Abstract
1. The effects of multivalent cations on membrane currents recorded from Xenopus oocytes were studied. 2. The hyperpolarization-activated chloride current was reversibly blocked by lanthanum; half-maximal block occurred at a concentration of 8 microM. Zinc, cadmium, cobalt and nickel were less potent than lanthanum, and gadolinium, manganese, barium and strontium had no effect at a concentration of 100 microM. 3. The calcium-activated chloride current was blocked by gadolinium (50 microM), and lanthanum, cadmium, cobalt, nickel and manganese were equally effective. The actions of gadolinium and lanthanum were almost irreversible, while partial (30-80%) recovery was observed with the other cations. Zinc (100 microM) had no effect. 4. In lanthanum (100 microM), membrane depolarizations from -70 mV activated an outward potassium current that was partially blocked by barium (0.1-2 mM). The barium-sensitive current was confined to potentials less negative than -70 mV. The current consisted of a time-independent as well as a time-dependent component, the latter of which had voltage dependence similar to the M-current. 5. It is proposed that lanthanum, gadolinium and barium can usefully separate these endogenous membrane currents in Xenopus oocytes.
Full text
PDF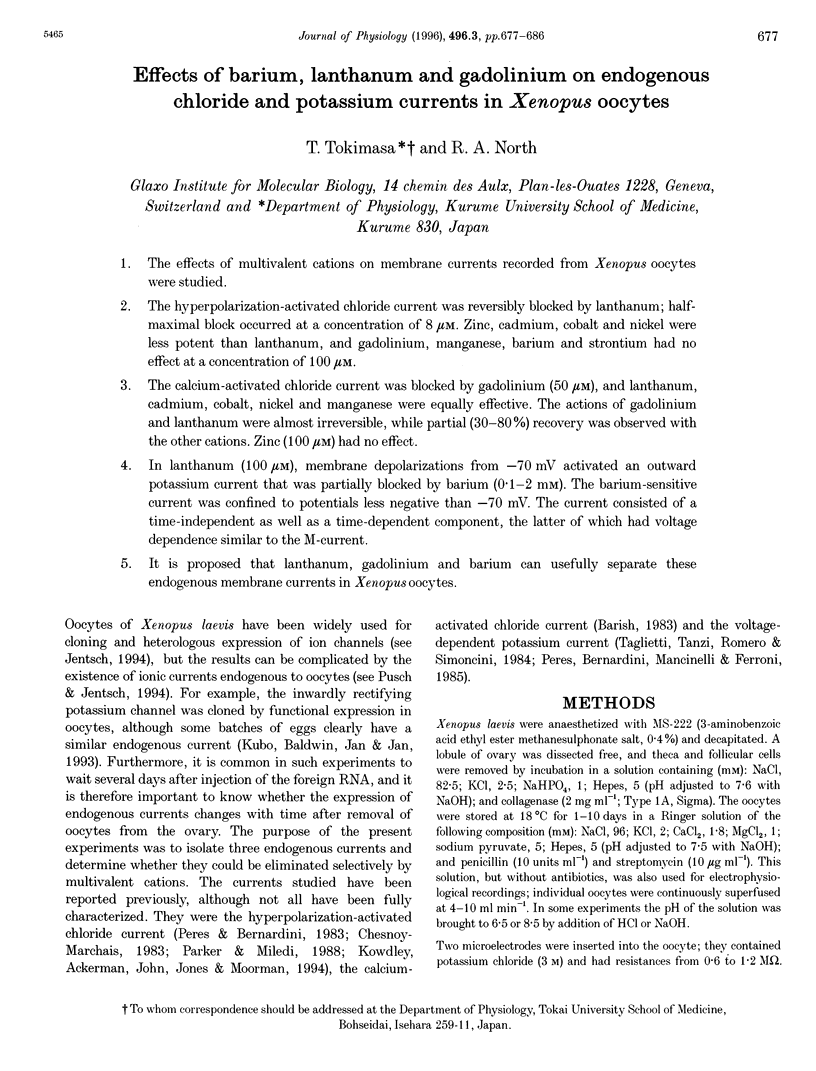
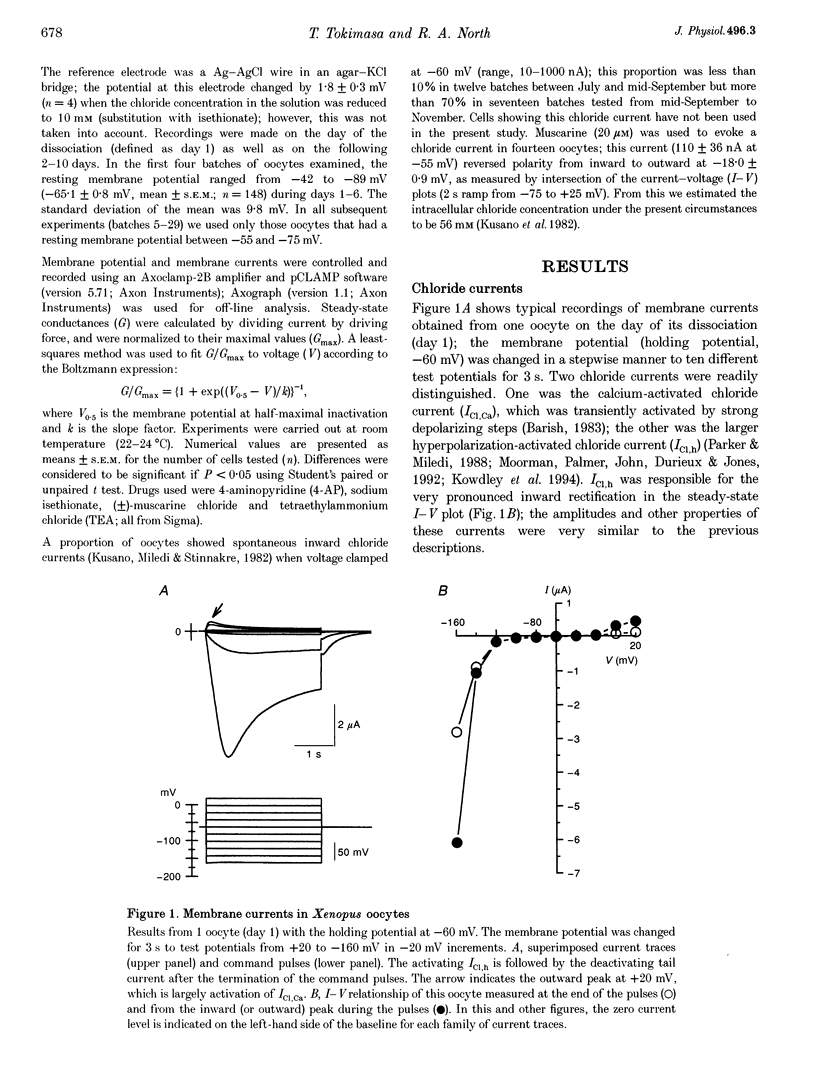
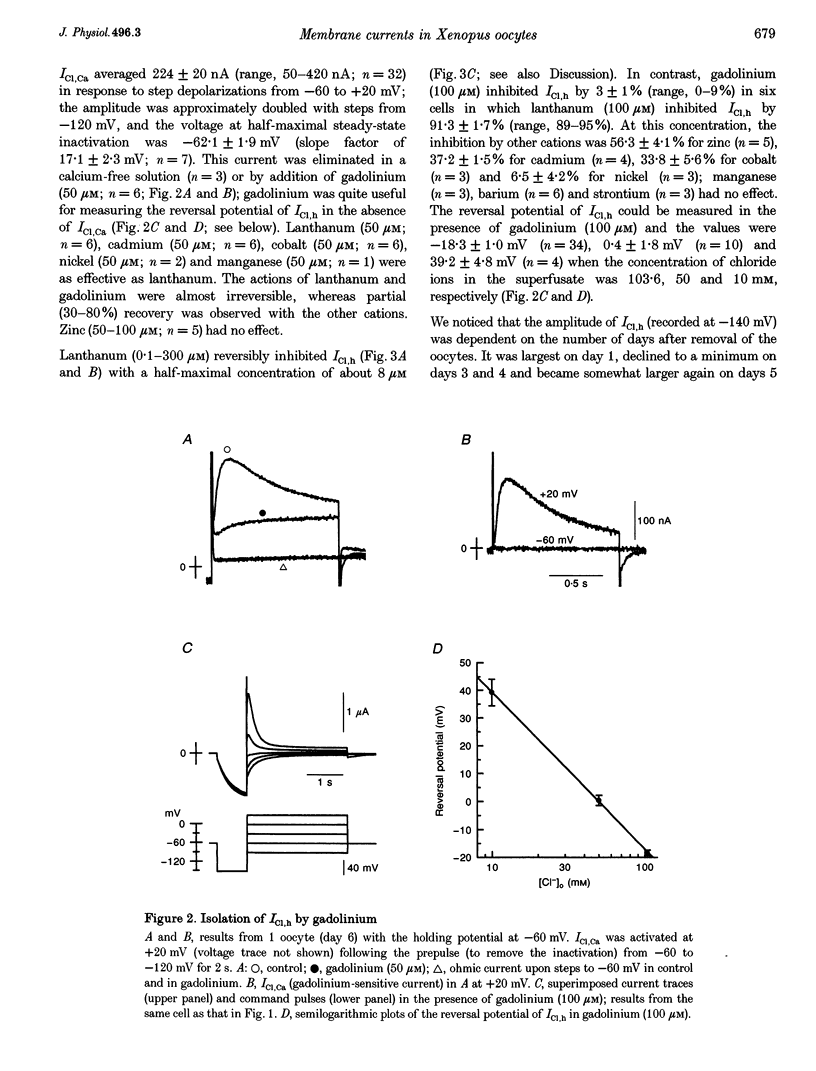
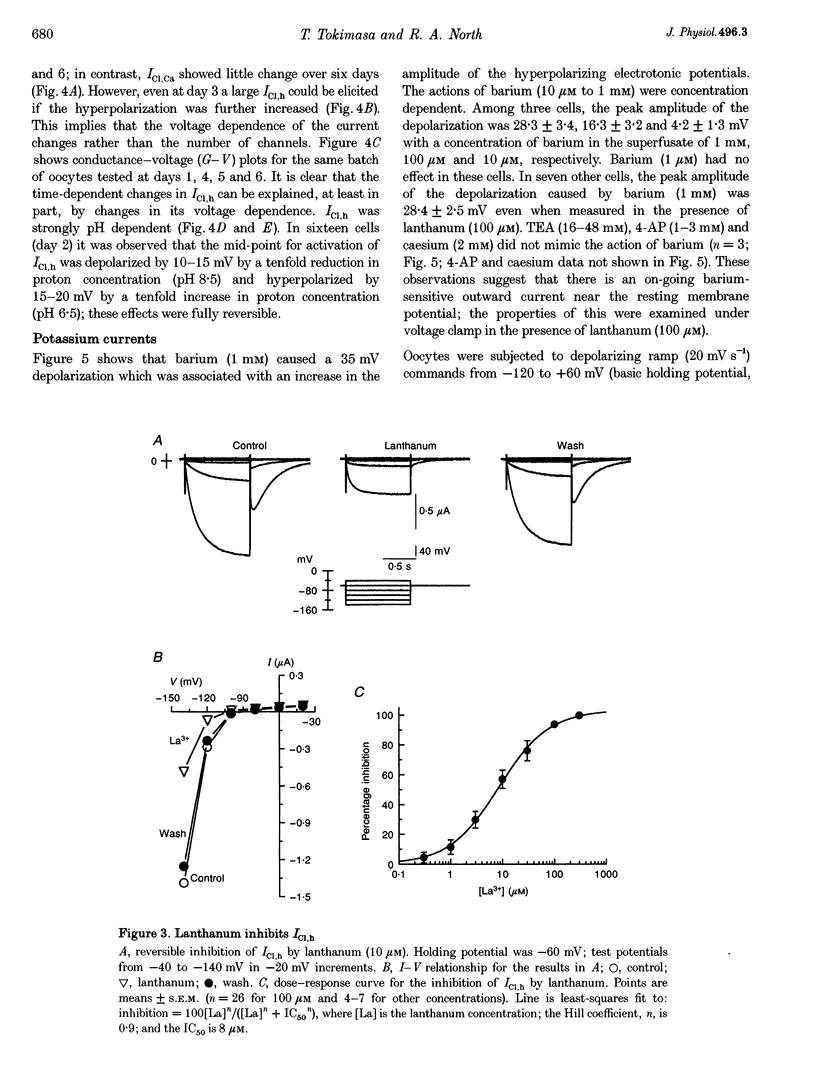
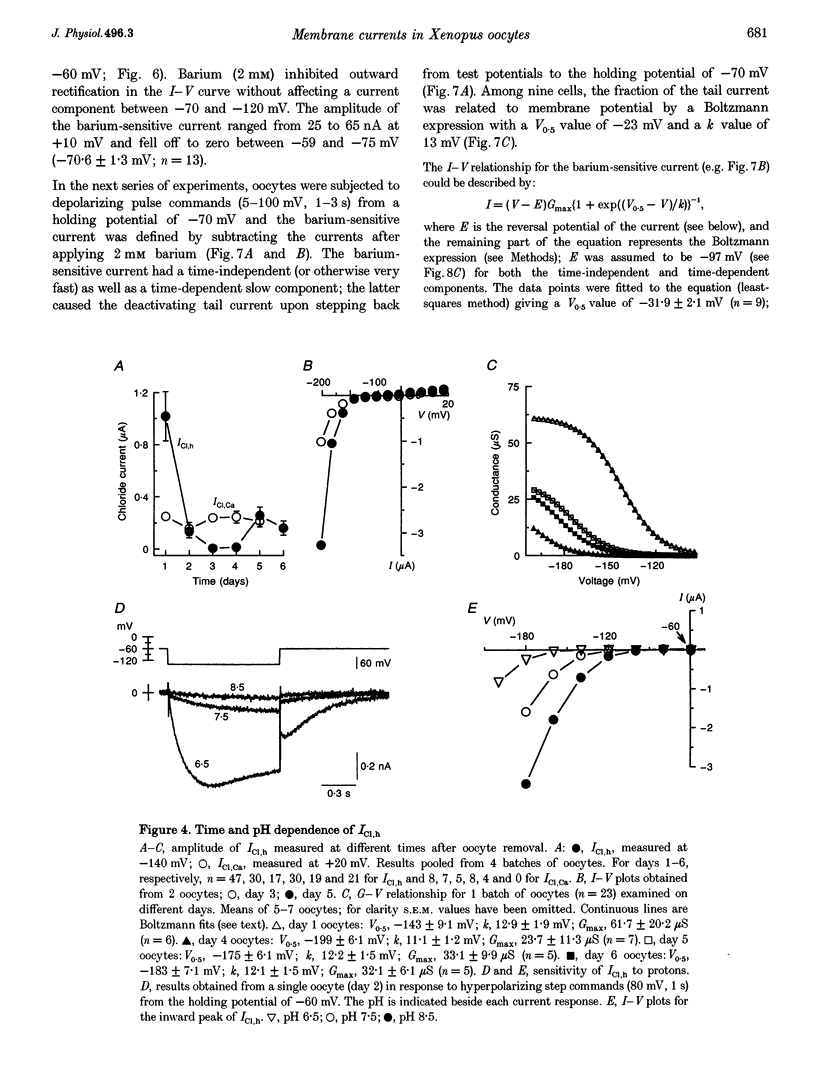
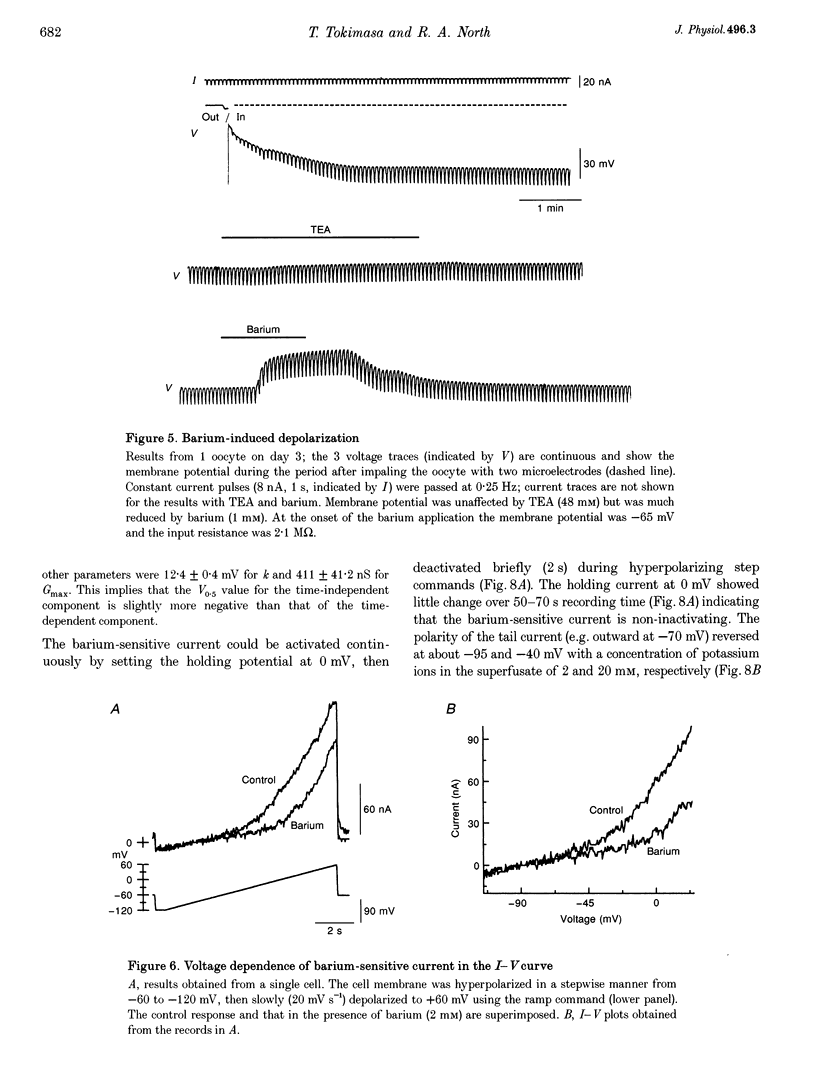
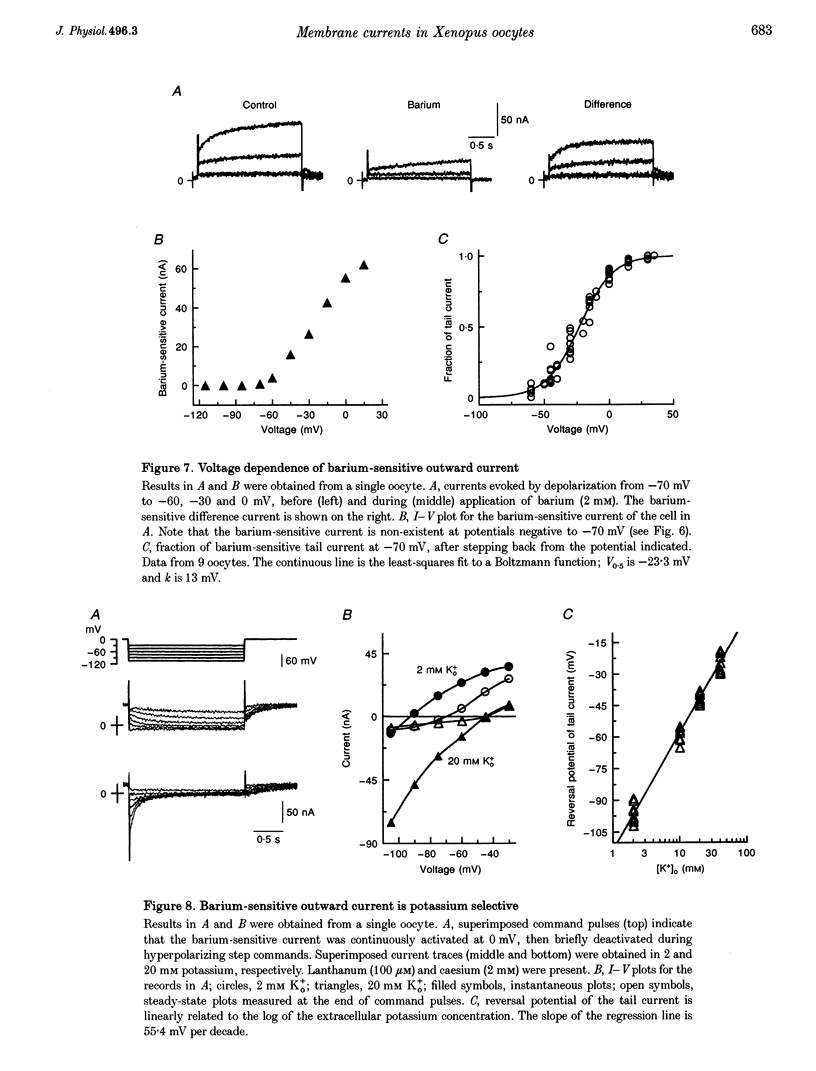
