Abstract
1. The rise of plasma [K+] during high intensity exercise is due to an initially rapid loss of K+ from the exercising muscle to the circulation. The K+ loss is primarily governed by the balance between K+ efflux rate from the muscle cells and the reuptake rate. It has been assumed that the reuptake rate is proportional to [K+] in the femoral vein ([K+]fv) during short-lasting uphill running, but this may not hold true for other types and durations of exercise. 2. In four subjects, initial rates of increase and decay of [K+]fv at start and end of bicycle exercise were quantified by means of K(+)-sensitive electrodes inserted into the femoral vein. Responses to exercise intensities between 90 and 440 W were examined. Both the initial rate of rise and the rate of decay of [K+]fv were linearly related to power. 3. In six subjects, exercising at 60, 85 and 110% of maximal oxygen uptake, blood was obtained from the femoral artery and vein. The veno-arterial concentration difference for K+ across the exercising leg decayed with half-times of about 3 min at all exercise levels and became not significantly different from zero at low powers. This fits with a good match between K+ efflux and reuptake rates at the cellular level. 4. Arterial plasma [K+] ([K+]a) rose faster with increasing exercise intensity, reaching peak values of 5.7 +/- 0.1, 6.0 +/- 0.2 and 8.0 +/- 0.2 mmol l-1. [K+]a fell again over the subsequent 5 min at the lowest intensity in spite of significant loss of muscle K+. Hence, released K+ was redistributed