Abstract
1. The origin of labile heat production, i.e. a heat component which rapidly decays after the onset of stimulation, and of stable (maintenance) heat production was investigated in intact single fast-twitch (type 1) and slow-twitch (type 3) iliofibularis muscle fibres from Xenopus laevis, at 20 degrees C, by varying stimulation frequency and by varying sarcomere length and the concentration of 2,3-butanedione 2-monoxime (BDM) added. 2. The labile heat produced consisted of a force-independent and a force-dependent part. The average parvalbumin (PA) content found in type 1 fibre bundles (0.84 +/- 0.08 mM; mean +/- S.E.M.; n = 5) and in type 3 fibre bundles (0.12 +/- 0.02 mM; n = 5) indicates that the force-independent labile heat is explained by Ca(2+)-Mg2+ exchange on PA, and amounts to a molar enthalpy change of -78 kJ (molPA)-1. 3. Force-dependent labile heat during fused contractions was similar to the calculated heat production resulting from the formation of force-generating cross-bridges, assuming an enthalpy change associated with cross-bridge formation of -30 kJ mol-1. 4. Activation heat, i.e. the part of the total stable heat that is not related to the contractile apparatus, and of which the calcium sequestration by the sarcoplasmic reticulum is the most important contributor, determined by varying sarcomere length or BDM concentration, was identical. For fused contractions the fraction activation heat of the stable maintenance rate of heat production was 34 +/- 4% (mean +/- S.E.M.; n = 13) in type 1 fibres, and 52 +/- 4% (n = 15) in type 3 fibres. In unfused contractions this was 48 +/- 5% (n = 13) in type 1 fibres, and 35 +/- 2% (n = 11) in type 3 fibres. 