Abstract
1. An apamin-sensitive Ca(2+)-activated K+ channel was characterized in turtle hair cells and utilized to monitor submembranous intracellular Ca2+ and to evaluate the concentration of the mobile endogenous calcium buffer. 2. Isolated hair cells were voltage clamped with whole-cell patch electrodes filled with a Cs(+)-based intracellular solution to block the large-conductance Ca(2+)-activated K+ (BK) channel. Ca2+ currents evoked by depolarization were followed by inward tail currents lasting several hundred milliseconds. Both the Ca2+ current and slow tail current were abolished by nifedipine. 3. The tail current was carried by K+ and Cs+ (relative permeabilities PCa/PK = 0.22), and was fully blocked by 0.1 microM apamin and half blocked by 5 mM external TEA. These properties suggest the tail current flows through a Ca(2+)-activated K+ channel distinct from the BK channels. 4. Intracellular Ca2+ was imaged with a confocal microscope in hair cells filled with the indicator Calcium Green-5N introduced via the patch pipette. Increases in Ca2+ evoked by depolarization were localized to hotspots on the basolateral surface of the cell. The time course of the tail current closely matched the fast component of the fluorescenece monitored at a hotspot. 5. Ca(2+)-ATPase pump inhibitors thapsigargin, 2,4-di-(t-butyl)hydroquinone (BHQ) and vanadate, which are known to influence calcium regulation in turtle hair cells, prolonged the time course of the tail current, supporting the idea that the channel monitors cytoplasmic Ca2+. 6. The mobile endogenous buffer was estimated by combining perforated-patch and whole-cell recordings on a single cell. After recording tail currents with an amphotericin-perforated patch, the patch was ruptured to obtain the whole-cell mode, thus allowing washout of soluble cytoplasmic proteins and exchange with pipette buffers. By varying the concentration of Ca2+ buffer in the pipette, the mobile endogenous buffer was found to be equivalent to about 1 mM BAPTA.
Full text
PDF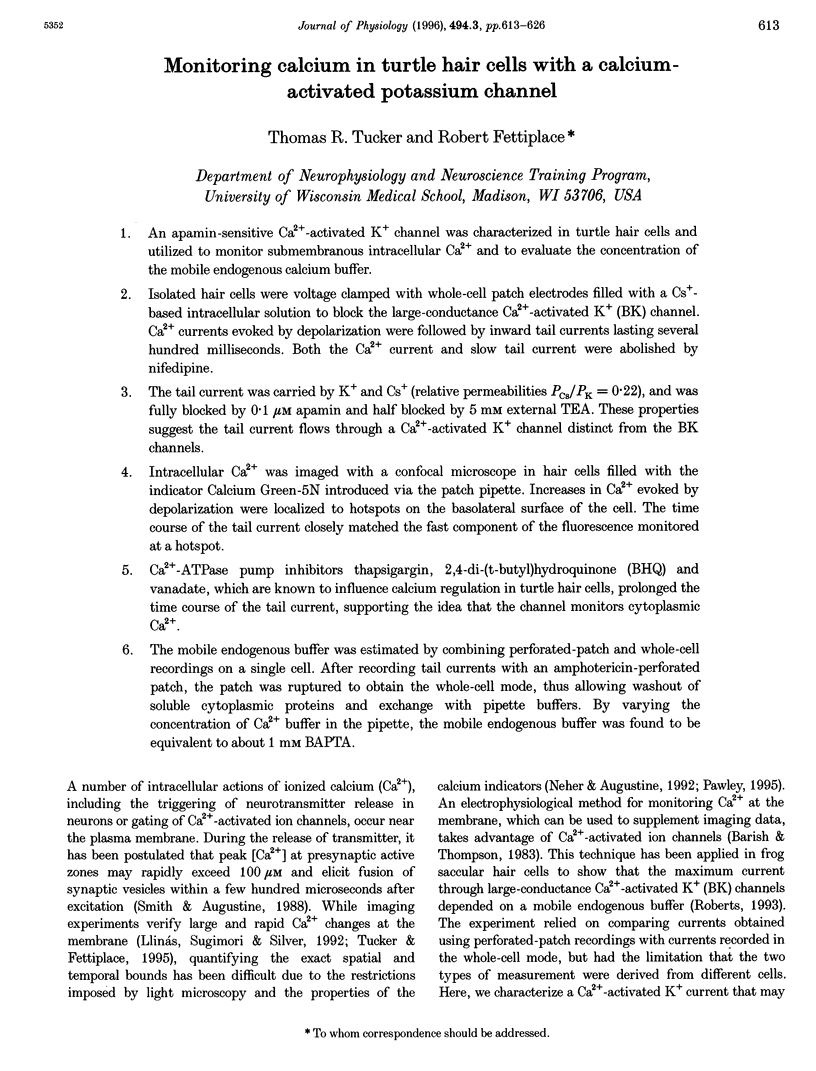
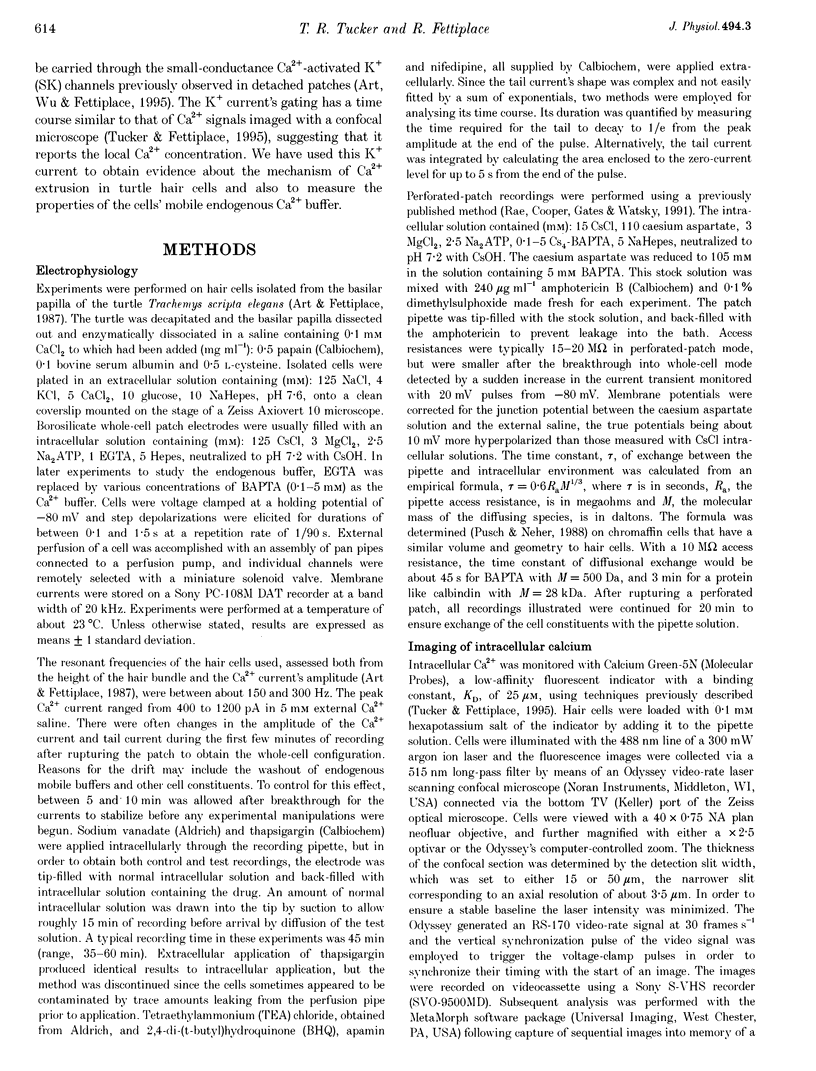
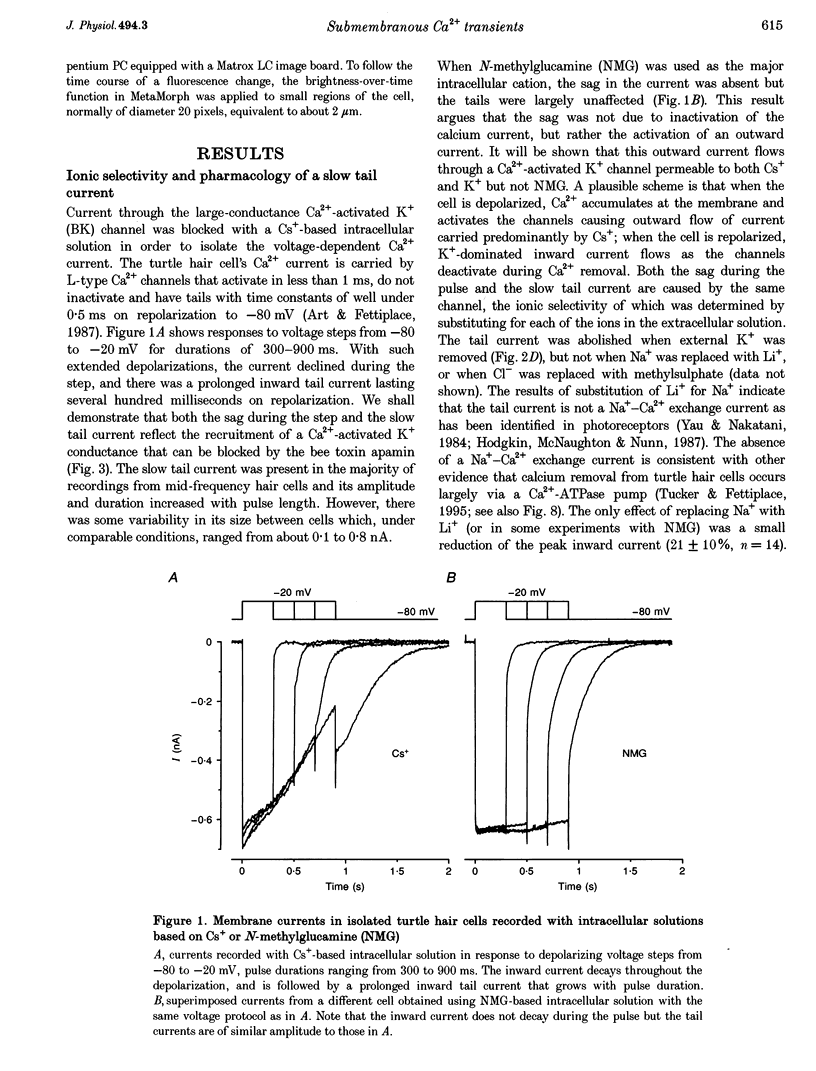
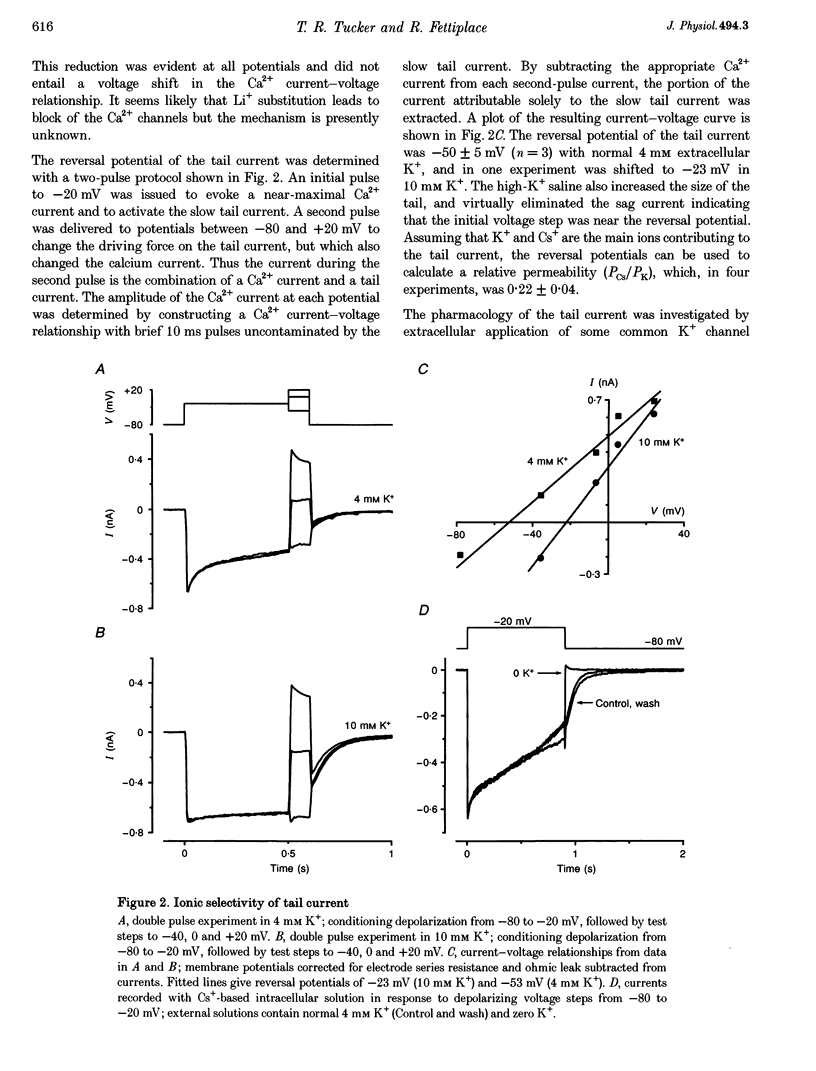
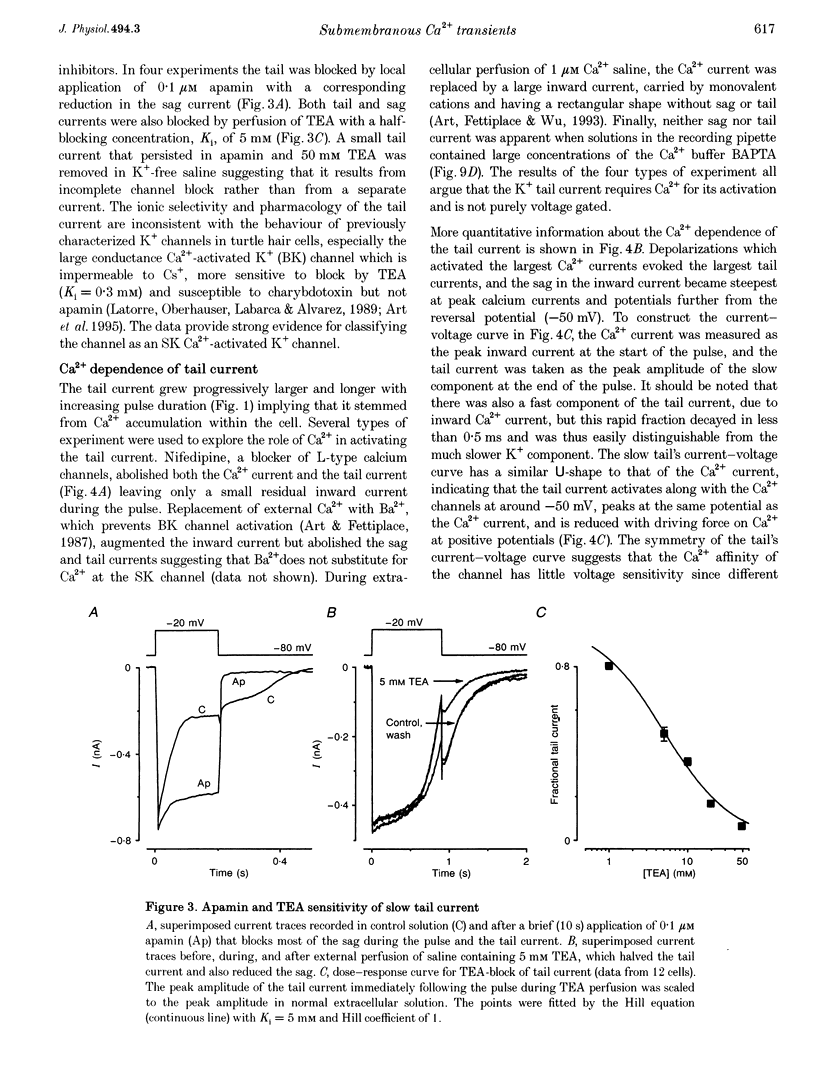
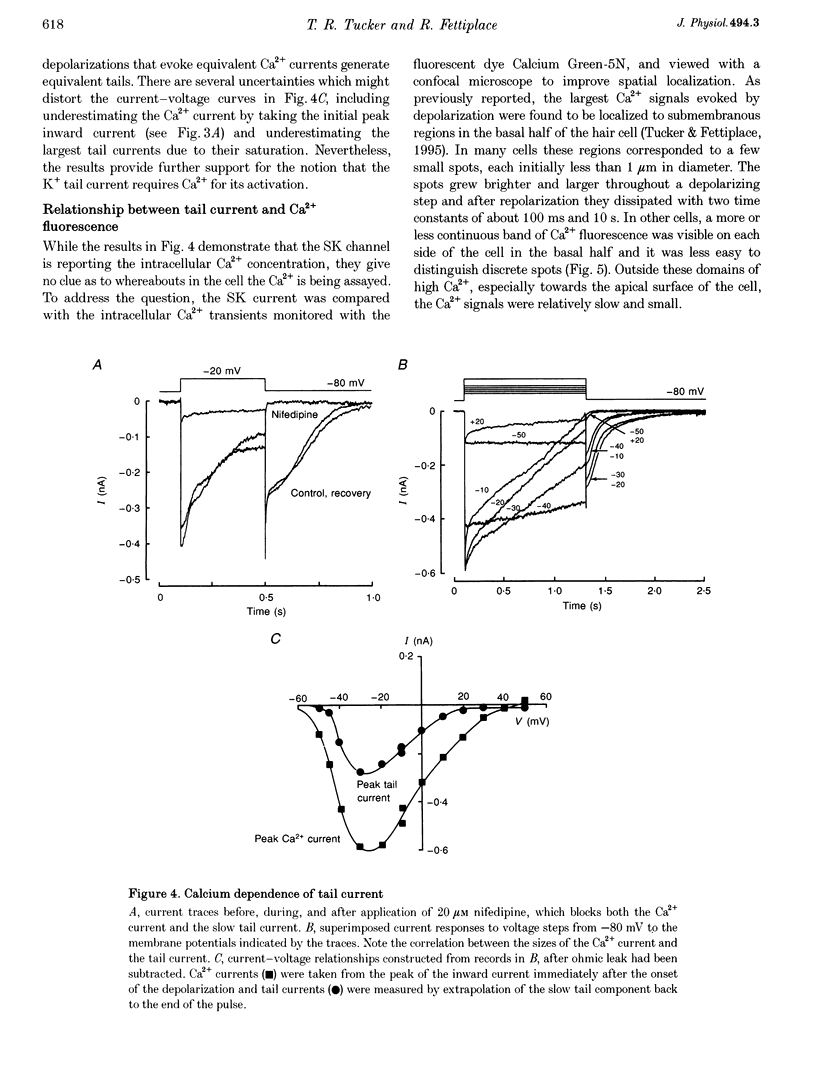
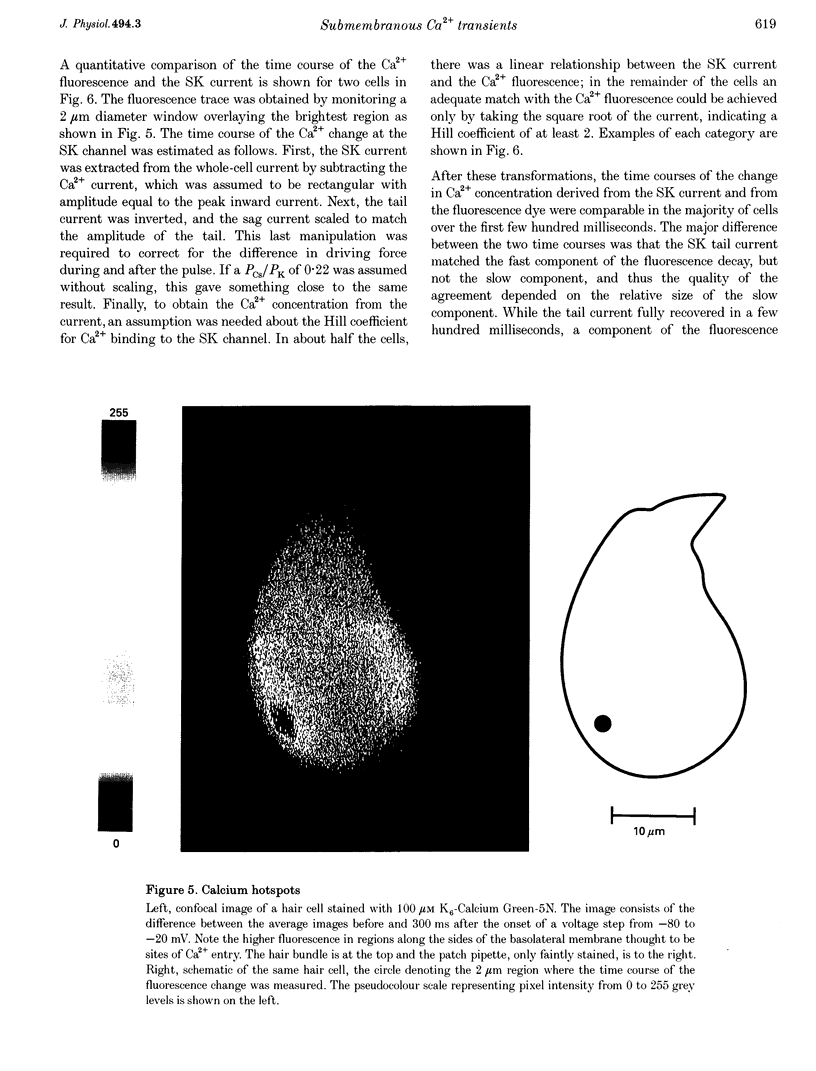
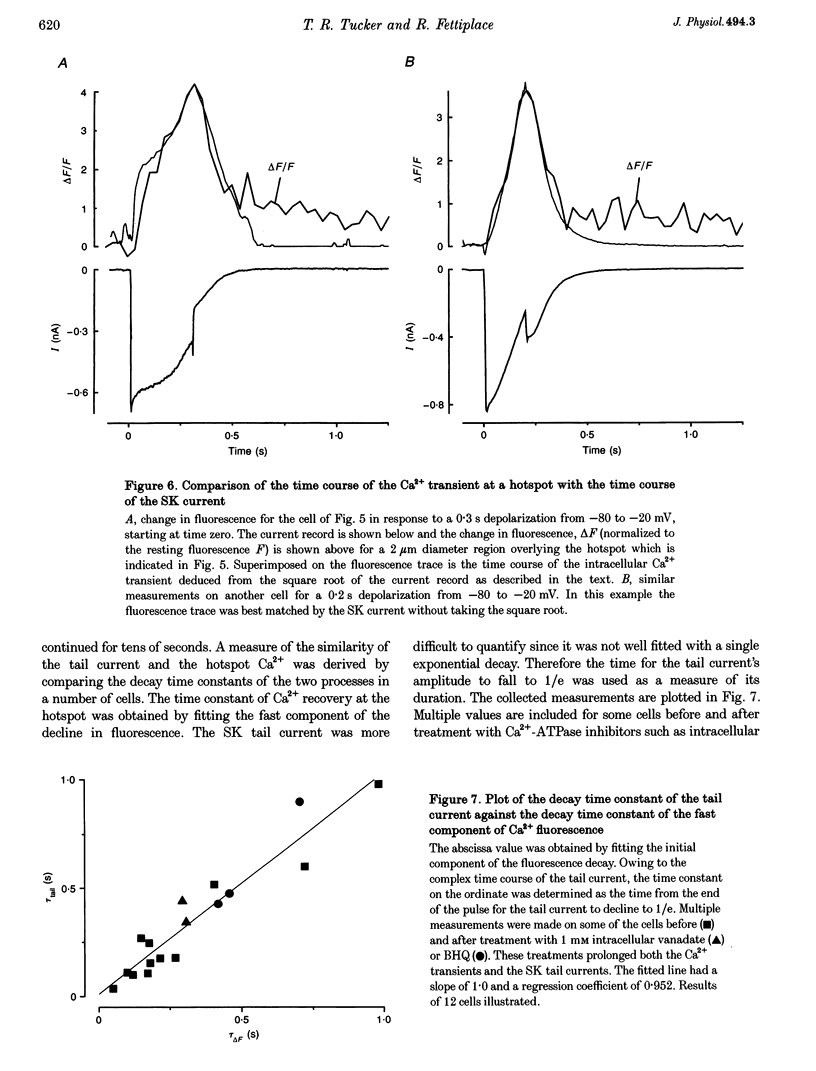
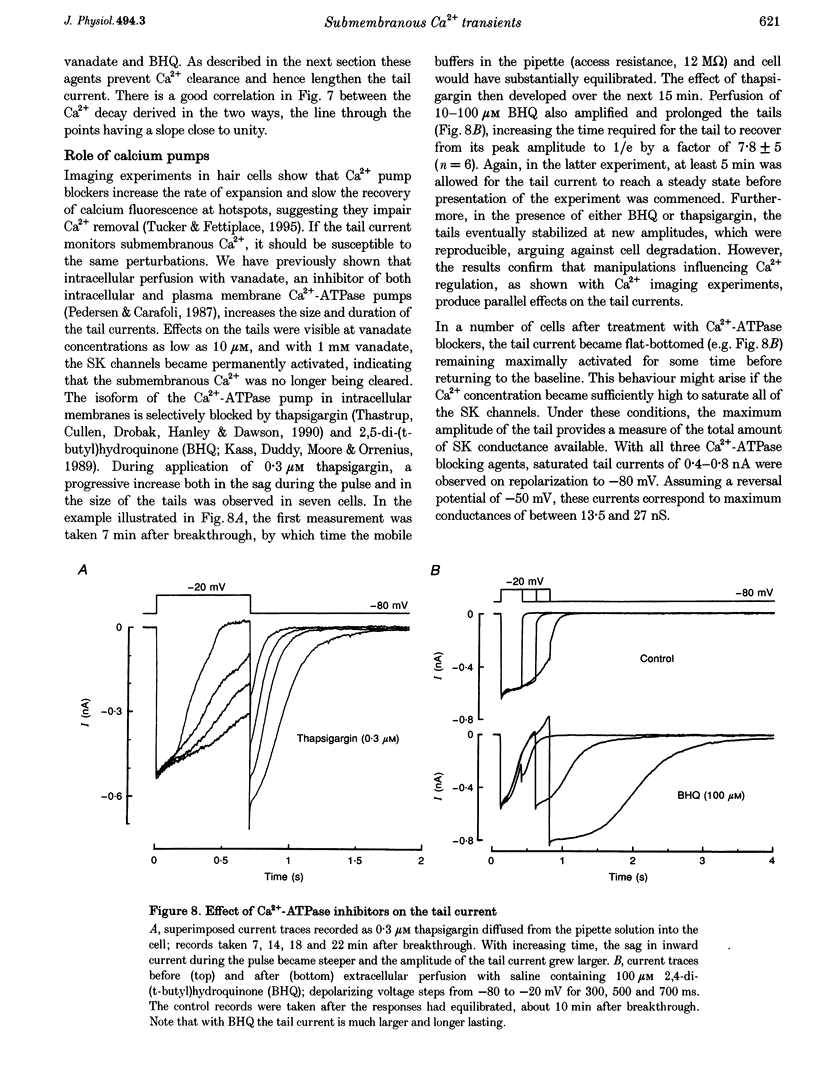
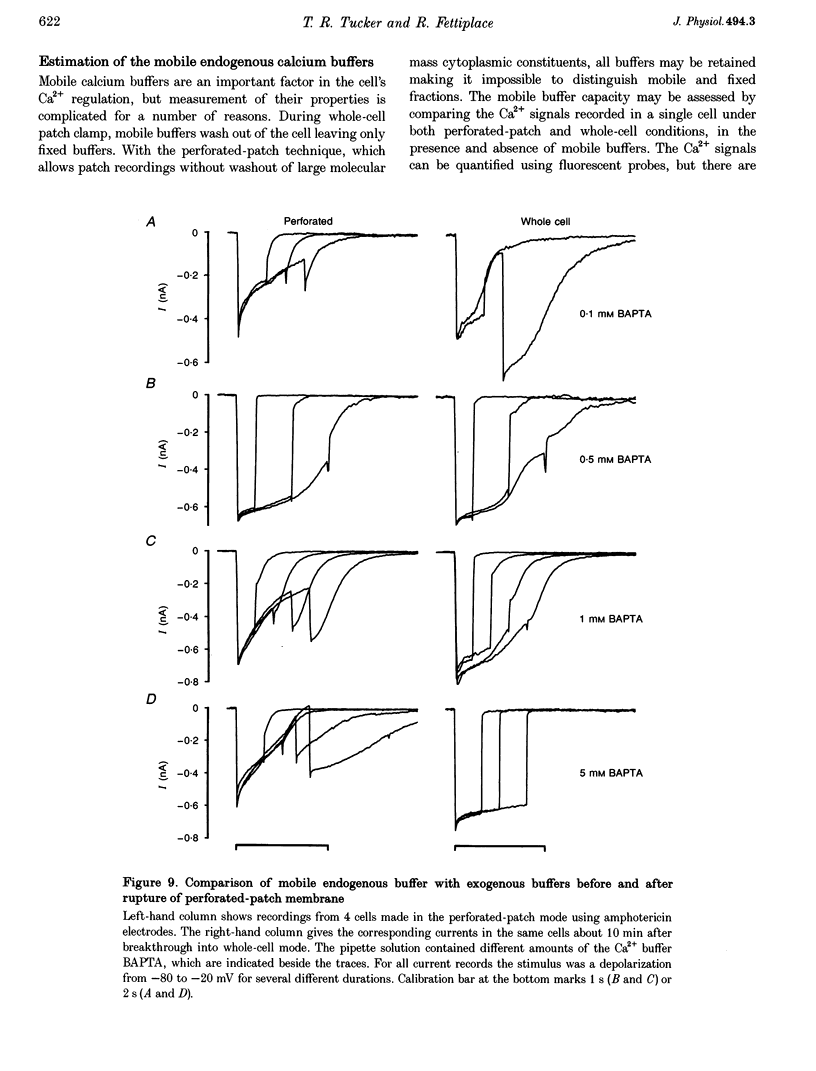
