Abstract
Sensitivity of voltage-activated calcium currents to flow-induced mechanical stress was examined in enriched populations of rat anterior pituitary somatotrophs. Voltage-activated calcium currents were recorded with the whole-cell configuration of the patch-clamp technique. Pituitary cells were exposed to flow (from pipettes) which was produced by a hydrostatic pressure of about 3 cmH2O. In 92% of the cells studied (n = 87 cells) flow reduced the amplitude of both low voltage-activated (LVA) and high voltage-activated (HVA) calcium currents. These effects of flow on calcium currents did not result from changes in either seal resistance or leak conductance of the cell and were dependent on the magnitude of flow. The effect of flow is selective. We found that LVA calcium currents were substantially more sensitive to flow than HVA calcium currents. Under constant flow conditions, LVA calcium currents were reduced by 57.6 +/- 29.6% (S.D.), whereas HVA currents (recorded from the same cells) were reduced by only 17.8 +/- 15.9% (S.D.). The effects of flow on calcium currents were associated with effects on their related calcium tail currents. Slowly deactivating calcium tail currents were reduced by 75.3 +/- 25.6% (S.D.), whereas rapidly deactivating calcium tail currents were reduced by 29.1 +/- 14.4% (S.D.). The effect of flow on calcium currents was not associated with any significant shift in the activation curves of the calcium currents (voltage range -60 to +30 mV), suggesting that the effect of flow is not voltage dependent. The effect of flow is not dependent on activation of calcium currents during the exposure to flow. Calcium currents which