Abstract
1. A novel slowly activating voltage-dependent K+ current was observed in isolated nerve terminals from rat neurohypophysis using the whole-cell configuration of the patch-clamp technique. 2. The activation kinetics of the slow current could be fitted assuming Hodgkin--Huxley-type kinetics, an exponential, n, of 1.3 and activation time constants decreasing from 4 s at -50 mV to 0.7s at +40 mV. 3. A positive shift of reversal potential was observed when [K+] was increased in the bath solution. The current is carried mainly but not exclusively by K+ ions. 4. When intracellular free [Mg2+] was low (approximately 60 microM), average current density was 74 pA pF-1 at membrane potentials around 0 mV. In 83% of nerve terminals current amplitude was > 10 pA pF-1. 5. The slow current was never observed when the pipette contained 4.6 mM free Mg2+. At a physiological level of free Mg2+ (0.5 mM) the average current density was 16 pA pF-1. 6. When nerve terminals were analysed after patch-clamp experiments for vasopressin content by immunodetection, no difference in current amplitude was found between the terminals containing vasopressin and all analysed terminals. 7. The voltage dependence of activation was fitted by a Boltzmann equation giving a half-activation potential of -37 mV and a slope factor of about 9 mV. 8. Tail current deactivation kinetics was biexponential with