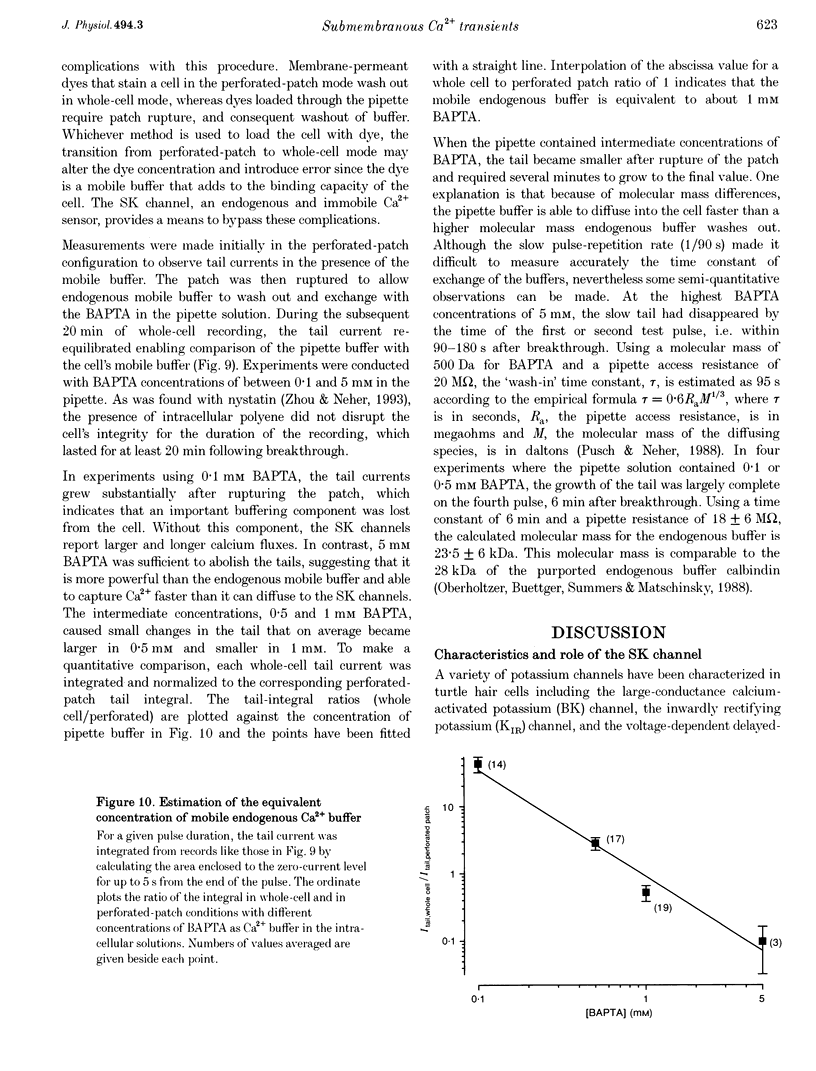
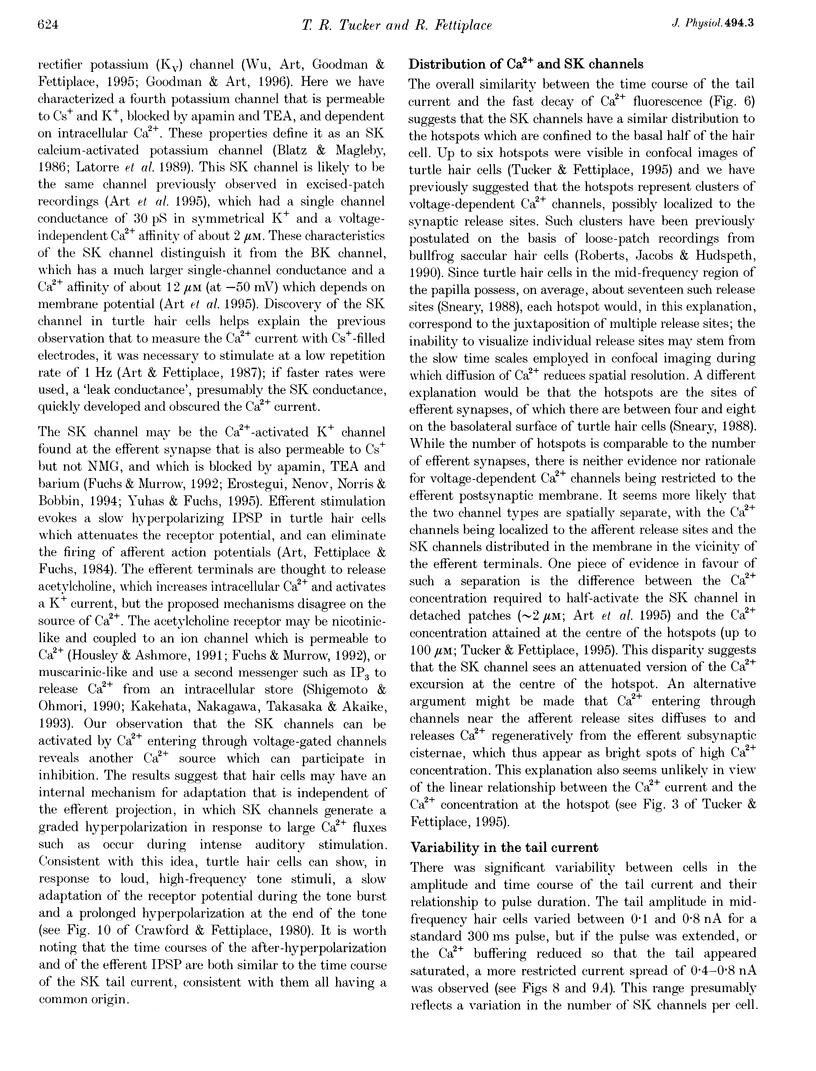
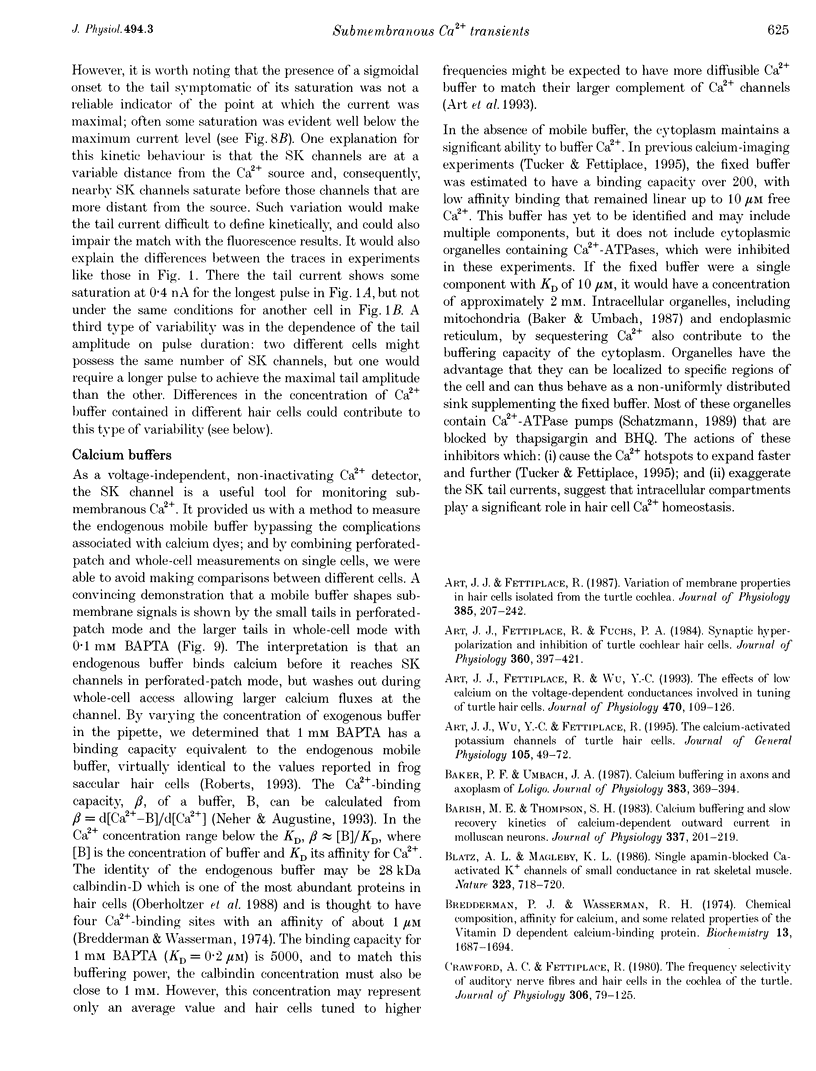
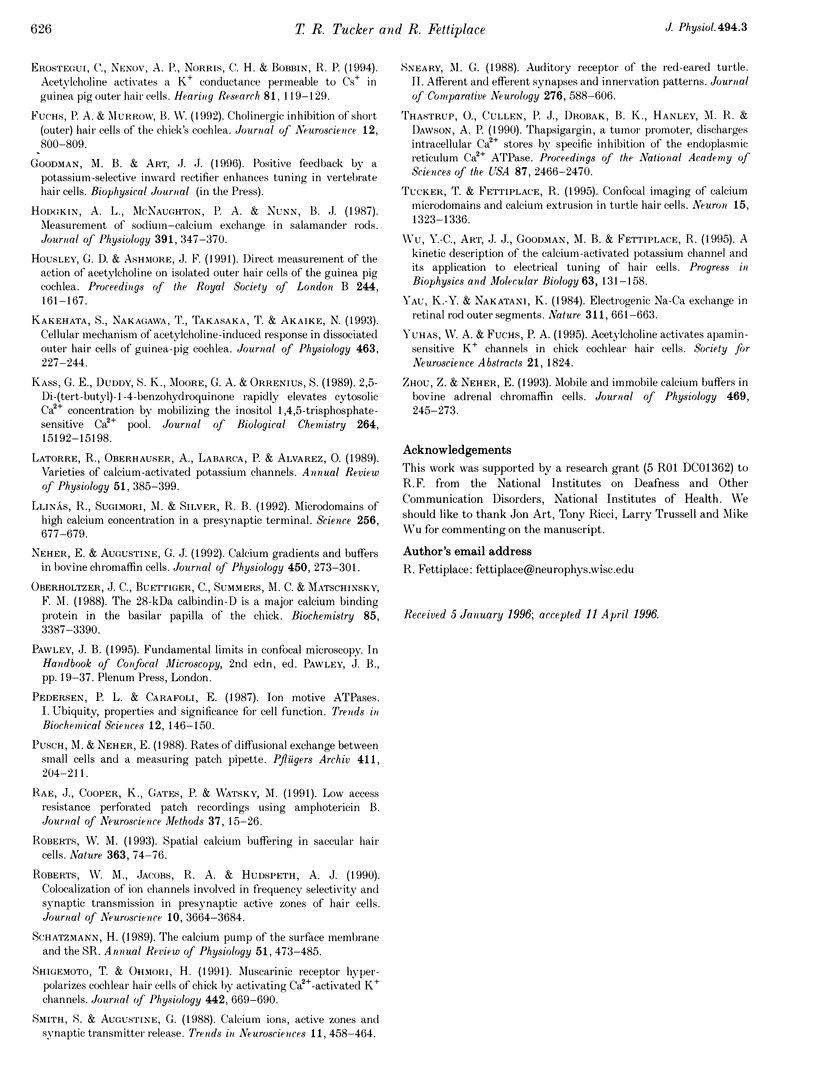
Images in this article
Selected References
These references are in PubMed. This may not be the complete list of references from this article.
- Art J. J., Fettiplace R. Variation of membrane properties in hair cells isolated from the turtle cochlea. J Physiol. 1987 Apr;385:207–242. doi: 10.1113/jphysiol.1987.sp016492. [DOI] [PMC free article] [PubMed] [Google Scholar]
- Art J. J., Fettiplace R., Wu Y. C. The effects of low calcium on the voltage-dependent conductances involved in tuning of turtle hair cells. J Physiol. 1993 Oct;470:109–126. doi: 10.1113/jphysiol.1993.sp019850. [DOI] [PMC free article] [PubMed] [Google Scholar]
- Art J. J., Wu Y. C., Fettiplace R. The calcium-activated potassium channels of turtle hair cells. J Gen Physiol. 1995 Jan;105(1):49–72. doi: 10.1085/jgp.105.1.49. [DOI] [PMC free article] [PubMed] [Google Scholar]
- Baker P. F., Umbach J. A. Calcium buffering in axons and axoplasm of Loligo. J Physiol. 1987 Feb;383:369–394. doi: 10.1113/jphysiol.1987.sp016414. [DOI] [PMC free article] [PubMed] [Google Scholar]