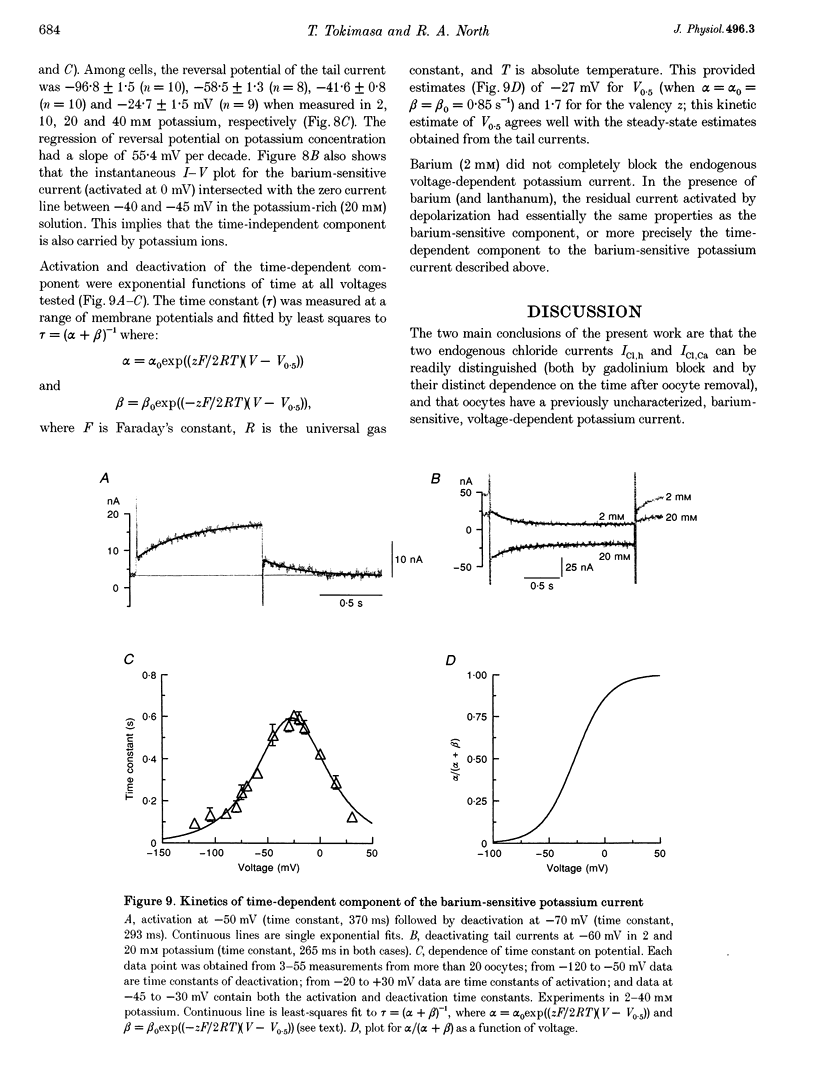
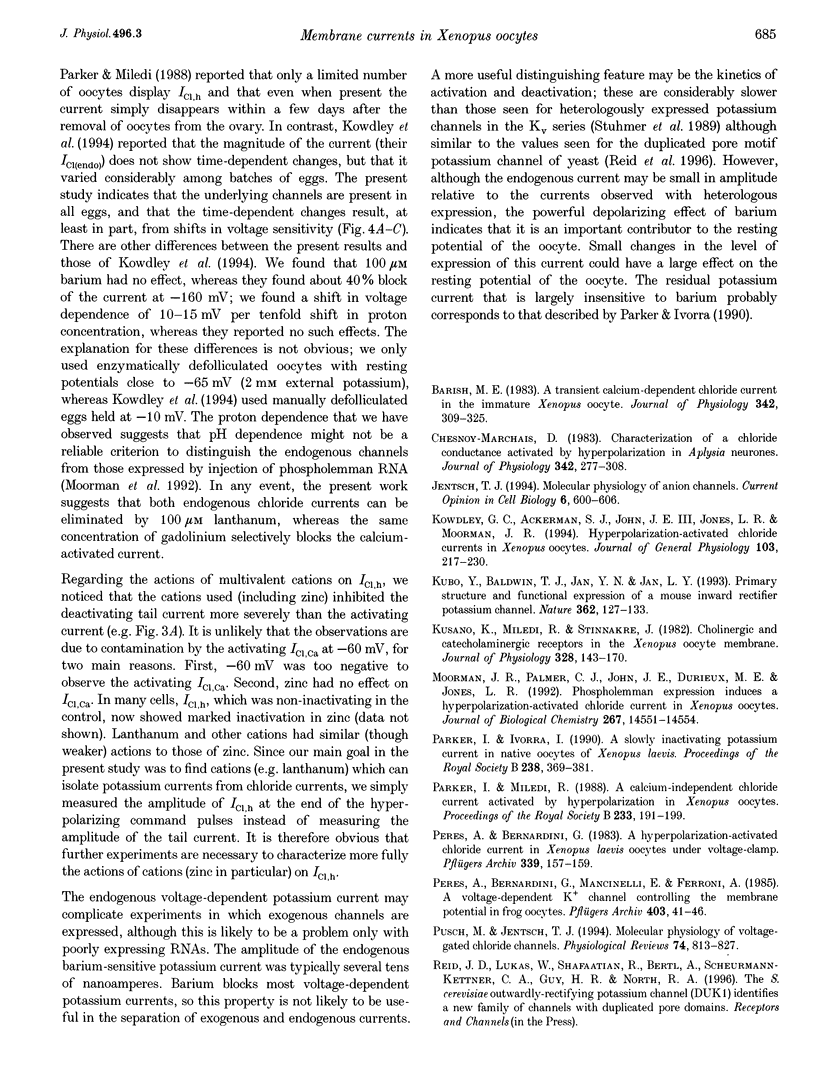
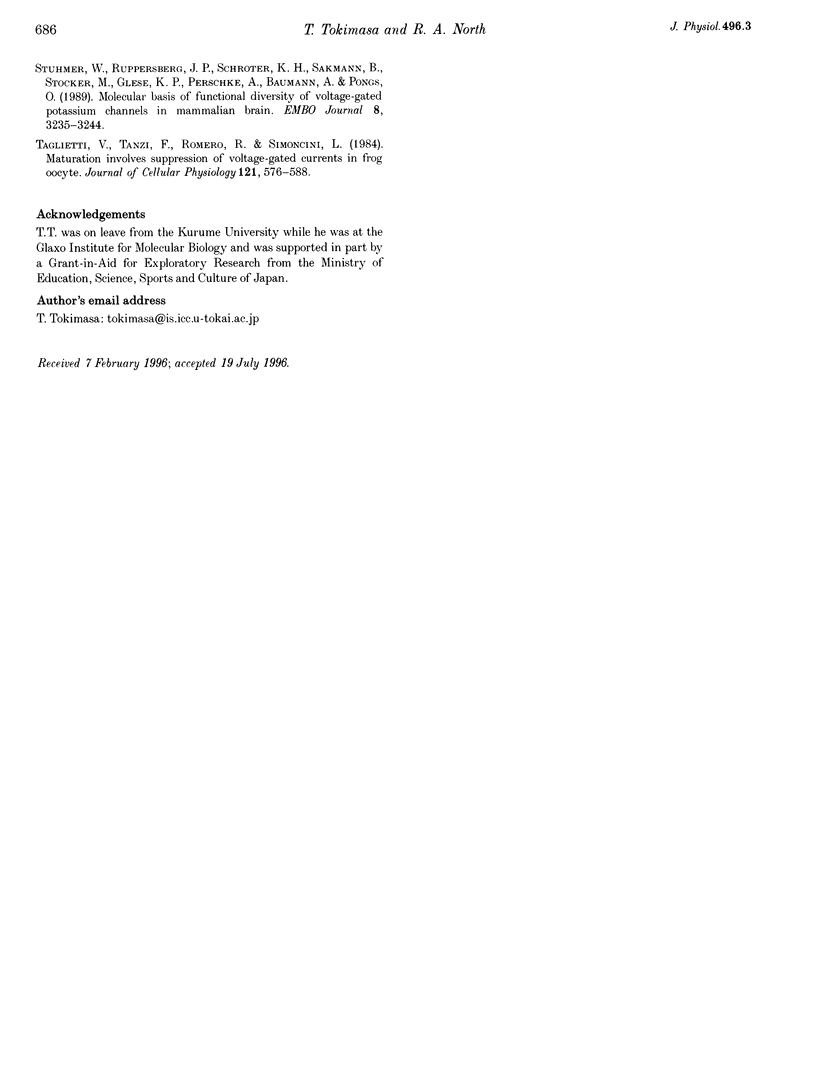
Selected References
These references are in PubMed. This may not be the complete list of references from this article.
