Abstract
The branched chain 2-oxo acid dehydrogenase from rat skeletal muscle, heart, kidney and liver mitochondria can undergo a reversible activation-inactivation cycle in vitro. Similar results were obtained with the enzyme from kidney mitochondria of pig and cow. The dehydrogenase is markedly inhibited by ATP and the inhibition is not reversed by removing the nucleotide. The non-metabolizable ATP analogue adenosine 5'-[beta gamma-imido] triphosphate can block the effect of ATP when added with the nucleotide, but has no effect by itself, nor can it reverse the inhibition in mitochondria preincubated with ATP. These findings suggest that the branched chain 2-oxo acid dehydrogenase undergoes a stable modification that requires the splitting of the ATP gamma-phosphate group. In skeletal muscle mitochondria the rate of inhibition by ATP is decreased by oxo acid substrates and enhanced by NADH. The dehydrogenase can be reactivated 10-20 fold by incubation at pH 7.8 in a buffer containing Mg2+ and cofactors. Reactivation is blocked by NaF (25 mM). The initial activity of dehydrogenase extracted from various tissues of fed rats varies considerably. Activity is near maximal in kidney and liver whereas the dehydrogenase in heart and skeletal muscle is almost completely inactivated. These studies emphasize that comparisons of branched chain 2-oxo acid dehydrogenase activity under various physiological conditions