- Barish M. E., Thompson S. H. Calcium buffering and slow recovery kinetics of calcium-dependent outward current in molluscan neurones. J Physiol. 1983 Apr;337:201–219. doi: 10.1113/jphysiol.1983.sp014620. [DOI] [PMC free article] [PubMed] [Google Scholar]
- Blatz A. L., Magleby K. L. Single apamin-blocked Ca-activated K+ channels of small conductance in cultured rat skeletal muscle. Nature. 1986 Oct 23;323(6090):718–720. doi: 10.1038/323718a0. [DOI] [PubMed] [Google Scholar]
- Bredderman P. J., Wasserman R. H. Chemical composition, affinity for calcium, and some related properties of the vitamin D dependent calcium-binding protein. Biochemistry. 1974 Apr 9;13(8):1687–1694. doi: 10.1021/bi00705a021. [DOI] [PubMed] [Google Scholar]
- Crawford A. C., Fettiplace R. The frequency selectivity of auditory nerve fibres and hair cells in the cochlea of the turtle. J Physiol. 1980 Sep;306:79–125. doi: 10.1113/jphysiol.1980.sp013387. [DOI] [PMC free article] [PubMed] [Google Scholar]
- Erostegui C., Nenov A. P., Norris C. H., Bobbin R. P. Acetylcholine activates a K+ conductance permeable to Cs+ in guinea pig outer hair cells. Hear Res. 1994 Dec;81(1-2):119–129. doi: 10.1016/0378-5955(94)90159-7. [DOI] [PubMed] [Google Scholar]