5. From the force-dependent stable rate of heat production the economy of cross-bridge cycling, expressed as the force-time integral for a single myosin head per ATP molecule hydrolysed, was calculated. It followed that cross-bridge interaction in type 3 fibres is more economical than in type 1 fibres, and that fused contractions are more economical than unfused contractions.
Full text
PDF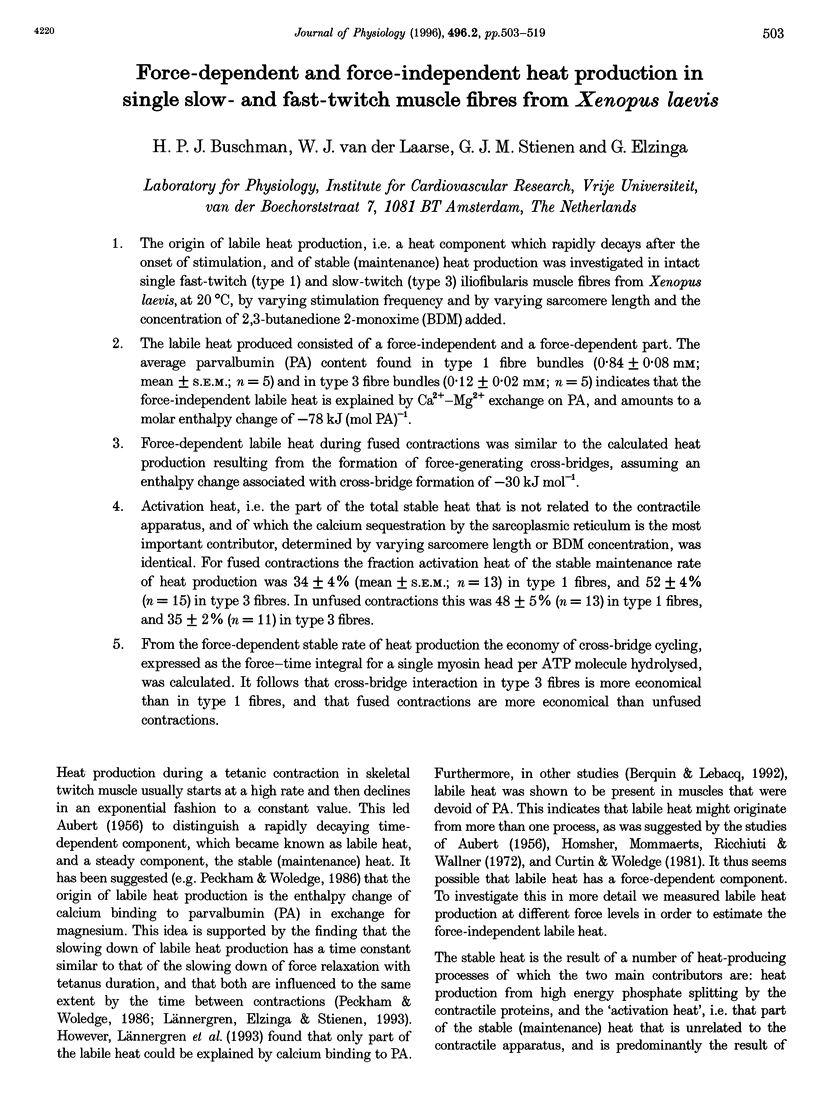
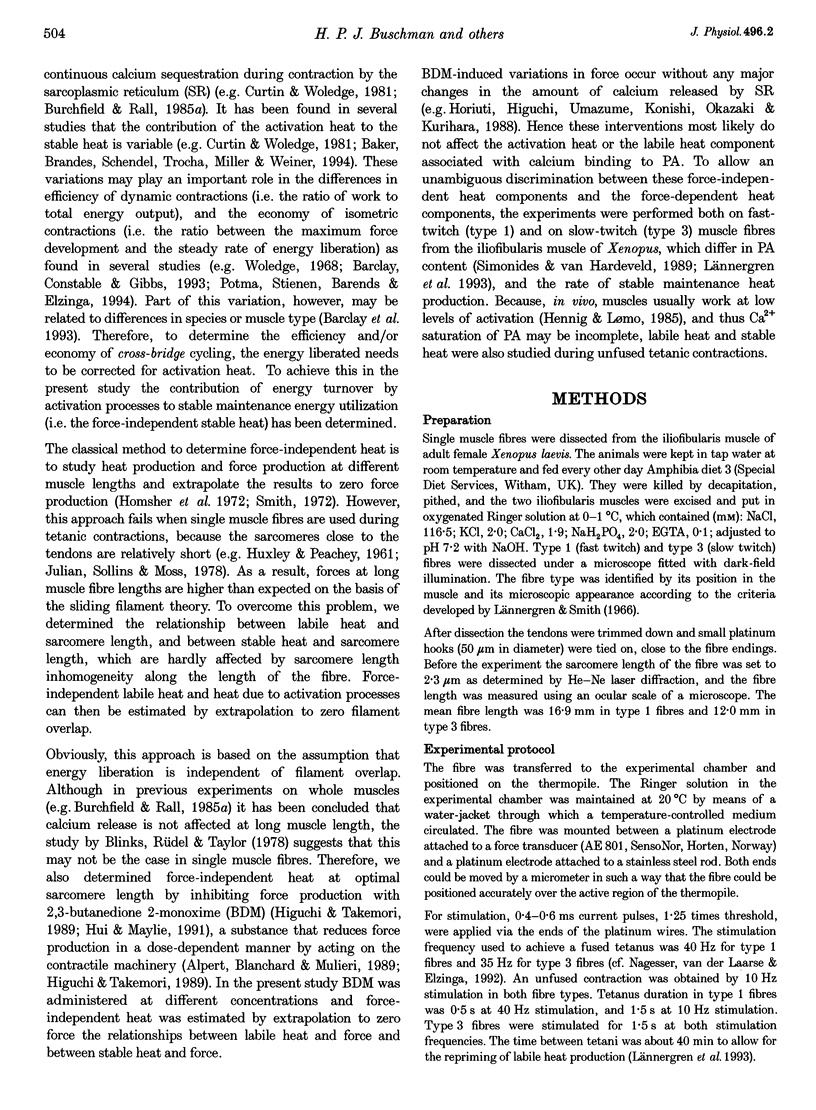
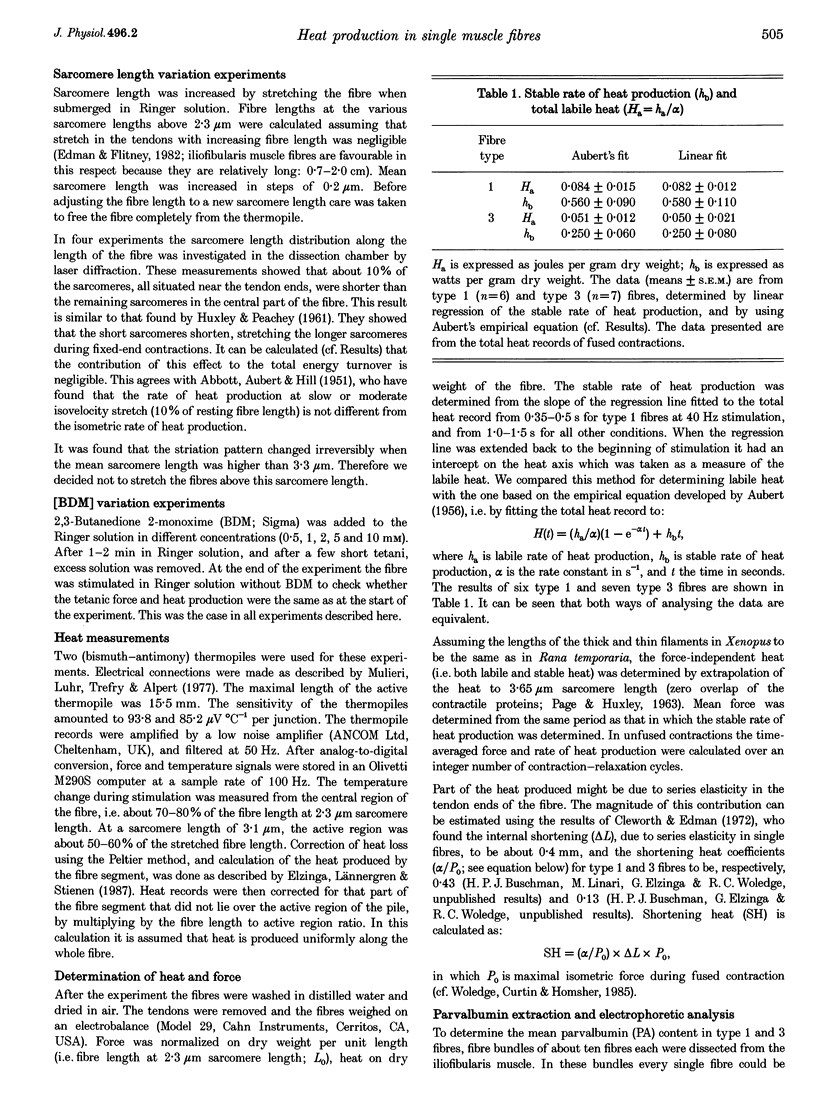
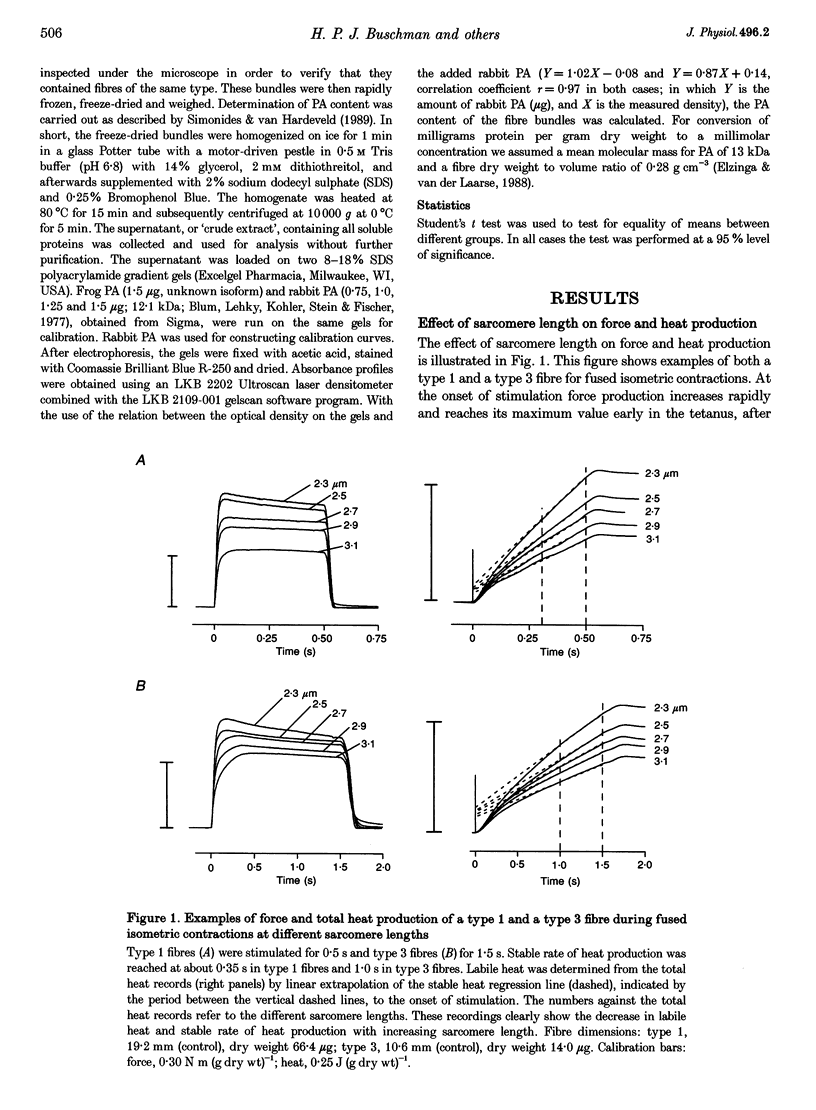
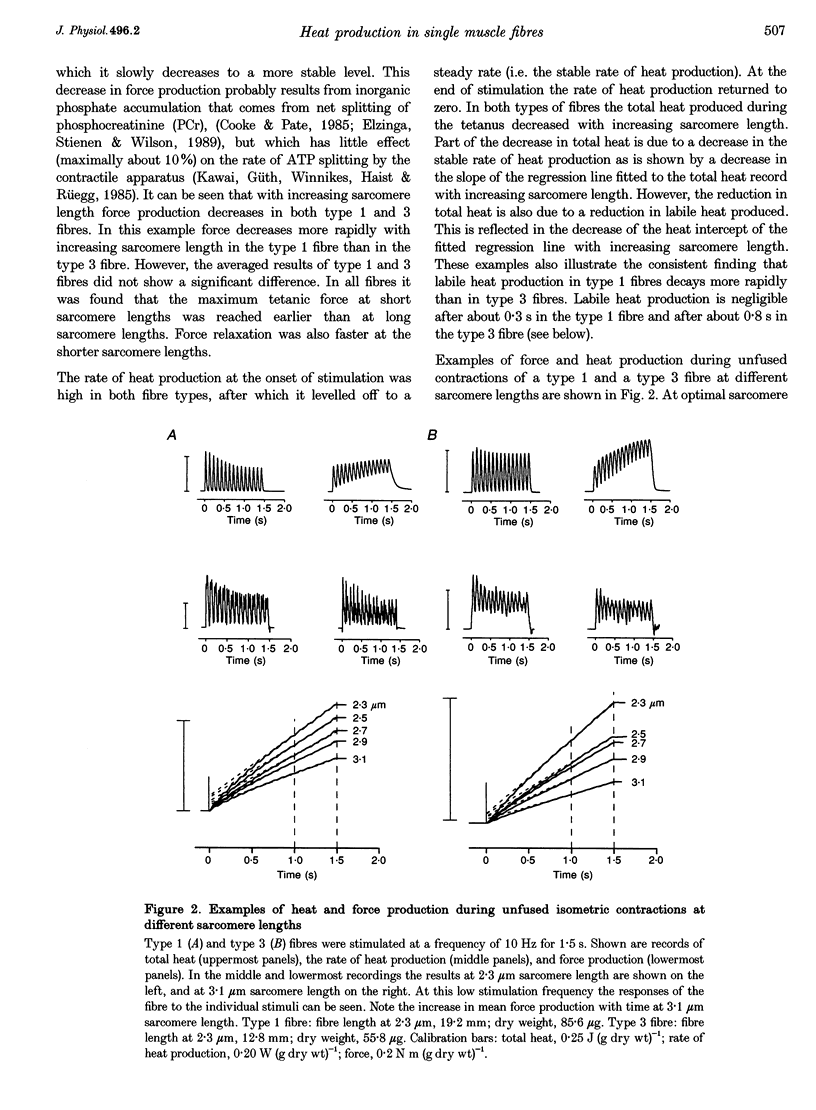
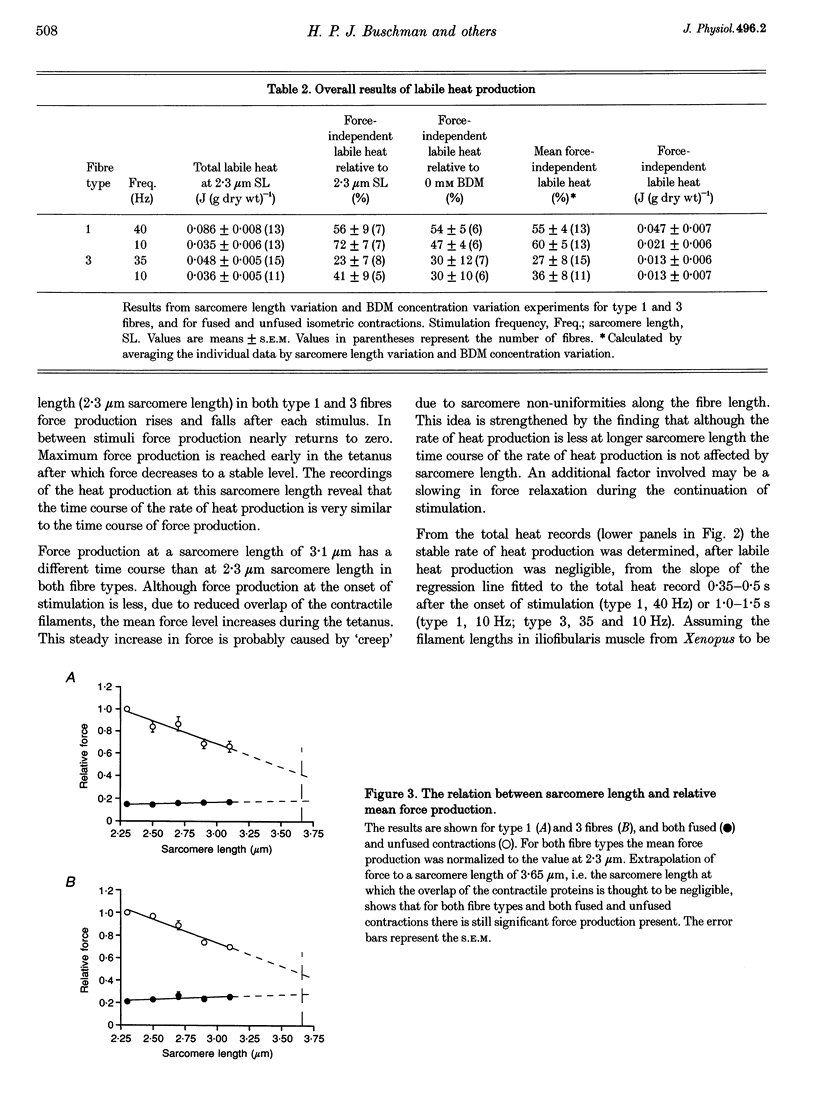
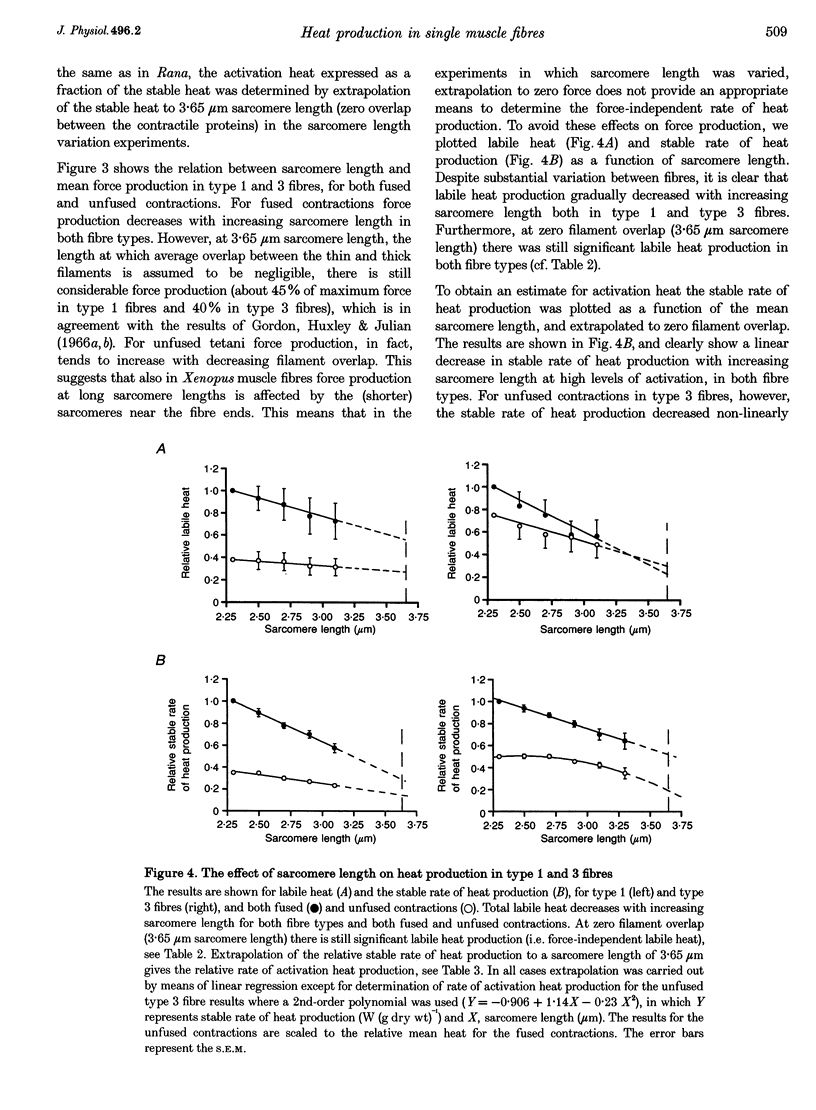
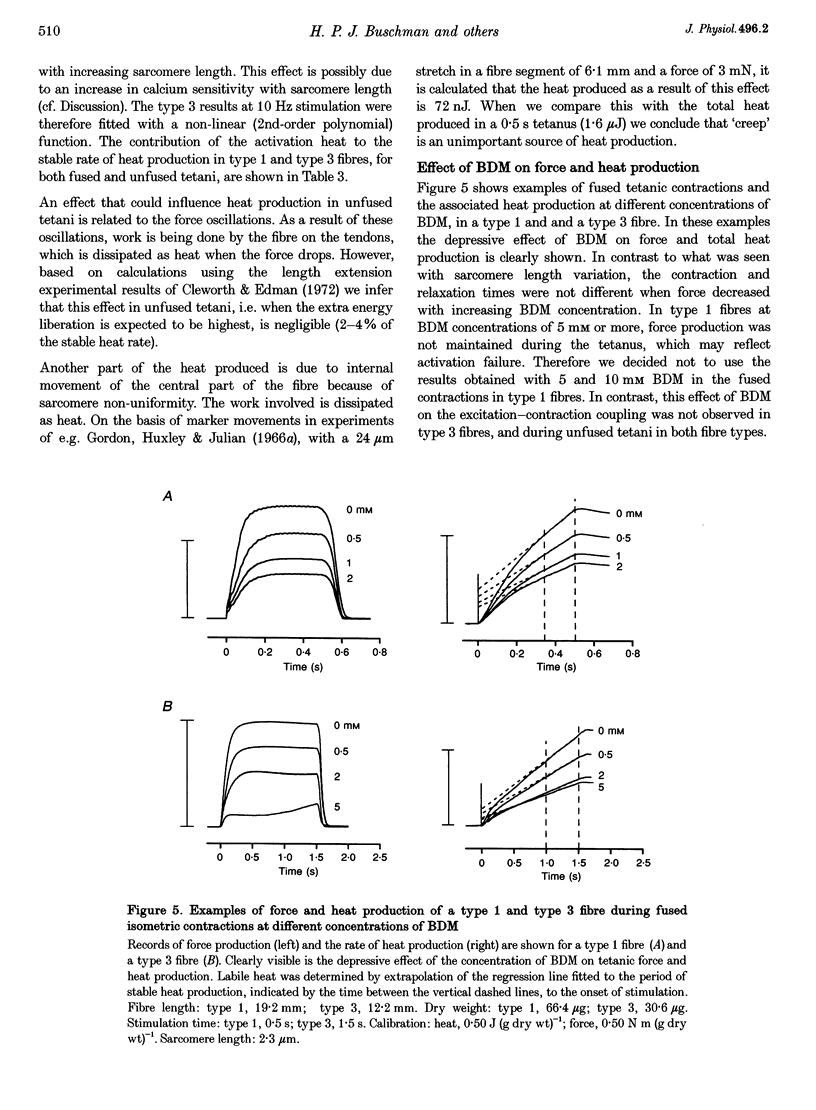
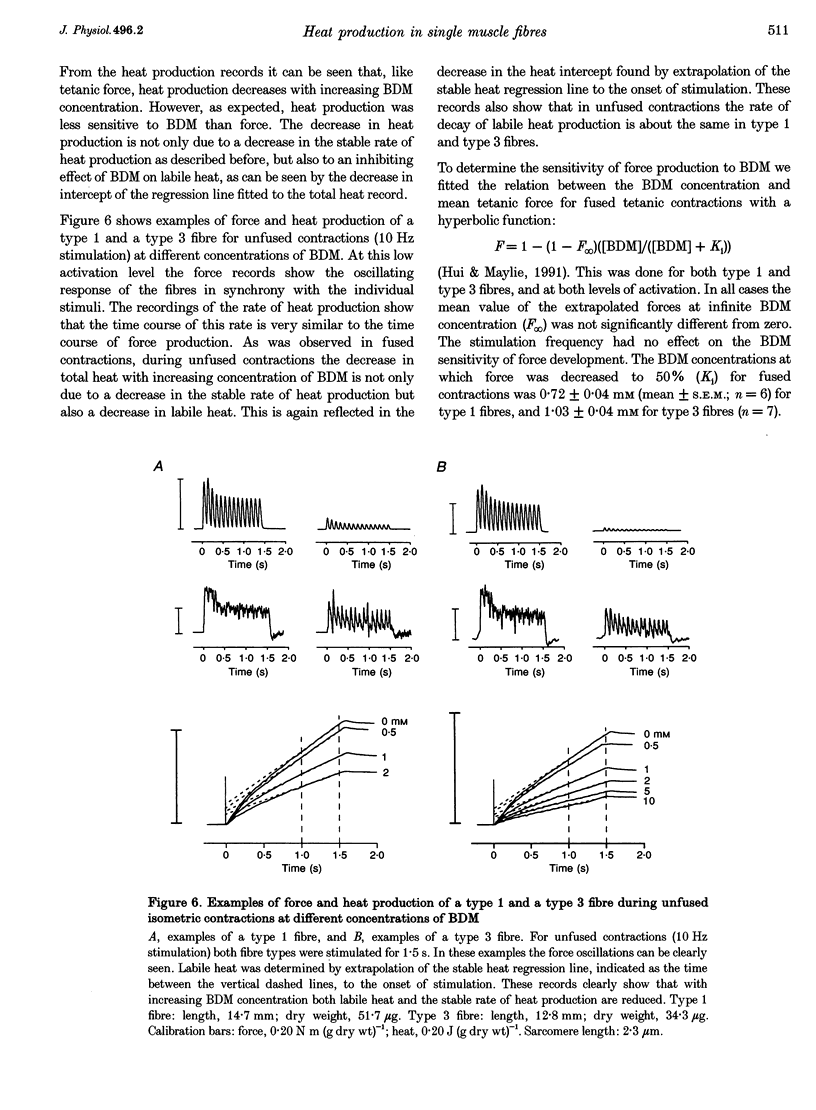
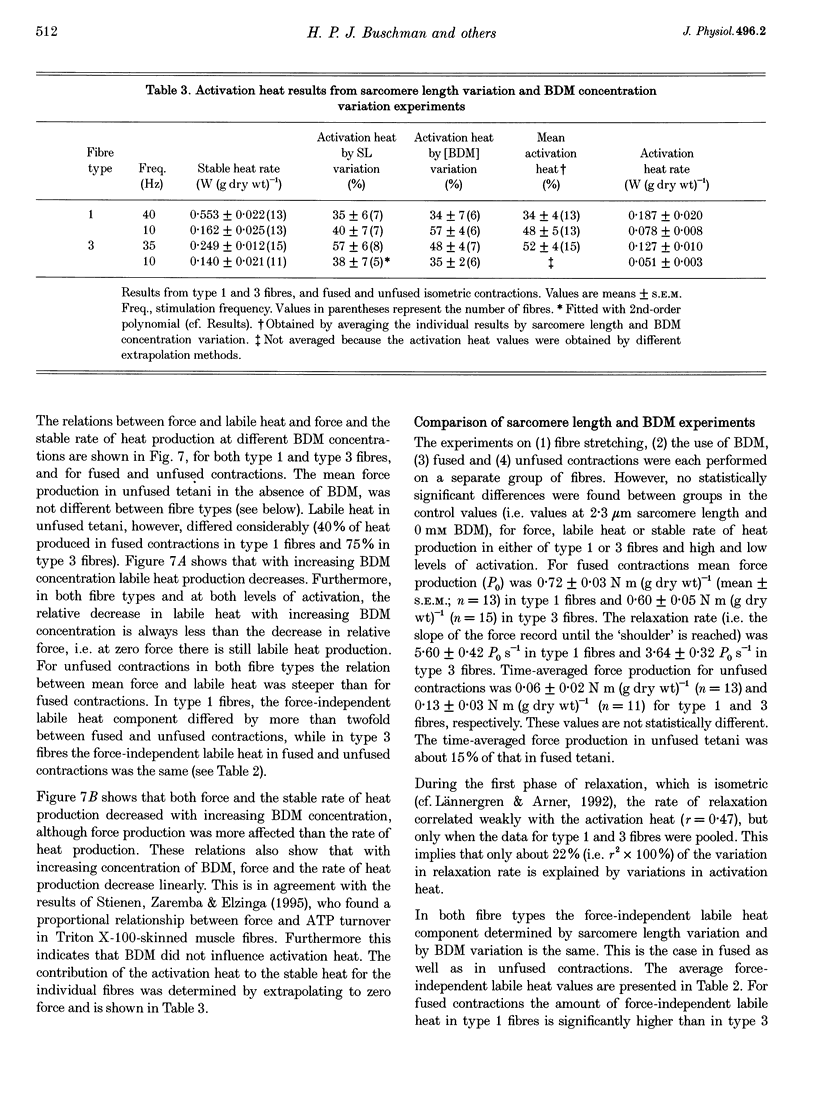
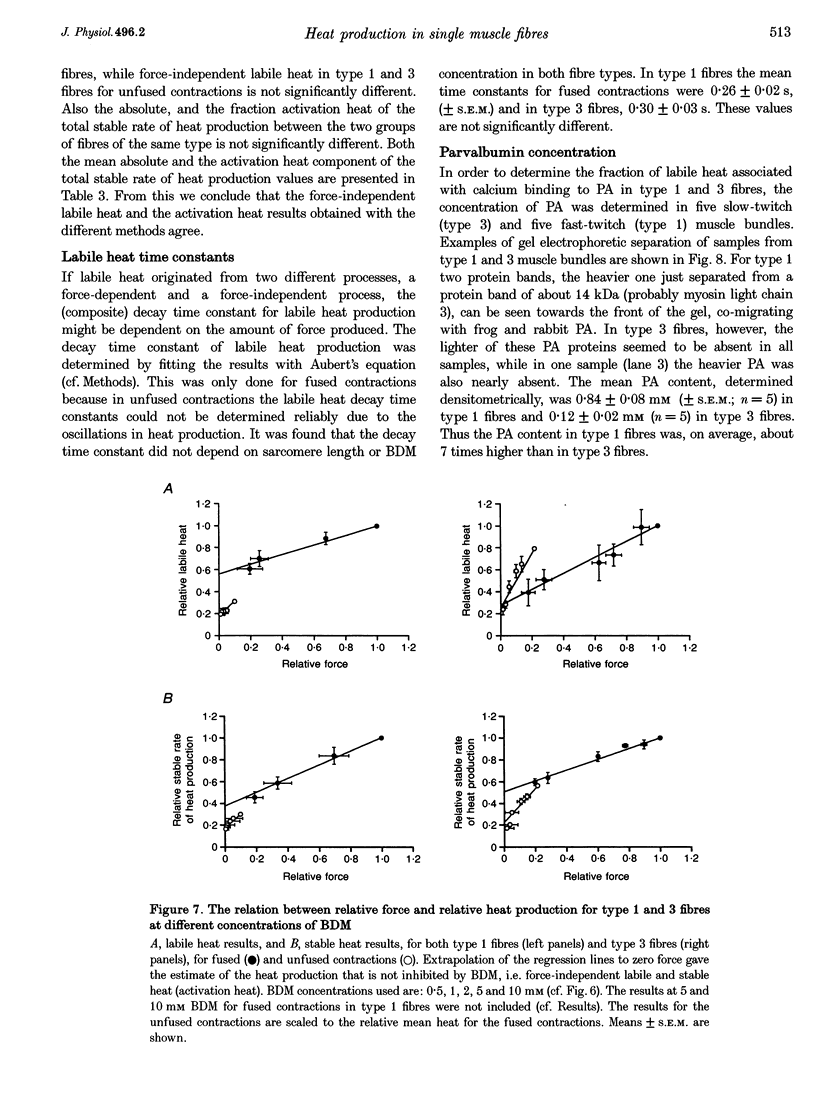
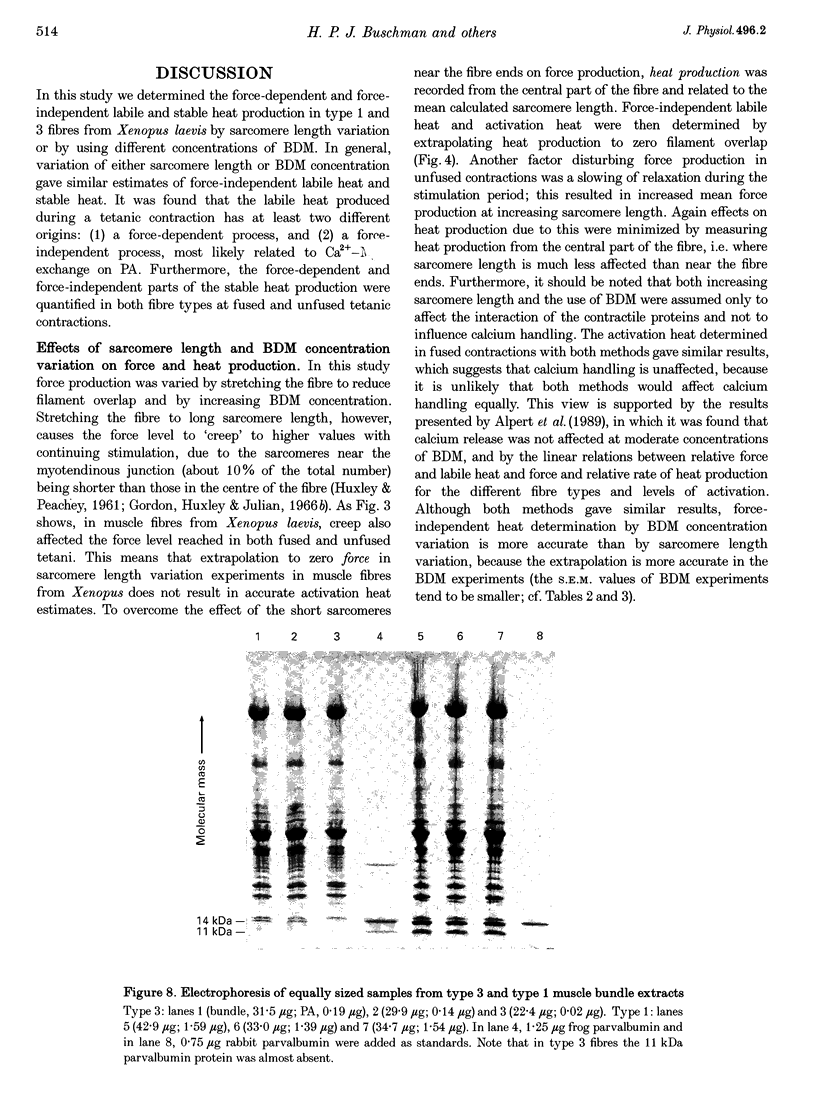
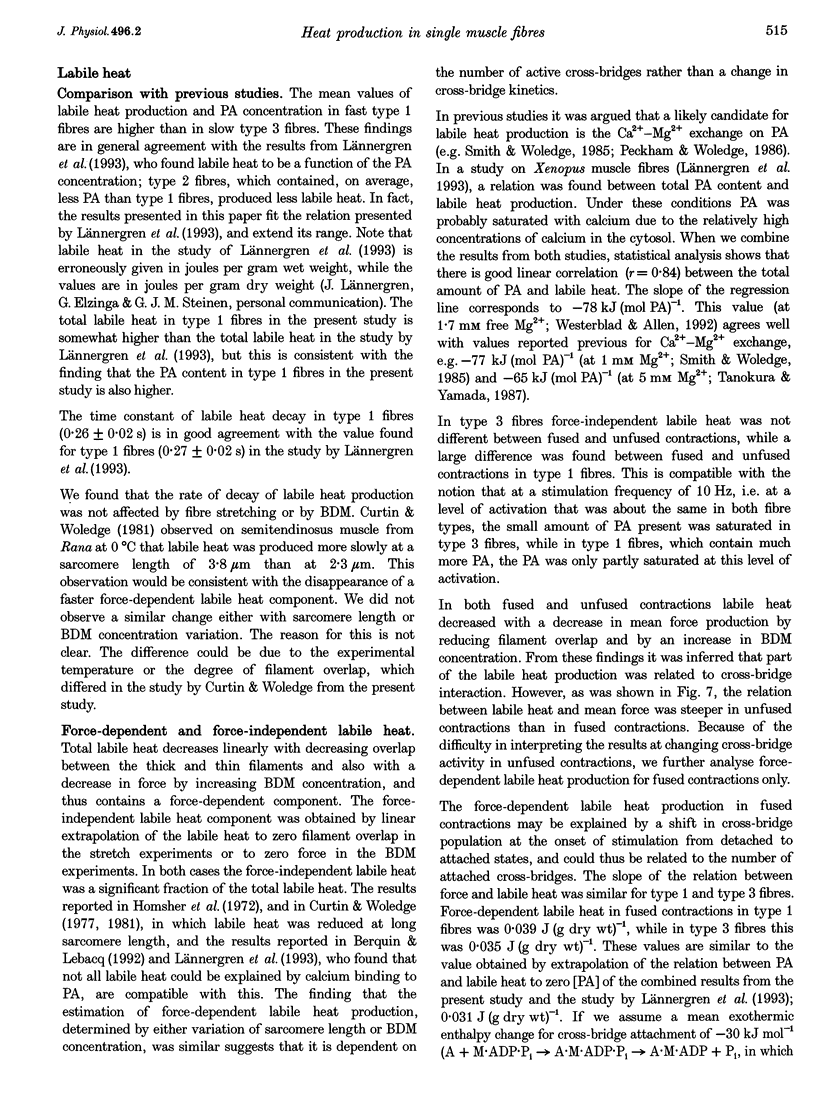
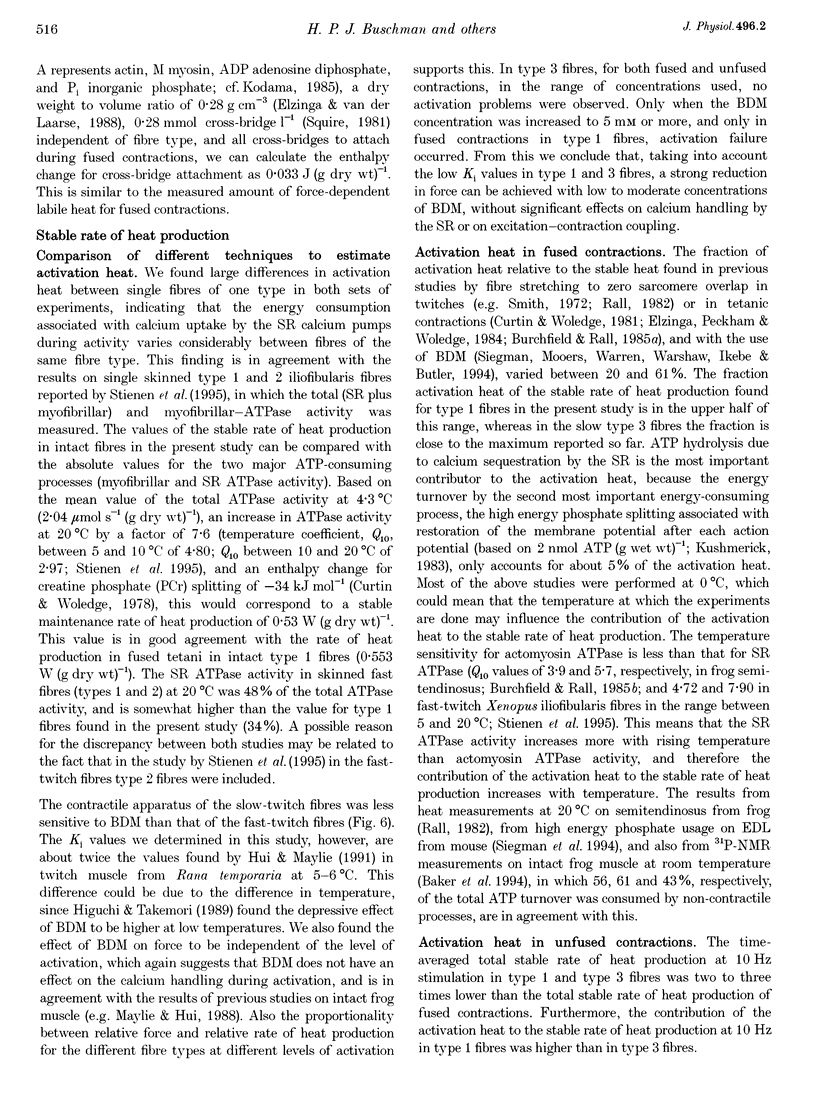
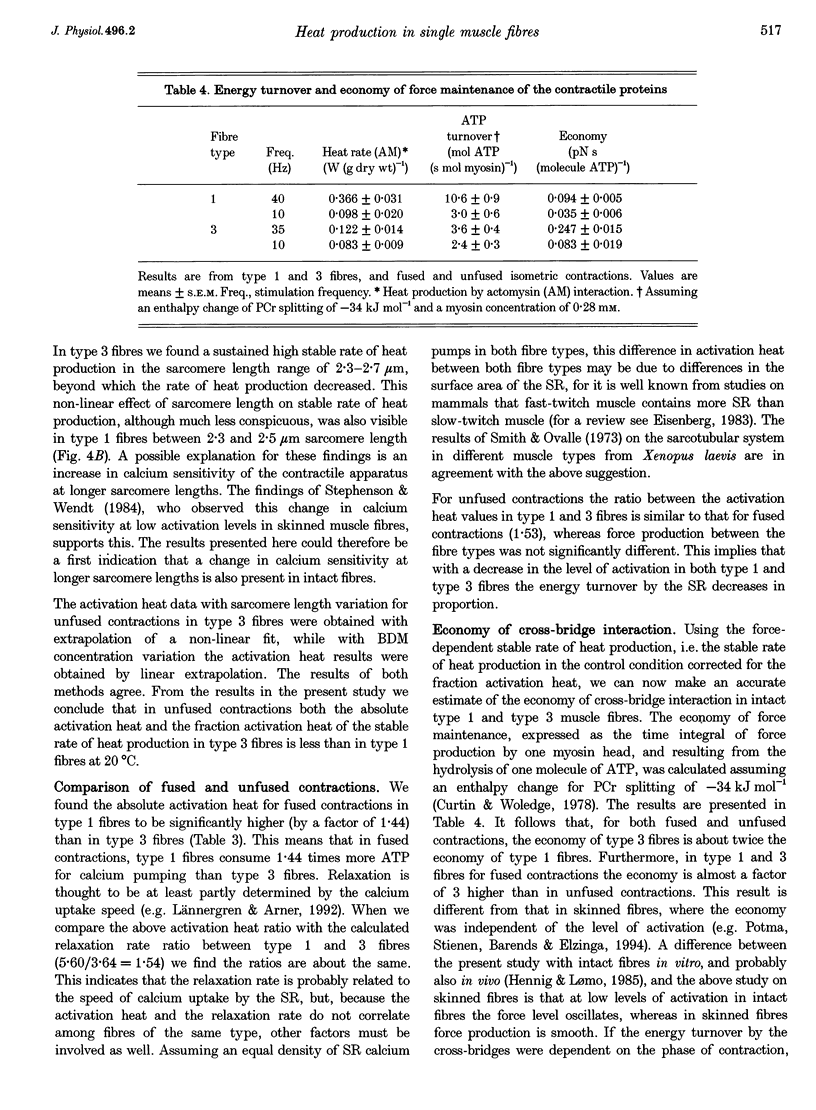
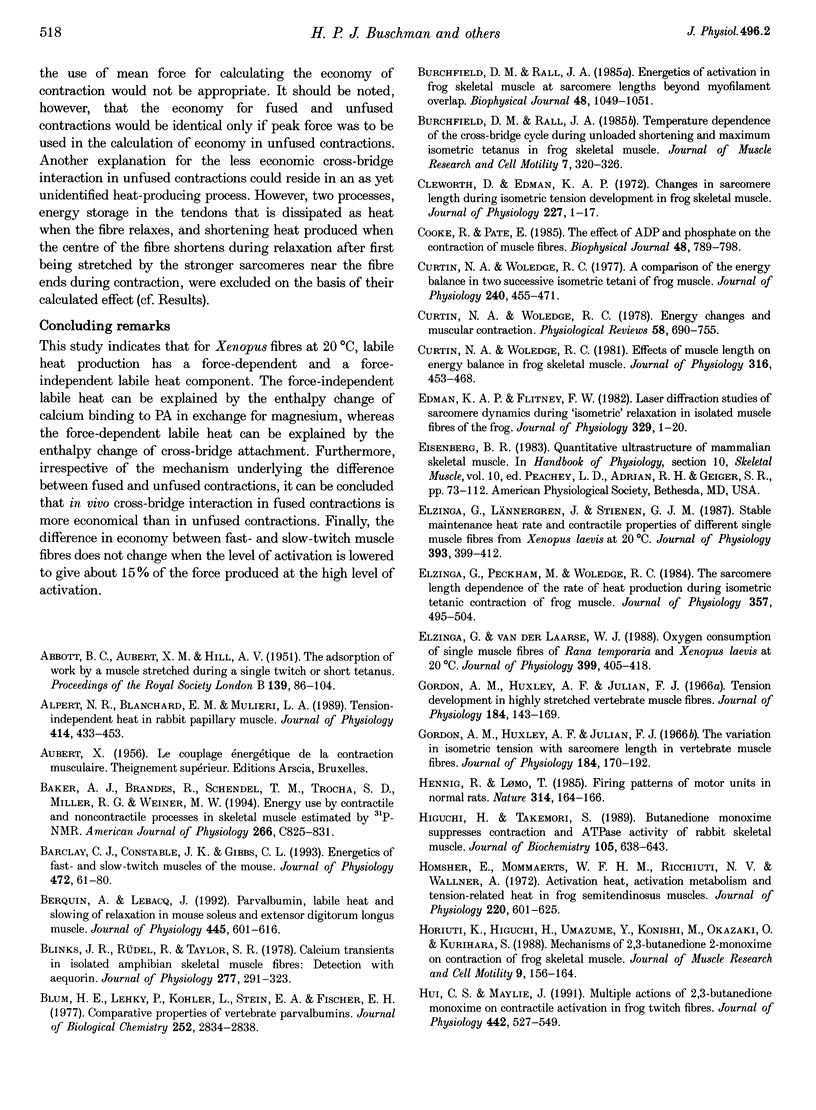
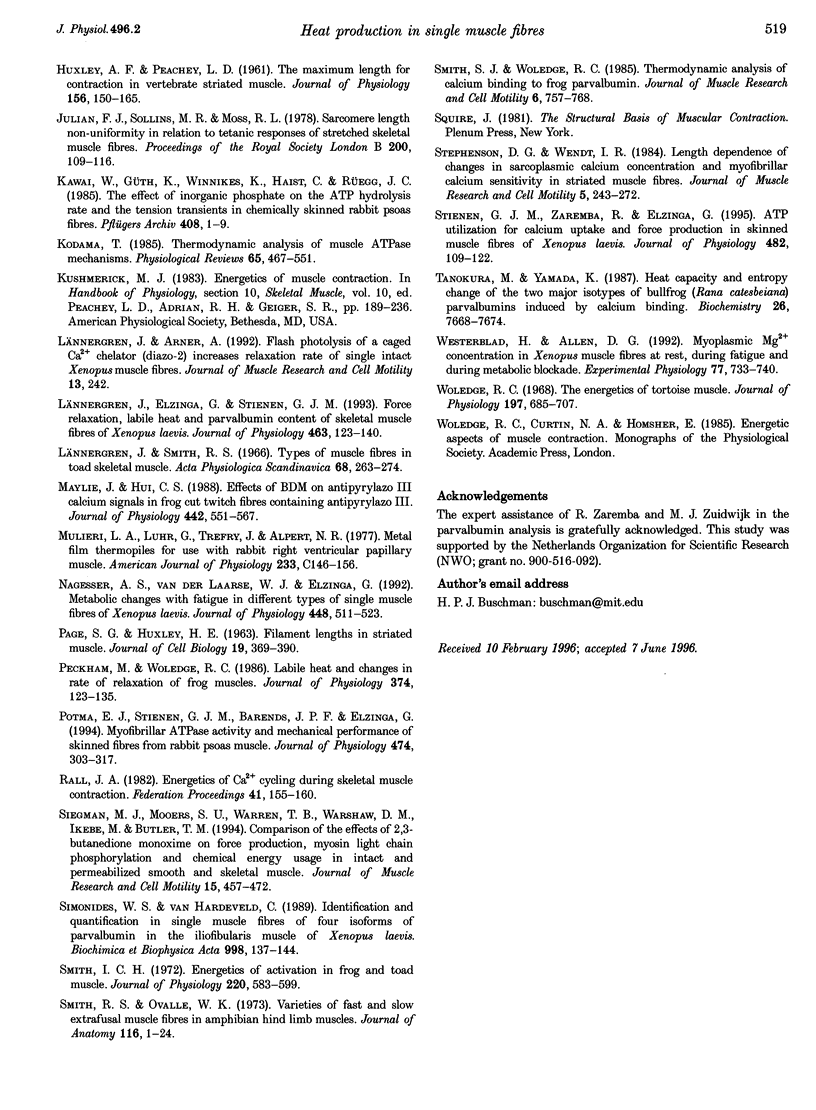
Images in this article
Selected References
These references are in PubMed. This may not be the complete list of references from this article.
- ABBOTT B. C., AUBERT X. M., HILL A. V. The absorption of work by a muscle stretched during a single twitch or a short tetanus. Proc R Soc Lond B Biol Sci. 1951 Dec 31;139(894):86–104. doi: 10.1098/rspb.1951.0048. [DOI] [PubMed] [Google Scholar]
- Alpert N. R., Blanchard E. M., Mulieri L. A. Tension-independent heat in rabbit papillary muscle. J Physiol. 1989 Jul;414:433–453. doi: 10.1113/jphysiol.1989.sp017697. [DOI] [PMC free article] [PubMed] [Google Scholar]
