Abstract
1. Intersegmental co-ordination in Xenopus embryos could be influenced by longitudinal gradients in neuronal properties or synaptic drive. To determine if such gradients exist intracellular recordings were made from putative motoneurones at different spinal levels. 2. No evidence was found of a longitudinal gradient in neuronal resting potentials. In a rostrocaudal direction the duration of current-evoked spikes increased and the amplitude of the spike after-hyperpolarization (AHP) decreased. 3. During fictive swimming the amplitude of the tonic excitatory synaptic input and the mid-cycle IPSPs declined in a rostrocaudal direction. The rise-time and fall-time of mid-cycle IPSPs increased in a rostrocaudal direction. 4. Rostral to the eighth post-otic segment mid-cycle IPSPs occurred on all cycles of fictive swimming episodes. More caudally IPSPs became irregular in occurrence and caudal to the twelfth post-otic segment no mid-cycle IPSPs could be detected, even during the injection of depolarizing current or when recording with KCl-filled electrodes. 5. The duration of spikes occurring during fictive swimming increased and the amplitude of spike AHP decreased in a rostrocaudal direction. A spike AHP was absent during fictive swimming activity in neurones caudal to the ninth post-otic segment even though it was present in current-evoked spikes in the same neurones. 6. On-cycle IPSPs (occurring shortly after the spike at phase values less than 0.4) were observed predominantly at the beginning of swimming episodes in neurones recorded rostral to the eighth segment, but were not detected at all in more caudal neurones. 7. If the rostrocaudal gradients in synaptic excitatory and inhibitory drive to putative motoneurones during fictive swimming are also