- Fuchs P. A., Murrow B. W. Cholinergic inhibition of short (outer) hair cells of the chick's cochlea. J Neurosci. 1992 Mar;12(3):800–809. doi: 10.1523/JNEUROSCI.12-03-00800.1992. [DOI] [PMC free article] [PubMed] [Google Scholar]
- Housley G. D., Ashmore J. F. Direct measurement of the action of acetylcholine on isolated outer hair cells of the guinea pig cochlea. Proc Biol Sci. 1991 May 22;244(1310):161–167. doi: 10.1098/rspb.1991.0065. [DOI] [PubMed] [Google Scholar]
- Kakehata S., Nakagawa T., Takasaka T., Akaike N. Cellular mechanism of acetylcholine-induced response in dissociated outer hair cells of guinea-pig cochlea. J Physiol. 1993 Apr;463:227–244. doi: 10.1113/jphysiol.1993.sp019592. [DOI] [PMC free article] [PubMed] [Google Scholar]
- Kass G. E., Duddy S. K., Moore G. A., Orrenius S. 2,5-Di-(tert-butyl)-1,4-benzohydroquinone rapidly elevates cytosolic Ca2+ concentration by mobilizing the inositol 1,4,5-trisphosphate-sensitive Ca2+ pool. J Biol Chem. 1989 Sep 15;264(26):15192–15198. [PubMed] [Google Scholar]
- Latorre R., Oberhauser A., Labarca P., Alvarez O. Varieties of calcium-activated potassium channels. Annu Rev Physiol. 1989;51:385–399. doi: 10.1146/annurev.ph.51.030189.002125. [DOI] [PubMed] [Google Scholar]