- Baker A. J., Brandes R., Schendel T. M., Trocha S. D., Miller R. G., Weiner M. W. Energy use by contractile and noncontractile processes in skeletal muscle estimated by 31P-NMR. Am J Physiol. 1994 Mar;266(3 Pt 1):C825–C831. doi: 10.1152/ajpcell.1994.266.3.C825. [DOI] [PubMed] [Google Scholar]
- Barclay C. J., Constable J. K., Gibbs C. L. Energetics of fast- and slow-twitch muscles of the mouse. J Physiol. 1993 Dec;472:61–80. doi: 10.1113/jphysiol.1993.sp019937. [DOI] [PMC free article] [PubMed] [Google Scholar]
- Berquin A., Lebacq J. Parvalbumin, labile heat and slowing of relaxation in mouse soleus and extensor digitorum longus muscles. J Physiol. 1992 Jan;445:601–616. doi: 10.1113/jphysiol.1992.sp018942. [DOI] [PMC free article] [PubMed] [Google Scholar]
- Blinks J. R., Rüdel R., Taylor S. R. Calcium transients in isolated amphibian skeletal muscle fibres: detection with aequorin. J Physiol. 1978 Apr;277:291–323. doi: 10.1113/jphysiol.1978.sp012273. [DOI] [PMC free article] [PubMed] [Google Scholar]
- Blum H. E., Lehky P., Kohler L., Stein E. A., Fischer E. H. Comparative properties of vertebrate parvalbumins. J Biol Chem. 1977 May 10;252(9):2834–2838. [PubMed] [Google Scholar]
- Burchfield D. M., Rall J. A. Energetics of activation in frog skeletal muscle at sarcomere lengths beyond myofilament overlap. Biophys J. 1985 Dec;48(6):1049–1051. doi: 10.1016/S0006-3495(85)83867-4. [DOI] [PMC free article] [PubMed] [Google Scholar]
- Burchfield D. M., Rall J. A. Temperature dependence of the crossbridge cycle during unloaded shortening and maximum isometric tetanus in frog skeletal muscle. J Muscle Res Cell Motil. 1986 Aug;7(4):320–326. doi: 10.1007/BF01753652. [DOI] [PubMed] [Google Scholar]