to other compartments outside the vascular bed. 5. While K+ loss increased linearly with increasing power, [K+]a showed a curvilinear relationship. Thus redistribution of K+ is less efficient at high intensities. [K+]a correlated better with relative work load than with absolute work load. 6. Reuptake of K+ after the end of the high intensity bout of exercise caused [K+]a to fall with a half-time of 31 s. The rate of K+ reuptake in the exercising muscle was not proportional to [K+]a or [K+]fv. However, at the level of the muscle cell, the rate of K+ reuptake was probably inversely related to intracellular [K+].
Full text
PDF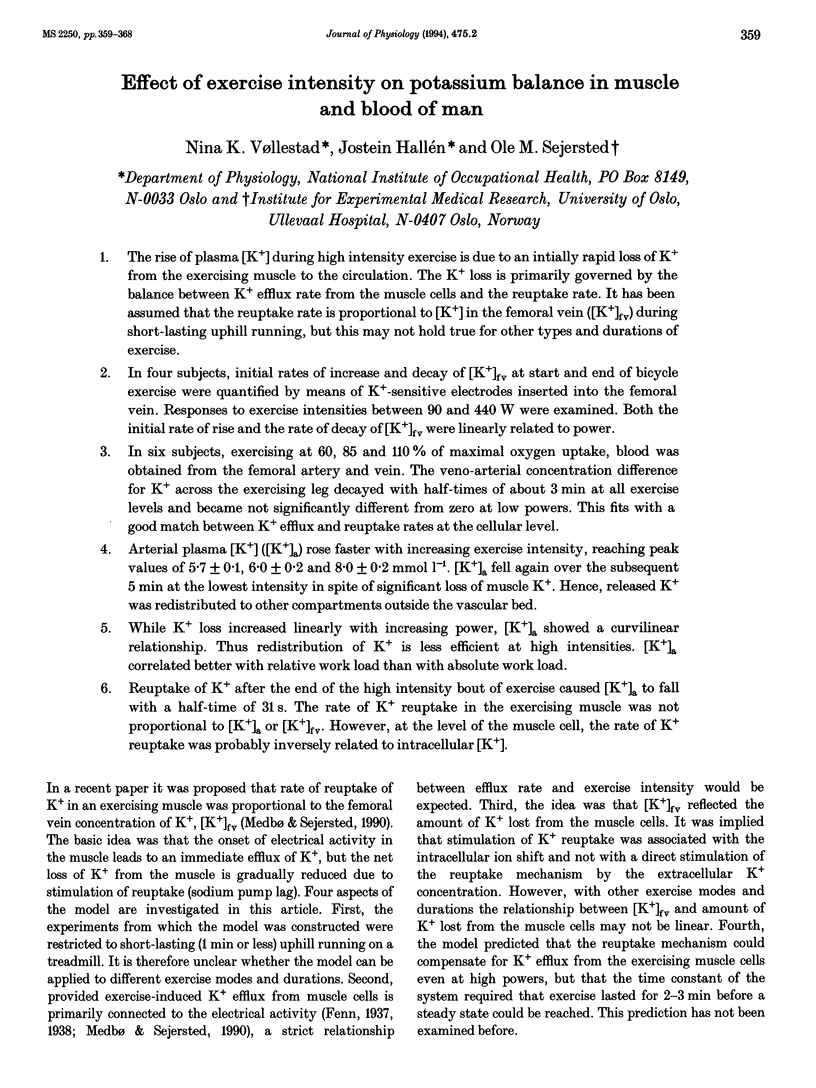
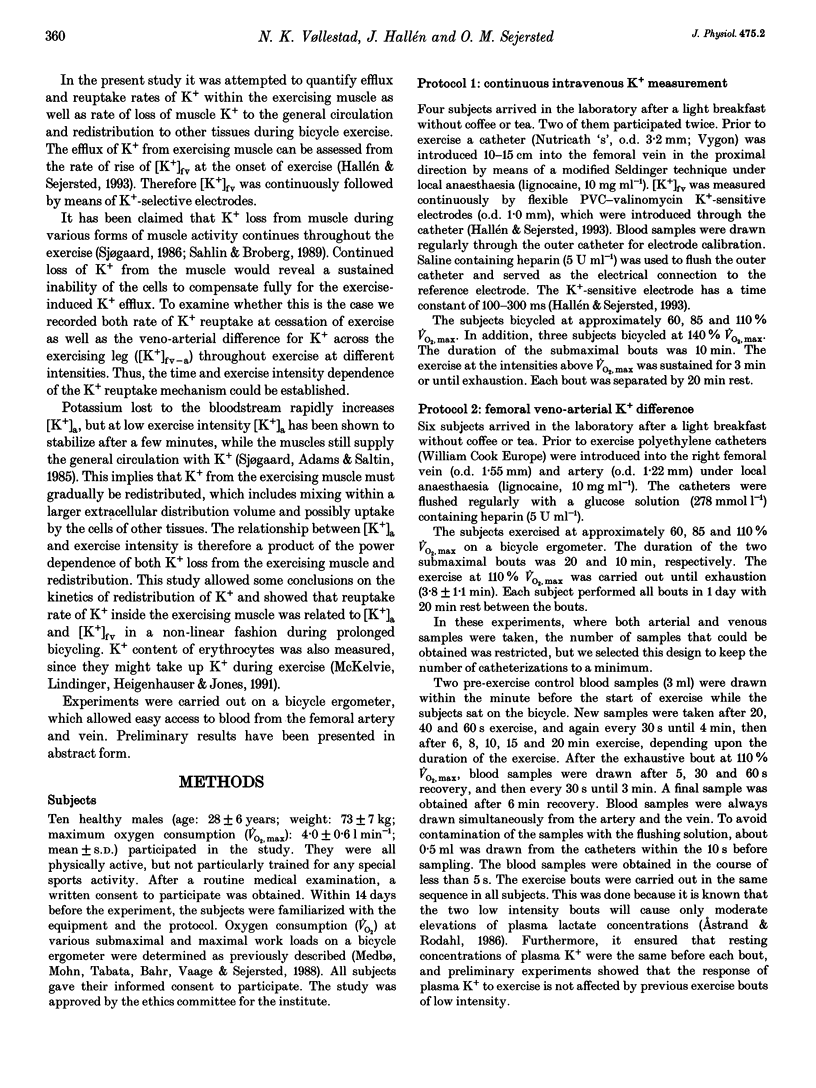
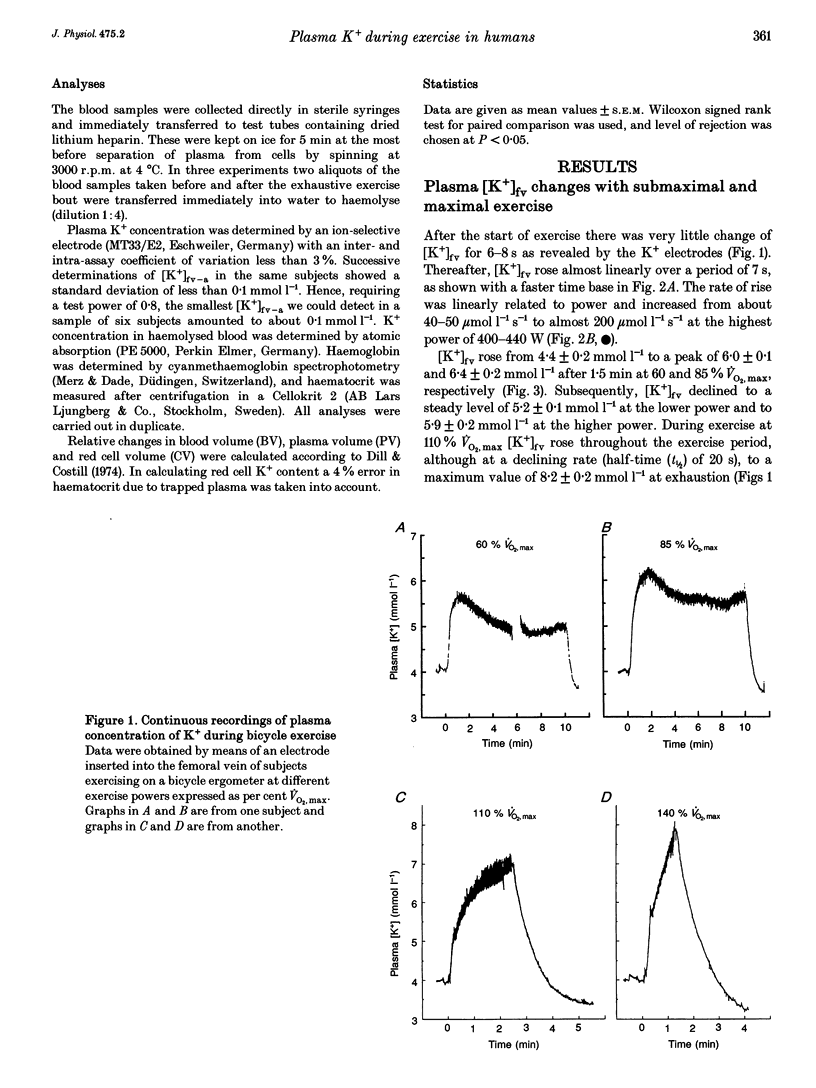