or in different tissues must take into account its state of activation. Thus the possibility exists that the branched chain 2-oxo acid dehydrogenase may be physiologically regulated via a covalent mechanism.
Full text
PDF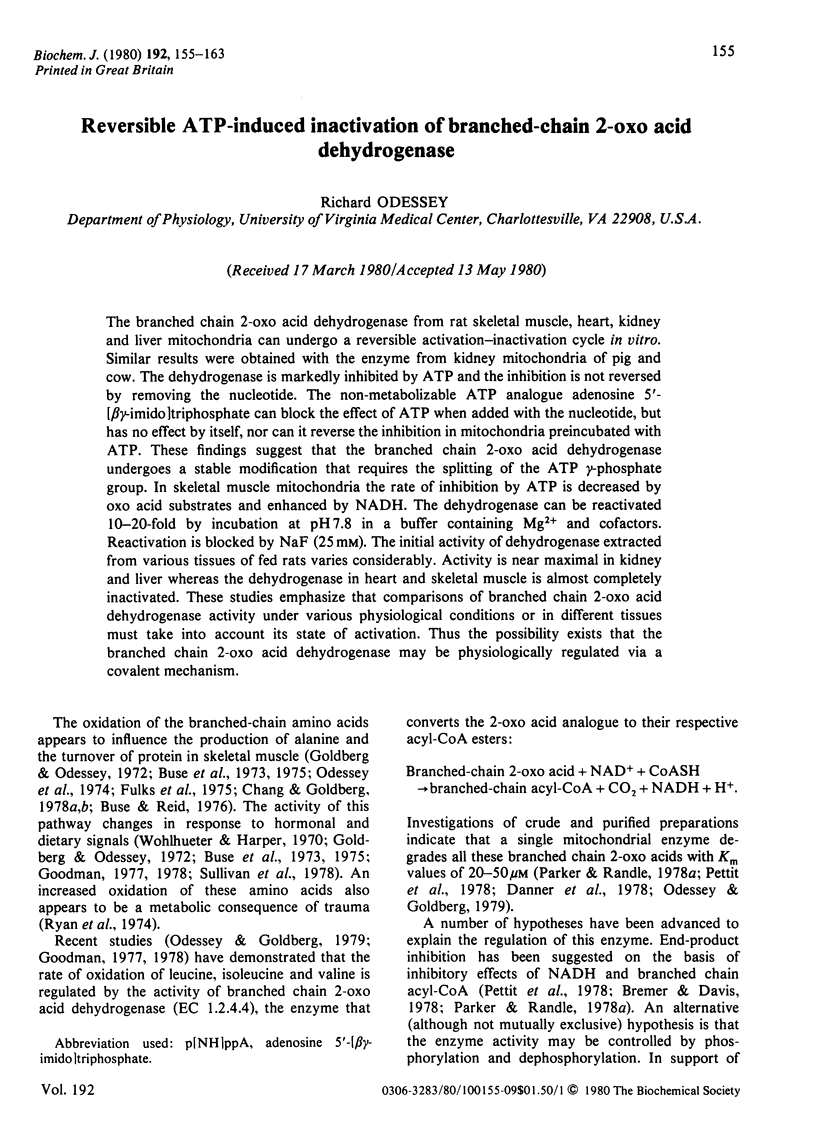
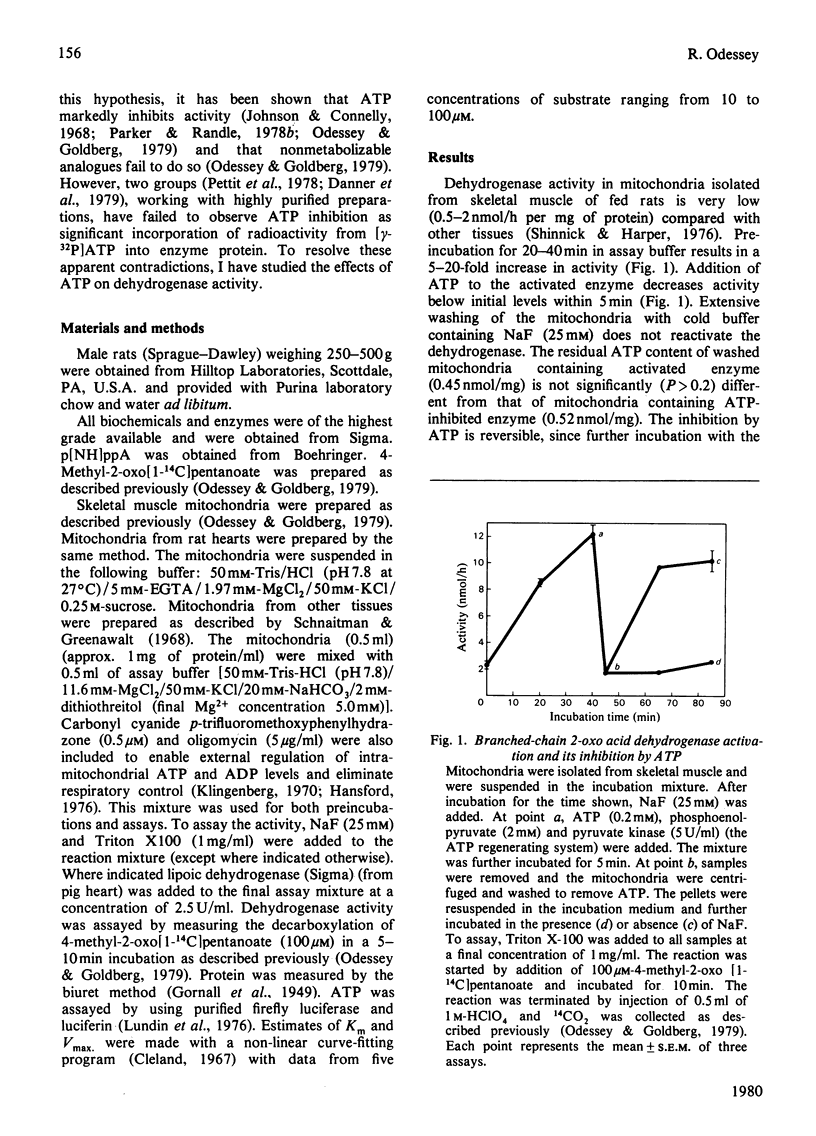
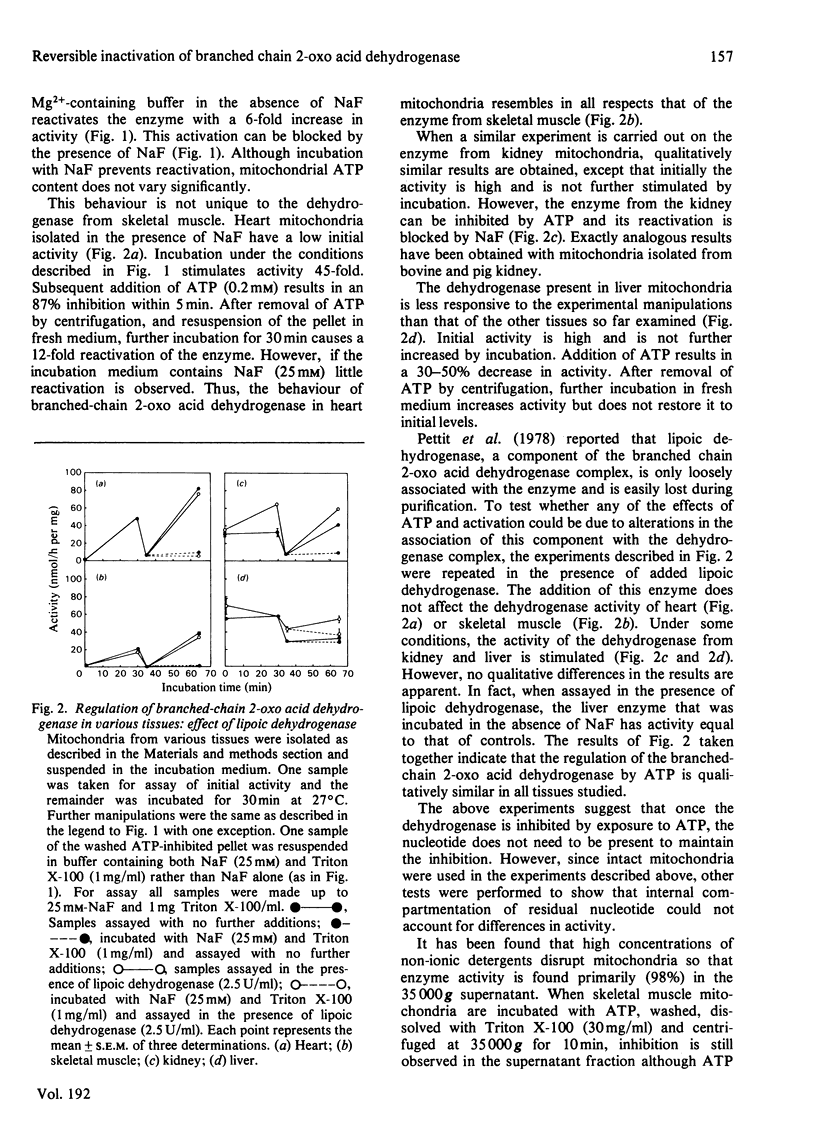