- Cleworth D. R., Edman K. A. Changes in sarcomere length during isometric tension development in frog skeletal muscle. J Physiol. 1972 Dec;227(1):1–17. doi: 10.1113/jphysiol.1972.sp010016. [DOI] [PMC free article] [PubMed] [Google Scholar]
- Cooke R., Pate E. The effects of ADP and phosphate on the contraction of muscle fibers. Biophys J. 1985 Nov;48(5):789–798. doi: 10.1016/S0006-3495(85)83837-6. [DOI] [PMC free article] [PubMed] [Google Scholar]
- Curtin N. A., Woledge R. C. A comparison of the energy balance in two successive isometric tetani of frog muscle. J Physiol. 1977 Sep;270(2):455–471. doi: 10.1113/jphysiol.1977.sp011962. [DOI] [PMC free article] [PubMed] [Google Scholar]
- Curtin N. A., Woledge R. C. Effect of muscle length on energy balance in frog skeletal muscle. J Physiol. 1981 Jul;316:453–468. doi: 10.1113/jphysiol.1981.sp013800. [DOI] [PMC free article] [PubMed] [Google Scholar]
- Curtin N. A., Woledge R. C. Energy changes and muscular contraction. Physiol Rev. 1978 Jul;58(3):690–761. doi: 10.1152/physrev.1978.58.3.690. [DOI] [PubMed] [Google Scholar]
- Edman K. A., Flitney F. W. Laser diffraction studies of sarcomere dynamics during 'isometric' relaxation in isolated muscle fibres of the frog. J Physiol. 1982 Aug;329:1–20. doi: 10.1113/jphysiol.1982.sp014287. [DOI] [PMC free article] [PubMed] [Google Scholar]
- Elzinga G., Lännergren J., Stienen G. J. Stable maintenance heat rate and contractile properties of different single muscle fibres from Xenopus laevis at 20 degrees C. J Physiol. 1987 Dec;393:399–412. doi: 10.1113/jphysiol.1987.sp016829. [DOI] [PMC free article] [PubMed] [Google Scholar]