- Llinás R., Sugimori M., Silver R. B. Microdomains of high calcium concentration in a presynaptic terminal. Science. 1992 May 1;256(5057):677–679. doi: 10.1126/science.1350109. [DOI] [PubMed] [Google Scholar]
- Neher E., Augustine G. J. Calcium gradients and buffers in bovine chromaffin cells. J Physiol. 1992 May;450:273–301. doi: 10.1113/jphysiol.1992.sp019127. [DOI] [PMC free article] [PubMed] [Google Scholar]
- Oberholtzer J. C., Buettger C., Summers M. C., Matschinsky F. M. The 28-kDa calbindin-D is a major calcium-binding protein in the basilar papilla of the chick. Proc Natl Acad Sci U S A. 1988 May;85(10):3387–3390. doi: 10.1073/pnas.85.10.3387. [DOI] [PMC free article] [PubMed] [Google Scholar]
- Pusch M., Neher E. Rates of diffusional exchange between small cells and a measuring patch pipette. Pflugers Arch. 1988 Feb;411(2):204–211. doi: 10.1007/BF00582316. [DOI] [PubMed] [Google Scholar]
- Rae J., Cooper K., Gates P., Watsky M. Low access resistance perforated patch recordings using amphotericin B. J Neurosci Methods. 1991 Mar;37(1):15–26. doi: 10.1016/0165-0270(91)90017-t. [DOI] [PubMed] [Google Scholar]