- Barish M. E. A transient calcium-dependent chloride current in the immature Xenopus oocyte. J Physiol. 1983 Sep;342:309–325. doi: 10.1113/jphysiol.1983.sp014852. [DOI] [PMC free article] [PubMed] [Google Scholar]
- Chesnoy-Marchais D. Characterization of a chloride conductance activated by hyperpolarization in Aplysia neurones. J Physiol. 1983 Sep;342:277–308. doi: 10.1113/jphysiol.1983.sp014851. [DOI] [PMC free article] [PubMed] [Google Scholar]
- Jentsch T. J. Molecular physiology of anion channels. Curr Opin Cell Biol. 1994 Aug;6(4):600–606. doi: 10.1016/0955-0674(94)90082-5. [DOI] [PubMed] [Google Scholar]
- Kowdley G. C., Ackerman S. J., John J. E., 3rd, Jones L. R., Moorman J. R. Hyperpolarization-activated chloride currents in Xenopus oocytes. J Gen Physiol. 1994 Feb;103(2):217–230. doi: 10.1085/jgp.103.2.217. [DOI] [PMC free article] [PubMed] [Google Scholar]
- Kubo Y., Baldwin T. J., Jan Y. N., Jan L. Y. Primary structure and functional expression of a mouse inward rectifier potassium channel. Nature. 1993 Mar 11;362(6416):127–133. doi: 10.1038/362127a0. [DOI] [PubMed] [Google Scholar]
- Kusano K., Miledi R., Stinnakre J. Cholinergic and catecholaminergic receptors in the Xenopus oocyte membrane. J Physiol. 1982 Jul;328:143–170. doi: 10.1113/jphysiol.1982.sp014257. [DOI] [PMC free article] [PubMed] [Google Scholar]
- Moorman J. R., Palmer C. J., John J. E., 3rd, Durieux M. E., Jones L. R. Phospholemman expression induces a hyperpolarization-activated chloride current in Xenopus oocytes. J Biol Chem. 1992 Jul 25;267(21):14551–14554. [PubMed] [Google Scholar]
- Parker I., Ivorra I. A slowly inactivating potassium current in native oocytes of Xenopus laevis. Proc R Soc Lond B Biol Sci. 1990 Jan 22;238(1293):369–381. doi: 10.1098/rspb.1990.0005. [DOI] [PubMed] [Google Scholar]
- Parker I., Miledi R. A calcium-independent chloride current activated by hyperpolarization in Xenopus oocytes. Proc R Soc Lond B Biol Sci. 1988 Mar 22;233(1271):191–199. doi: 10.1098/rspb.1988.0018. [DOI] [PubMed] [Google Scholar]
- Peres A., Bernardini G. A hyperpolarization-activated chloride current in Xenopus laevis oocytes under voltage-clamp. Pflugers Arch. 1983 Oct;399(2):157–159. doi: 10.1007/BF00663914. [DOI] [PubMed] [Google Scholar]
- Peres A., Bernardini G., Mancinelli E., Ferroni A. A voltage-dependent K+ channel controlling the membrane potential in frog oocytes. Pflugers Arch. 1985 Jan;403(1):41–46. doi: 10.1007/BF00583279. [DOI] [PubMed] [Google Scholar]
- Pusch M., Jentsch T. J. Molecular physiology of voltage-gated chloride channels. Physiol Rev. 1994 Oct;74(4):813–827. doi: 10.1152/physrev.1994.74.4.813. [DOI] [PubMed] [Google Scholar]
- Stühmer W., Ruppersberg J. P., Schröter K. H., Sakmann B., Stocker M., Giese K. P., Perschke A., Baumann A., Pongs O. Molecular basis of functional diversity of voltage-gated potassium channels in mammalian brain. EMBO J. 1989 Nov;8(11):3235–3244. doi: 10.1002/j.1460-2075.1989.tb08483.x. [DOI] [PMC free article] [PubMed] [Google Scholar]
- Taglietti V., Tanzi F., Romero R., Simoncini L. Maturation involves suppression of voltage-gated currents in the frog oocyte. J Cell Physiol. 1984 Dec;121(3):576–588. doi: 10.1002/jcp.1041210317. [DOI] [PubMed] [Google Scholar]