- Elzinga G., Peckham M., Woledge R. C. The sarcomere length dependence of the rate of heat production during isometric tetanic contraction of frog muscles. J Physiol. 1984 Dec;357:495–504. doi: 10.1113/jphysiol.1984.sp015513. [DOI] [PMC free article] [PubMed] [Google Scholar]
- Elzinga G., van der Laarse W. J. Oxygen consumption of single muscle fibres of Rana temporaria and Xenopus laevis at 20 degrees C. J Physiol. 1988 May;399:405–418. doi: 10.1113/jphysiol.1988.sp017088. [DOI] [PMC free article] [PubMed] [Google Scholar]
- Gordon A. M., Huxley A. F., Julian F. J. Tension development in highly stretched vertebrate muscle fibres. J Physiol. 1966 May;184(1):143–169. doi: 10.1113/jphysiol.1966.sp007908. [DOI] [PMC free article] [PubMed] [Google Scholar]
- Gordon A. M., Huxley A. F., Julian F. J. The variation in isometric tension with sarcomere length in vertebrate muscle fibres. J Physiol. 1966 May;184(1):170–192. doi: 10.1113/jphysiol.1966.sp007909. [DOI] [PMC free article] [PubMed] [Google Scholar]
- HUXLEY A. F., PEACHEY L. D. The maximum length for contraction in vertebrate straiated muscle. J Physiol. 1961 Apr;156:150–165. doi: 10.1113/jphysiol.1961.sp006665. [DOI] [PMC free article] [PubMed] [Google Scholar]
- Homsher E., Mommaerts W. F., Ricchiuti N. V., Wallner A. Activation heat, activation metabolism and tension-related heat in frog semitendinosus muscles. J Physiol. 1972 Feb;220(3):601–625. doi: 10.1113/jphysiol.1972.sp009725. [DOI] [PMC free article] [PubMed] [Google Scholar]
- Horiuti K., Higuchi H., Umazume Y., Konishi M., Okazaki O., Kurihara S. Mechanism of action of 2, 3-butanedione 2-monoxime on contraction of frog skeletal muscle fibres. J Muscle Res Cell Motil. 1988 Apr;9(2):156–164. doi: 10.1007/BF01773737. [DOI] [PubMed] [Google Scholar]