were evoked immediately after cessation of the exposure to flow were reduced in amplitude and recovered to control values. Possible mechanisms underlying the flow effect and possible physiological relevance of the effect on pituitary cells are discussed.
Full text
PDF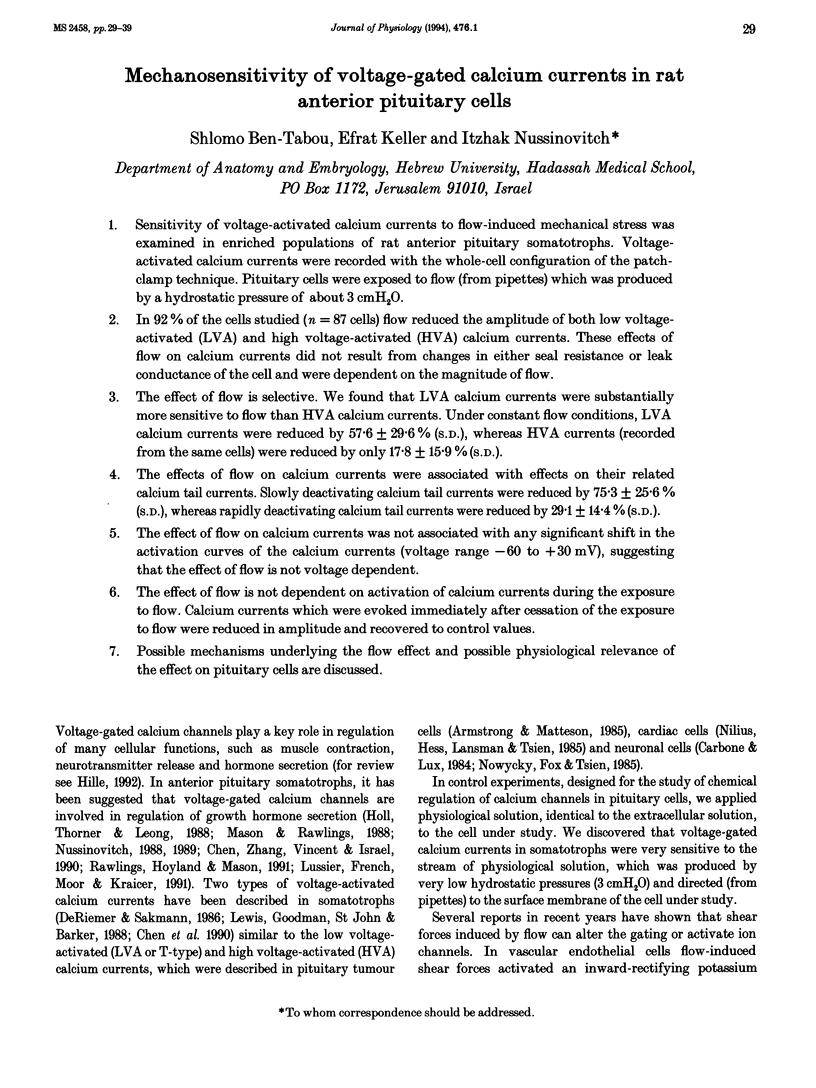
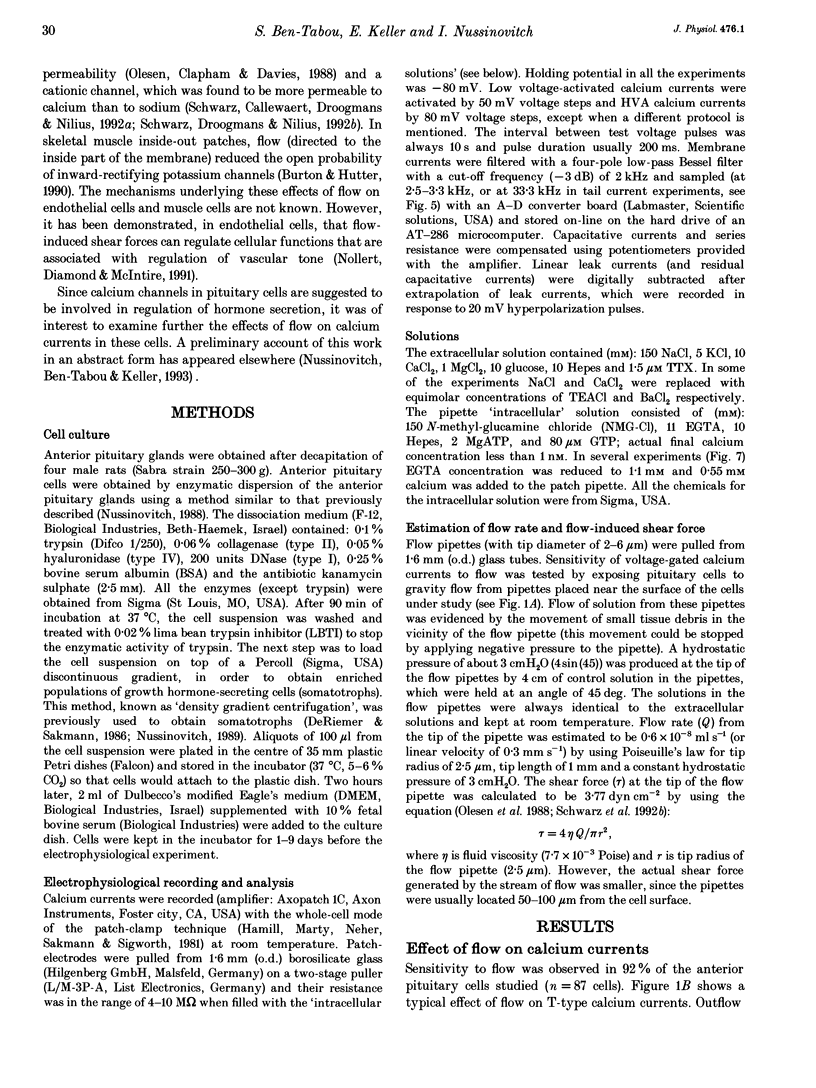
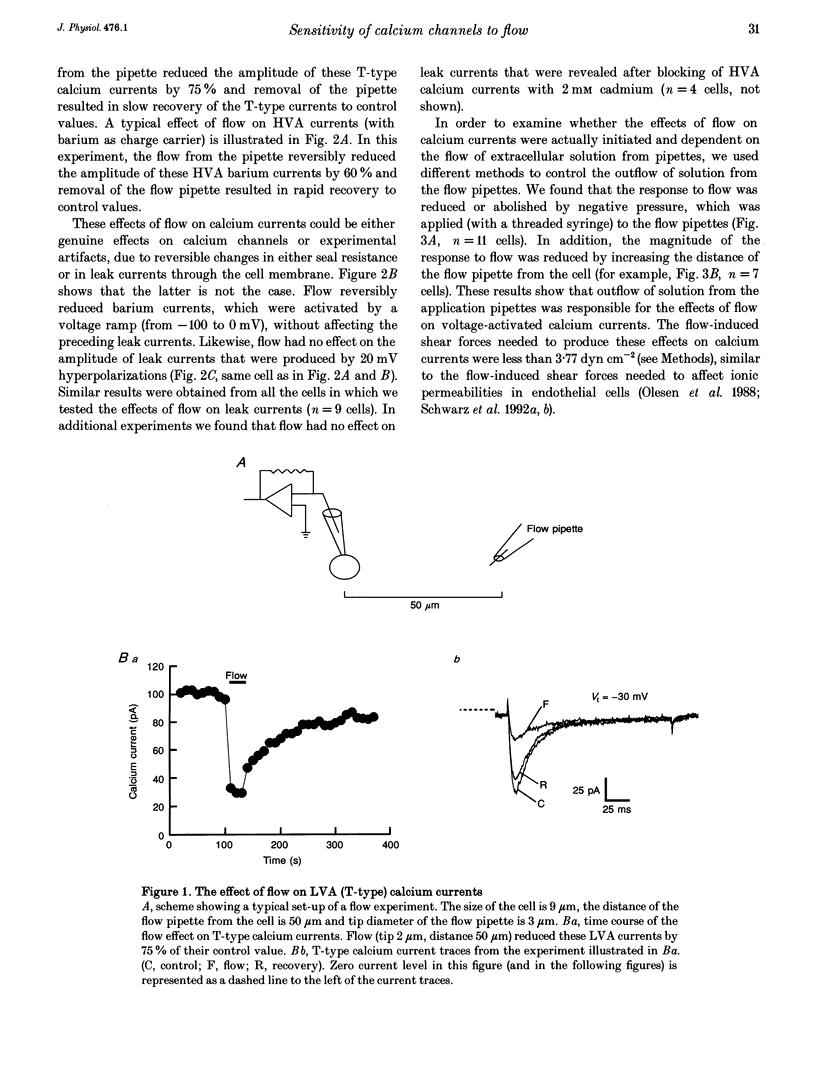
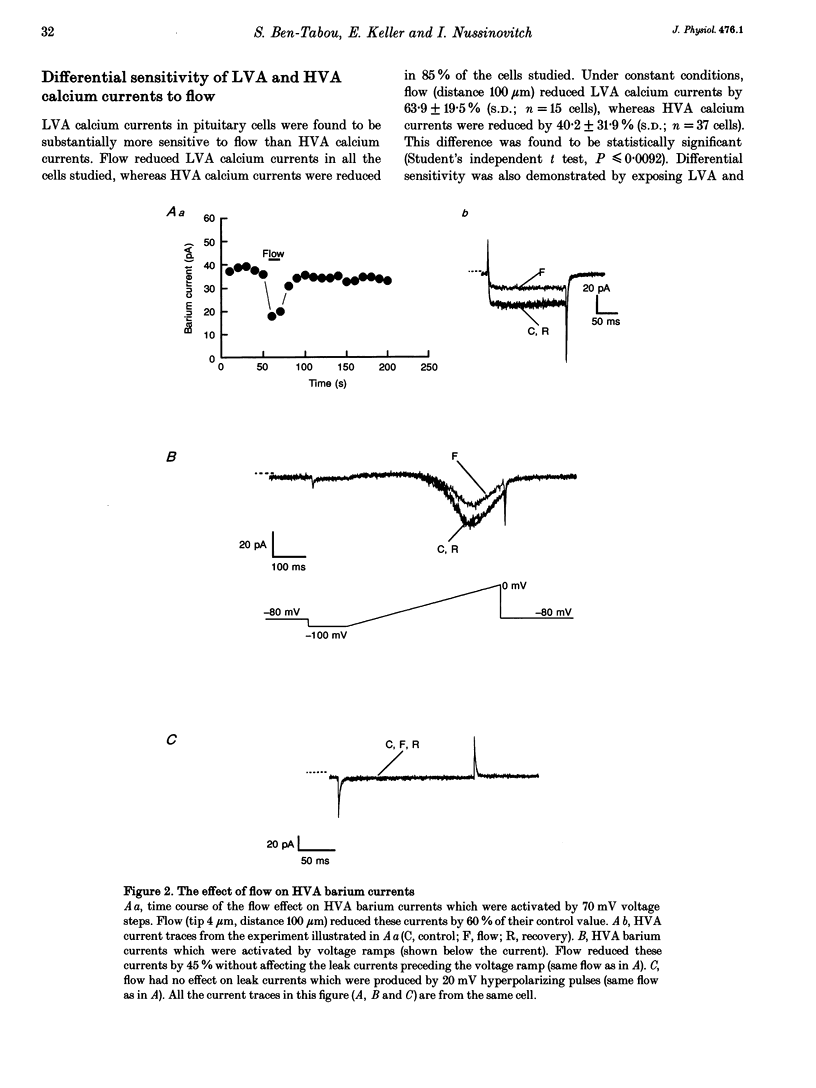