time constants of 0.12 and 1.5s. Kinetics was dependent on the duration of the activating pulse. 9. Noise analysis of the slow current indicated a single-channel current of 0.33 pA at +6 mV, corresponding to a single-channel conductance of 4.3 pS. 10. This is the first demonstration of a current similar to the slow K+ current, IKs, in a neurone, suggesting that a protein similar to the IKs-inducing channel protein IsK (minK) may be present in peptidergic nerve terminals. 11. The activation properties are consistent with a role of the slow current in inhibition of excitability, at least at the level of the nerve terminal.
Full text
PDF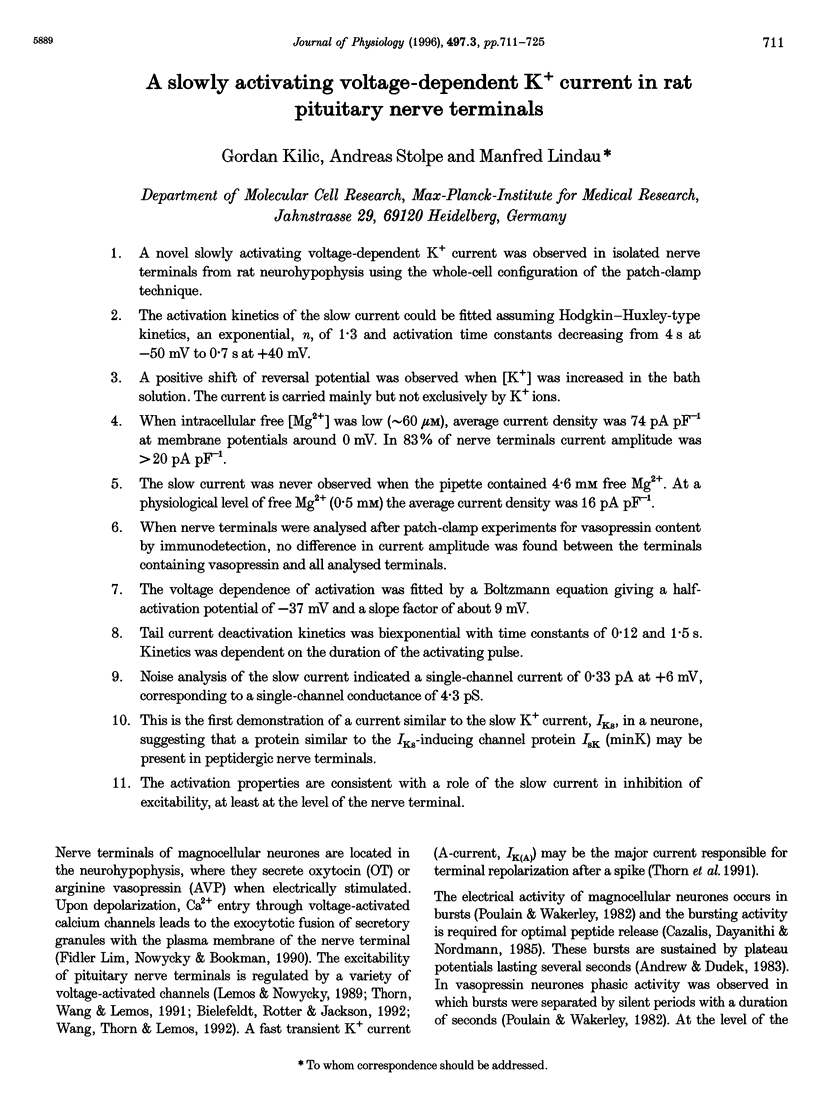
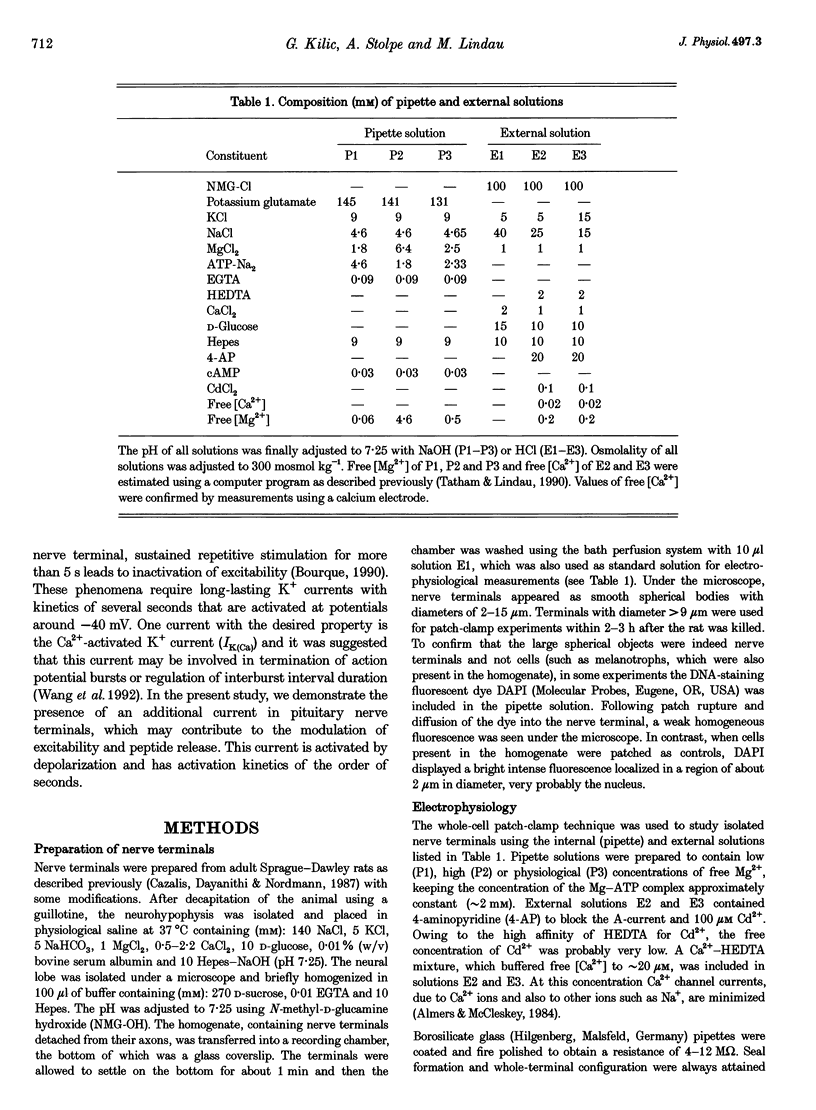
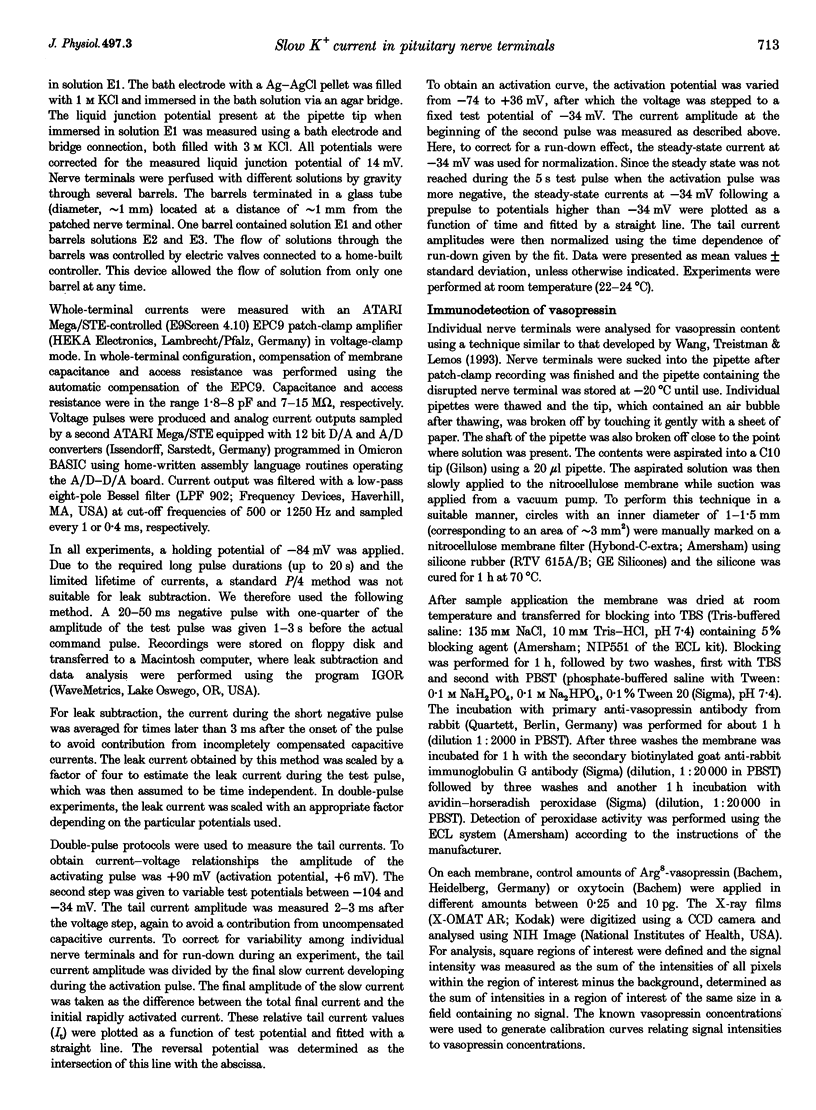
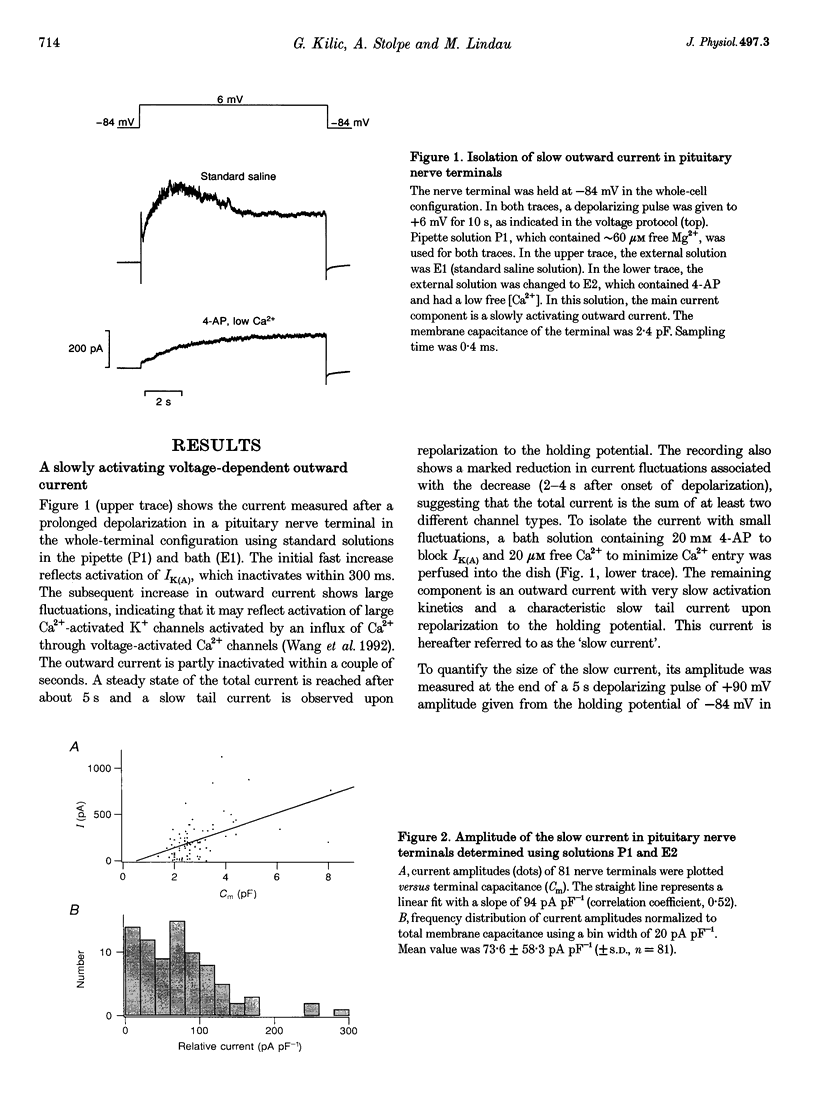
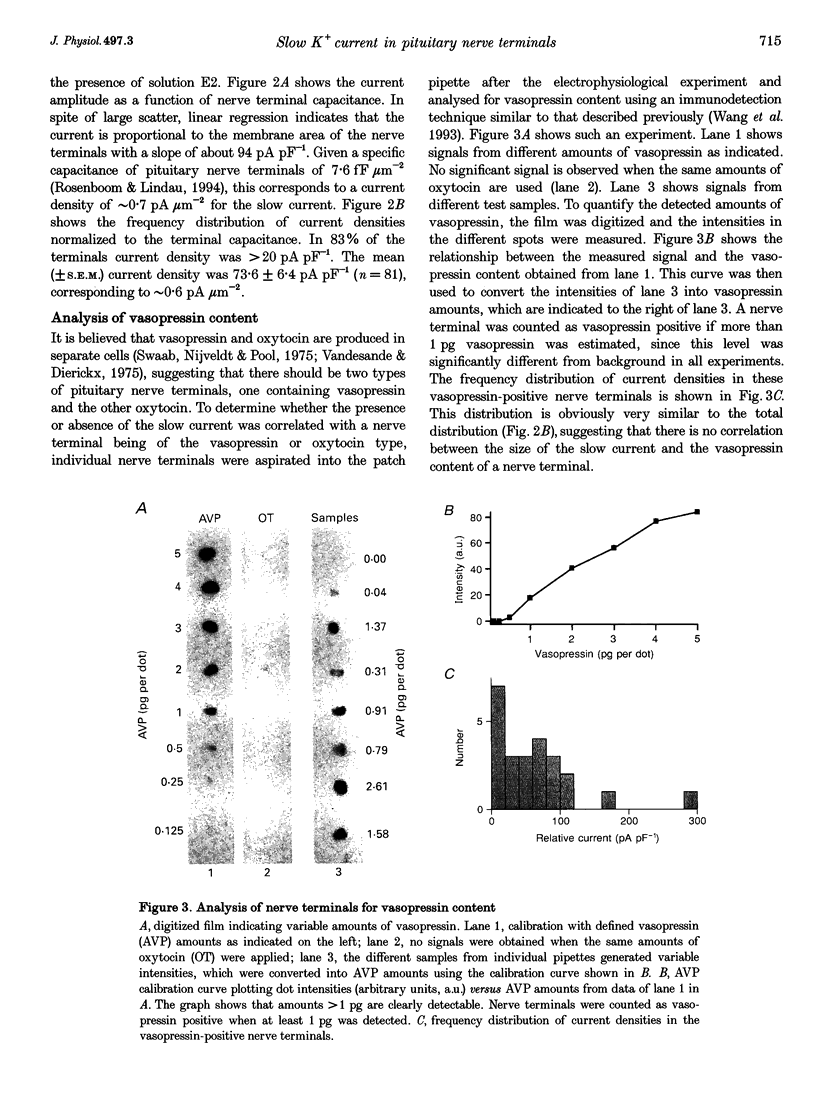
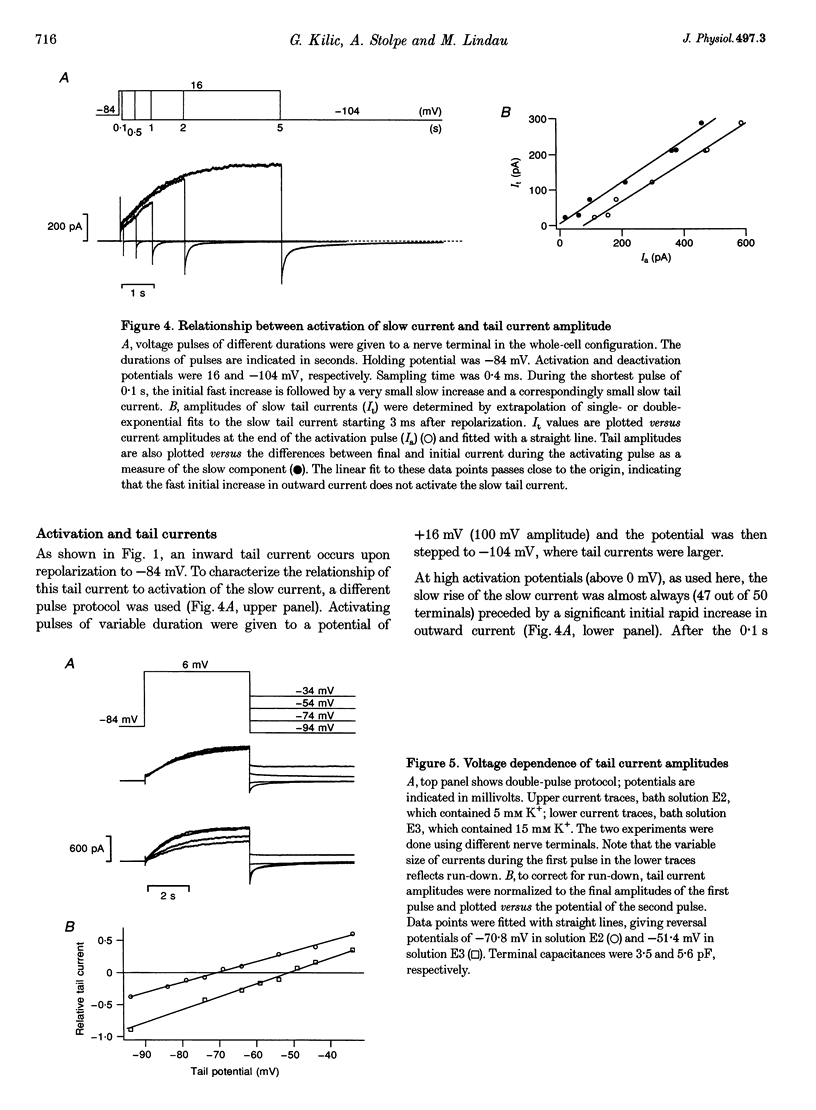
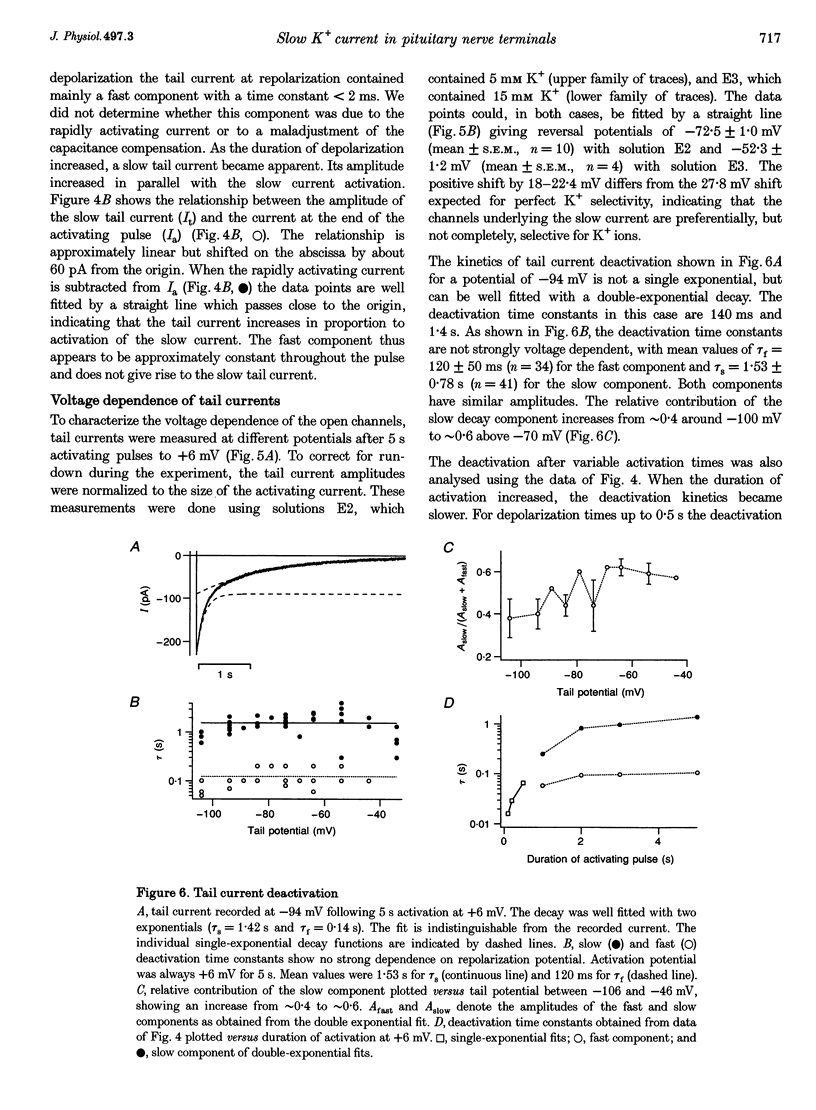