- Hui C. S., Maylie J. Multiple actions of 2,3-butanedione monoxime on contractile activation in frog twitch fibres. J Physiol. 1991 Oct;442:527–549. doi: 10.1113/jphysiol.1991.sp018807. [DOI] [PMC free article] [PubMed] [Google Scholar]
- Julian F. J., Sollins M. R., Moss R. L. Sarcomere length non-uniformity in relation to tetanic responses of stretched skeletal muscle fibres. Proc R Soc Lond B Biol Sci. 1978 Jan 24;200(1138):109–116. doi: 10.1098/rspb.1978.0009. [DOI] [PubMed] [Google Scholar]
- Kawai M., Güth K., Winnikes K., Haist C., Rüegg J. C. The effect of inorganic phosphate on the ATP hydrolysis rate and the tension transients in chemically skinned rabbit psoas fibers. Pflugers Arch. 1987 Jan;408(1):1–9. doi: 10.1007/BF00581833. [DOI] [PubMed] [Google Scholar]
- Kodama T. Thermodynamic analysis of muscle ATPase mechanisms. Physiol Rev. 1985 Apr;65(2):467–551. doi: 10.1152/physrev.1985.65.2.467. [DOI] [PubMed] [Google Scholar]
- Lännergren J., Elzinga G., Stienen G. J. Force relaxation, labile heat and parvalbumin content of skeletal muscle fibres of Xenopus laevis. J Physiol. 1993 Apr;463:123–140. doi: 10.1113/jphysiol.1993.sp019587. [DOI] [PMC free article] [PubMed] [Google Scholar]
- Maylie J., Hui C. S. Action of 2,3-butanedione monoxime on calcium signals in frog cut twitch fibres containing antipyrylazo III. J Physiol. 1991 Oct;442:551–567. doi: 10.1113/jphysiol.1991.sp018808. [DOI] [PMC free article] [PubMed] [Google Scholar]
- Mulieri L. A., Luhr G., Trefry J., Alpert N. R. Metal-film thermopiles for use with rabbit right ventricular papillary muscles. Am J Physiol. 1977 Nov;233(5):C146–C156. doi: 10.1152/ajpcell.1977.233.5.C146. [DOI] [PubMed] [Google Scholar]