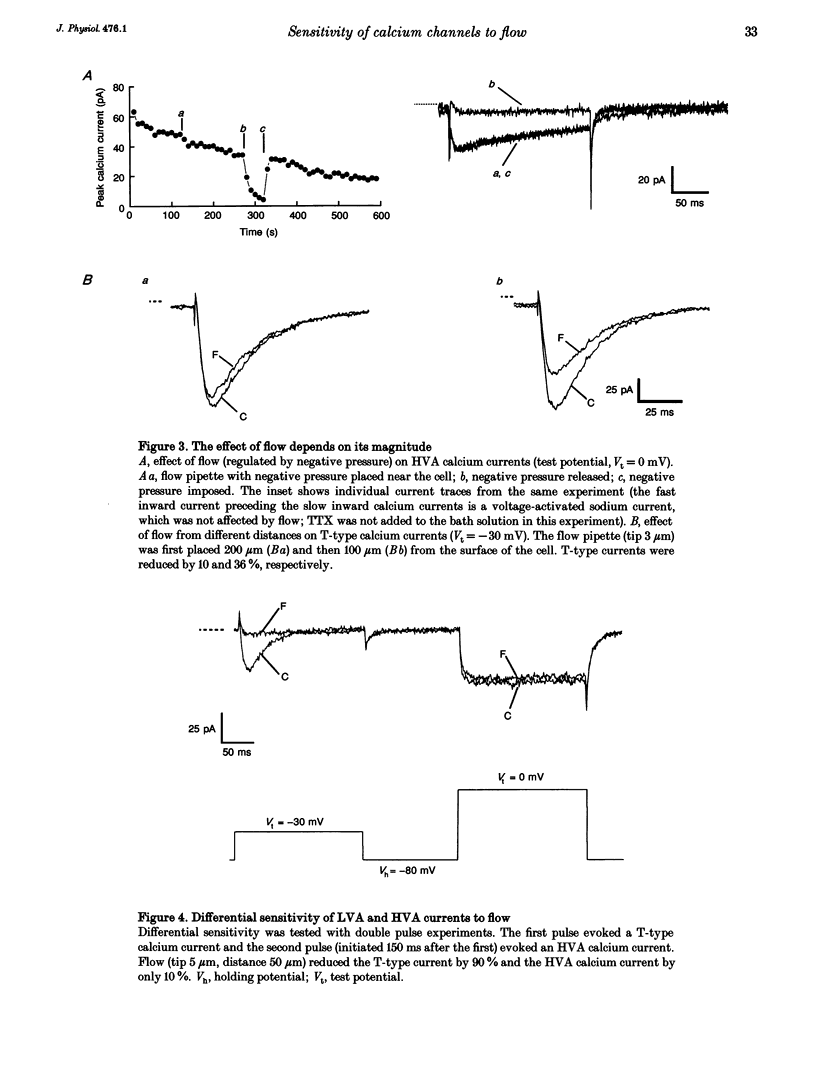
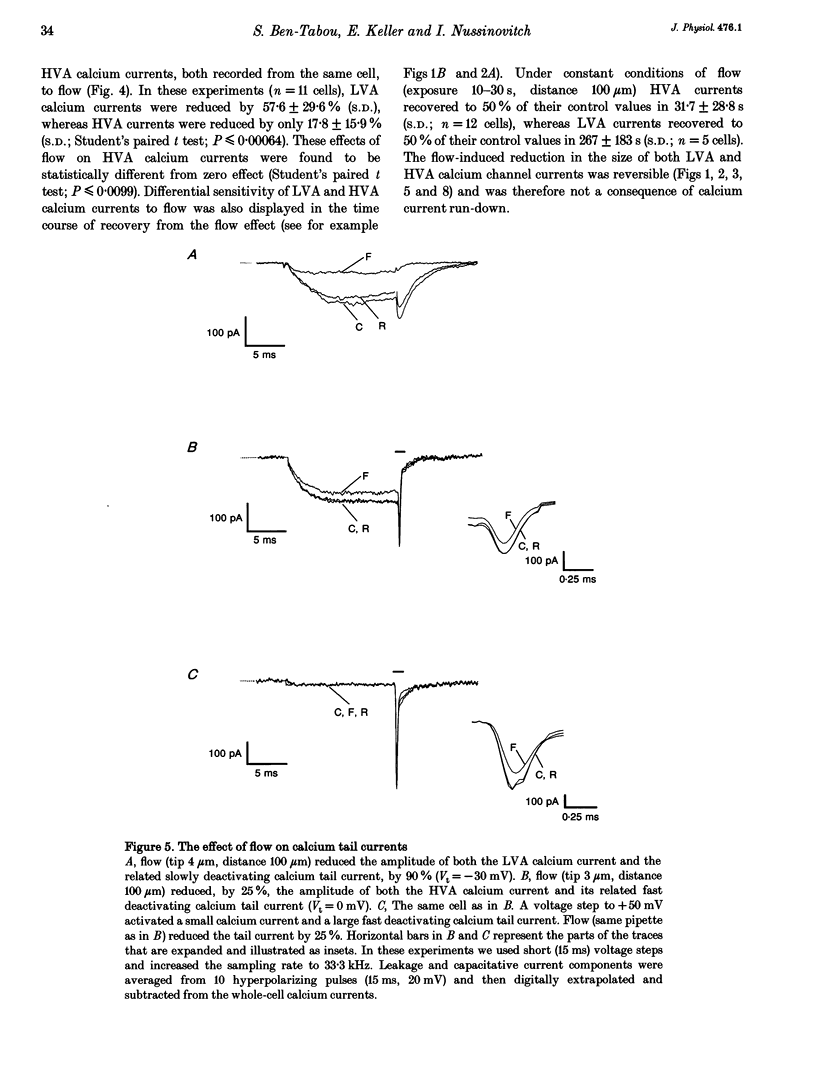
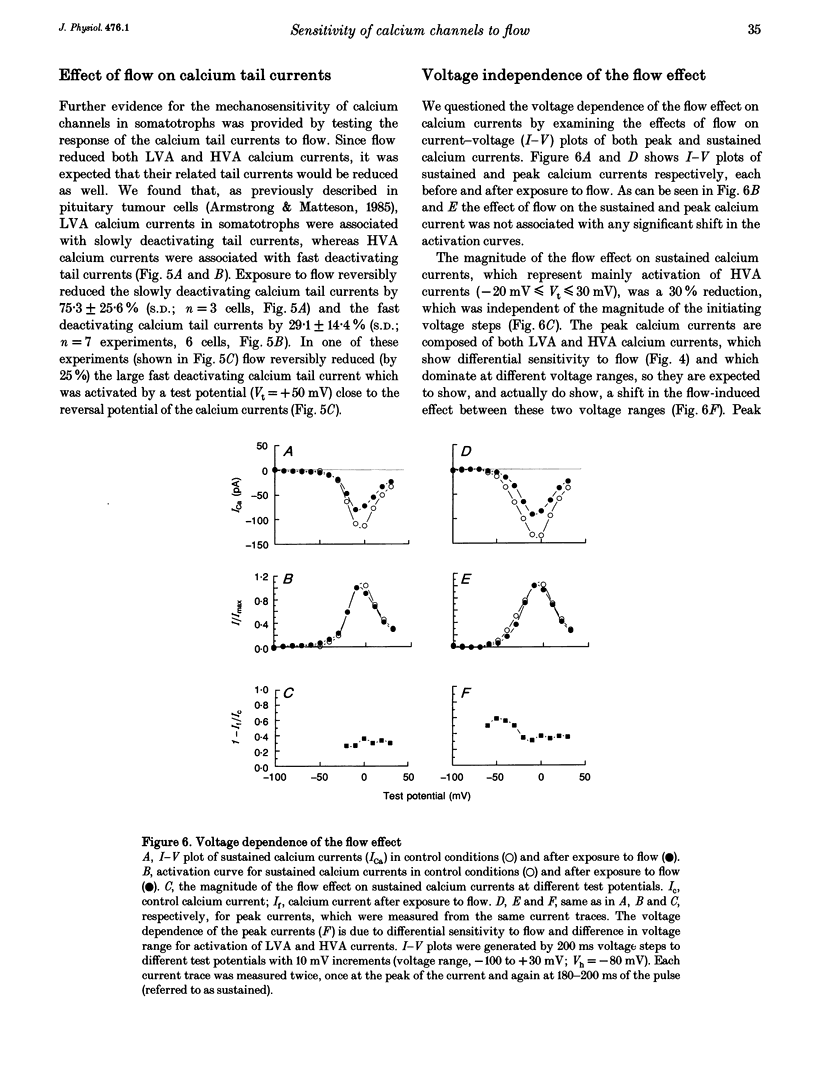
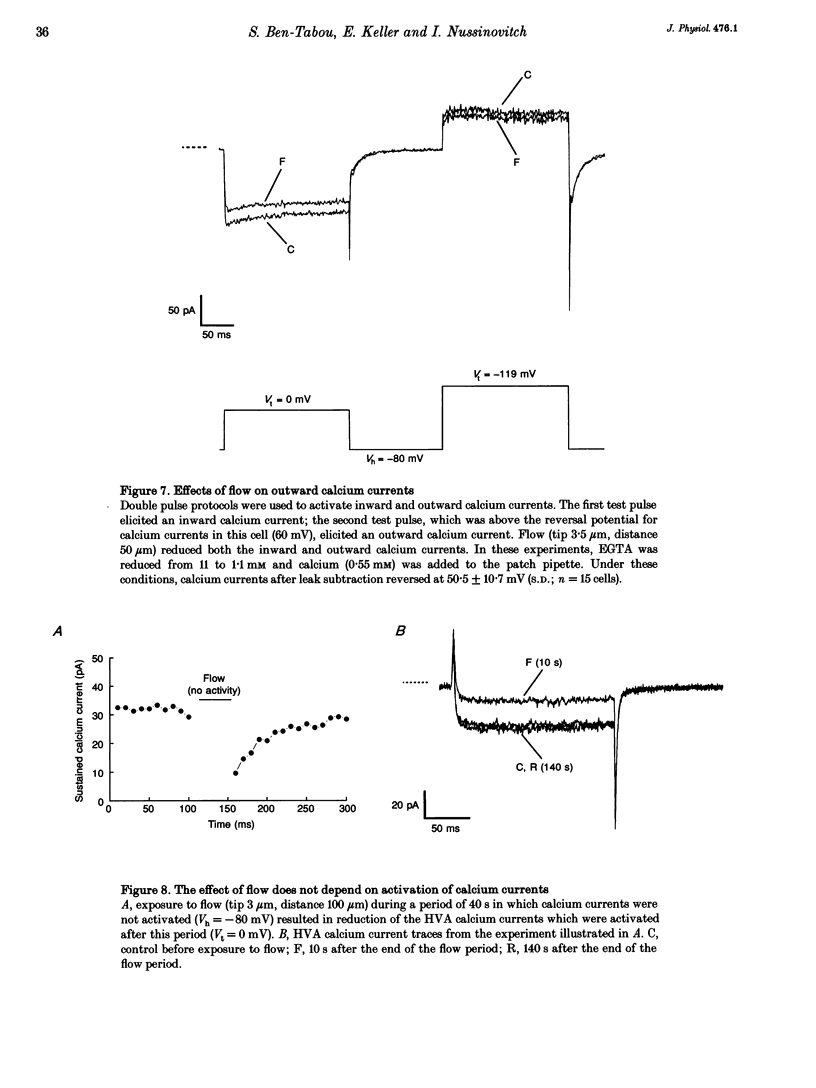
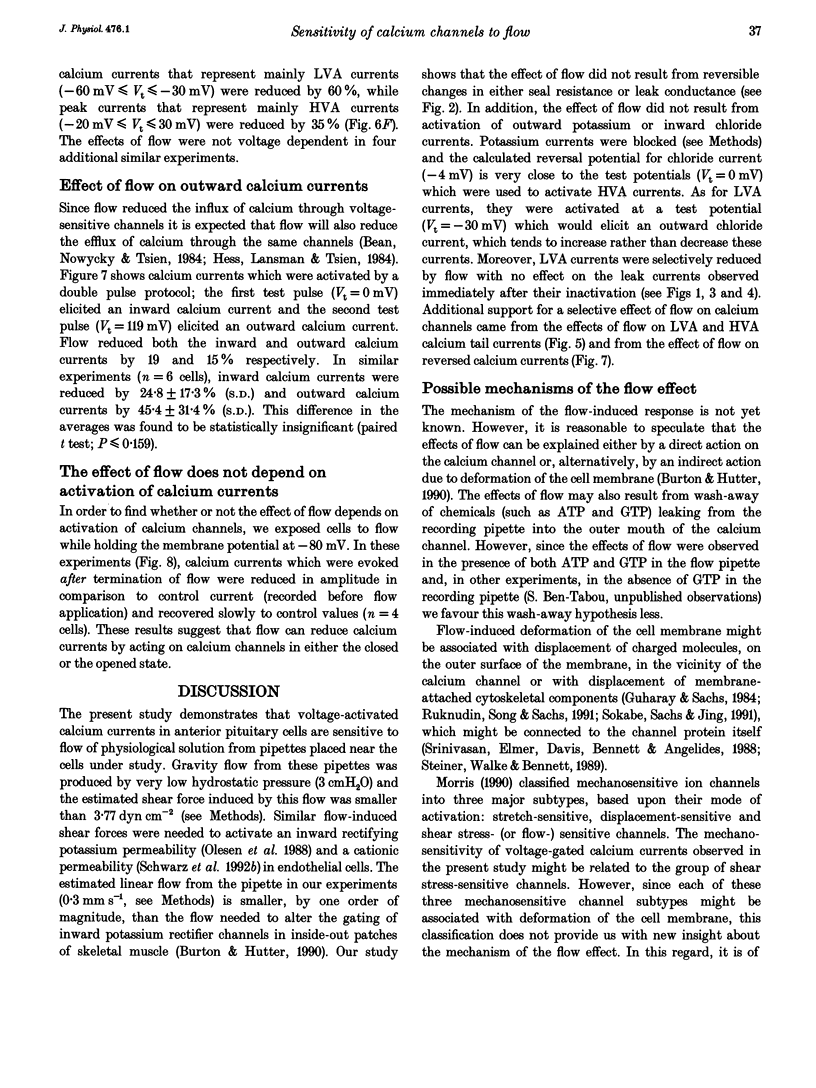
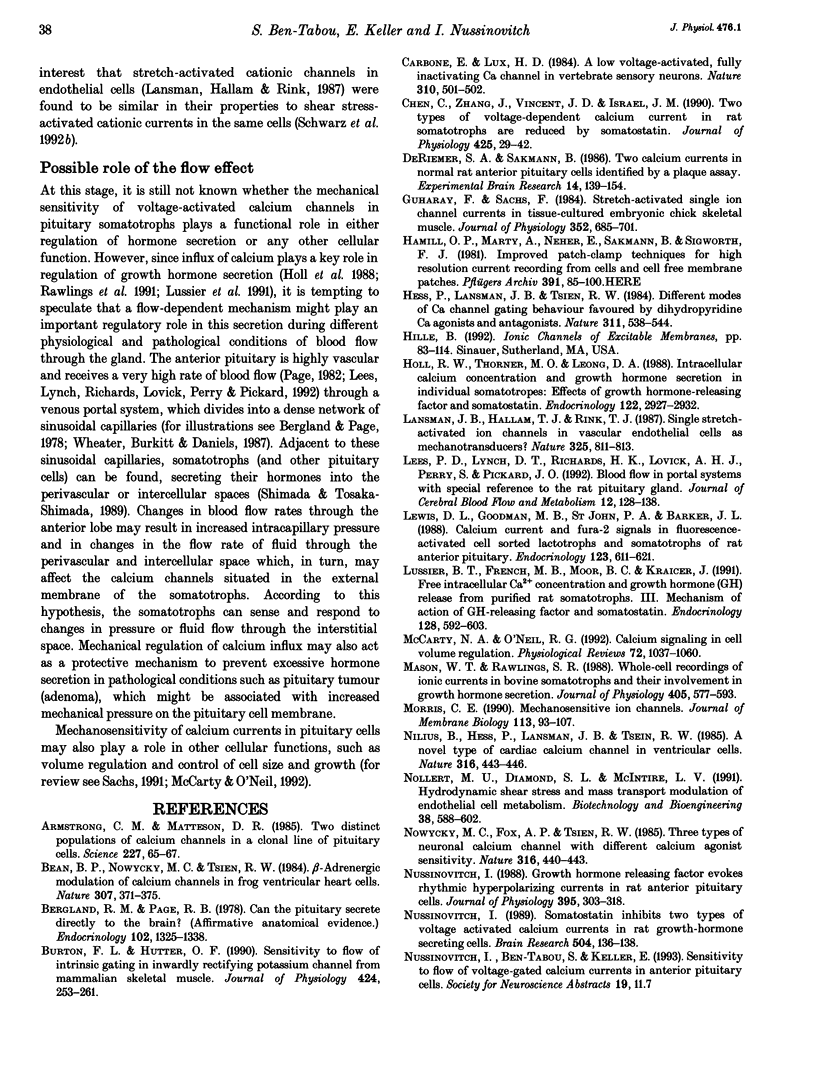
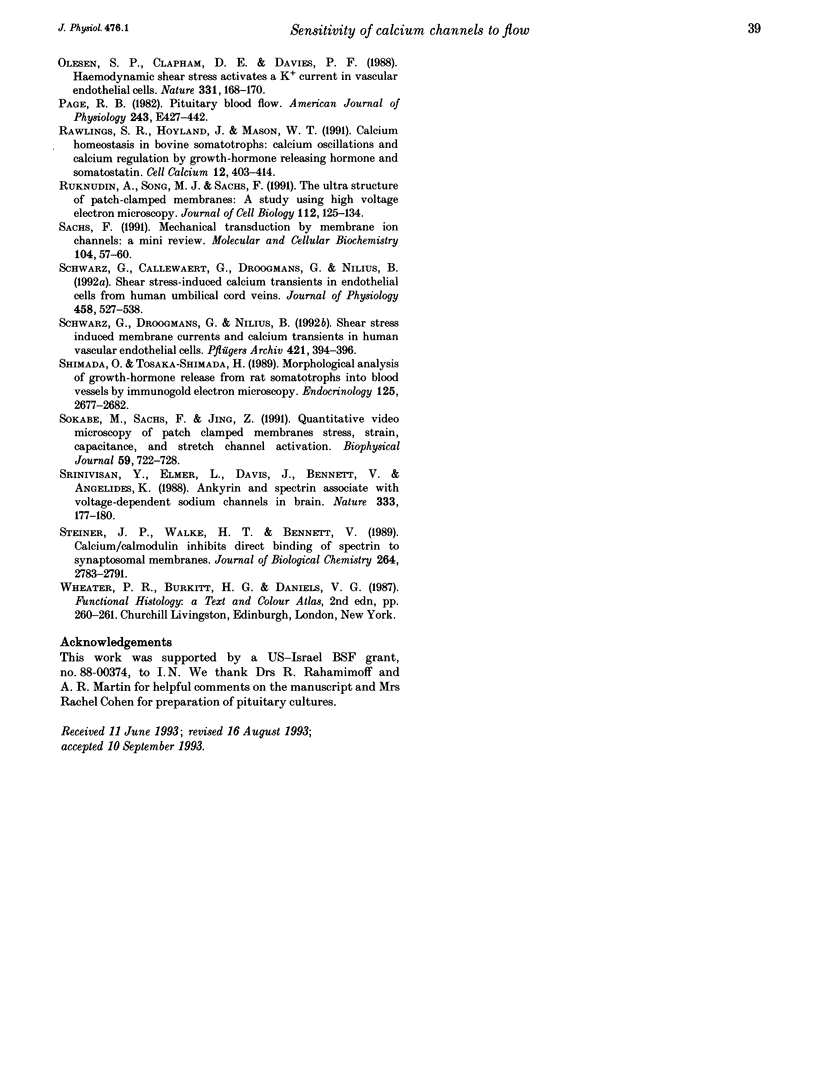
Selected References
These references are in PubMed. This may not be the complete list of references from this article.