present in premotor spinal interneurones they would be expected to have a strong influence on rostrocaudal delays. Such gradients could therefore be important components of the mechanism underlying intersegmental co-ordination.
Full text
PDF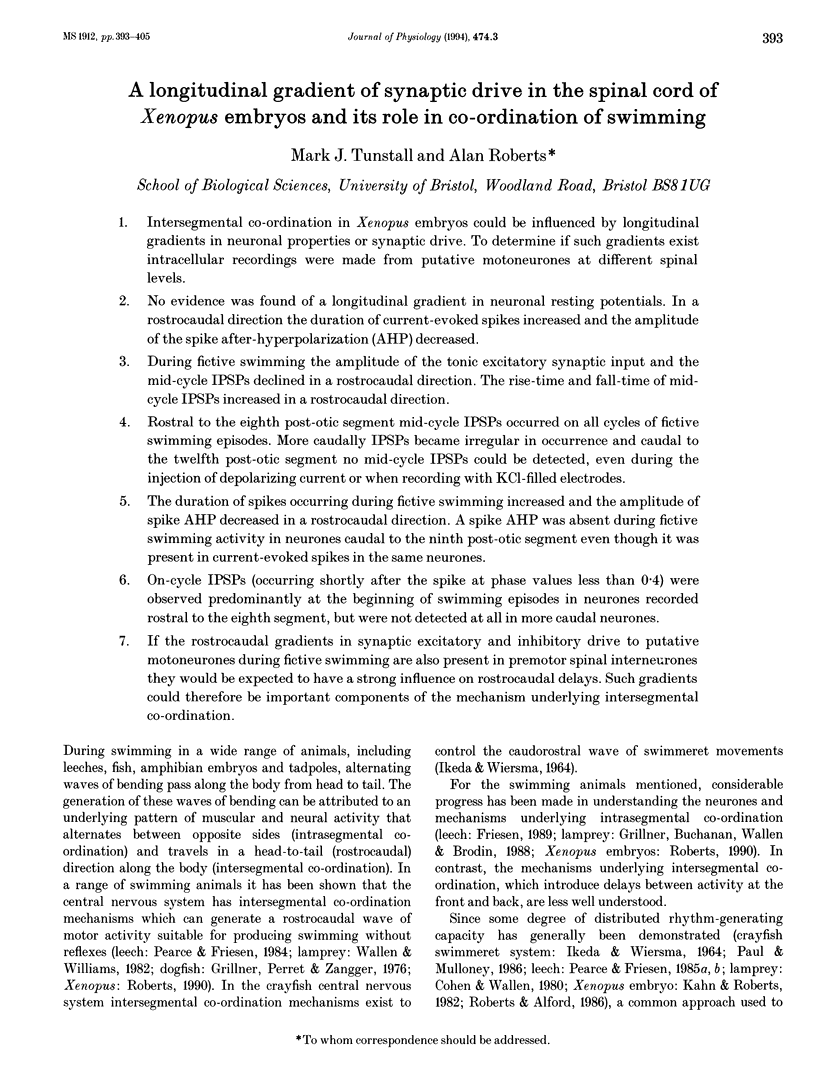
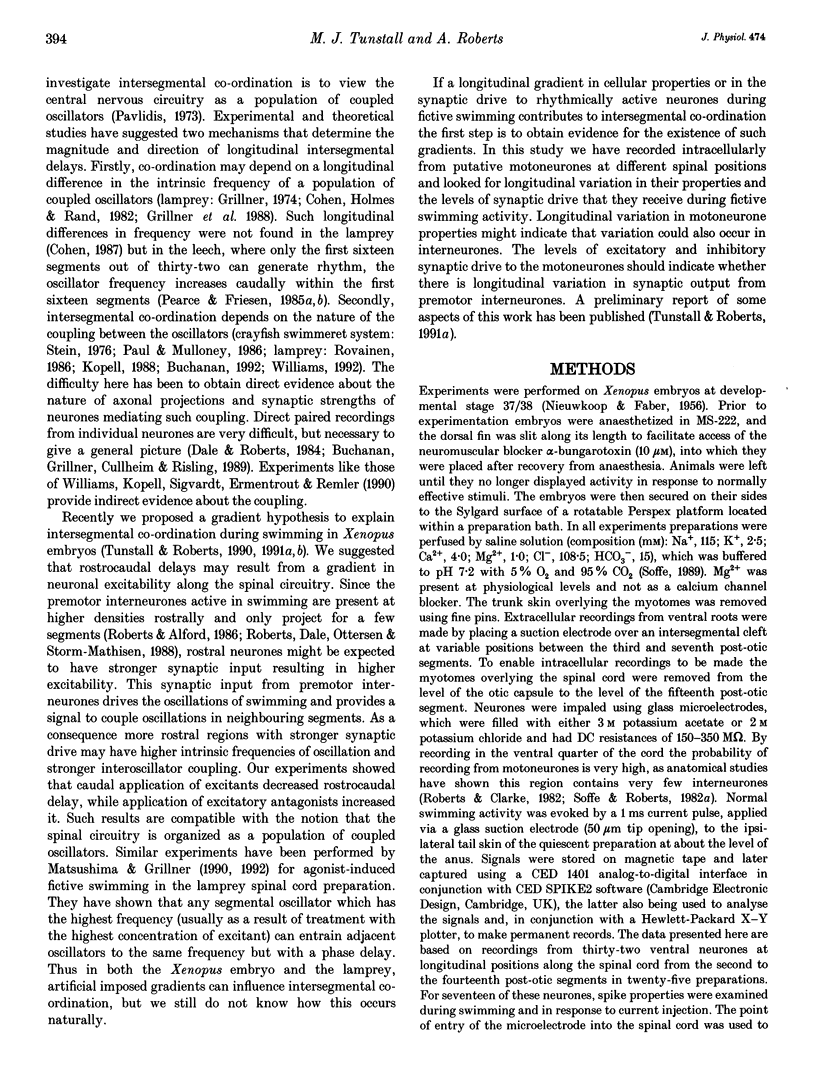
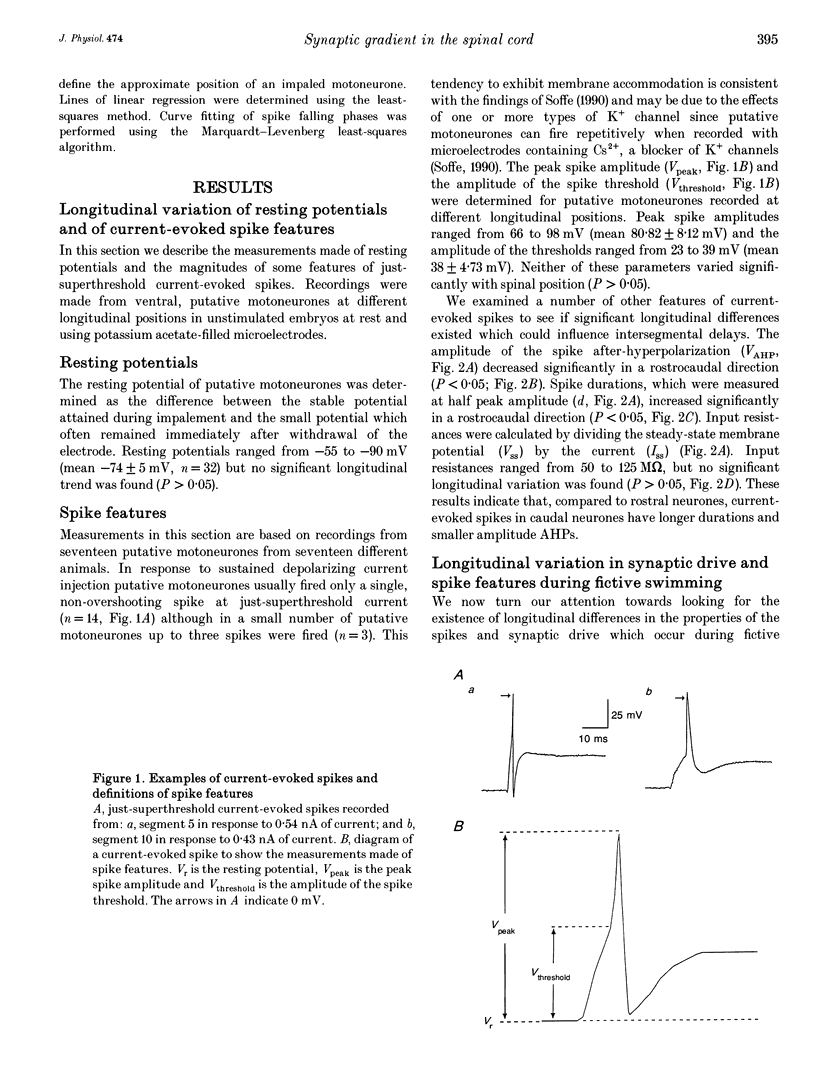
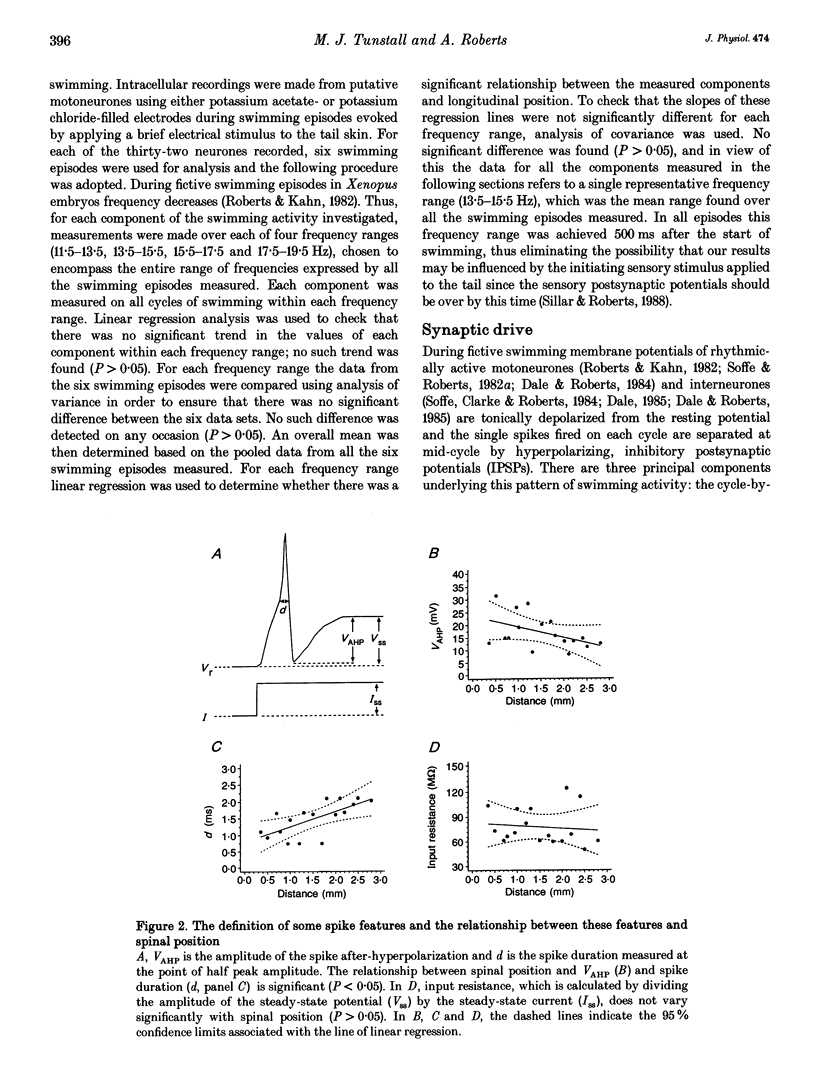
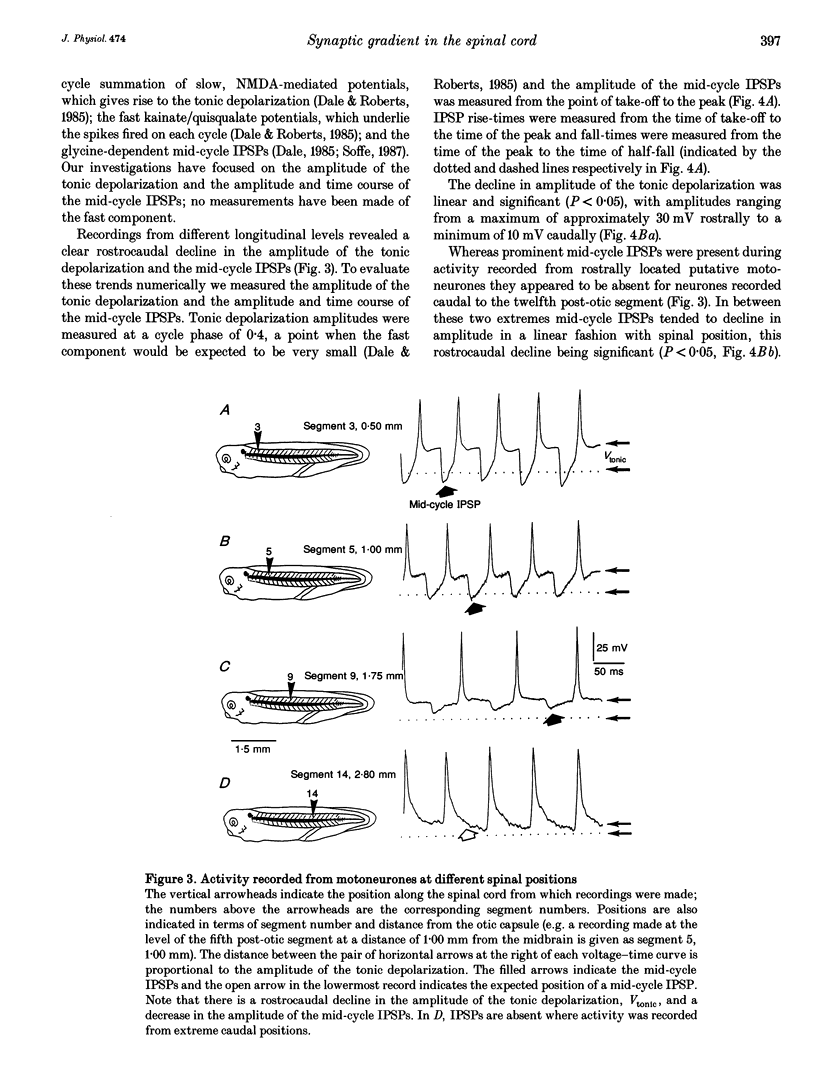