- Nagesser A. S., van der Laarse W. J., Elzinga G. Metabolic changes with fatigue in different types of single muscle fibres of Xenopus laevis. J Physiol. 1992 Mar;448:511–523. doi: 10.1113/jphysiol.1992.sp019054. [DOI] [PMC free article] [PubMed] [Google Scholar]
- PAGE S. G., HUXLEY H. E. FILAMENT LENGTHS IN STRIATED MUSCLE. J Cell Biol. 1963 Nov;19:369–390. doi: 10.1083/jcb.19.2.369. [DOI] [PMC free article] [PubMed] [Google Scholar]
- Peckham M., Woledge R. C. Labile heat and changes in rate of relaxation of frog muscles. J Physiol. 1986 May;374:123–135. doi: 10.1113/jphysiol.1986.sp016070. [DOI] [PMC free article] [PubMed] [Google Scholar]
- Potma E. J., Stienen G. J., Barends J. P., Elzinga G. Myofibrillar ATPase activity and mechanical performance of skinned fibres from rabbit psoas muscle. J Physiol. 1994 Jan 15;474(2):303–317. doi: 10.1113/jphysiol.1994.sp020023. [DOI] [PMC free article] [PubMed] [Google Scholar]
- Rall J. A. Energetics of Ca2+ cycling during skeletal muscle contraction. Fed Proc. 1982 Feb;41(2):155–160. [PubMed] [Google Scholar]
- Siegman M. J., Mooers S. U., Warren T. B., Warshaw D. M., Ikebe M., Butler T. M. Comparison of the effects of 2,3-butanedione monoxime on force production, myosin light chain phosphorylation and chemical energy usage in intact and permeabilized smooth and skeletal muscles. J Muscle Res Cell Motil. 1994 Aug;15(4):457–472. doi: 10.1007/BF00122119. [DOI] [PubMed] [Google Scholar]
- Simonides W. S., van Hardeveld C. Identification and quantification in single muscle fibers of four isoforms of parvalbumin in the iliofibularis muscle of Xenopus laevis. Biochim Biophys Acta. 1989 Oct 5;998(2):137–144. doi: 10.1016/0167-4838(89)90265-3. [DOI] [PubMed] [Google Scholar]