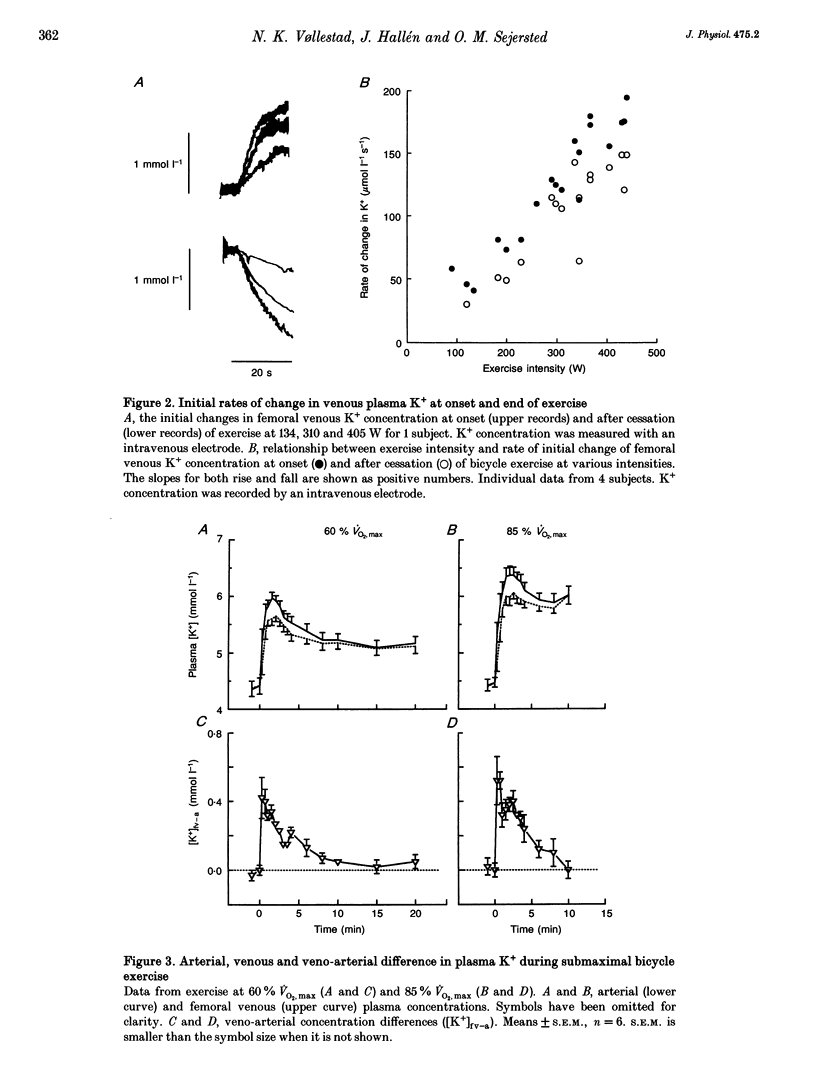
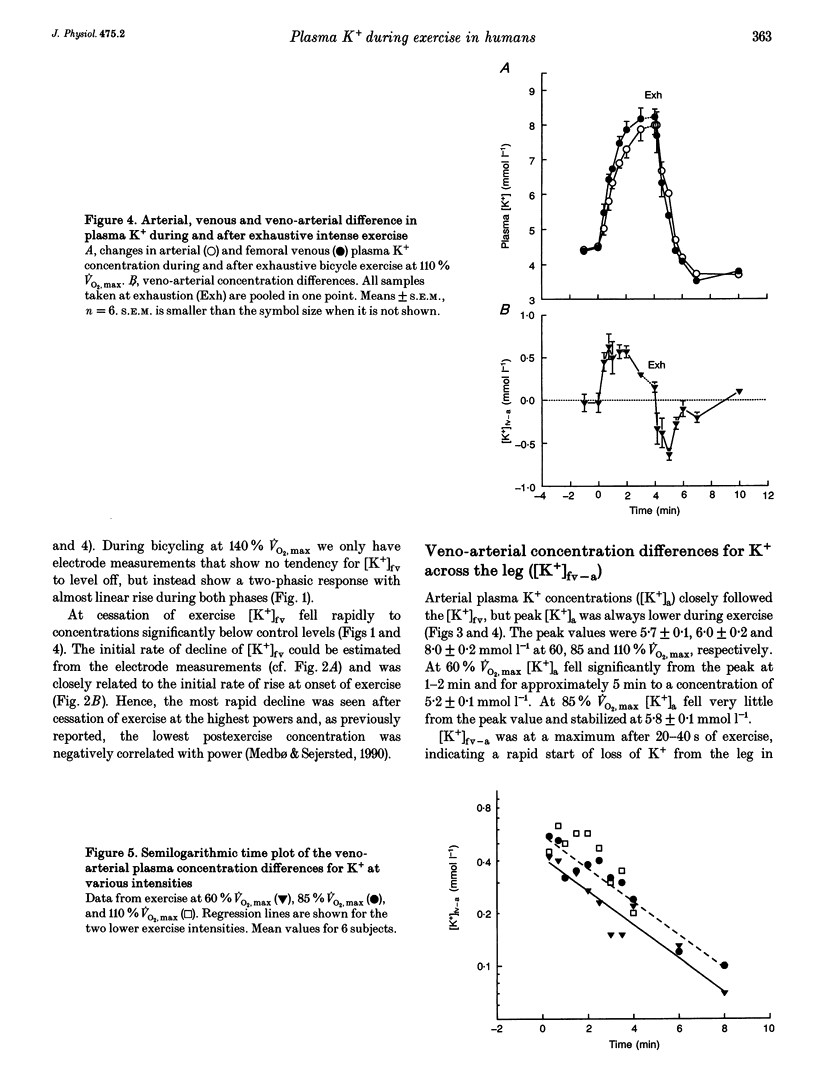
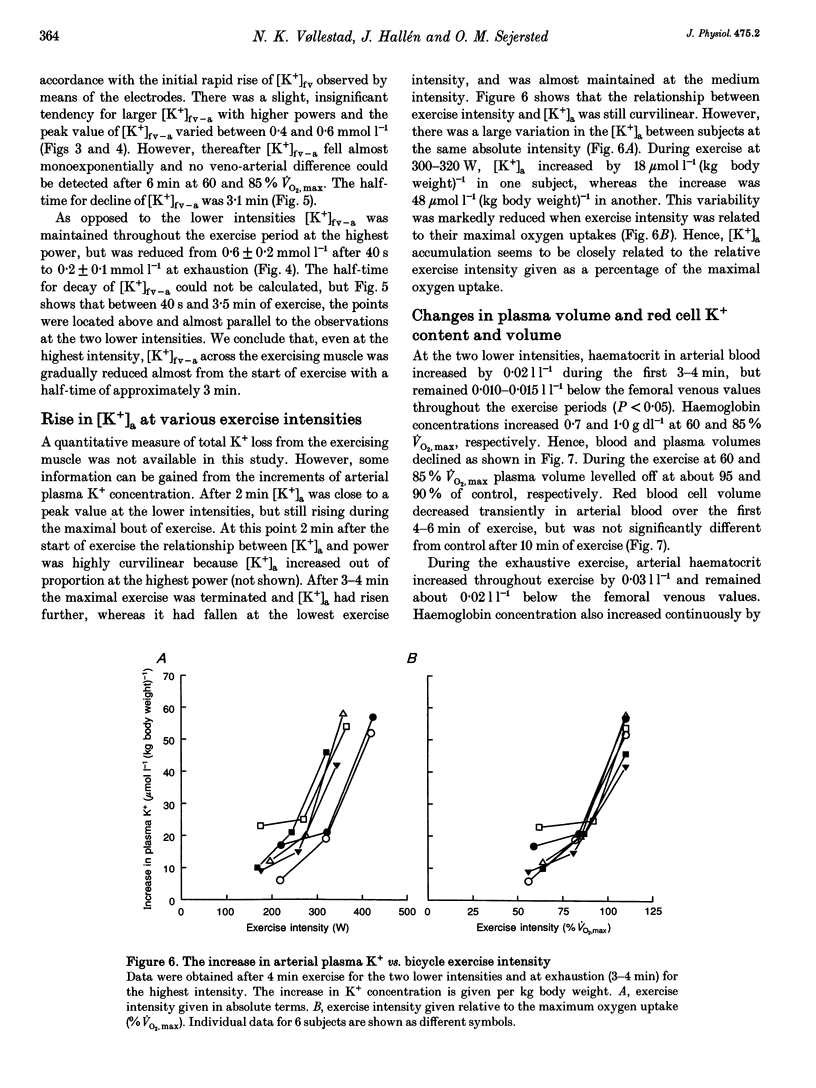
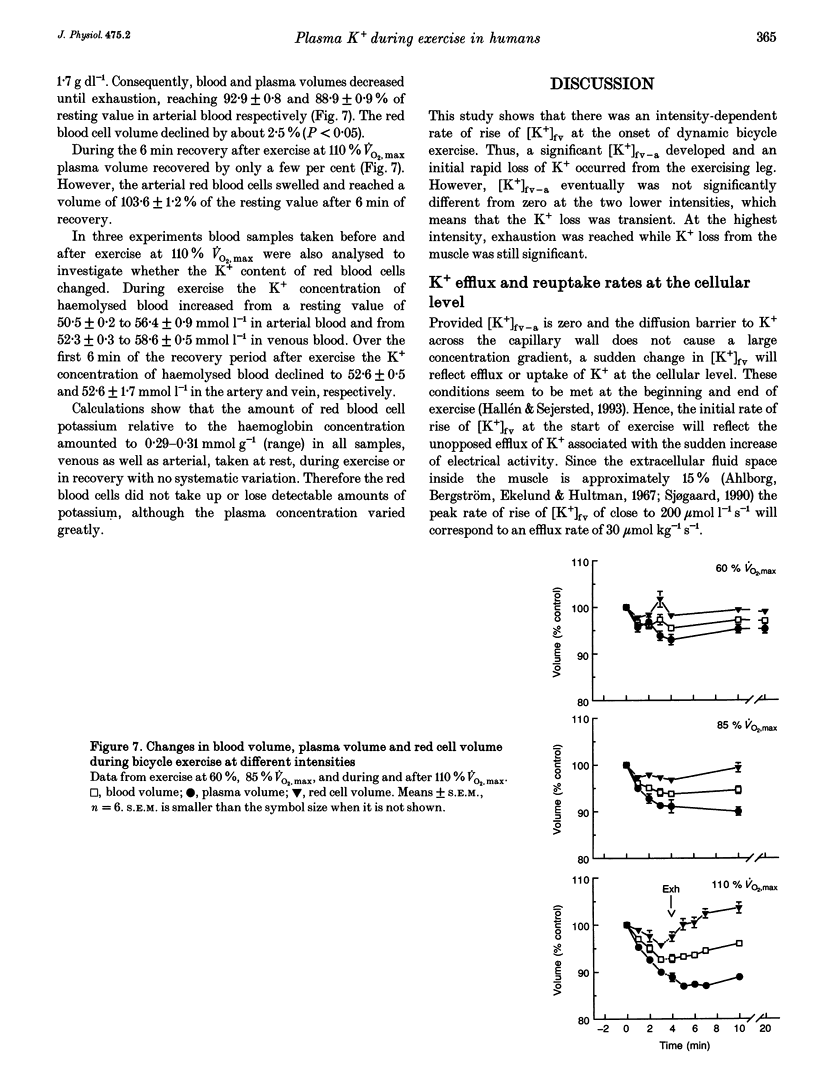
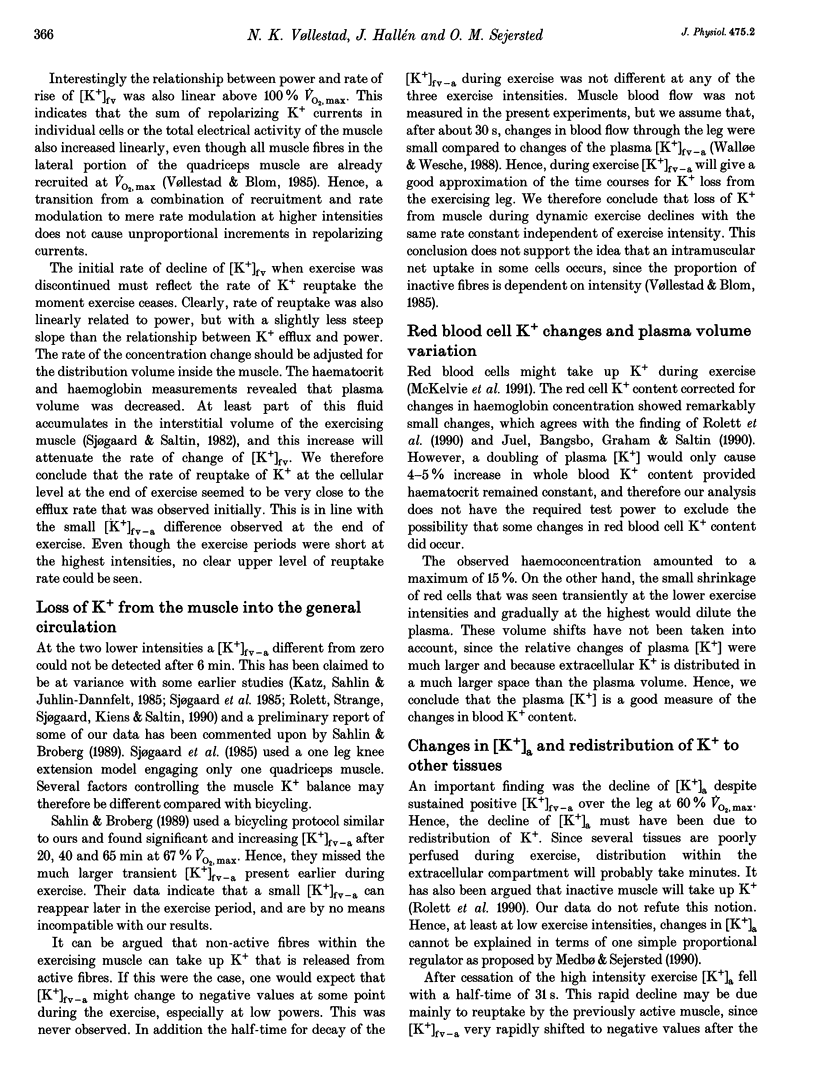
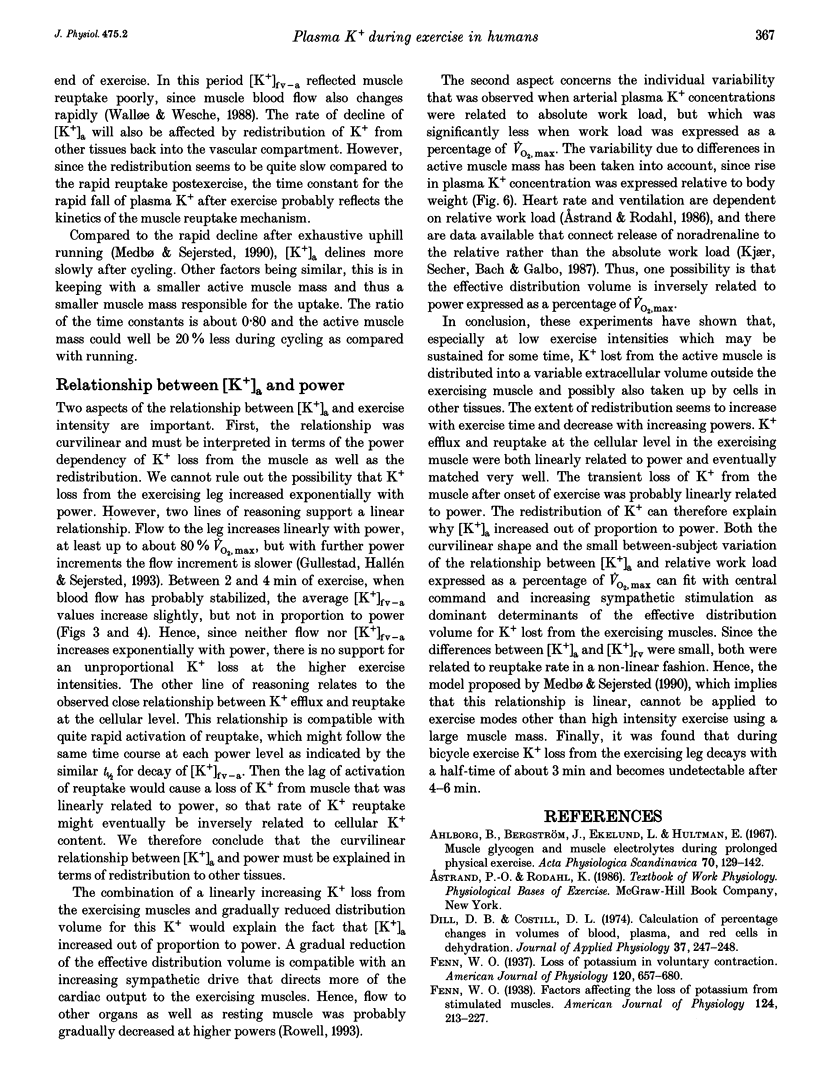
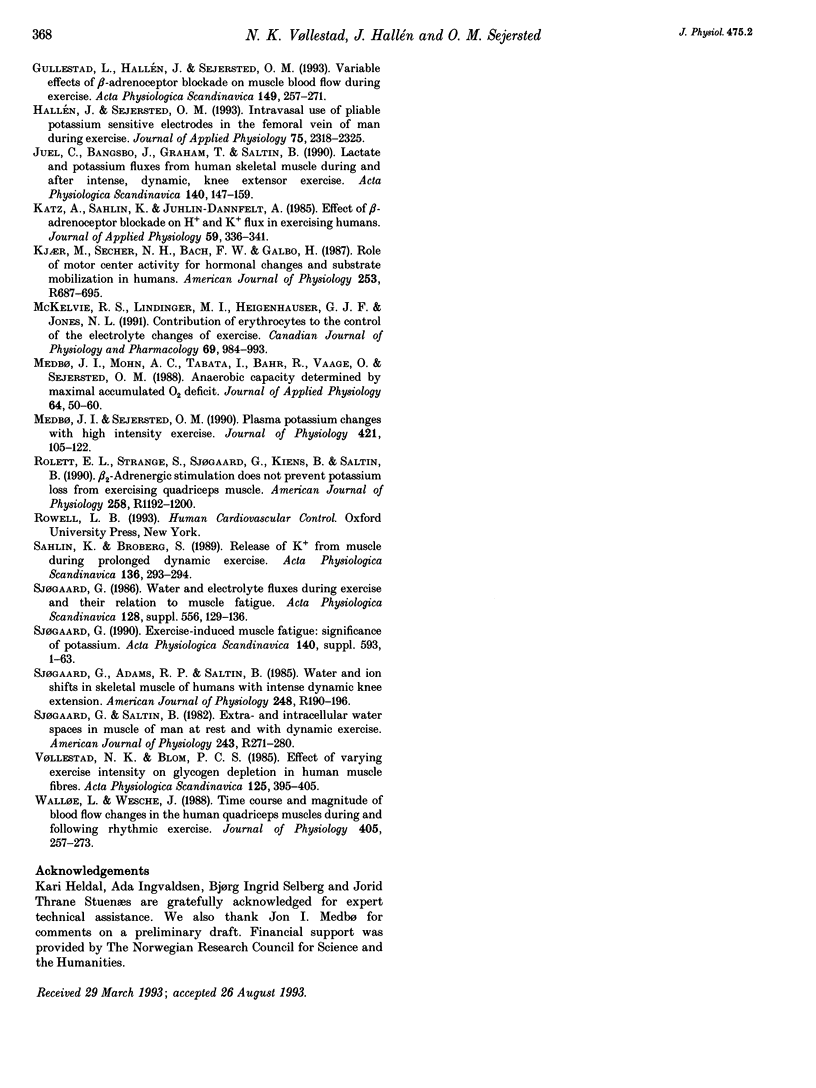
Selected References
These references are in PubMed. This may not be the complete list of references from this article.
