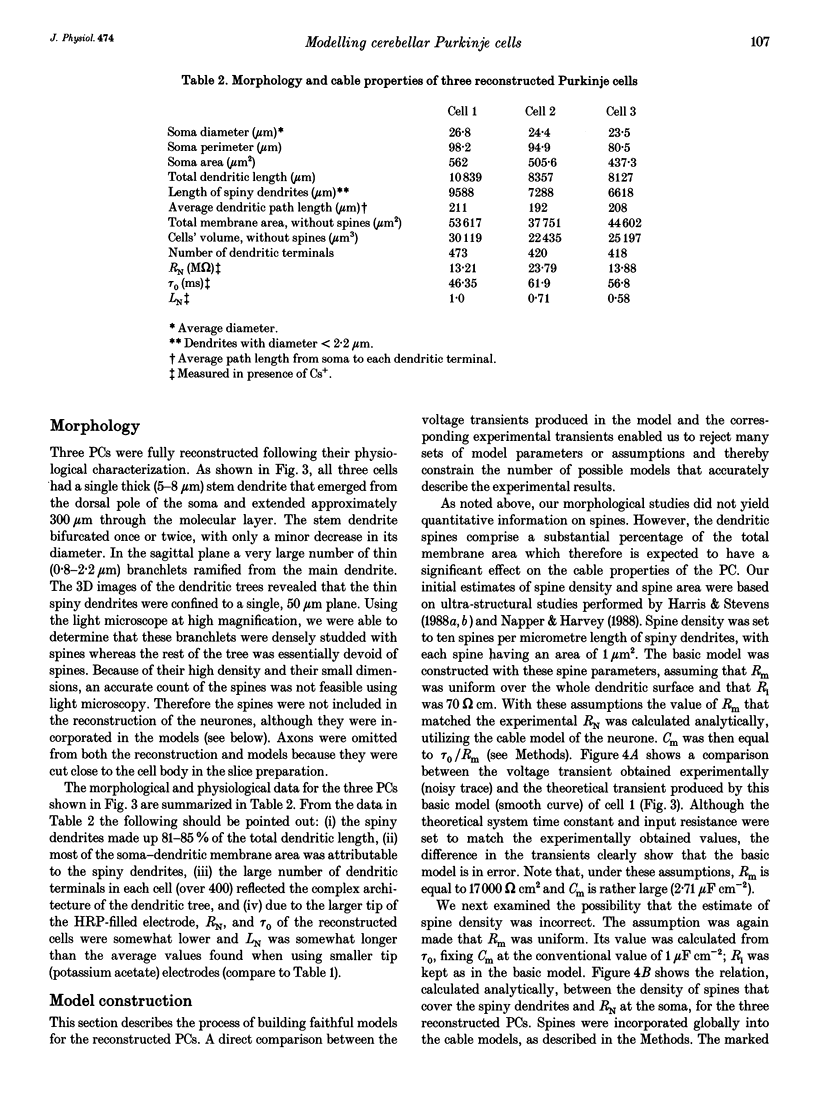
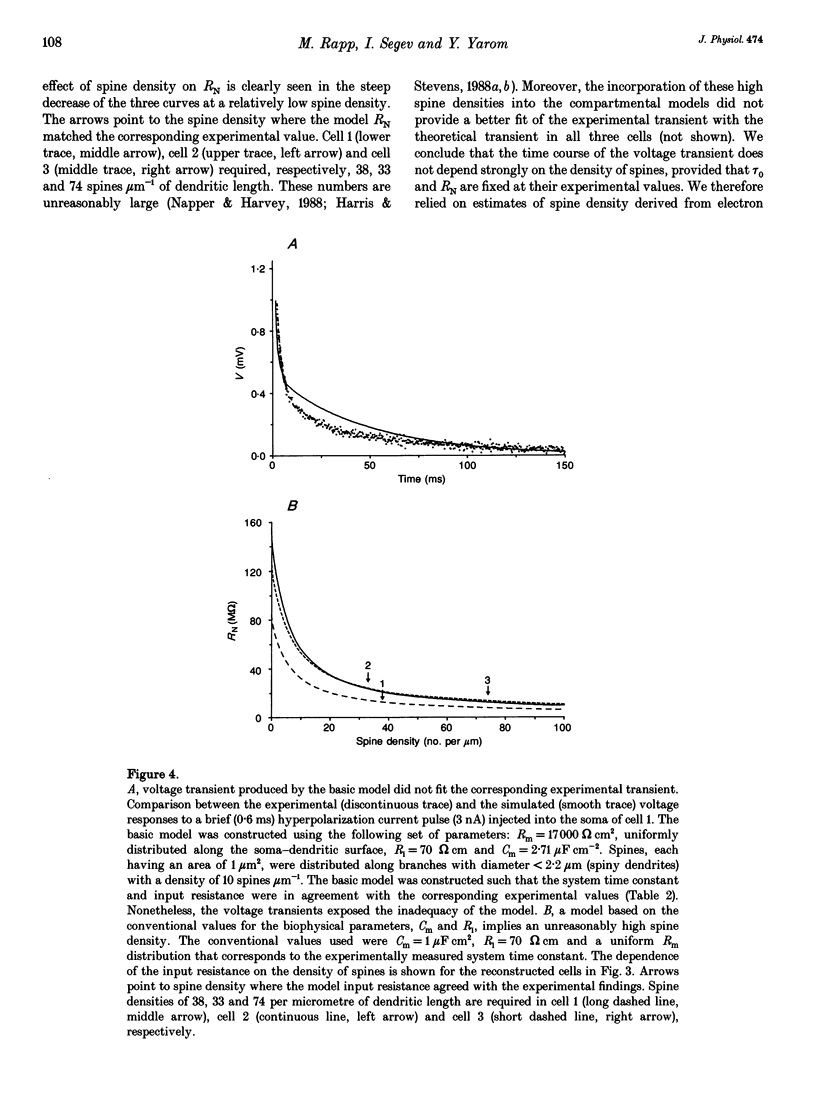
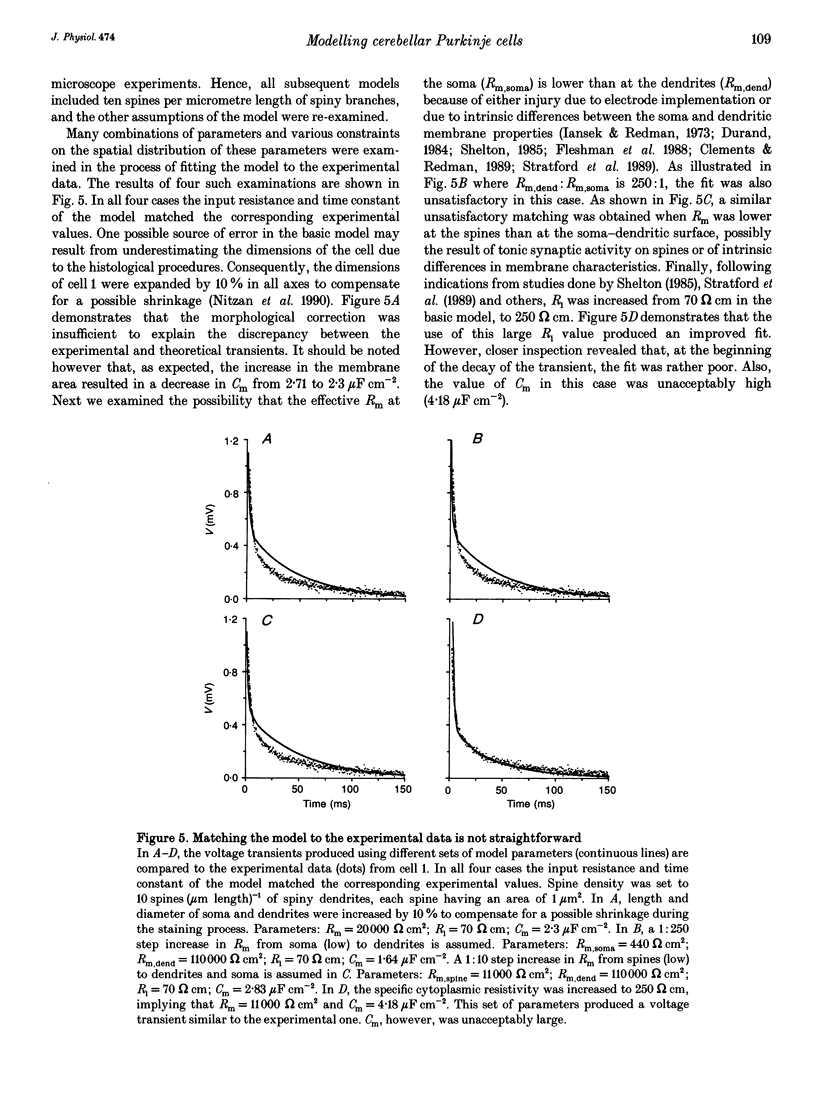
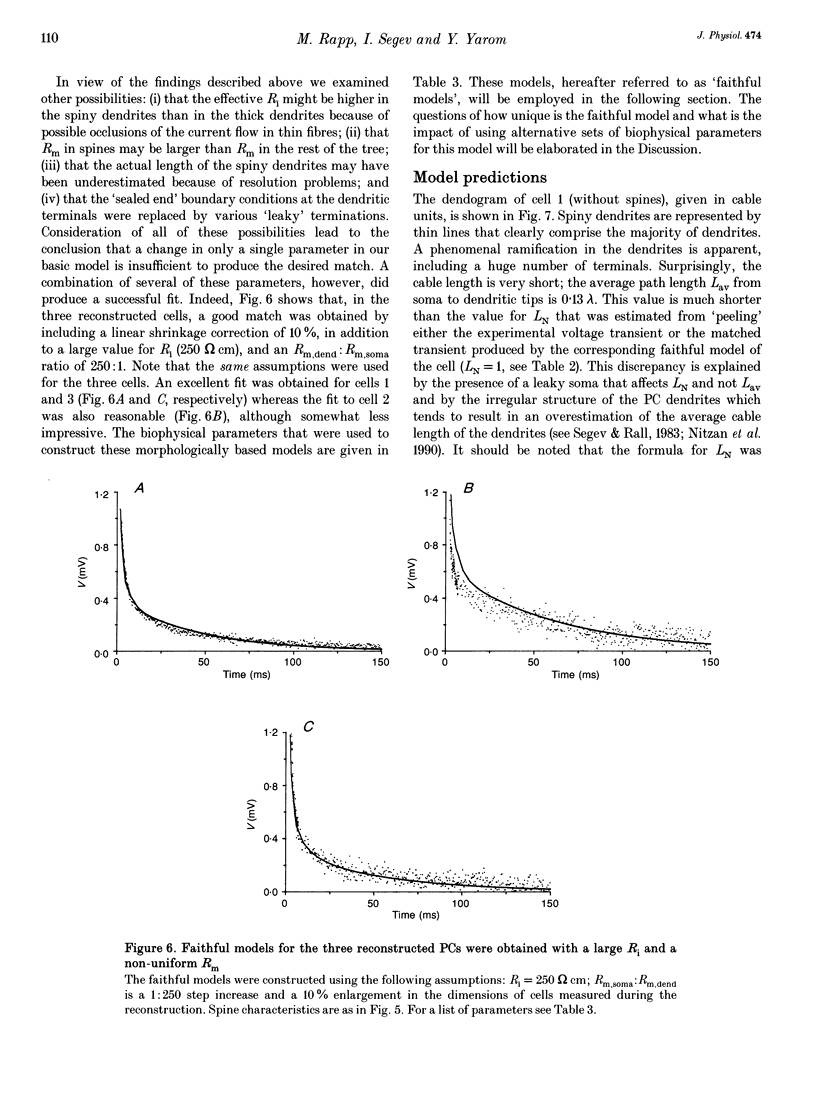
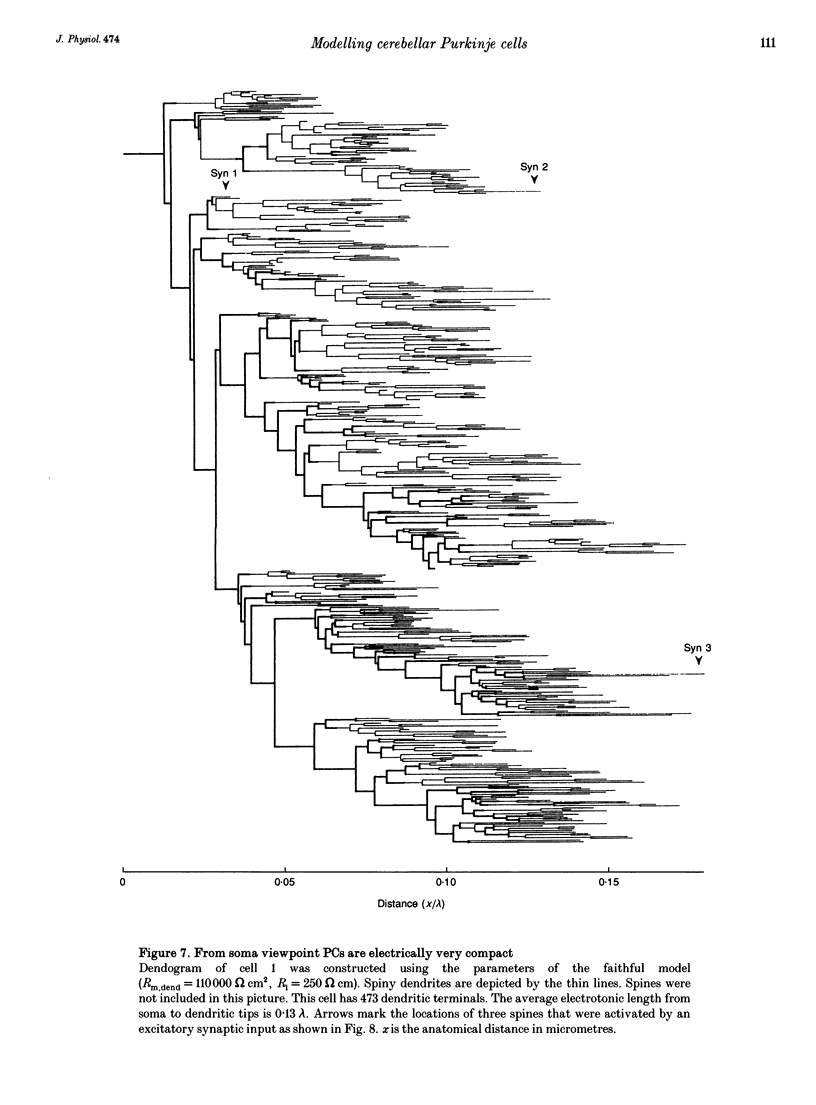
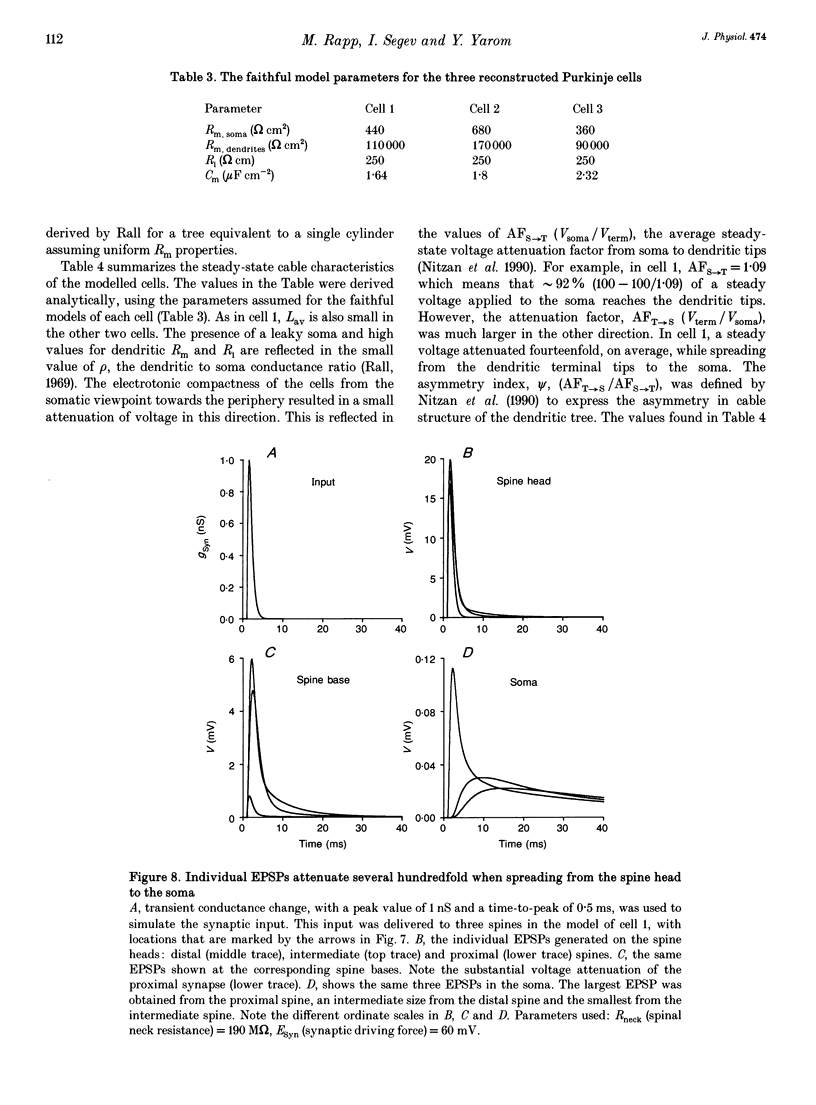
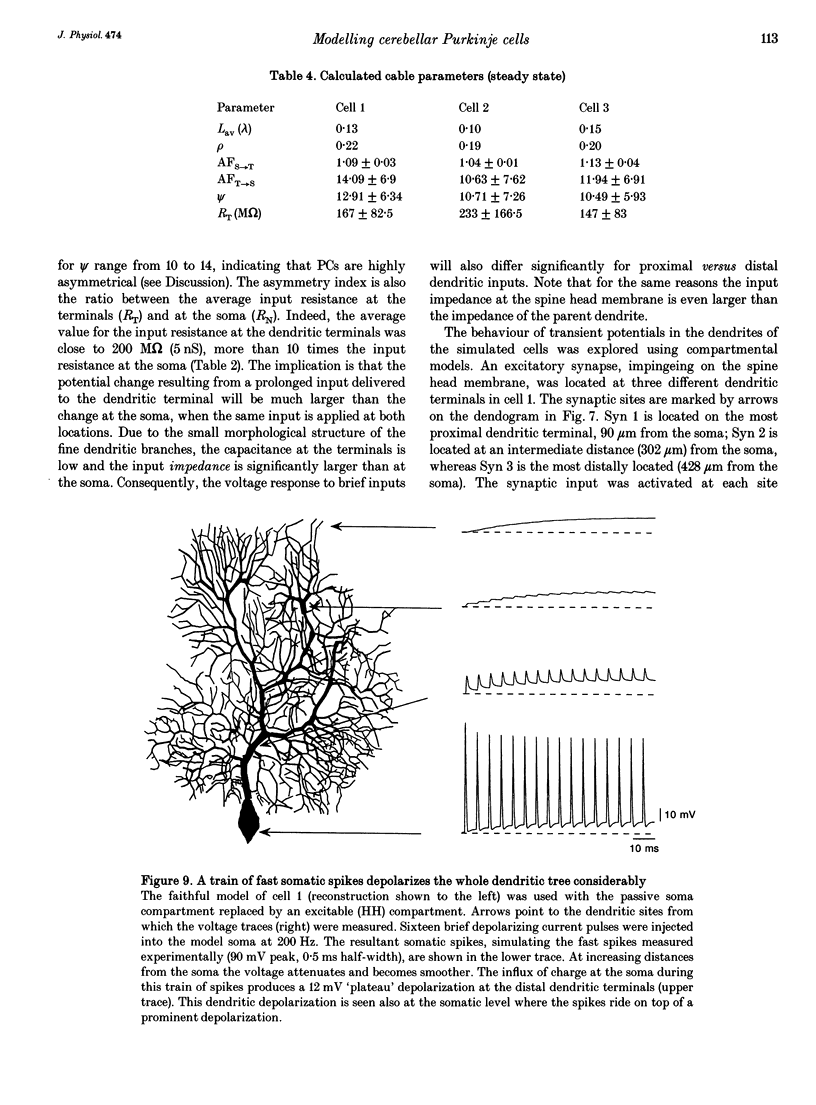
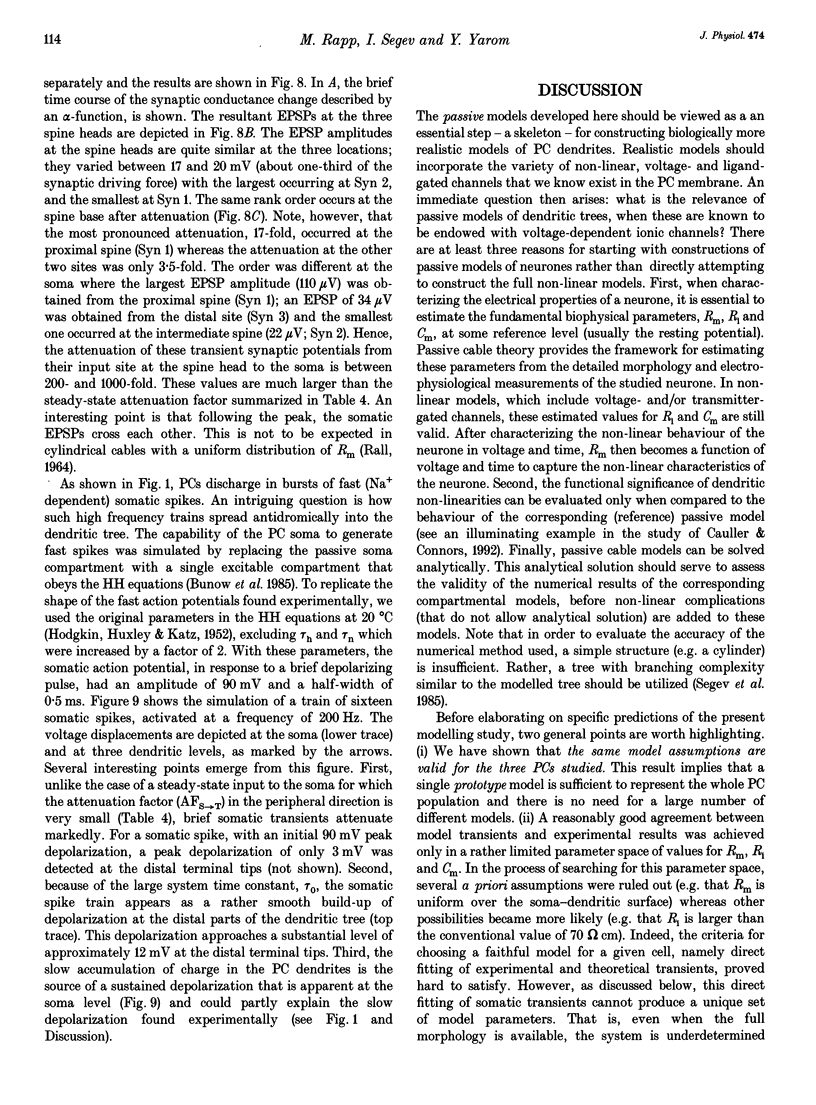
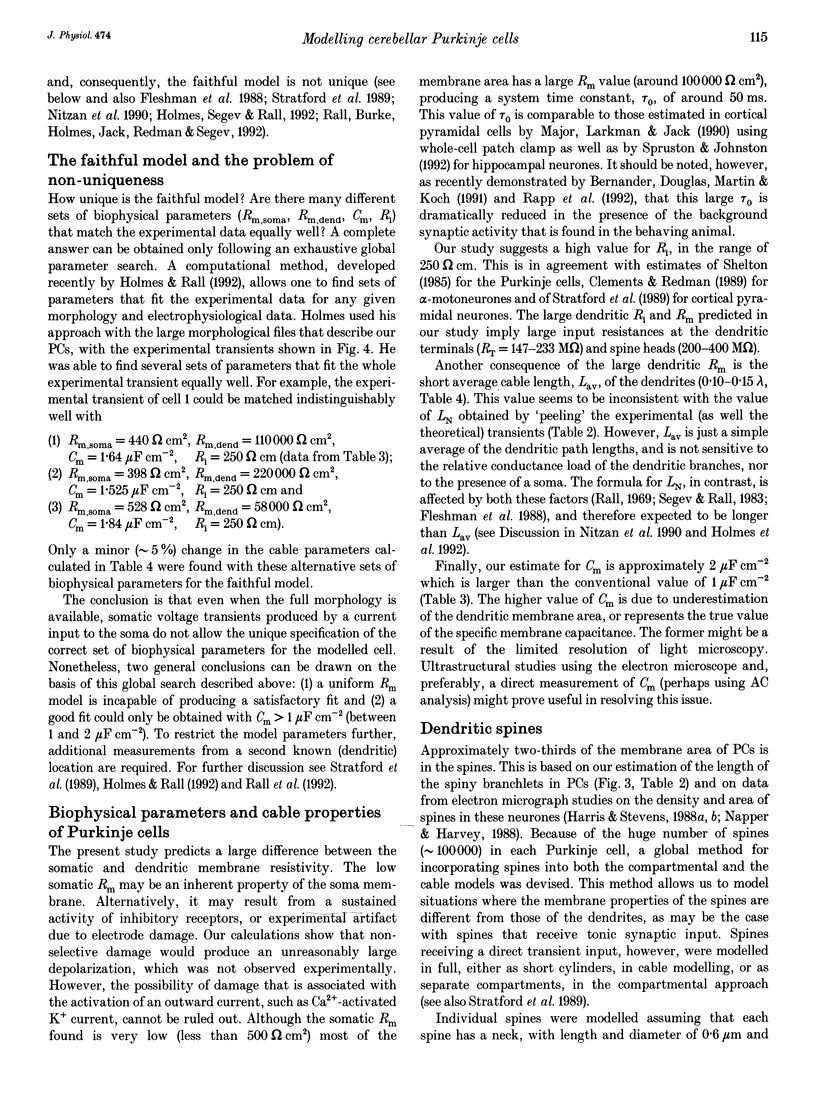
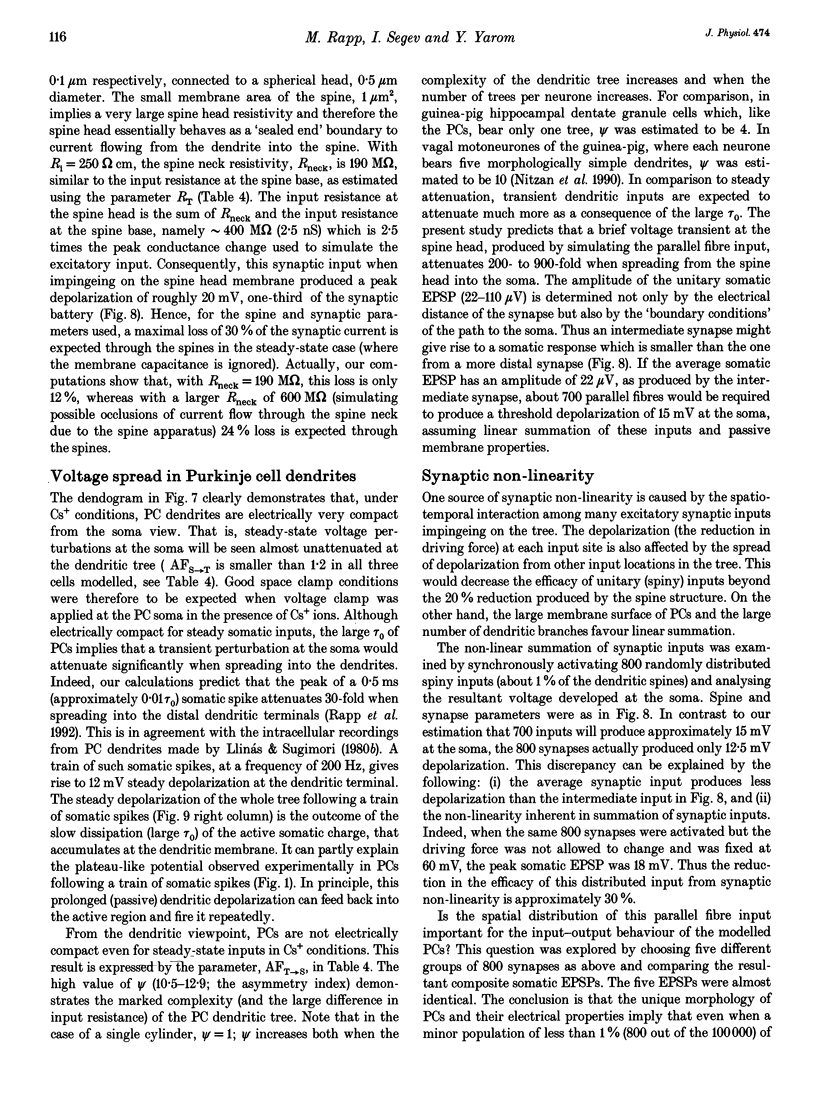
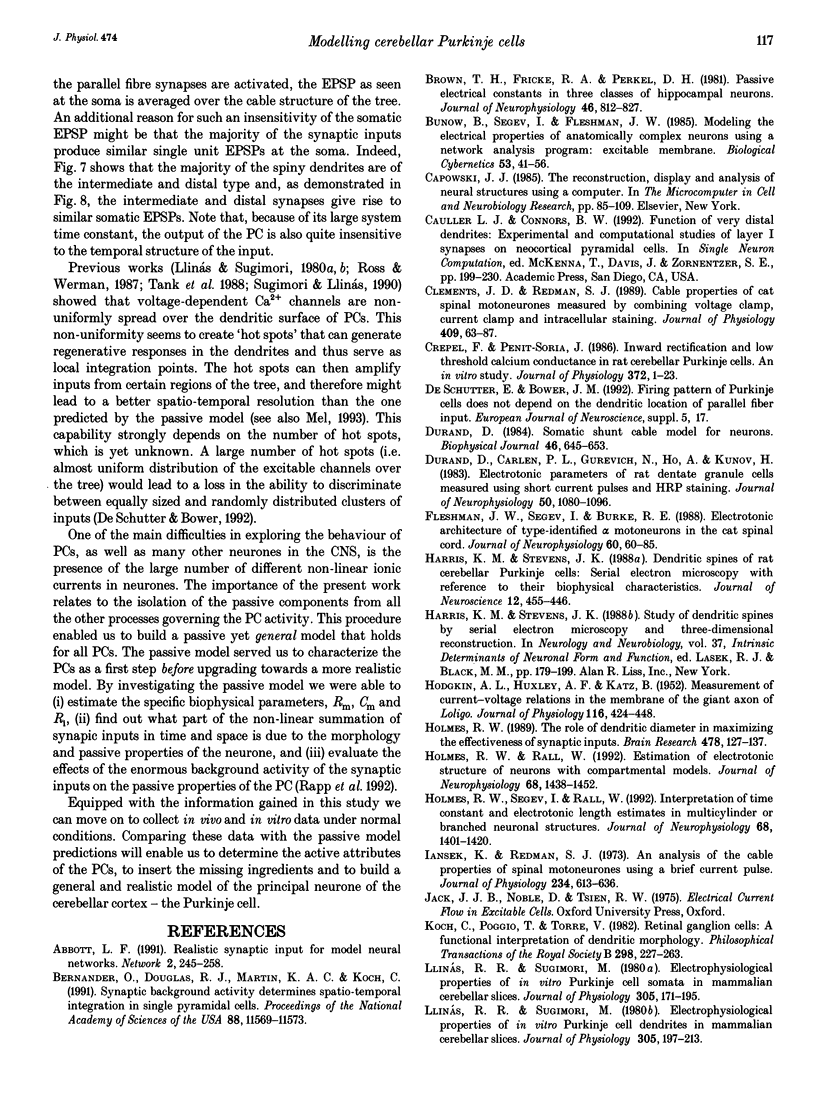
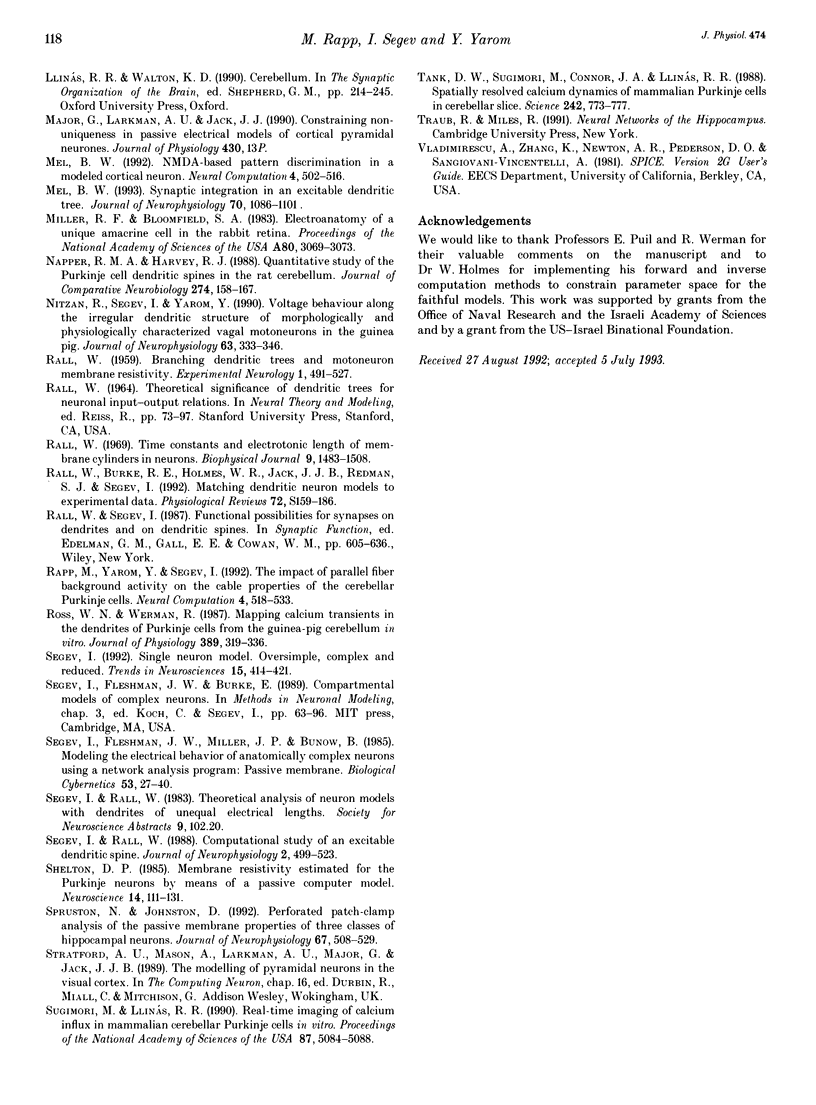
Selected References
These references are in PubMed. This may not be the complete list of references from this article.
- Bernander O., Douglas R. J., Martin K. A., Koch C. Synaptic background activity influences spatiotemporal integration in single pyramidal cells. Proc Natl Acad Sci U S A. 1991 Dec 15;88(24):11569–11573. doi: 10.1073/pnas.88.24.11569. [DOI] [PMC free article] [PubMed] [Google Scholar]