- Smith I. C. Energetics of activation in frog and toad muscle. J Physiol. 1972 Feb;220(3):583–599. doi: 10.1113/jphysiol.1972.sp009724. [DOI] [PMC free article] [PubMed] [Google Scholar]
- Smith R. S., Ovalle W. K., Jr Varieties of fast and slow extrafusal muscle fibres in amphibian hind limb muscles. J Anat. 1973 Oct;116(Pt 1):1–24. [PMC free article] [PubMed] [Google Scholar]
- Smith S. J., Woledge R. C. Thermodynamic analysis of calcium binding to frog parvalbumin. J Muscle Res Cell Motil. 1985 Dec;6(6):757–768. doi: 10.1007/BF00712240. [DOI] [PubMed] [Google Scholar]
- Stephenson D. G., Wendt I. R. Length dependence of changes in sarcoplasmic calcium concentration and myofibrillar calcium sensitivity in striated muscle fibres. J Muscle Res Cell Motil. 1984 Jun;5(3):243–272. doi: 10.1007/BF00713107. [DOI] [PubMed] [Google Scholar]
- Stienen G. J., Zaremba R., Elzinga G. ATP utilization for calcium uptake and force production in skinned muscle fibres of Xenopus laevis. J Physiol. 1995 Jan 1;482(Pt 1):109–122. doi: 10.1113/jphysiol.1995.sp020503. [DOI] [PMC free article] [PubMed] [Google Scholar]
- Tanokura M., Yamada K. Heat capacity and entropy changes of the two major isotypes of bullfrog (Rana catesbeiana) parvalbumins induced by calcium binding. Biochemistry. 1987 Dec 1;26(24):7668–7674. doi: 10.1021/bi00398a020. [DOI] [PubMed] [Google Scholar]
- Westerblad H., Allen D. G. Myoplasmic Mg2+ concentration in Xenopus muscle fibres at rest, during fatigue and during metabolic blockade. Exp Physiol. 1992 Sep;77(5):733–740. doi: 10.1113/expphysiol.1992.sp003639. [DOI] [PubMed] [Google Scholar]
- Woledge R. C. The energetics of tortoise muscle. J Physiol. 1968 Aug;197(3):685–707. doi: 10.1113/jphysiol.1968.sp008582. [DOI] [PMC free article] [PubMed] [Google Scholar]