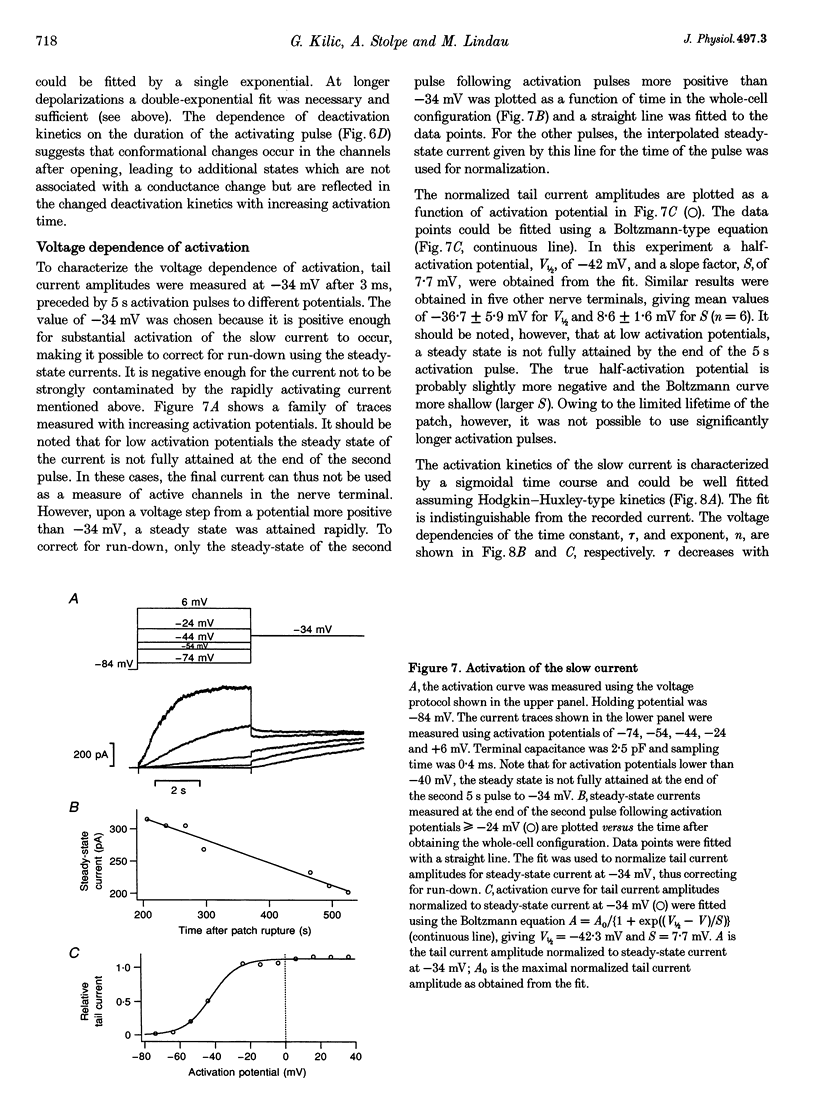
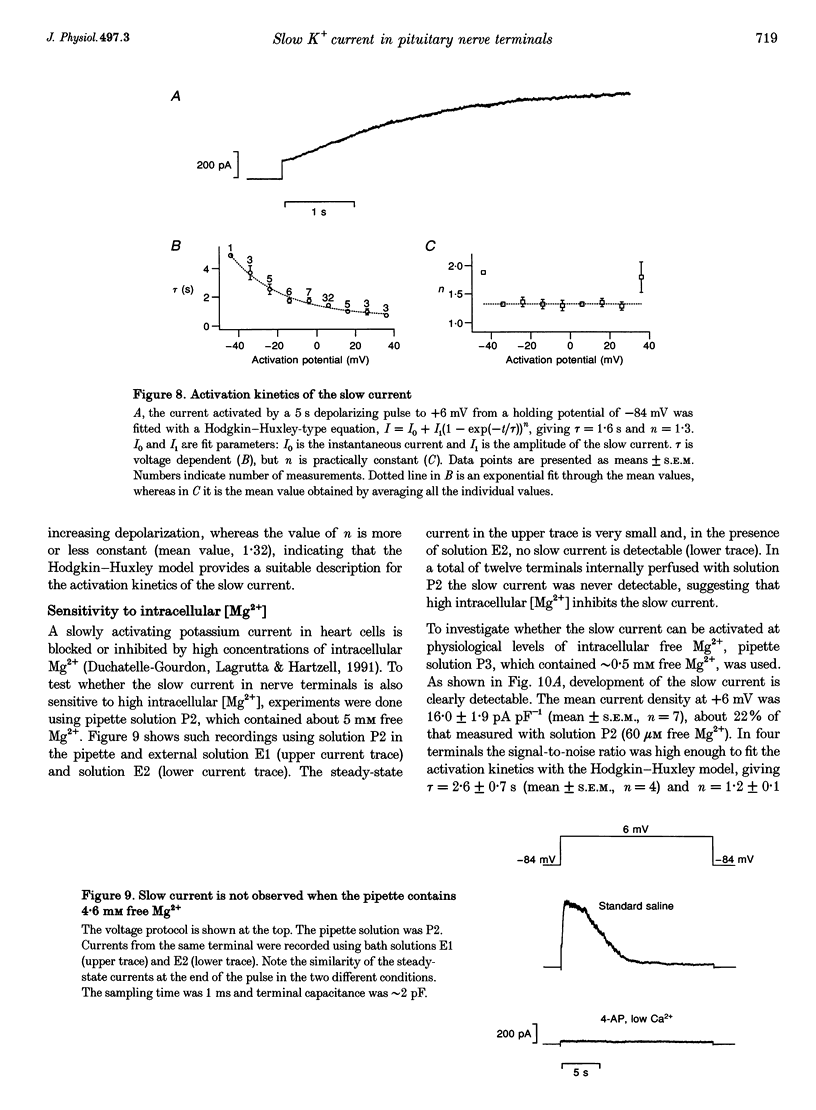
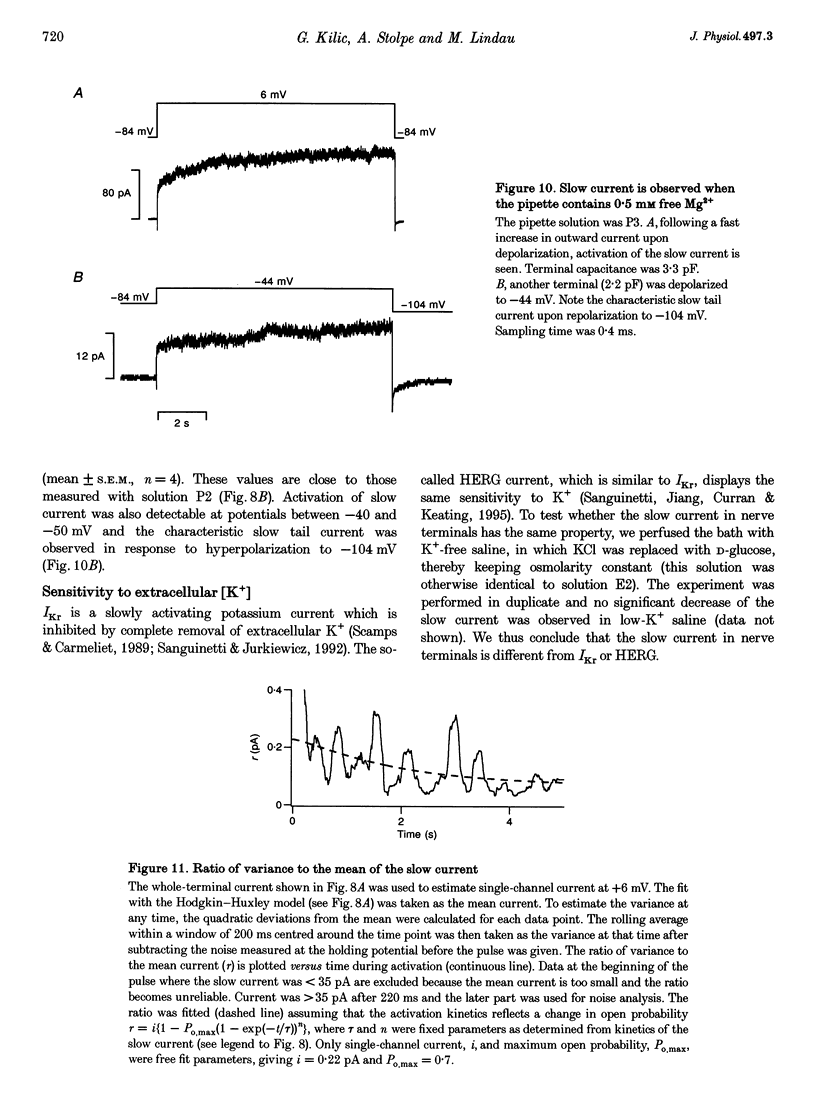
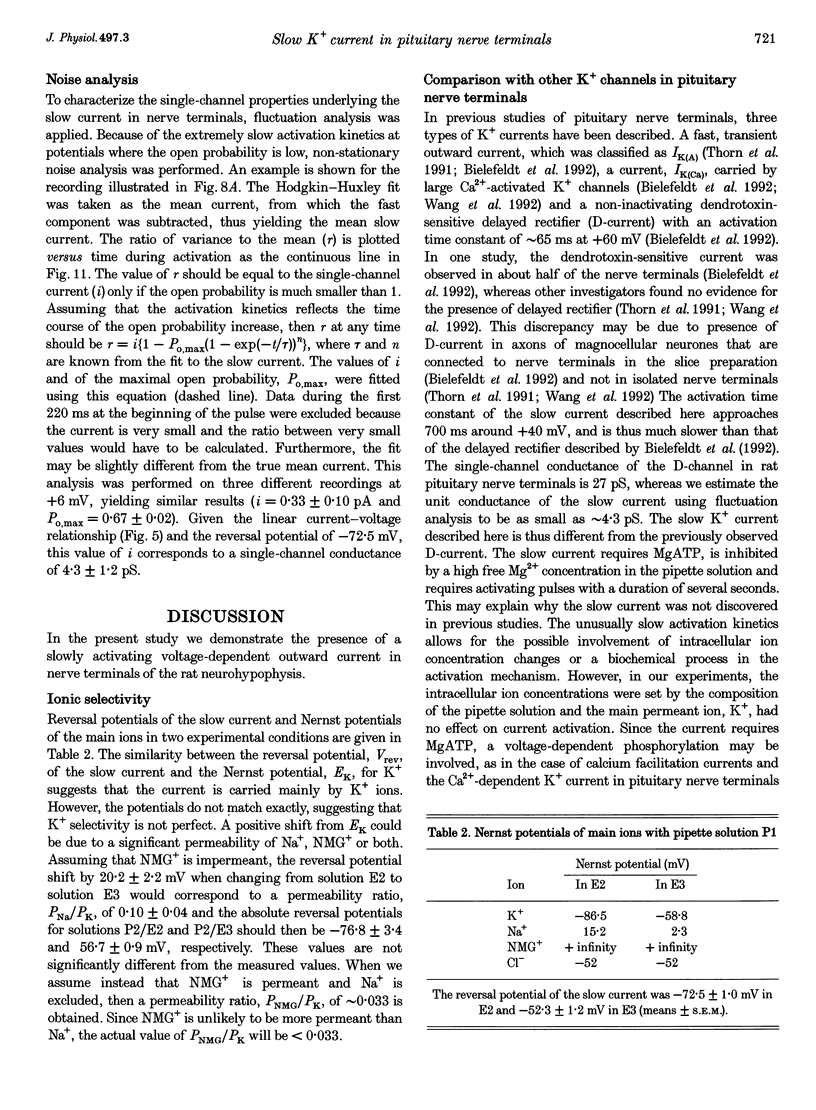
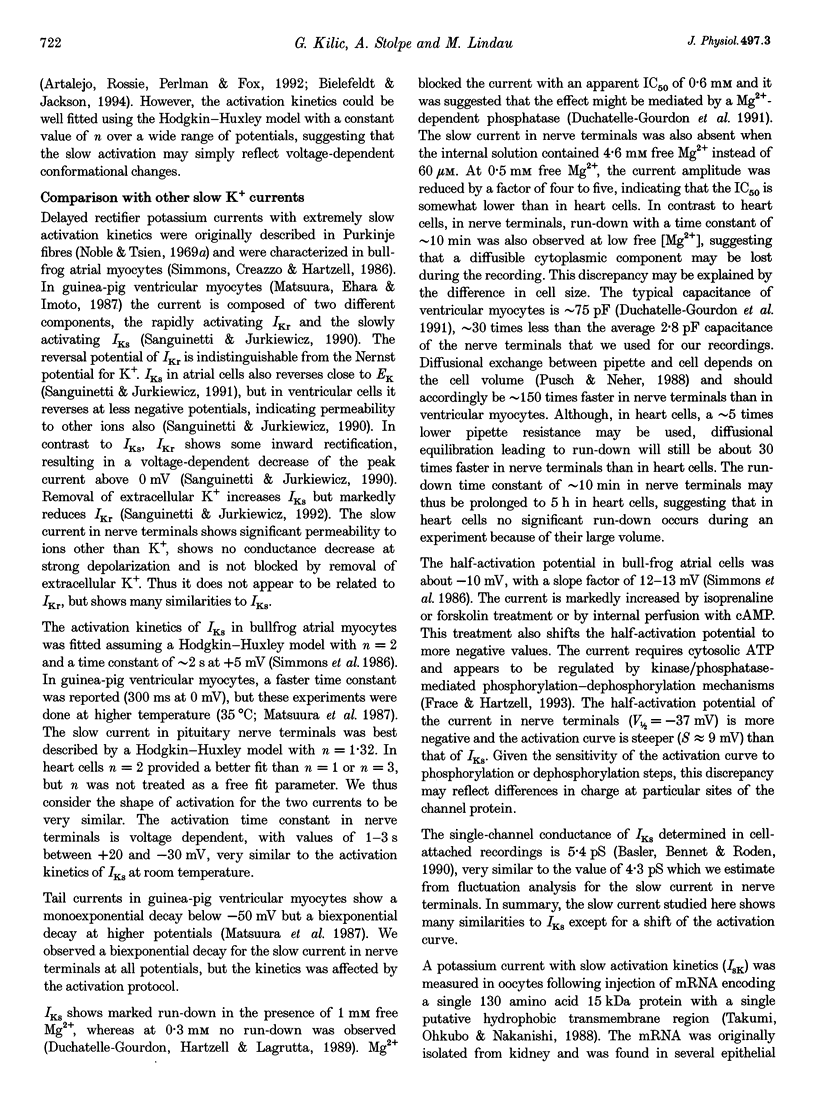
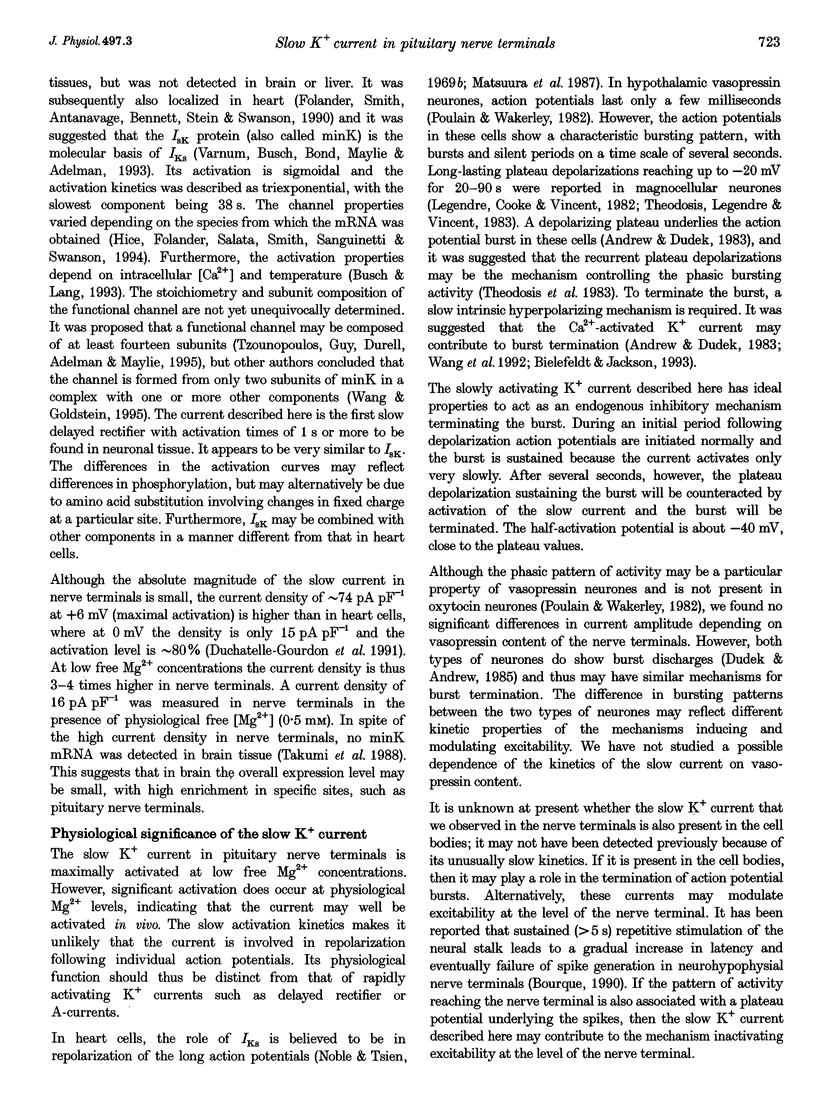
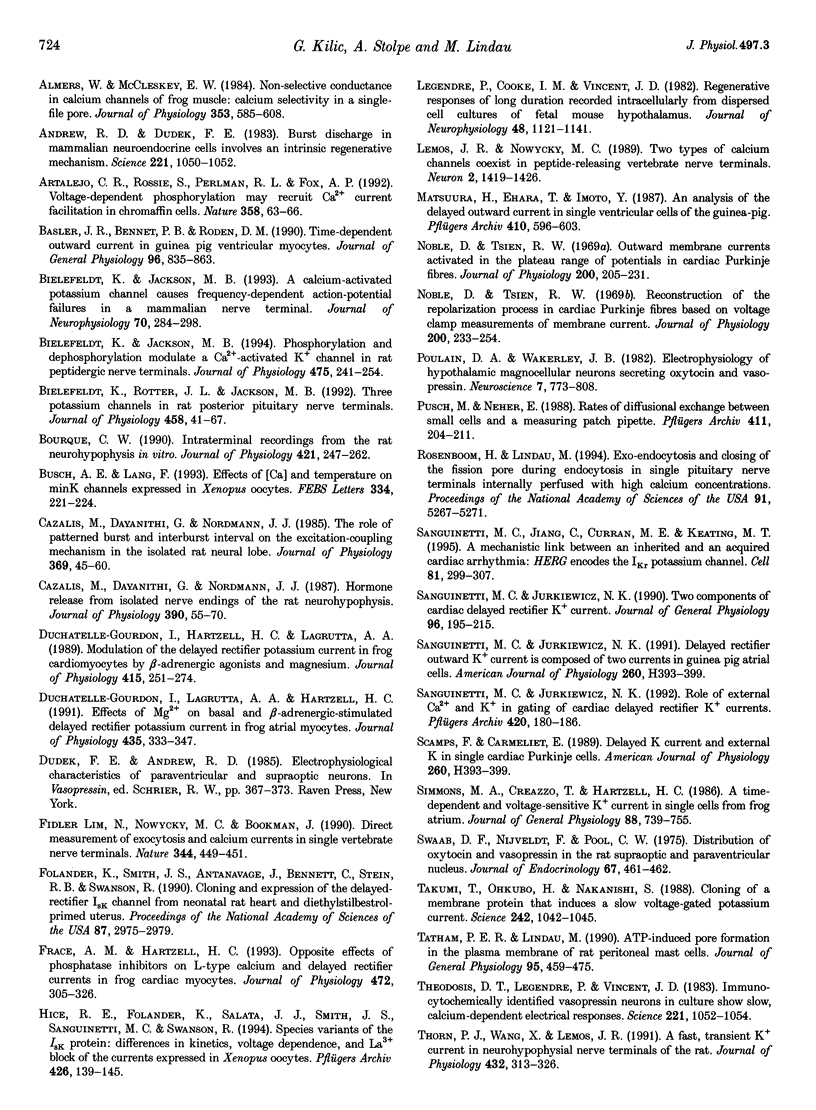
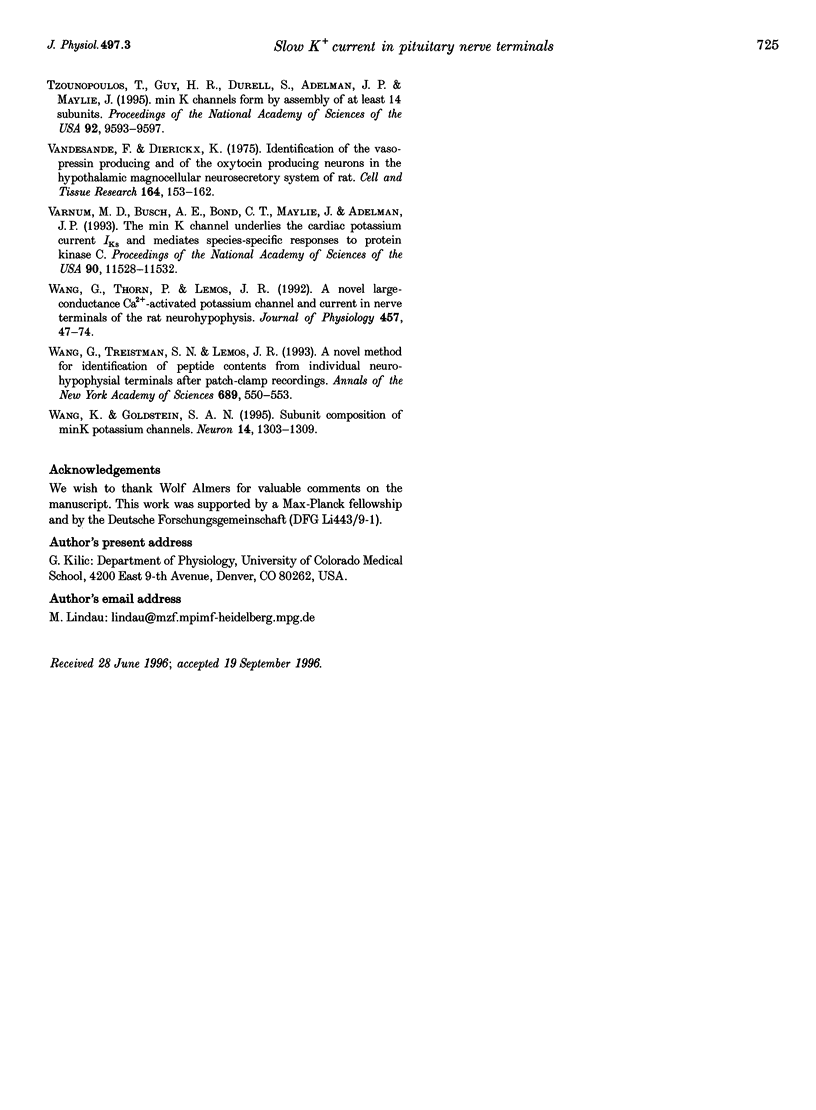
Images in this article
Selected References
These references are in PubMed. This may not be the complete list of references from this article.