- Brown T. H., Fricke R. A., Perkel D. H. Passive electrical constants in three classes of hippocampal neurons. J Neurophysiol. 1981 Oct;46(4):812–827. doi: 10.1152/jn.1981.46.4.812. [DOI] [PubMed] [Google Scholar]
- Bunow B., Segev I., Fleshman J. W. Modeling the electrical behavior of anatomically complex neurons using a network analysis program: excitable membrane. Biol Cybern. 1985;53(1):41–56. doi: 10.1007/BF00355689. [DOI] [PubMed] [Google Scholar]
- Clements J. D., Redman S. J. Cable properties of cat spinal motoneurones measured by combining voltage clamp, current clamp and intracellular staining. J Physiol. 1989 Feb;409:63–87. doi: 10.1113/jphysiol.1989.sp017485. [DOI] [PMC free article] [PubMed] [Google Scholar]
- Crepel F., Penit-Soria J. Inward rectification and low threshold calcium conductance in rat cerebellar Purkinje cells. An in vitro study. J Physiol. 1986 Mar;372:1–23. doi: 10.1113/jphysiol.1986.sp015993. [DOI] [PMC free article] [PubMed] [Google Scholar]
- Durand D., Carlen P. L., Gurevich N., Ho A., Kunov H. Electrotonic parameters of rat dentate granule cells measured using short current pulses and HRP staining. J Neurophysiol. 1983 Nov;50(5):1080–1097. doi: 10.1152/jn.1983.50.5.1080. [DOI] [PubMed] [Google Scholar]
- Durand D. The somatic shunt cable model for neurons. Biophys J. 1984 Nov;46(5):645–653. doi: 10.1016/S0006-3495(84)84063-1. [DOI] [PMC free article] [PubMed] [Google Scholar]
- Fleshman J. W., Segev I., Burke R. B. Electrotonic architecture of type-identified alpha-motoneurons in the cat spinal cord. J Neurophysiol. 1988 Jul;60(1):60–85. doi: 10.1152/jn.1988.60.1.60. [DOI] [PubMed] [Google Scholar]
- HODGKIN A. L., HUXLEY A. F., KATZ B. Measurement of current-voltage relations in the membrane of the giant axon of Loligo. J Physiol. 1952 Apr;116(4):424–448. doi: 10.1113/jphysiol.1952.sp004716. [DOI] [PMC free article] [PubMed] [Google Scholar]
- Holmes W. R., Rall W. Estimating the electrotonic structure of neurons with compartmental models. J Neurophysiol. 1992 Oct;68(4):1438–1452. doi: 10.1152/jn.1992.68.4.1438. [DOI] [PubMed] [Google Scholar]