- Dill D. B., Costill D. L. Calculation of percentage changes in volumes of blood, plasma, and red cells in dehydration. J Appl Physiol. 1974 Aug;37(2):247–248. doi: 10.1152/jappl.1974.37.2.247. [DOI] [PubMed] [Google Scholar]
- Gullestad L., Hallén J., Sejersted O. M. Variable effects of beta-adrenoceptor blockade on muscle blood flow during exercise. Acta Physiol Scand. 1993 Nov;149(3):257–271. doi: 10.1111/j.1748-1716.1993.tb09621.x. [DOI] [PubMed] [Google Scholar]
- Hallén J., Sejersted O. M. Intravasal use of pliable K(+)-selective electrodes in the femoral vein of humans during exercise. J Appl Physiol (1985) 1993 Nov;75(5):2318–2325. doi: 10.1152/jappl.1993.75.5.2318. [DOI] [PubMed] [Google Scholar]
- Juel C., Bangsbo J., Graham T., Saltin B. Lactate and potassium fluxes from human skeletal muscle during and after intense, dynamic, knee extensor exercise. Acta Physiol Scand. 1990 Oct;140(2):147–159. doi: 10.1111/j.1748-1716.1990.tb08986.x. [DOI] [PubMed] [Google Scholar]
- Katz A., Sahlin K., Juhlin-Dannfelt A. Effect of beta-adrenoceptor blockade on H+ and K+ flux in exercising humans. J Appl Physiol (1985) 1985 Aug;59(2):336–341. doi: 10.1152/jappl.1985.59.2.336. [DOI] [PubMed] [Google Scholar]
- Kjaer M., Secher N. H., Bach F. W., Galbo H. Role of motor center activity for hormonal changes and substrate mobilization in humans. Am J Physiol. 1987 Nov;253(5 Pt 2):R687–R695. doi: 10.1152/ajpregu.1987.253.5.R687. [DOI] [PubMed] [Google Scholar]
- McKelvie R. S., Lindinger M. I., Heigenhauser G. J., Jones N. L. Contribution of erythrocytes to the control of the electrolyte changes of exercise. Can J Physiol Pharmacol. 1991 Jul;69(7):984–993. doi: 10.1139/y91-148. [DOI] [PubMed] [Google Scholar]
- Medbø J. I., Mohn A. C., Tabata I., Bahr R., Vaage O., Sejersted O. M. Anaerobic capacity determined by maximal accumulated O2 deficit. J Appl Physiol (1985) 1988 Jan;64(1):50–60. doi: 10.1152/jappl.1988.64.1.50. [DOI] [PubMed] [Google Scholar]
- Medbø J. I., Sejersted O. M. Plasma potassium changes with high intensity exercise. J Physiol. 1990 Feb;421:105–122. doi: 10.1113/jphysiol.1990.sp017935. [DOI] [PMC free article] [PubMed] [Google Scholar]
- Rolett E. L., Strange S., Sjøgaard G., Kiens B., Saltin B. Beta 2-adrenergic stimulation does not prevent potassium loss from exercising quadriceps muscle. Am J Physiol. 1990 May;258(5 Pt 2):R1192–R1200. doi: 10.1152/ajpregu.1990.258.5.R1192. [DOI] [PubMed] [Google Scholar]
- Sahlin K., Broberg S. Release of K+ from muscle during prolonged dynamic exercise. Acta Physiol Scand. 1989 Jun;136(2):293–294. doi: 10.1111/j.1748-1716.1989.tb08666.x. [DOI] [PubMed] [Google Scholar]
- Sjøgaard G., Adams R. P., Saltin B. Water and ion shifts in skeletal muscle of humans with intense dynamic knee extension. Am J Physiol. 1985 Feb;248(2 Pt 2):R190–R196. doi: 10.1152/ajpregu.1985.248.2.R190. [DOI] [PubMed] [Google Scholar]
- Sjøgaard G., Saltin B. Extra- and intracellular water spaces in muscles of man at rest and with dynamic exercise. Am J Physiol. 1982 Sep;243(3):R271–R280. doi: 10.1152/ajpregu.1982.243.3.R271. [DOI] [PubMed] [Google Scholar]
- Vøllestad N. K., Blom P. C. Effect of varying exercise intensity on glycogen depletion in human muscle fibres. Acta Physiol Scand. 1985 Nov;125(3):395–405. doi: 10.1111/j.1748-1716.1985.tb07735.x. [DOI] [PubMed] [Google Scholar]
- Walløe L., Wesche J. Time course and magnitude of blood flow changes in the human quadriceps muscles during and following rhythmic exercise. J Physiol. 1988 Nov;405:257–273. doi: 10.1113/jphysiol.1988.sp017332. [DOI] [PMC free article] [PubMed] [Google Scholar]