- Almers W., McCleskey E. W. Non-selective conductance in calcium channels of frog muscle: calcium selectivity in a single-file pore. J Physiol. 1984 Aug;353:585–608. doi: 10.1113/jphysiol.1984.sp015352. [DOI] [PMC free article] [PubMed] [Google Scholar]
- Andrew R. D., Dudek F. E. Burst discharge in mammalian neuroendocrine cells involves an intrinsic regenerative mechanism. Science. 1983 Sep 9;221(4615):1050–1052. doi: 10.1126/science.6879204. [DOI] [PubMed] [Google Scholar]
- Artalejo C. R., Rossie S., Perlman R. L., Fox A. P. Voltage-dependent phosphorylation may recruit Ca2+ current facilitation in chromaffin cells. Nature. 1992 Jul 2;358(6381):63–66. doi: 10.1038/358063a0. [DOI] [PubMed] [Google Scholar]
- Balser J. R., Bennett P. B., Roden D. M. Time-dependent outward current in guinea pig ventricular myocytes. Gating kinetics of the delayed rectifier. J Gen Physiol. 1990 Oct;96(4):835–863. doi: 10.1085/jgp.96.4.835. [DOI] [PMC free article] [PubMed] [Google Scholar]
- Bielefeldt K., Jackson M. B. A calcium-activated potassium channel causes frequency-dependent action-potential failures in a mammalian nerve terminal. J Neurophysiol. 1993 Jul;70(1):284–298. doi: 10.1152/jn.1993.70.1.284. [DOI] [PubMed] [Google Scholar]
- Bielefeldt K., Jackson M. B. Phosphorylation and dephosphorylation modulate a Ca(2+)-activated K+ channel in rat peptidergic nerve terminals. J Physiol. 1994 Mar 1;475(2):241–254. doi: 10.1113/jphysiol.1994.sp020065. [DOI] [PMC free article] [PubMed] [Google Scholar]
- Bielefeldt K., Rotter J. L., Jackson M. B. Three potassium channels in rat posterior pituitary nerve terminals. J Physiol. 1992 Dec;458:41–67. doi: 10.1113/jphysiol.1992.sp019405. [DOI] [PMC free article] [PubMed] [Google Scholar]
- Bourque C. W. Intraterminal recordings from the rat neurohypophysis in vitro. J Physiol. 1990 Feb;421:247–262. doi: 10.1113/jphysiol.1990.sp017943. [DOI] [PMC free article] [PubMed] [Google Scholar]
- Busch A. E., Lang F. Effects of [Ca2+]i and temperature on minK channels expressed in Xenopus oocytes. FEBS Lett. 1993 Nov 15;334(2):221–224. doi: 10.1016/0014-5793(93)81715-c. [DOI] [PubMed] [Google Scholar]
- Cazalis M., Dayanithi G., Nordmann J. J. Hormone release from isolated nerve endings of the rat neurohypophysis. J Physiol. 1987 Sep;390:55–70. doi: 10.1113/jphysiol.1987.sp016686. [DOI] [PMC free article] [PubMed] [Google Scholar]
- Cazalis M., Dayanithi G., Nordmann J. J. The role of patterned burst and interburst interval on the excitation-coupling mechanism in the isolated rat neural lobe. J Physiol. 1985 Dec;369:45–60. doi: 10.1113/jphysiol.1985.sp015887. [DOI] [PMC free article] [PubMed] [Google Scholar]
- Duchatelle-Gourdon I., Hartzell H. C., Lagrutta A. A. Modulation of the delayed rectifier potassium current in frog cardiomyocytes by beta-adrenergic agonists and magnesium. J Physiol. 1989 Aug;415:251–274. doi: 10.1113/jphysiol.1989.sp017721. [DOI] [PMC free article] [PubMed] [Google Scholar]
- Duchatelle-Gourdon I., Lagrutta A. A., Hartzell H. C. Effects of Mg2+ on basal and beta-adrenergic-stimulated delayed rectifier potassium current in frog atrial myocytes. J Physiol. 1991 Apr;435:333–347. doi: 10.1113/jphysiol.1991.sp018513. [DOI] [PMC free article] [PubMed] [Google Scholar]
- Folander K., Smith J. S., Antanavage J., Bennett C., Stein R. B., Swanson R. Cloning and expression of the delayed-rectifier IsK channel from neonatal rat heart and diethylstilbestrol-primed rat uterus. Proc Natl Acad Sci U S A. 1990 Apr;87(8):2975–2979. doi: 10.1073/pnas.87.8.2975. [DOI] [PMC free article] [PubMed] [Google Scholar]
- Frace A. M., Hartzell H. C. Opposite effects of phosphatase inhibitors on L-type calcium and delayed rectifier currents in frog cardiac myocytes. J Physiol. 1993 Dec;472:305–326. doi: 10.1113/jphysiol.1993.sp019948. [DOI] [PMC free article] [PubMed] [Google Scholar]
- Hice R. E., Folander K., Salata J. J., Smith J. S., Sanguinetti M. C., Swanson R. Species variants of the IsK protein: differences in kinetics, voltage dependence, and La3+ block of the currents expressed in Xenopus oocytes. Pflugers Arch. 1994 Jan;426(1-2):139–145. doi: 10.1007/BF00374681. [DOI] [PubMed] [Google Scholar]
- Legendre P., Cooke I. M., Vincent J. D. Regenerative responses of long duration recorded intracellularly from dispersed cell cultures of fetal mouse hypothalamus. J Neurophysiol. 1982 Nov;48(5):1121–1141. doi: 10.1152/jn.1982.48.5.1121. [DOI] [PubMed] [Google Scholar]
- Lemos J. R., Nowycky M. C. Two types of calcium channels coexist in peptide-releasing vertebrate nerve terminals. Neuron. 1989 May;2(5):1419–1426. doi: 10.1016/0896-6273(89)90187-6. [DOI] [PubMed] [Google Scholar]
- Lim N. F., Nowycky M. C., Bookman R. J. Direct measurement of exocytosis and calcium currents in single vertebrate nerve terminals. Nature. 1990 Mar 29;344(6265):449–451. doi: 10.1038/344449a0. [DOI] [PubMed] [Google Scholar]
- Matsuura H., Ehara T., Imoto Y. An analysis of the delayed outward current in single ventricular cells of the guinea-pig. Pflugers Arch. 1987 Dec;410(6):596–603. doi: 10.1007/BF00581319. [DOI] [PubMed] [Google Scholar]