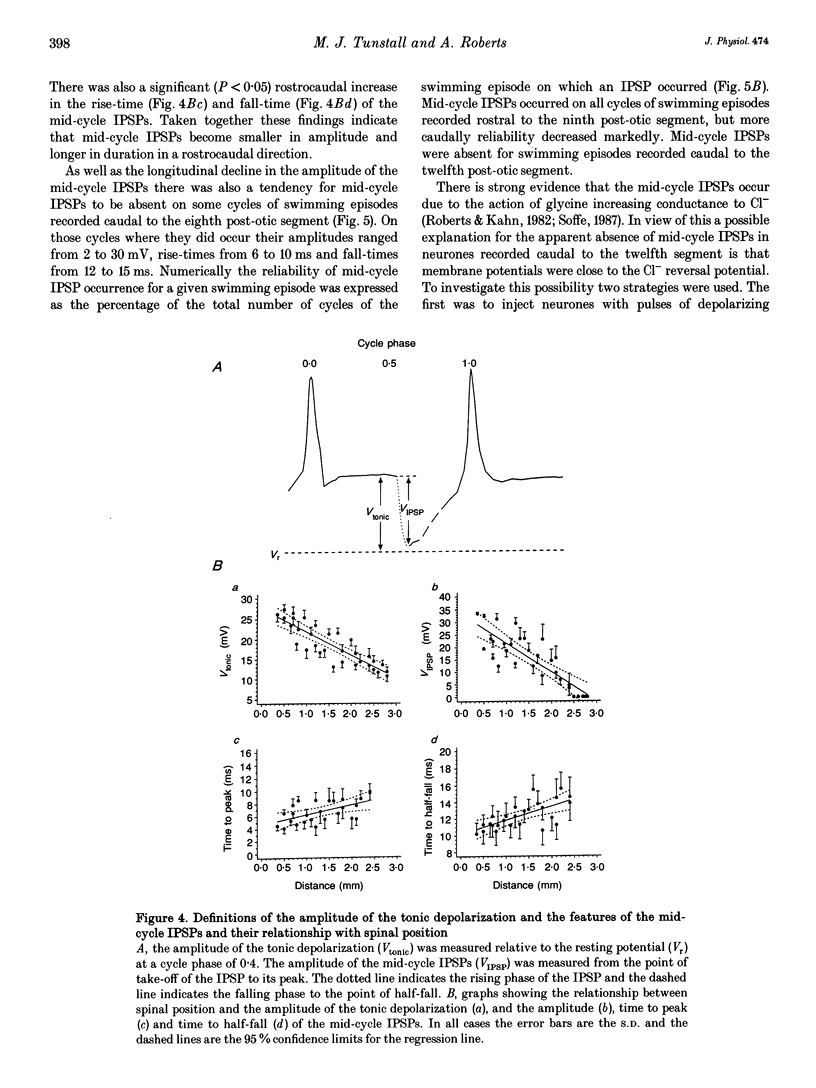
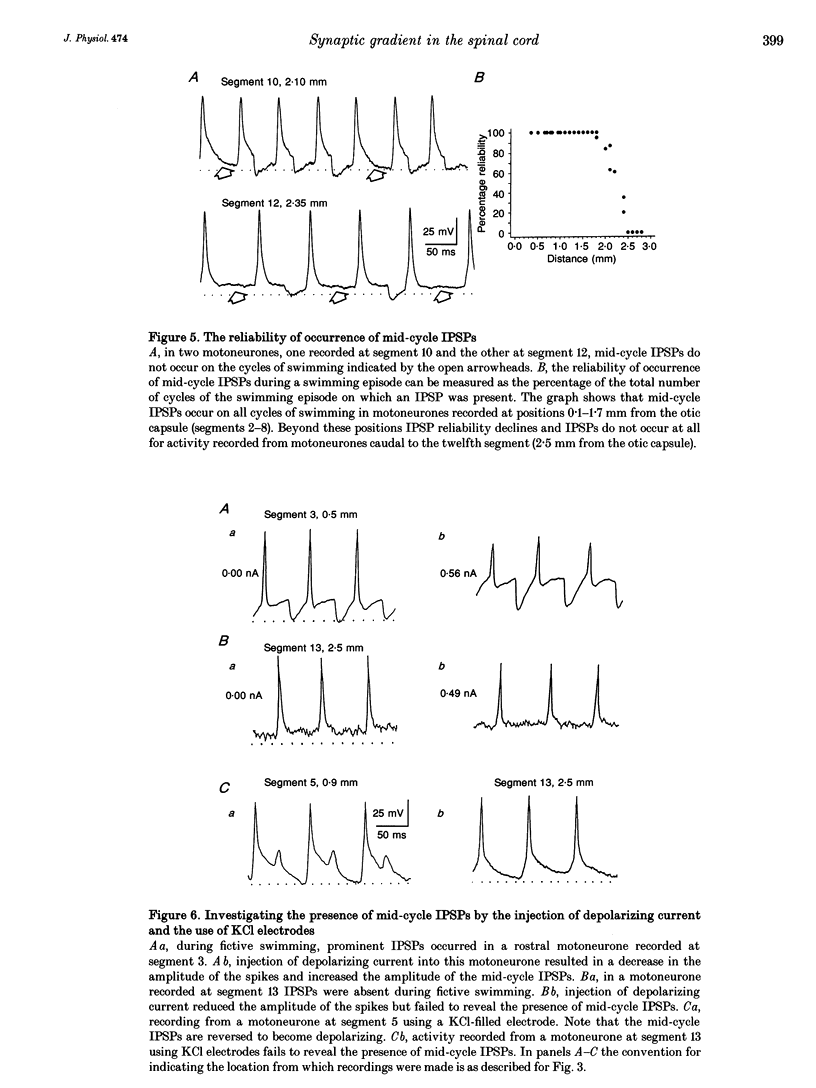
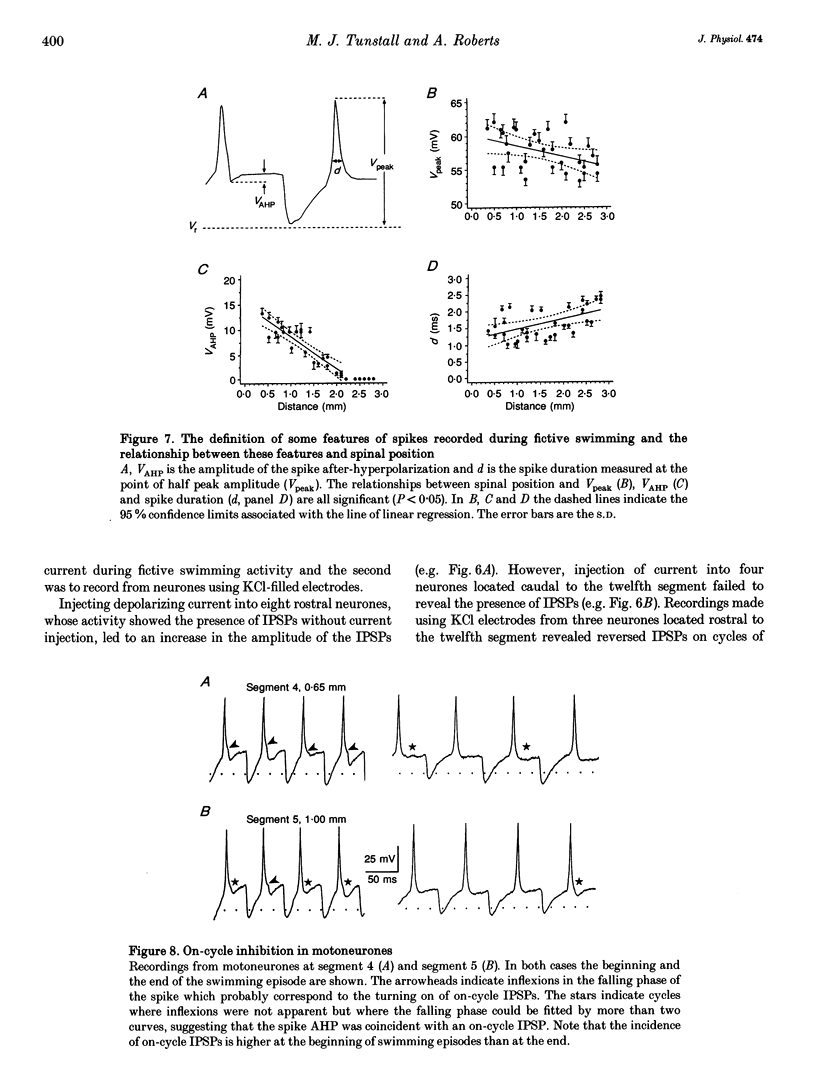
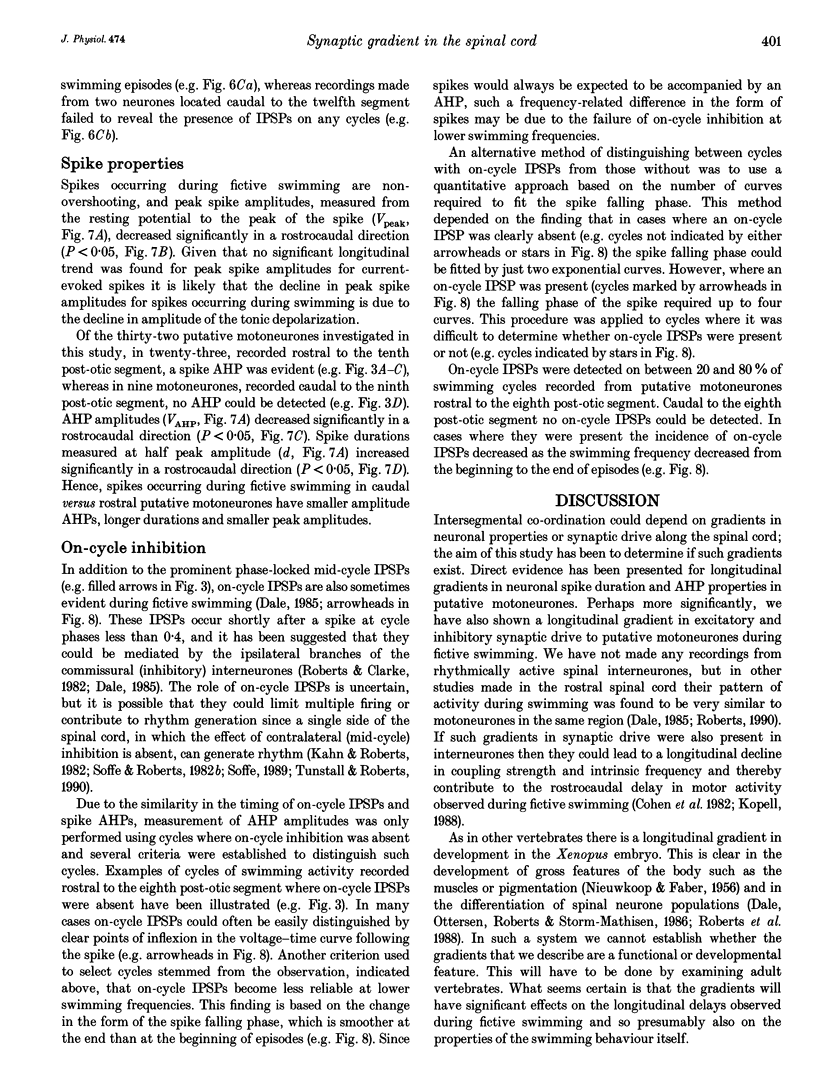
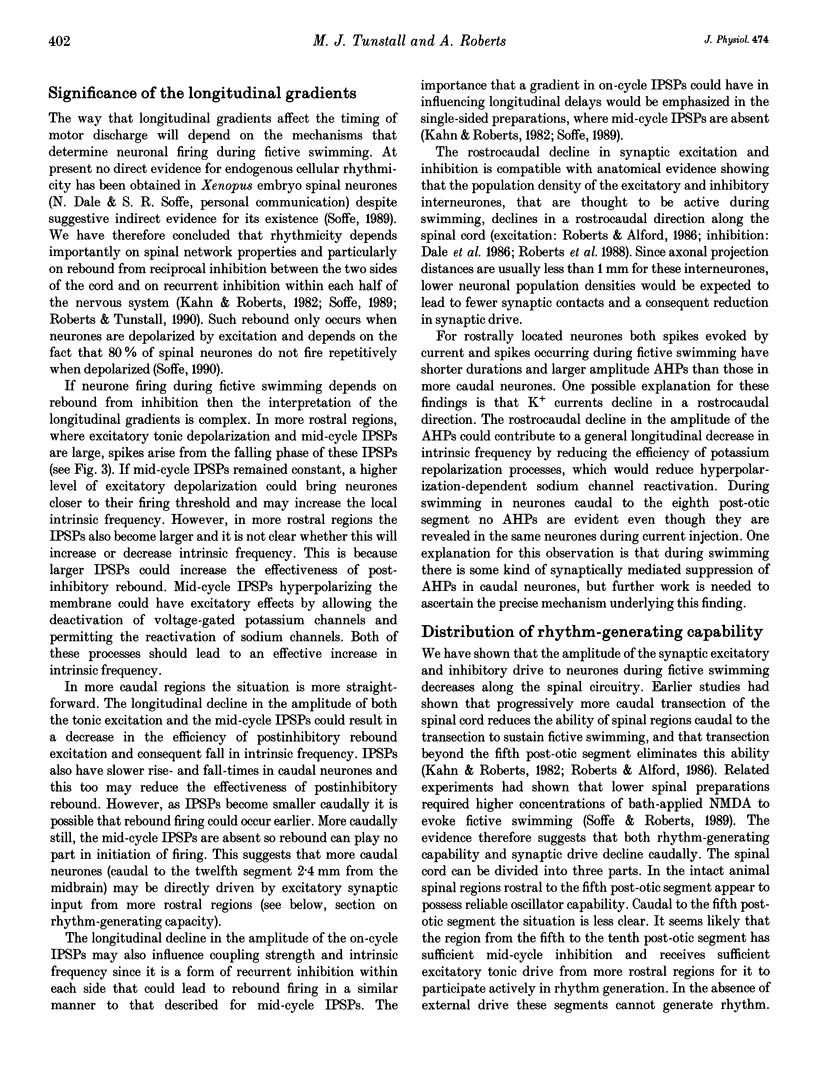
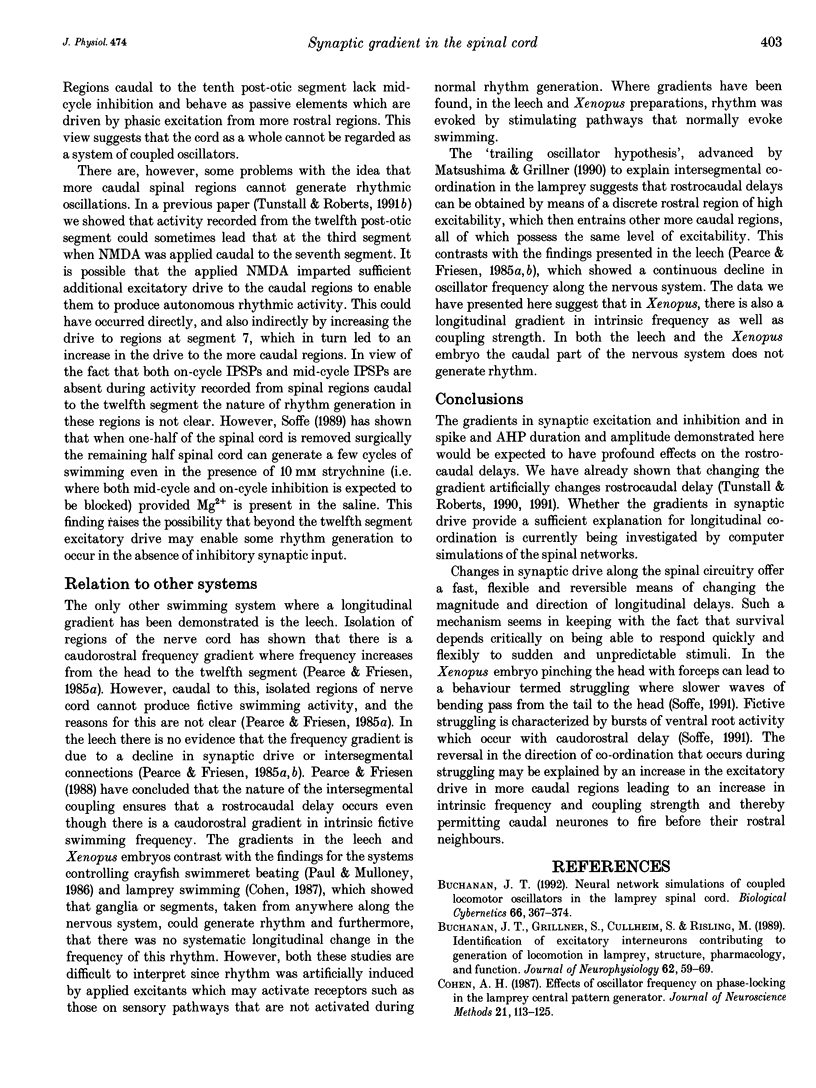
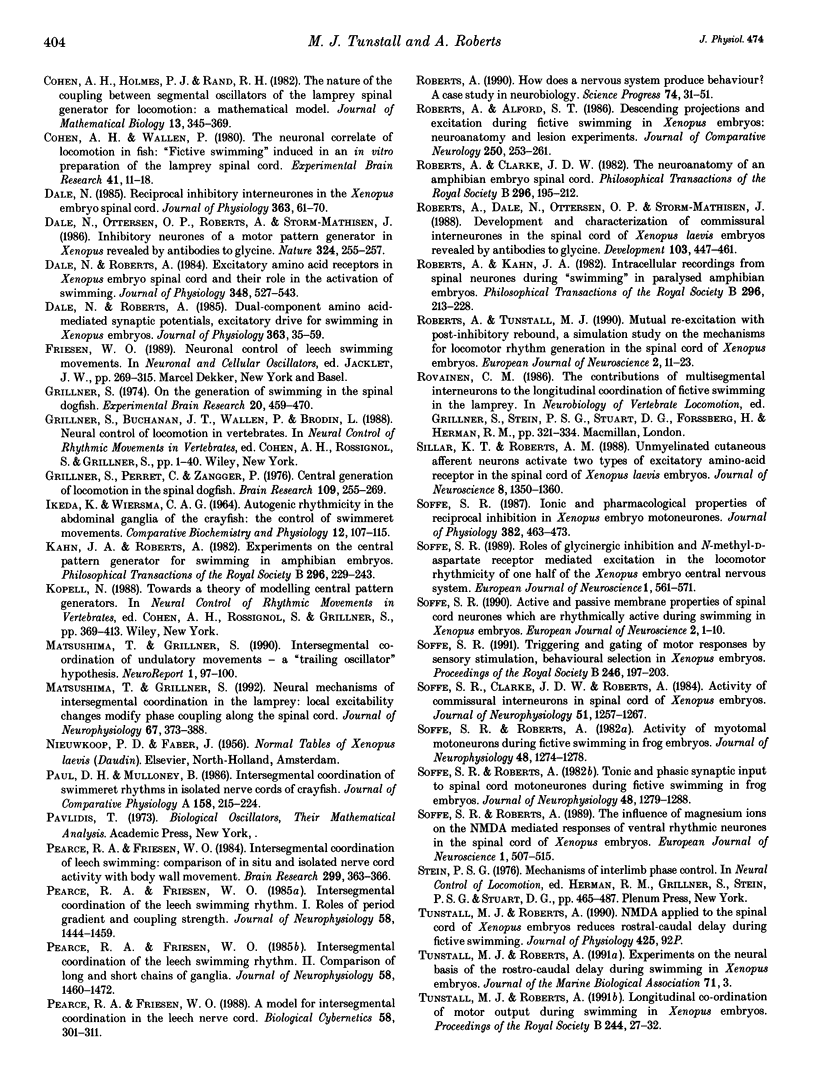
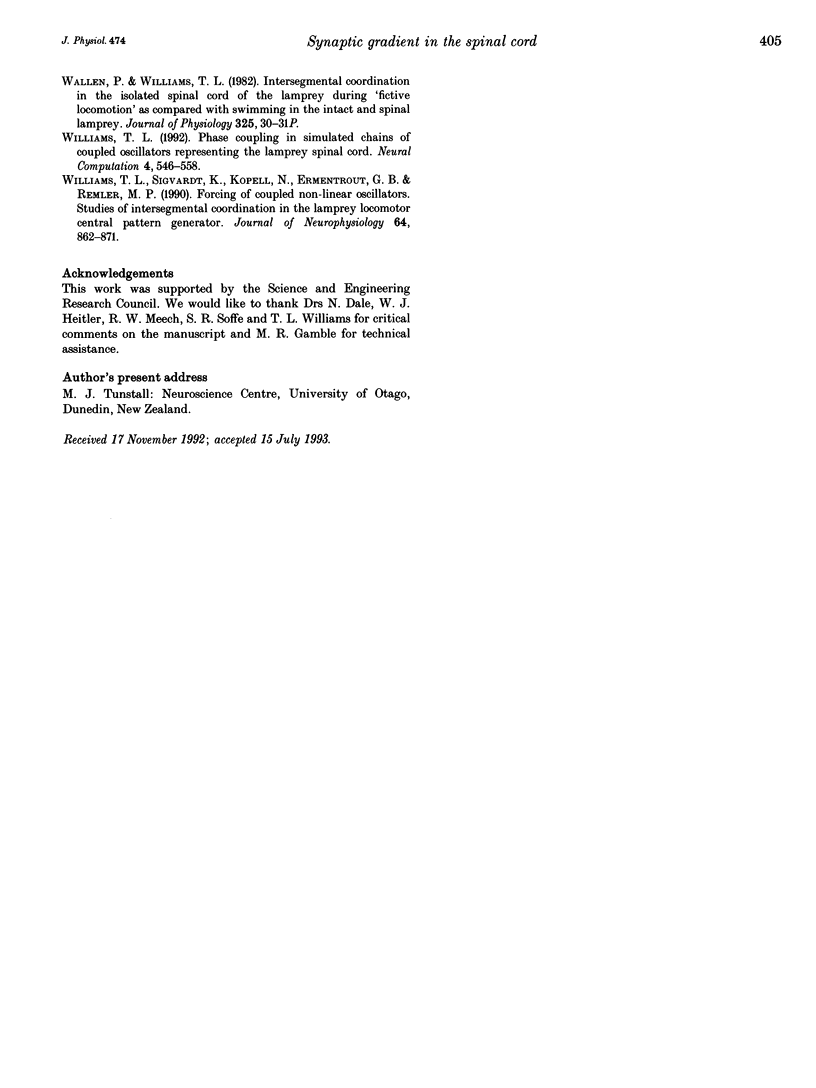
Selected References
These references are in PubMed. This may not be the complete list of references from this article.