- Holmes W. R., Segev I., Rall W. Interpretation of time constant and electrotonic length estimates in multicylinder or branched neuronal structures. J Neurophysiol. 1992 Oct;68(4):1401–1420. doi: 10.1152/jn.1992.68.4.1401. [DOI] [PubMed] [Google Scholar]
- Holmes W. R. The role of dendritic diameters in maximizing the effectiveness of synaptic inputs. Brain Res. 1989 Jan 23;478(1):127–137. doi: 10.1016/0006-8993(89)91484-4. [DOI] [PubMed] [Google Scholar]
- Hurst A. M., Hunter M. Stretch-activated channels in single early distal tubule cells of the frog. J Physiol. 1990 Nov;430:13–24. doi: 10.1113/jphysiol.1990.sp018278. [DOI] [PMC free article] [PubMed] [Google Scholar]
- Iansek R., Redman S. J. An analysis of the cable properties of spinal motoneurones using a brief intracellular current pulse. J Physiol. 1973 Nov;234(3):613–636. doi: 10.1113/jphysiol.1973.sp010364. [DOI] [PMC free article] [PubMed] [Google Scholar]
- Koch C., Poggio T., Torre V. Retinal ganglion cells: a functional interpretation of dendritic morphology. Philos Trans R Soc Lond B Biol Sci. 1982 Jul 27;298(1090):227–263. doi: 10.1098/rstb.1982.0084. [DOI] [PubMed] [Google Scholar]
- Llinás R., Sugimori M. Electrophysiological properties of in vitro Purkinje cell dendrites in mammalian cerebellar slices. J Physiol. 1980 Aug;305:197–213. doi: 10.1113/jphysiol.1980.sp013358. [DOI] [PMC free article] [PubMed] [Google Scholar]
- Llinás R., Sugimori M. Electrophysiological properties of in vitro Purkinje cell somata in mammalian cerebellar slices. J Physiol. 1980 Aug;305:171–195. doi: 10.1113/jphysiol.1980.sp013357. [DOI] [PMC free article] [PubMed] [Google Scholar]
- Mel B. W. Synaptic integration in an excitable dendritic tree. J Neurophysiol. 1993 Sep;70(3):1086–1101. doi: 10.1152/jn.1993.70.3.1086. [DOI] [PubMed] [Google Scholar]
- Miller R. F., Bloomfield S. A. Electroanatomy of a unique amacrine cell in the rabbit retina. Proc Natl Acad Sci U S A. 1983 May;80(10):3069–3073. doi: 10.1073/pnas.80.10.3069. [DOI] [PMC free article] [PubMed] [Google Scholar]