- Armstrong C. M., Matteson D. R. Two distinct populations of calcium channels in a clonal line of pituitary cells. Science. 1985 Jan 4;227(4682):65–67. doi: 10.1126/science.2578071. [DOI] [PubMed] [Google Scholar]
- Bean B. P., Nowycky M. C., Tsien R. W. Beta-adrenergic modulation of calcium channels in frog ventricular heart cells. 1984 Jan 26-Feb 1Nature. 307(5949):371–375. doi: 10.1038/307371a0. [DOI] [PubMed] [Google Scholar]
- Bergland R. M., Page R. B. Can the pituitary secrete directly to the brain? (Affirmative anatomical evidence). Endocrinology. 1978 May;102(5):1325–1338. doi: 10.1210/endo-102-5-1325. [DOI] [PubMed] [Google Scholar]
- Burton F. L., Hutter O. F. Sensitivity to flow of intrinsic gating in inwardly rectifying potassium channel from mammalian skeletal muscle. J Physiol. 1990 May;424:253–261. doi: 10.1113/jphysiol.1990.sp018065. [DOI] [PMC free article] [PubMed] [Google Scholar]
- Carbone E., Lux H. D. A low voltage-activated, fully inactivating Ca channel in vertebrate sensory neurones. Nature. 1984 Aug 9;310(5977):501–502. doi: 10.1038/310501a0. [DOI] [PubMed] [Google Scholar]
- Chen C., Zhang J., Vincent J. D., Israel J. M. Two types of voltage-dependent calcium current in rat somatotrophs are reduced by somatostatin. J Physiol. 1990 Jun;425:29–42. doi: 10.1113/jphysiol.1990.sp018090. [DOI] [PMC free article] [PubMed] [Google Scholar]
- Guharay F., Sachs F. Stretch-activated single ion channel currents in tissue-cultured embryonic chick skeletal muscle. J Physiol. 1984 Jul;352:685–701. doi: 10.1113/jphysiol.1984.sp015317. [DOI] [PMC free article] [PubMed] [Google Scholar]
- Hamill O. P., Marty A., Neher E., Sakmann B., Sigworth F. J. Improved patch-clamp techniques for high-resolution current recording from cells and cell-free membrane patches. Pflugers Arch. 1981 Aug;391(2):85–100. doi: 10.1007/BF00656997. [DOI] [PubMed] [Google Scholar]
- Hess P., Lansman J. B., Tsien R. W. Different modes of Ca channel gating behaviour favoured by dihydropyridine Ca agonists and antagonists. Nature. 1984 Oct 11;311(5986):538–544. doi: 10.1038/311538a0. [DOI] [PubMed] [Google Scholar]
- Holl R. W., Thorner M. O., Leong D. A. Intracellular calcium concentration and growth hormone secretion in individual somatotropes: effects of growth hormone-releasing factor and somatostatin. Endocrinology. 1988 Jun;122(6):2927–2932. doi: 10.1210/endo-122-6-2927. [DOI] [PubMed] [Google Scholar]
- Lansman J. B., Hallam T. J., Rink T. J. Single stretch-activated ion channels in vascular endothelial cells as mechanotransducers? 1987 Feb 26-Mar 4Nature. 325(6107):811–813. doi: 10.1038/325811a0. [DOI] [PubMed] [Google Scholar]
- Lees P. D., Lynch D. T., Richards H. K., Lovick A. H., Perry S., Pickard J. D. Blood flow in portal systems with special reference to the rat pituitary gland. J Cereb Blood Flow Metab. 1992 Jan;12(1):128–138. doi: 10.1038/jcbfm.1992.16. [DOI] [PubMed] [Google Scholar]
- Lewis D. L., Goodman M. B., St John P. A., Barker J. L. Calcium currents and fura-2 signals in fluorescence-activated cell sorted lactotrophs and somatotrophs of rat anterior pituitary. Endocrinology. 1988 Jul;123(1):611–621. doi: 10.1210/endo-123-1-611. [DOI] [PubMed] [Google Scholar]
- Lussier B. T., French M. B., Moor B. C., Kraicer J. Free intracellular Ca2+ concentration and growth hormone (GH) release from purified rat somatotrophs. III. Mechanism of action of GH-releasing factor and somatostatin. Endocrinology. 1991 Jan;128(1):592–603. doi: 10.1210/endo-128-1-592. [DOI] [PubMed] [Google Scholar]
- Mason W. T., Rawlings S. R. Whole-cell recordings of ionic currents in bovine somatotrophs and their involvement in growth hormone secretion. J Physiol. 1988 Nov;405:577–593. doi: 10.1113/jphysiol.1988.sp017349. [DOI] [PMC free article] [PubMed] [Google Scholar]