- Buchanan J. T., Grillner S., Cullheim S., Risling M. Identification of excitatory interneurons contributing to generation of locomotion in lamprey: structure, pharmacology, and function. J Neurophysiol. 1989 Jul;62(1):59–69. doi: 10.1152/jn.1989.62.1.59. [DOI] [PubMed] [Google Scholar]
- Buchanan J. T. Neural network simulations of coupled locomotor oscillators in the lamprey spinal cord. Biol Cybern. 1992;66(4):367–374. doi: 10.1007/BF00203673. [DOI] [PubMed] [Google Scholar]
- Cohen A. H. Effects of oscillator frequency on phase-locking in the lamprey central pattern generator. J Neurosci Methods. 1987 Oct;21(2-4):113–125. doi: 10.1016/0165-0270(87)90109-9. [DOI] [PubMed] [Google Scholar]
- Cohen A. H., Holmes P. J., Rand R. H. The nature of the coupling between segmental oscillators of the lamprey spinal generator for locomotion: a mathematical model. J Math Biol. 1982;13(3):345–369. doi: 10.1007/BF00276069. [DOI] [PubMed] [Google Scholar]
- Cohen A. H., Wallén P. The neuronal correlate of locomotion in fish. "Fictive swimming" induced in an in vitro preparation of the lamprey spinal cord. Exp Brain Res. 1980;41(1):11–18. doi: 10.1007/BF00236674. [DOI] [PubMed] [Google Scholar]
- Dale N., Ottersen O. P., Roberts A., Storm-Mathisen J. Inhibitory neurones of a motor pattern generator in Xenopus revealed by antibodies to glycine. Nature. 1986 Nov 20;324(6094):255–257. doi: 10.1038/324255a0. [DOI] [PubMed] [Google Scholar]
- Dale N. Reciprocal inhibitory interneurones in the Xenopus embryo spinal cord. J Physiol. 1985 Jun;363:61–70. doi: 10.1113/jphysiol.1985.sp015695. [DOI] [PMC free article] [PubMed] [Google Scholar]
- Dale N., Roberts A. Dual-component amino-acid-mediated synaptic potentials: excitatory drive for swimming in Xenopus embryos. J Physiol. 1985 Jun;363:35–59. doi: 10.1113/jphysiol.1985.sp015694. [DOI] [PMC free article] [PubMed] [Google Scholar]
- Dale N., Roberts A. Excitatory amino acid receptors in Xenopus embryo spinal cord and their role in the activation of swimming. J Physiol. 1984 Mar;348:527–543. doi: 10.1113/jphysiol.1984.sp015123. [DOI] [PMC free article] [PubMed] [Google Scholar]
- Grillner S. On the generation of locomotion in the spinal dogfish. Exp Brain Res. 1974;20(5):459–470. doi: 10.1007/BF00238013. [DOI] [PubMed] [Google Scholar]
- Grillner S., Perret C., Zangger P. Central generation of locomotion in the spinal dogfish. Brain Res. 1976 Jun 11;109(2):255–269. doi: 10.1016/0006-8993(76)90529-1. [DOI] [PubMed] [Google Scholar]
- IKEDA K., WIERSMA C. A. AUTOGENIC RHYTHMICITY IN THE ABDOMINAL GANGLIA OF THE CRAYFISH: THE CONTROL OF SWIMMERET MOVEMENTS. Comp Biochem Physiol. 1964 May;12:107–115. doi: 10.1016/0010-406x(64)90053-2. [DOI] [PubMed] [Google Scholar]
- Kahn J. A., Roberts A. Experiments on the central pattern generator for swimming in amphibian embryos. Philos Trans R Soc Lond B Biol Sci. 1982 Jan 27;296(1081):229–243. doi: 10.1098/rstb.1982.0004. [DOI] [PubMed] [Google Scholar]
- Matsushima T., Grillner S. Intersegmental co-ordination of undulatory movements--a "trailing oscillator" hypothesis. Neuroreport. 1990 Oct;1(2):97–100. doi: 10.1097/00001756-199010000-00003. [DOI] [PubMed] [Google Scholar]
- Matsushima T., Grillner S. Neural mechanisms of intersegmental coordination in lamprey: local excitability changes modify the phase coupling along the spinal cord. J Neurophysiol. 1992 Feb;67(2):373–388. doi: 10.1152/jn.1992.67.2.373. [DOI] [PubMed] [Google Scholar]
- Pearce R. A., Friesen W. O. A model for intersegmental coordination in the leech nerve cord. Biol Cybern. 1988;58(5):301–311. doi: 10.1007/BF00363939. [DOI] [PubMed] [Google Scholar]
- Pearce R. A., Friesen W. O. Intersegmental coordination of leech swimming: comparison of in situ and isolated nerve cord activity with body wall movement. Brain Res. 1984 May 14;299(2):363–366. doi: 10.1016/0006-8993(84)90720-0. [DOI] [PubMed] [Google Scholar]
- Pearce R. A., Friesen W. O. Intersegmental coordination of the leech swimming rhythm. I. Roles of cycle period gradient and coupling strength. J Neurophysiol. 1985 Dec;54(6):1444–1459. doi: 10.1152/jn.1985.54.6.1444. [DOI] [PubMed] [Google Scholar]
- Pearce R. A., Friesen W. O. Intersegmental coordination of the leech swimming rhythm. II. Comparison of long and short chains of ganglia. J Neurophysiol. 1985 Dec;54(6):1460–1472. doi: 10.1152/jn.1985.54.6.1460. [DOI] [PubMed] [Google Scholar]