- Napper R. M., Harvey R. J. Quantitative study of the Purkinje cell dendritic spines in the rat cerebellum. J Comp Neurol. 1988 Aug 8;274(2):158–167. doi: 10.1002/cne.902740203. [DOI] [PubMed] [Google Scholar]
- Nitzan R., Segev I., Yarom Y. Voltage behavior along the irregular dendritic structure of morphologically and physiologically characterized vagal motoneurons in the guinea pig. J Neurophysiol. 1990 Feb;63(2):333–346. doi: 10.1152/jn.1990.63.2.333. [DOI] [PubMed] [Google Scholar]
- RALL W. Branching dendritic trees and motoneuron membrane resistivity. Exp Neurol. 1959 Nov;1:491–527. doi: 10.1016/0014-4886(59)90046-9. [DOI] [PubMed] [Google Scholar]
- Rall W., Burke R. E., Holmes W. R., Jack J. J., Redman S. J., Segev I. Matching dendritic neuron models to experimental data. Physiol Rev. 1992 Oct;72(4 Suppl):S159–S186. doi: 10.1152/physrev.1992.72.suppl_4.S159. [DOI] [PubMed] [Google Scholar]
- Rall W. Time constants and electrotonic length of membrane cylinders and neurons. Biophys J. 1969 Dec;9(12):1483–1508. doi: 10.1016/S0006-3495(69)86467-2. [DOI] [PMC free article] [PubMed] [Google Scholar]
- Ross W. N., Werman R. Mapping calcium transients in the dendrites of Purkinje cells from the guinea-pig cerebellum in vitro. J Physiol. 1987 Aug;389:319–336. doi: 10.1113/jphysiol.1987.sp016659. [DOI] [PMC free article] [PubMed] [Google Scholar]
- Segev I., Fleshman J. W., Miller J. P., Bunow B. Modeling the electrical behavior of anatomically complex neurons using a network analysis program: passive membrane. Biol Cybern. 1985;53(1):27–40. doi: 10.1007/BF00355688. [DOI] [PubMed] [Google Scholar]
- Segev I., Rall W. Computational study of an excitable dendritic spine. J Neurophysiol. 1988 Aug;60(2):499–523. doi: 10.1152/jn.1988.60.2.499. [DOI] [PubMed] [Google Scholar]
- Segev I. Single neurone models: oversimple, complex and reduced. Trends Neurosci. 1992 Nov;15(11):414–421. doi: 10.1016/0166-2236(92)90003-q. [DOI] [PubMed] [Google Scholar]
- Shelton D. P. Membrane resistivity estimated for the Purkinje neuron by means of a passive computer model. Neuroscience. 1985 Jan;14(1):111–131. doi: 10.1016/0306-4522(85)90168-x. [DOI] [PubMed] [Google Scholar]
- Spruston N., Johnston D. Perforated patch-clamp analysis of the passive membrane properties of three classes of hippocampal neurons. J Neurophysiol. 1992 Mar;67(3):508–529. doi: 10.1152/jn.1992.67.3.508. [DOI] [PubMed] [Google Scholar]
- Sugimori M., Llinás R. R. Real-time imaging of calcium influx in mammalian cerebellar Purkinje cells in vitro. Proc Natl Acad Sci U S A. 1990 Jul;87(13):5084–5088. doi: 10.1073/pnas.87.13.5084. [DOI] [PMC free article] [PubMed] [Google Scholar]
- Tank D. W., Sugimori M., Connor J. A., Llinás R. R. Spatially resolved calcium dynamics of mammalian Purkinje cells in cerebellar slice. Science. 1988 Nov 4;242(4879):773–777. doi: 10.1126/science.2847315. [DOI] [PubMed] [Google Scholar]