- Roberts W. M., Jacobs R. A., Hudspeth A. J. Colocalization of ion channels involved in frequency selectivity and synaptic transmission at presynaptic active zones of hair cells. J Neurosci. 1990 Nov;10(11):3664–3684. doi: 10.1523/JNEUROSCI.10-11-03664.1990. [DOI] [PMC free article] [PubMed] [Google Scholar]
- Roberts W. M. Spatial calcium buffering in saccular hair cells. Nature. 1993 May 6;363(6424):74–76. doi: 10.1038/363074a0. [DOI] [PubMed] [Google Scholar]
- Schatzmann H. J. The calcium pump of the surface membrane and of the sarcoplasmic reticulum. Annu Rev Physiol. 1989;51:473–485. doi: 10.1146/annurev.ph.51.030189.002353. [DOI] [PubMed] [Google Scholar]
- Shigemoto T., Ohmori H. Muscarinic receptor hyperpolarizes cochlear hair cells of chick by activating Ca(2+)-activated K+ channels. J Physiol. 1991 Oct;442:669–690. doi: 10.1113/jphysiol.1991.sp018814. [DOI] [PMC free article] [PubMed] [Google Scholar]
- Smith S. J., Augustine G. J. Calcium ions, active zones and synaptic transmitter release. Trends Neurosci. 1988 Oct;11(10):458–464. doi: 10.1016/0166-2236(88)90199-3. [DOI] [PubMed] [Google Scholar]
- Sneary M. G. Auditory receptor of the red-eared turtle: II. Afferent and efferent synapses and innervation patterns. J Comp Neurol. 1988 Oct 22;276(4):588–606. doi: 10.1002/cne.902760411. [DOI] [PubMed] [Google Scholar]