- Noble D., Tsien R. W. Outward membrane currents activated in the plateau range of potentials in cardiac Purkinje fibres. J Physiol. 1969 Jan;200(1):205–231. doi: 10.1113/jphysiol.1969.sp008689. [DOI] [PMC free article] [PubMed] [Google Scholar]
- Noble D., Tsien R. W. Reconstruction of the repolarization process in cardiac Purkinje fibres based on voltage clamp measurements of membrane current. J Physiol. 1969 Jan;200(1):233–254. doi: 10.1113/jphysiol.1969.sp008690. [DOI] [PMC free article] [PubMed] [Google Scholar]
- Poulain D. A., Wakerley J. B. Electrophysiology of hypothalamic magnocellular neurones secreting oxytocin and vasopressin. Neuroscience. 1982 Apr;7(4):773–808. doi: 10.1016/0306-4522(82)90044-6. [DOI] [PubMed] [Google Scholar]
- Pusch M., Neher E. Rates of diffusional exchange between small cells and a measuring patch pipette. Pflugers Arch. 1988 Feb;411(2):204–211. doi: 10.1007/BF00582316. [DOI] [PubMed] [Google Scholar]
- Rosenboom H., Lindau M. Exo-endocytosis and closing of the fission pore during endocytosis in single pituitary nerve terminals internally perfused with high calcium concentrations. Proc Natl Acad Sci U S A. 1994 Jun 7;91(12):5267–5271. doi: 10.1073/pnas.91.12.5267. [DOI] [PMC free article] [PubMed] [Google Scholar]
- Sanguinetti M. C., Jiang C., Curran M. E., Keating M. T. A mechanistic link between an inherited and an acquired cardiac arrhythmia: HERG encodes the IKr potassium channel. Cell. 1995 Apr 21;81(2):299–307. doi: 10.1016/0092-8674(95)90340-2. [DOI] [PubMed] [Google Scholar]
- Sanguinetti M. C., Jurkiewicz N. K. Delayed rectifier outward K+ current is composed of two currents in guinea pig atrial cells. Am J Physiol. 1991 Feb;260(2 Pt 2):H393–H399. doi: 10.1152/ajpheart.1991.260.2.H393. [DOI] [PubMed] [Google Scholar]
- Sanguinetti M. C., Jurkiewicz N. K. Role of external Ca2+ and K+ in gating of cardiac delayed rectifier K+ currents. Pflugers Arch. 1992 Feb;420(2):180–186. doi: 10.1007/BF00374988. [DOI] [PubMed] [Google Scholar]
- Sanguinetti M. C., Jurkiewicz N. K. Two components of cardiac delayed rectifier K+ current. Differential sensitivity to block by class III antiarrhythmic agents. J Gen Physiol. 1990 Jul;96(1):195–215. doi: 10.1085/jgp.96.1.195. [DOI] [PMC free article] [PubMed] [Google Scholar]
- Simmons M. A., Creazzo T., Hartzell H. C. A time-dependent and voltage-sensitive K+ current in single cells from frog atrium. J Gen Physiol. 1986 Dec;88(6):739–755. doi: 10.1085/jgp.88.6.739. [DOI] [PMC free article] [PubMed] [Google Scholar]
- Swaab D. F., Nijveldt F., Pool C. W. Distribution of oxytocin and vasopressin in the rat supraoptic and paraventricular nucleus. J Endocrinol. 1975 Dec;67(3):461–462. doi: 10.1677/joe.0.0670461. [DOI] [PubMed] [Google Scholar]
- Takumi T., Ohkubo H., Nakanishi S. Cloning of a membrane protein that induces a slow voltage-gated potassium current. Science. 1988 Nov 18;242(4881):1042–1045. doi: 10.1126/science.3194754. [DOI] [PubMed] [Google Scholar]
- Tatham P. E., Lindau M. ATP-induced pore formation in the plasma membrane of rat peritoneal mast cells. J Gen Physiol. 1990 Mar;95(3):459–476. doi: 10.1085/jgp.95.3.459. [DOI] [PMC free article] [PubMed] [Google Scholar]
- Theodosis D. T., Legendre P., Vincent J. D., Cooke I. Immunocytochemically identified vasopressin neurons in culture show slow, calcium-dependent electrical responses. Science. 1983 Sep 9;221(4615):1052–1054. doi: 10.1126/science.6348947. [DOI] [PubMed] [Google Scholar]
- Thorn P. J., Wang X. M., Lemos J. R. A fast, transient K+ current in neurohypophysial nerve terminals of the rat. J Physiol. 1991 Jan;432:313–326. doi: 10.1113/jphysiol.1991.sp018386. [DOI] [PMC free article] [PubMed] [Google Scholar]
- Tzounopoulos T., Guy H. R., Durell S., Adelman J. P., Maylie J. min K channels form by assembly of at least 14 subunits. Proc Natl Acad Sci U S A. 1995 Oct 10;92(21):9593–9597. doi: 10.1073/pnas.92.21.9593. [DOI] [PMC free article] [PubMed] [Google Scholar]
- Vandesande F., Dierickx K. Identification of the vasopressin producing and of the oxytocin producing neurons in the hypothalamic magnocellular neurosecretroy system of the rat. Cell Tissue Res. 1975 Dec 2;164(2):153–162. doi: 10.1007/BF00218970. [DOI] [PubMed] [Google Scholar]
- Varnum M. D., Busch A. E., Bond C. T., Maylie J., Adelman J. P. The min K channel underlies the cardiac potassium current IKs and mediates species-specific responses to protein kinase C. Proc Natl Acad Sci U S A. 1993 Dec 15;90(24):11528–11532. doi: 10.1073/pnas.90.24.11528. [DOI] [PMC free article] [PubMed] [Google Scholar]
- Wang G., Thorn P., Lemos J. R. A novel large-conductance Ca(2+)-activated potassium channel and current in nerve terminals of the rat neurohypophysis. J Physiol. 1992 Nov;457:47–74. doi: 10.1113/jphysiol.1992.sp019364. [DOI] [PMC free article] [PubMed] [Google Scholar]
- Wang K. W., Goldstein S. A. Subunit composition of minK potassium channels. Neuron. 1995 Jun;14(6):1303–1309. doi: 10.1016/0896-6273(95)90277-5. [DOI] [PubMed] [Google Scholar]
- Wang X., Treistman S. N., Lemos J. R. A novel method for identification of peptide contents from individual neurohypophysial terminals after patch-clamp recordings. Ann N Y Acad Sci. 1993 Jul 22;689:550–553. doi: 10.1111/j.1749-6632.1993.tb55591.x. [DOI] [PubMed] [Google Scholar]