- McCarty N. A., O'Neil R. G. Calcium signaling in cell volume regulation. Physiol Rev. 1992 Oct;72(4):1037–1061. doi: 10.1152/physrev.1992.72.4.1037. [DOI] [PubMed] [Google Scholar]
- Morris C. E. Mechanosensitive ion channels. J Membr Biol. 1990 Feb;113(2):93–107. doi: 10.1007/BF01872883. [DOI] [PubMed] [Google Scholar]
- Nilius B., Hess P., Lansman J. B., Tsien R. W. A novel type of cardiac calcium channel in ventricular cells. Nature. 1985 Aug 1;316(6027):443–446. doi: 10.1038/316443a0. [DOI] [PubMed] [Google Scholar]
- Nowycky M. C., Fox A. P., Tsien R. W. Three types of neuronal calcium channel with different calcium agonist sensitivity. Nature. 1985 Aug 1;316(6027):440–443. doi: 10.1038/316440a0. [DOI] [PubMed] [Google Scholar]
- Nussinovitch I. Growth hormone releasing factor evokes rhythmic hyperpolarizing currents in rat anterior pituitary cells. J Physiol. 1988 Jan;395:303–318. doi: 10.1113/jphysiol.1988.sp016920. [DOI] [PMC free article] [PubMed] [Google Scholar]
- Nussinovitch I. Somatostatin inhibits two types of voltage-activated calcium currents in rat growth-hormone secreting cells. Brain Res. 1989 Dec 11;504(1):136–138. doi: 10.1016/0006-8993(89)91610-7. [DOI] [PubMed] [Google Scholar]
- Olesen S. P., Clapham D. E., Davies P. F. Haemodynamic shear stress activates a K+ current in vascular endothelial cells. Nature. 1988 Jan 14;331(6152):168–170. doi: 10.1038/331168a0. [DOI] [PubMed] [Google Scholar]
- Page R. B. Pituitary blood flow. Am J Physiol. 1982 Dec;243(6):E427–E442. doi: 10.1152/ajpendo.1982.243.6.E427. [DOI] [PubMed] [Google Scholar]
- Rawlings S. R., Hoyland J., Mason W. T. Calcium homeostasis in bovine somatotrophs: calcium oscillations and calcium regulation by growth hormone-releasing hormone and somatostatin. Cell Calcium. 1991 Jun;12(6):403–414. doi: 10.1016/0143-4160(91)90066-n. [DOI] [PubMed] [Google Scholar]
- Ruknudin A., Song M. J., Sachs F. The ultrastructure of patch-clamped membranes: a study using high voltage electron microscopy. J Cell Biol. 1991 Jan;112(1):125–134. doi: 10.1083/jcb.112.1.125. [DOI] [PMC free article] [PubMed] [Google Scholar]
- Schwarz G., Callewaert G., Droogmans G., Nilius B. Shear stress-induced calcium transients in endothelial cells from human umbilical cord veins. J Physiol. 1992 Dec;458:527–538. doi: 10.1113/jphysiol.1992.sp019432. [DOI] [PMC free article] [PubMed] [Google Scholar]
- Schwarz G., Droogmans G., Nilius B. Shear stress induced membrane currents and calcium transients in human vascular endothelial cells. Pflugers Arch. 1992 Jul;421(4):394–396. doi: 10.1007/BF00374230. [DOI] [PubMed] [Google Scholar]
- Shimada O., Tosaka-Shimada H. Morphological analysis of growth hormone release from rat somatotrophs into blood vessels by immunogold electron microscopy. Endocrinology. 1989 Nov;125(5):2677–2682. doi: 10.1210/endo-125-5-2677. [DOI] [PubMed] [Google Scholar]
- Sokabe M., Sachs F., Jing Z. Q. Quantitative video microscopy of patch clamped membranes stress, strain, capacitance, and stretch channel activation. Biophys J. 1991 Mar;59(3):722–728. doi: 10.1016/S0006-3495(91)82285-8. [DOI] [PMC free article] [PubMed] [Google Scholar]
- Srinivasan Y., Elmer L., Davis J., Bennett V., Angelides K. Ankyrin and spectrin associate with voltage-dependent sodium channels in brain. Nature. 1988 May 12;333(6169):177–180. doi: 10.1038/333177a0. [DOI] [PubMed] [Google Scholar]
- Steiner J. P., Walke H. T., Jr, Bennett V. Calcium/calmodulin inhibits direct binding of spectrin to synaptosomal membranes. J Biol Chem. 1989 Feb 15;264(5):2783–2791. [PubMed] [Google Scholar]