- Thastrup O., Cullen P. J., Drøbak B. K., Hanley M. R., Dawson A. P. Thapsigargin, a tumor promoter, discharges intracellular Ca2+ stores by specific inhibition of the endoplasmic reticulum Ca2(+)-ATPase. Proc Natl Acad Sci U S A. 1990 Apr;87(7):2466–2470. doi: 10.1073/pnas.87.7.2466. [DOI] [PMC free article] [PubMed] [Google Scholar]
- Tucker T., Fettiplace R. Confocal imaging of calcium microdomains and calcium extrusion in turtle hair cells. Neuron. 1995 Dec;15(6):1323–1335. doi: 10.1016/0896-6273(95)90011-x. [DOI] [PubMed] [Google Scholar]
- Wu Y. C., Art J. J., Goodman M. B., Fettiplace R. A kinetic description of the calcium-activated potassium channel and its application to electrical tuning of hair cells. Prog Biophys Mol Biol. 1995;63(2):131–158. doi: 10.1016/0079-6107(95)00002-5. [DOI] [PubMed] [Google Scholar]
- Yau K. W., Nakatani K. Electrogenic Na-Ca exchange in retinal rod outer segment. Nature. 1984 Oct 18;311(5987):661–663. doi: 10.1038/311661a0. [DOI] [PubMed] [Google Scholar]
- Zhou Z., Neher E. Mobile and immobile calcium buffers in bovine adrenal chromaffin cells. J Physiol. 1993 Sep;469:245–273. doi: 10.1113/jphysiol.1993.sp019813. [DOI] [PMC free article] [PubMed] [Google Scholar]