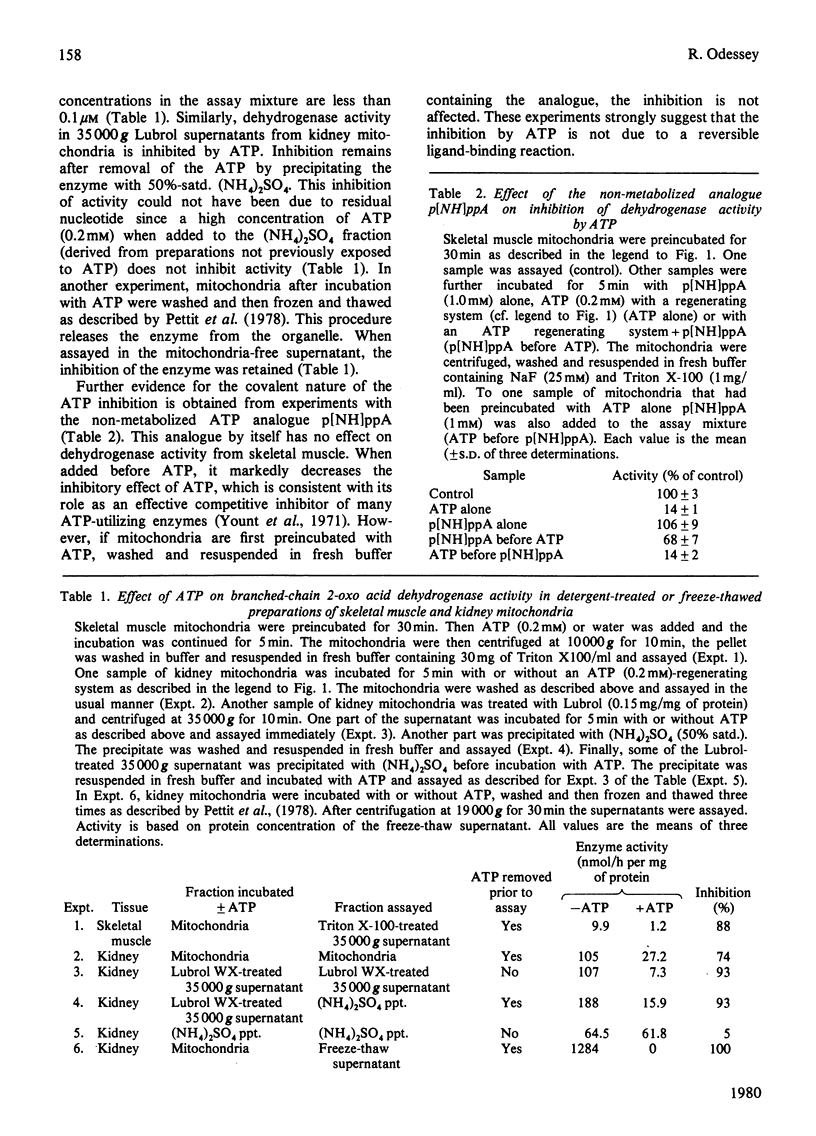
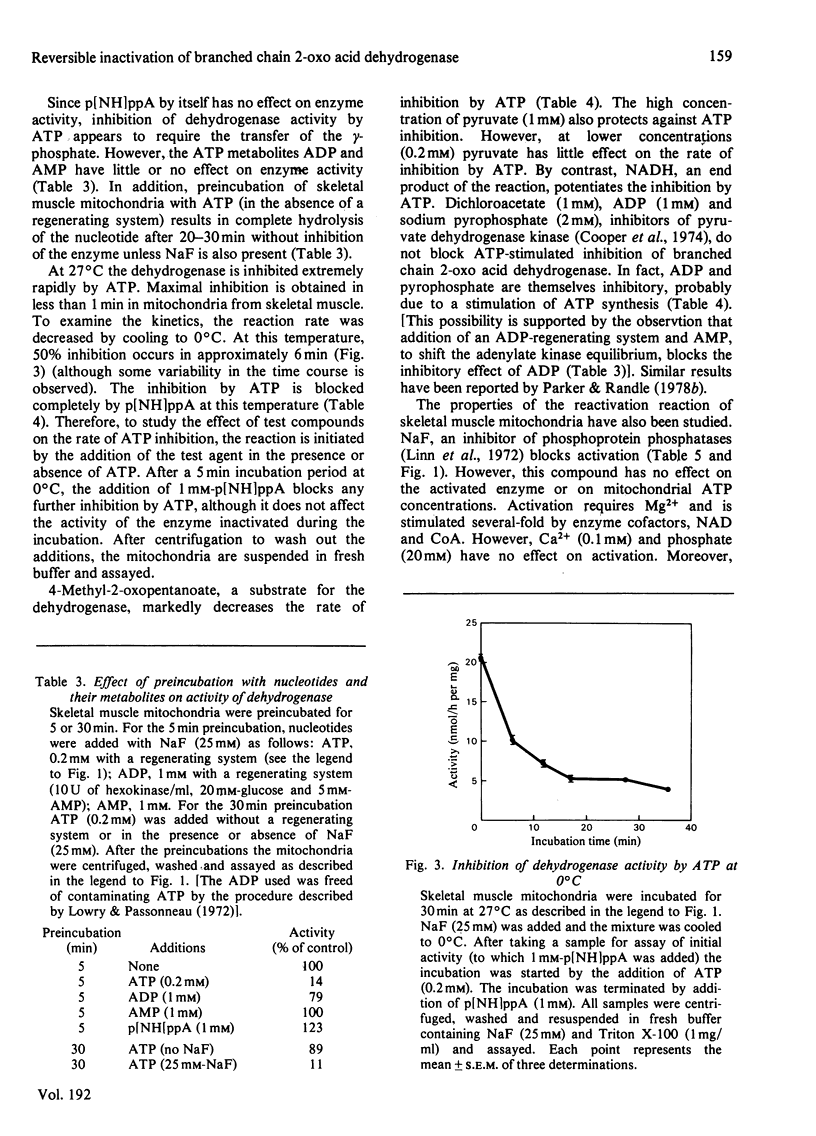
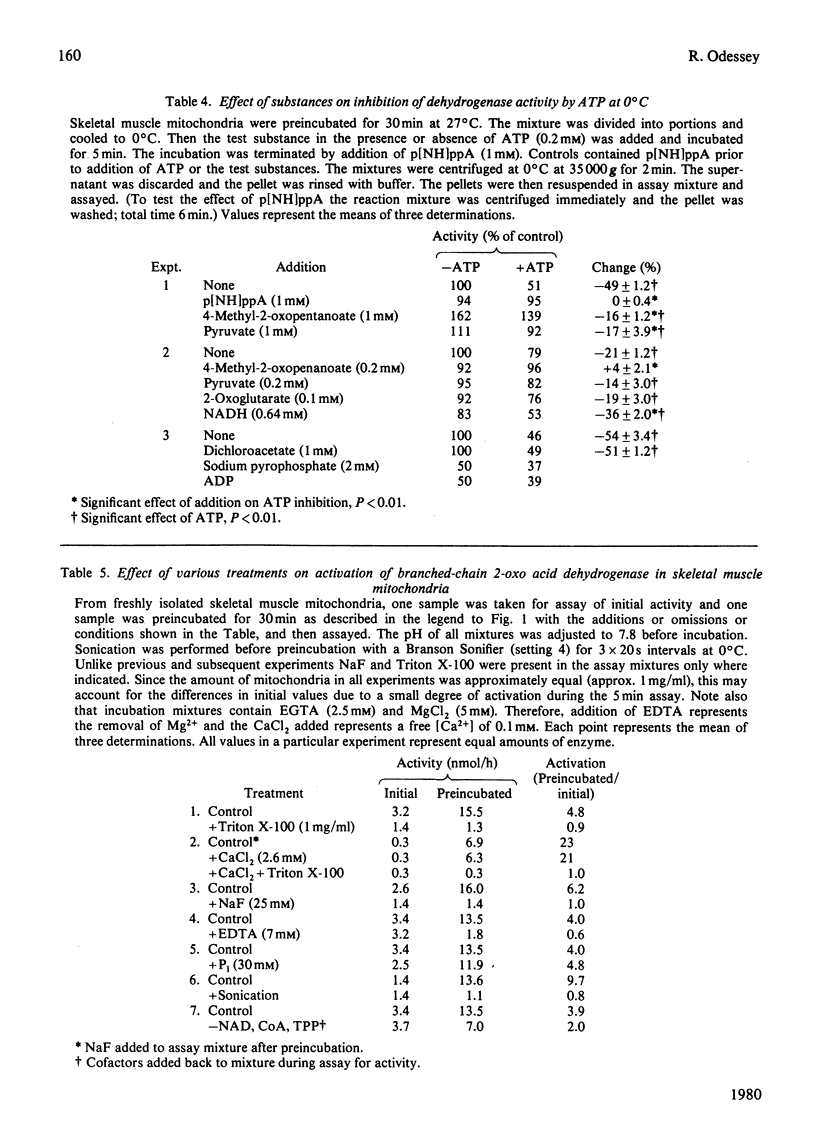
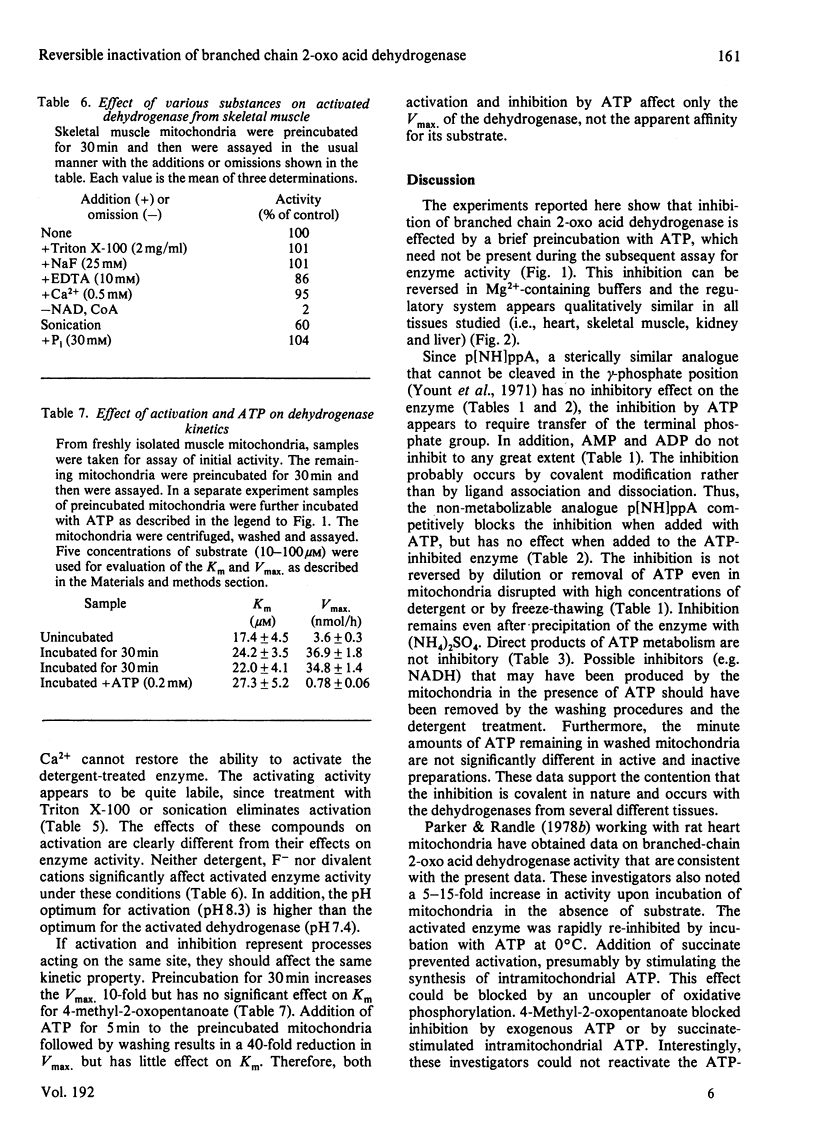
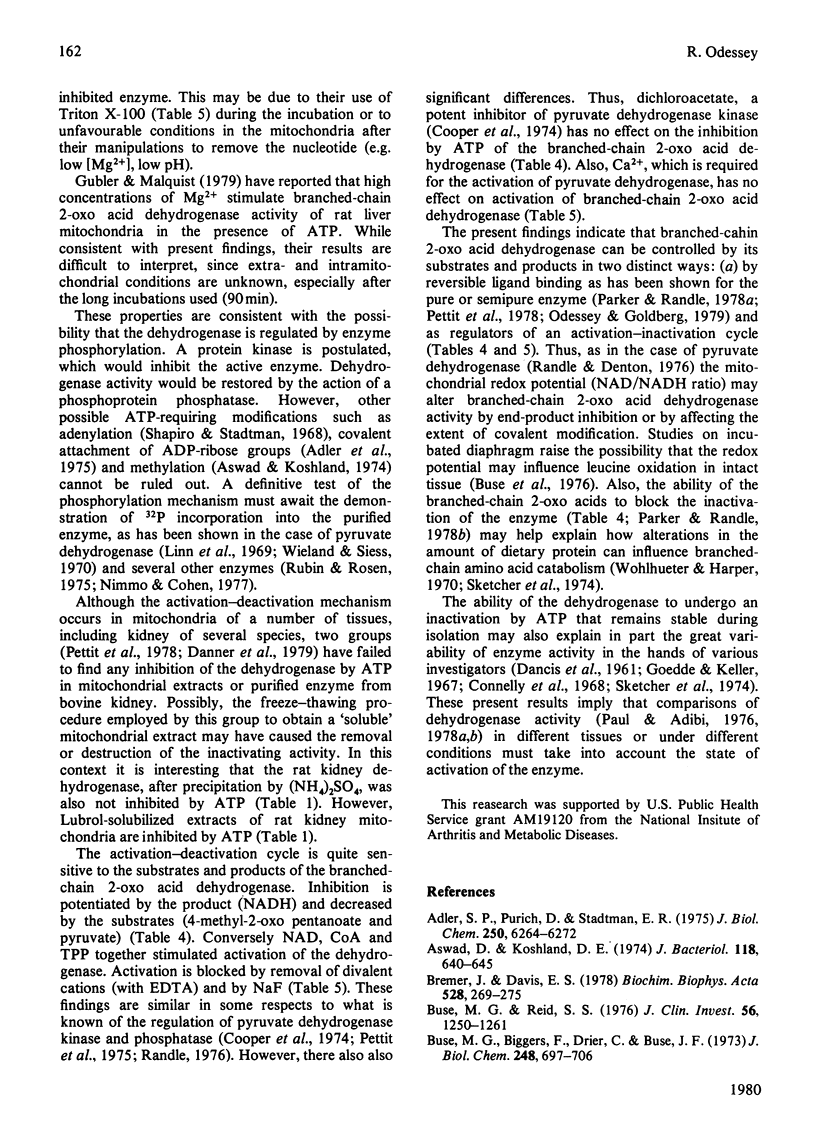
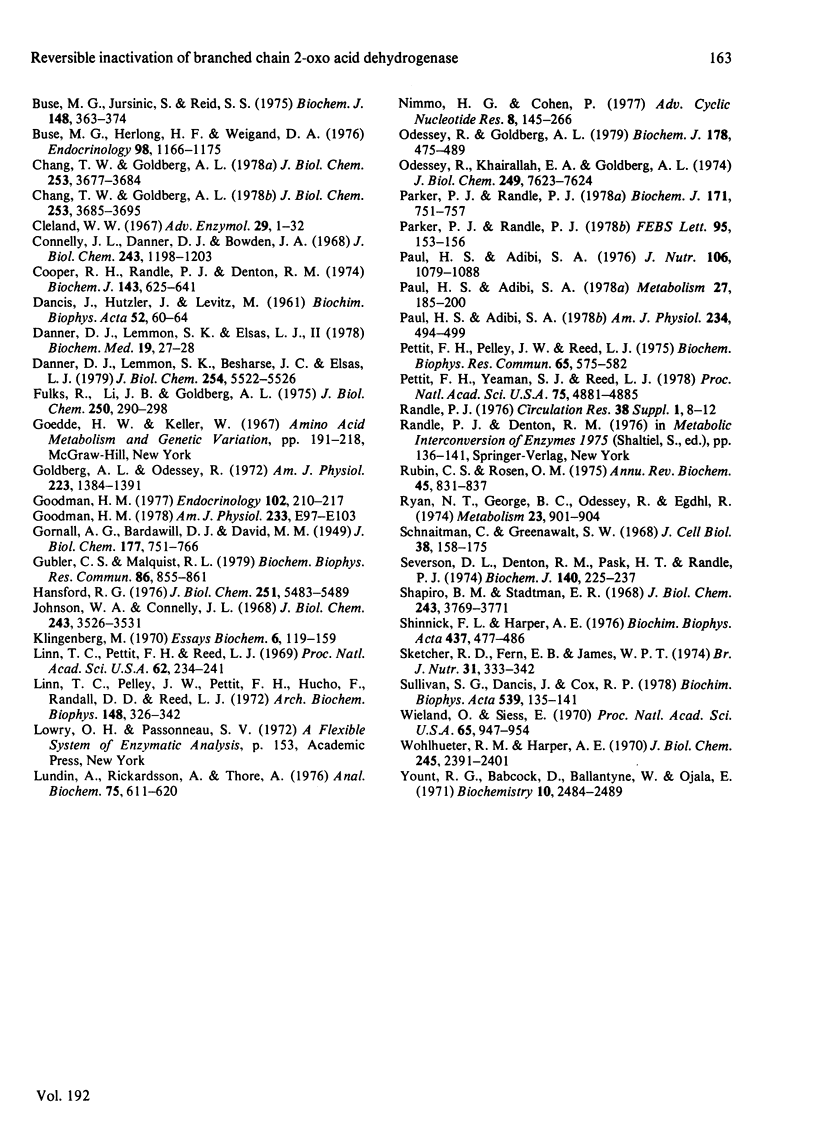
Selected References
These references are in PubMed. This may not be the complete list of references from this article.