- Roberts A., Alford S. T. Descending projections and excitation during fictive swimming in Xenopus embryos: neuroanatomy and lesion experiments. J Comp Neurol. 1986 Aug 8;250(2):253–261. doi: 10.1002/cne.902500212. [DOI] [PubMed] [Google Scholar]
- Roberts A., Clarke J. D. The neuroanatomy of an amphibian embryo spinal cord. Philos Trans R Soc Lond B Biol Sci. 1982 Jan 27;296(1081):195–212. doi: 10.1098/rstb.1982.0002. [DOI] [PubMed] [Google Scholar]
- Roberts A., Dale N., Ottersen O. P., Storm-Mathisen J. Development and characterization of commissural interneurones in the spinal cord of Xenopus laevis embryos revealed by antibodies to glycine. Development. 1988 Jul;103(3):447–461. doi: 10.1242/dev.103.3.447. [DOI] [PubMed] [Google Scholar]
- Roberts A. How does a nervous system produce behaviour? A case study in neurobiology. Sci Prog. 1990;74(293 Pt 1):31–51. [PubMed] [Google Scholar]
- Roberts A., Khan J. A. Intracellular recordings from spinal neurons during 'swimming' in paralysed amphibian embryos. Philos Trans R Soc Lond B Biol Sci. 1982 Jan 27;296(1081):213–228. doi: 10.1098/rstb.1982.0003. [DOI] [PubMed] [Google Scholar]
- Roberts A., Tunstall M. J. Mutual Re-excitation with Post-Inhibitory Rebound: A Simulation Study on the Mechanisms for Locomotor Rhythm Generation in the Spinal Cord of Xenopus Embryos. Eur J Neurosci. 1990;2(1):11–23. doi: 10.1111/j.1460-9568.1990.tb00377.x. [DOI] [PubMed] [Google Scholar]
- Sillar K. T., Roberts A. Unmyelinated cutaneous afferent neurons activate two types of excitatory amino acid receptor in the spinal cord of Xenopus laevis embryos. J Neurosci. 1988 Apr;8(4):1350–1360. doi: 10.1523/JNEUROSCI.08-04-01350.1988. [DOI] [PMC free article] [PubMed] [Google Scholar]
- Soffe S. R. Active and Passive Membrane Properties of Spinal Cord Neurons that Are Rhythmically Active during Swimming in Xenopus Embryos. Eur J Neurosci. 1990 Jan;2(1):1–10. doi: 10.1111/j.1460-9568.1990.tb00376.x. [DOI] [PubMed] [Google Scholar]
- Soffe S. R., Clarke J. D., Roberts A. Activity of commissural interneurons in spinal cord of Xenopus embryos. J Neurophysiol. 1984 Jun;51(6):1257–1267. doi: 10.1152/jn.1984.51.6.1257. [DOI] [PubMed] [Google Scholar]
- Soffe S. R. Ionic and pharmacological properties of reciprocal inhibition in Xenopus embryo motoneurones. J Physiol. 1987 Jan;382:463–473. doi: 10.1113/jphysiol.1987.sp016378. [DOI] [PMC free article] [PubMed] [Google Scholar]
- Soffe S. R., Roberts A. Activity of myotomal motoneurons during fictive swimming in frog embryos. J Neurophysiol. 1982 Dec;48(6):1274–1278. doi: 10.1152/jn.1982.48.6.1274. [DOI] [PubMed] [Google Scholar]
- Soffe S. R., Roberts A. Tonic and phasic synaptic input to spinal cord motoneurons during fictive locomotion in frog embryos. J Neurophysiol. 1982 Dec;48(6):1279–1288. doi: 10.1152/jn.1982.48.6.1279. [DOI] [PubMed] [Google Scholar]
- Soffe S. R., Roberts Alan. The Influence of Magnesium Ions on the NMDA Mediated Responses of Ventral Rhythmic Neurons in the Spinal Cord of Xenopus Embryos. Eur J Neurosci. 1989 Sep;1(5):507–515. doi: 10.1111/j.1460-9568.1989.tb00357.x. [DOI] [PubMed] [Google Scholar]
- Soffe S. R. Roles of Glycinergic Inhibition and N-Methyl-D-Aspartate Receptor Mediated Excitation in the Locomotor Rhythmicity of One Half of the Xenopus Embryo Central Nervous System. Eur J Neurosci. 1989 Jan;1(6):561–571. doi: 10.1111/j.1460-9568.1989.tb00363.x. [DOI] [PubMed] [Google Scholar]
- Soffe S. R. Triggering and gating of motor responses by sensory stimulation: behavioural selection in Xenopus embryos. Proc Biol Sci. 1991 Dec 23;246(1317):197–203. doi: 10.1098/rspb.1991.0145. [DOI] [PubMed] [Google Scholar]
- Tunstall M. J., Roberts A. Longitudinal coordination of motor output during swimming in Xenopus embryos. Proc Biol Sci. 1991 Apr 22;244(1309):27–32. doi: 10.1098/rspb.1991.0046. [DOI] [PubMed] [Google Scholar]
- Williams T. L., Sigvardt K. A., Kopell N., Ermentrout G. B., Remler M. P. Forcing of coupled nonlinear oscillators: studies of intersegmental coordination in the lamprey locomotor central pattern generator. J Neurophysiol. 1990 Sep;64(3):862–871. doi: 10.1152/jn.1990.64.3.862. [DOI] [PubMed] [Google Scholar]