- Adler S. P., Purich D., Stadtman E. R. Cascade control of Escherichia coli glutamine synthetase. Properties of the PII regulatory protein and the uridylyltransferase-uridylyl-removing enzyme. J Biol Chem. 1975 Aug 25;250(16):6264–6272. [PubMed] [Google Scholar]
- Aswad D., Koshland D. E., Jr Role of methionine in bacterial chemotaxis. J Bacteriol. 1974 May;118(2):640–645. doi: 10.1128/jb.118.2.640-645.1974. [DOI] [PMC free article] [PubMed] [Google Scholar]
- Bowden J. A., Connelly J. L. Branched chain alpha-keto acid metabolism. II. Evidence for the common identity of alpha-ketoisocaproic acid and alpha-keto-beta-methyl-valeric acid dehydrogenases. J Biol Chem. 1968 Jun 25;243(12):3526–3531. [PubMed] [Google Scholar]
- Bremer J., Davis E. J. The effect of acylcarnitines on the oxidation of branched chain alpha-keto acids in mitochondria. Biochim Biophys Acta. 1978 Mar 30;528(3):269–275. doi: 10.1016/0005-2760(78)90016-4. [DOI] [PubMed] [Google Scholar]
- Buse M. G., Biggers J. F., Drier C., Buse J. F. The effect of epinephrine, glucagon, and the nutritional state on the oxidation of branched chain amino acids and pyruvate by isolated hearts and diaphragms of the rat. J Biol Chem. 1973 Jan 25;248(2):697–706. [PubMed] [Google Scholar]
- Buse M. G., Herlong H. F., Weigand D. A. The effect of diabetes, insulin, and the redox potential on leucine metabolism by isolated rat hemidiaphragm. Endocrinology. 1976 May;98(5):1166–1175. doi: 10.1210/endo-98-5-1166. [DOI] [PubMed] [Google Scholar]
- Buse M. G., Jursinic S., Reid S. S. Regulation of branched-chain amino acid oxidation in isolated muscles, nerves and aortas of rats. Biochem J. 1975 Jun;148(3):363–374. doi: 10.1042/bj1480363. [DOI] [PMC free article] [PubMed] [Google Scholar]
- Buse M. G., Reid S. S. Leucine. A possible regulator of protein turnover in muscle. J Clin Invest. 1975 Nov;56(5):1250–1261. doi: 10.1172/JCI108201. [DOI] [PMC free article] [PubMed] [Google Scholar]
- Chang T. W., Goldberg A. L. The metabolic fates of amino acids and the formation of glutamine in skeletal muscle. J Biol Chem. 1978 May 25;253(10):3685–3693. [PubMed] [Google Scholar]
- Chang T. W., Goldberg A. L. The origin of alanine produced in skeletal muscle. J Biol Chem. 1978 May 25;253(10):3677–3684. [PubMed] [Google Scholar]
- Cleland W. W. The statistical analysis of enzyme kinetic data. Adv Enzymol Relat Areas Mol Biol. 1967;29:1–32. doi: 10.1002/9780470122747.ch1. [DOI] [PubMed] [Google Scholar]
- Connelly J. L., Danner D. J., Bowden J. A. Branched chain alpha-keto acid metabolism. I. Isolation, purification, and partial characterization of bovine liver alpha-ketoisocaproic:alpha-keto-beta-methylvaleric acid dehydrogenase. J Biol Chem. 1968 Mar 25;243(6):1198–1203. [PubMed] [Google Scholar]
- Cooper R. H., Randle P. J., Denton R. M. Regulation of heart muscle pyruvate dehydrogenase kinase. Biochem J. 1974 Dec;143(3):625–641. doi: 10.1042/bj1430625. [DOI] [PMC free article] [PubMed] [Google Scholar]
- DANCIS J., HUTZLER J., LEVITZ M. Tissue distribution of branched chain ketoacid decarboxylase. Biochim Biophys Acta. 1961 Sep 2;52:60–64. doi: 10.1016/0006-3002(61)90903-9. [DOI] [PubMed] [Google Scholar]
- Danner D. J., Lemmon S. K., Besharse J. C., Elsas L. J., 2nd Purification and characterization of branched chain alpha-ketoacid dehydrogenase from bovine liver mitochondria. J Biol Chem. 1979 Jun 25;254(12):5522–5526. [PubMed] [Google Scholar]
- Danner D. J., Lemmon S. K., Elsas L. J., 2nd Substrate specificity and stabilization by thiamine pyrophosphate of rat liver branched chain alpha-ketoacid dehydrogenase. Biochem Med. 1978 Feb;19(1):27–38. doi: 10.1016/0006-2944(78)90004-2. [DOI] [PubMed] [Google Scholar]
- Fulks R. M., Li J. B., Goldberg A. L. Effects of insulin, glucose, and amino acids on protein turnover in rat diaphragm. J Biol Chem. 1975 Jan 10;250(1):290–298. [PubMed] [Google Scholar]
- Goldberg A. L., Odessey R. Oxidation of amino acids by diaphragms from fed and fasted rats. Am J Physiol. 1972 Dec;223(6):1384–1391. doi: 10.1152/ajplegacy.1972.223.6.1384. [DOI] [PubMed] [Google Scholar]
- Goodman H. M. Site of action of insulin in promoting leucine utilization in adipose tissue. Am J Physiol. 1977 Aug;233(2):E97–103. doi: 10.1152/ajpendo.1977.233.2.E97. [DOI] [PubMed] [Google Scholar]
- Goodman H. M. The effects of growth hormone on the utilization of L-leucine in adipose tissue. Endocrinology. 1978 Jan;102(1):210–217. doi: 10.1210/endo-102-1-210. [DOI] [PubMed] [Google Scholar]
- Gubler C. J., Malquist R. L. Effects of conditions favoring enzyme phosphorylation and dephosphorylation on the activity of the alpha-keto acid dehydrogenases, with particular reference to the branched-chain alpha-keto acid dehydrogenase activities. Biochem Biophys Res Commun. 1979 Feb 14;86(3):855–861. doi: 10.1016/0006-291x(79)91791-1. [DOI] [PubMed] [Google Scholar]
- Hansford R. G. Studies on the effects of coenzyme A-SH: acetyl coenzyme A, nicotinamide adenine dinucleotide: reduced nicotinamide adenine dinucleotide, and adenosine diphosphate: adenosine triphosphate ratios on the interconversion of active and inactive pyruvate dehydrogenase in isolated rat heart mitochondria. J Biol Chem. 1976 Sep 25;251(18):5483–5489. [PubMed] [Google Scholar]
- Klingenberg M. Metabolite transport in mitochondria: an example for intracellular membrane function. Essays Biochem. 1970;6:119–159. [PubMed] [Google Scholar]
- Linn T. C., Pelley J. W., Pettit F. H., Hucho F., Randall D. D., Reed L. J. -Keto acid dehydrogenase complexes. XV. Purification and properties of the component enzymes of the pyruvate dehydrogenase complexes from bovine kidney and heart. Arch Biochem Biophys. 1972 Feb;148(2):327–342. doi: 10.1016/0003-9861(72)90151-8. [DOI] [PubMed] [Google Scholar]
- Linn T. C., Pettit F. H., Reed L. J. Alpha-keto acid dehydrogenase complexes. X. Regulation of the activity of the pyruvate dehydrogenase complex from beef kidney mitochondria by phosphorylation and dephosphorylation. Proc Natl Acad Sci U S A. 1969 Jan;62(1):234–241. doi: 10.1073/pnas.62.1.234. [DOI] [PMC free article] [PubMed] [Google Scholar]
- Lundin A., Richardsson A., Thore A. Continous monitoring of ATP-converting reactions by purified firefly luciferase. Anal Biochem. 1976 Oct;75(2):611–620. doi: 10.1016/0003-2697(76)90116-0. [DOI] [PubMed] [Google Scholar]
- Nimmo H. G., Cohen P. Hormonal control of protein phosphorylation. Adv Cyclic Nucleotide Res. 1977;8:145–266. [PubMed] [Google Scholar]
- Odessey R., Goldberg A. L. Leucine degradation in cell-free extracts of skeletal muscle. Biochem J. 1979 Feb 15;178(2):475–489. doi: 10.1042/bj1780475. [DOI] [PMC free article] [PubMed] [Google Scholar]
- Odessey R., Khairallah E. A., Goldberg A. L. Origin and possible significance of alanine production by skeletal muscle. J Biol Chem. 1974 Dec 10;249(23):7623–7629. [PubMed] [Google Scholar]
- Parker P. J., Randle P. J. Inactivation of rat heart branched-chain 2-oxoacid dehydrogenase complex by adenosine triphosphate. FEBS Lett. 1978 Nov 1;95(1):153–156. doi: 10.1016/0014-5793(78)80072-6. [DOI] [PubMed] [Google Scholar]
- Parker P. J., Randle P. J. Partial purification and properties of branched-chain 2-oxo acid dehydrogenase of ox liver. Biochem J. 1978 Jun 1;171(3):751–757. doi: 10.1042/bj1710751. [DOI] [PMC free article] [PubMed] [Google Scholar]
- Paul H. S., Adibi S. A. Assessment of effect of starvation, glucose, fatty acids and hormones on alpha-decarboxylation of leucine in skeletal muscle of rat. J Nutr. 1976 Aug;106(8):1079–1088. doi: 10.1093/jn/106.8.1079. [DOI] [PubMed] [Google Scholar]
- Paul H. S., Adibi S. A. Leucine oxidation in diabetes and starvation: effects of ketone bodies on branched-chain amino acid oxidation in vitro. Metabolism. 1978 Feb;27(2):185–200. doi: 10.1016/0026-0495(78)90164-6. [DOI] [PubMed] [Google Scholar]
- Pettit F. H., Yeaman S. J., Reed L. J. Purification and characterization of branched chain alpha-keto acid dehydrogenase complex of bovine kidney. Proc Natl Acad Sci U S A. 1978 Oct;75(10):4881–4885. doi: 10.1073/pnas.75.10.4881. [DOI] [PMC free article] [PubMed] [Google Scholar]
- Rubin C. S., Rosen O. M. Protein phosphorylation. Annu Rev Biochem. 1975;44:831–887. doi: 10.1146/annurev.bi.44.070175.004151. [DOI] [PubMed] [Google Scholar]
- Schnaitman C., Greenawalt J. W. Enzymatic properties of the inner and outer membranes of rat liver mitochondria. J Cell Biol. 1968 Jul;38(1):158–175. doi: 10.1083/jcb.38.1.158. [DOI] [PMC free article] [PubMed] [Google Scholar]
- Severson D. L., Denton R. M., Pask H. T., Randle P. J. Calcium and magnesium ions as effectors of adipose-tissue pyruvate dehydrogenase phosphate phosphatase. Biochem J. 1974 May;140(2):225–237. doi: 10.1042/bj1400225. [DOI] [PMC free article] [PubMed] [Google Scholar]
- Shapiro B. M., Stadtman E. R. 5'-adenylyl-O-tyrosine. The novel phosphodiester residue of adenylylated glutamine synthetase from Escherichia coli. J Biol Chem. 1968 Jul 10;243(13):3769–3771. [PubMed] [Google Scholar]
- Shinnick F. L., Harper A. E. Branched-chain amino acid oxidation by isolated rat tissue preparations. Biochim Biophys Acta. 1976 Jul 21;437(2):477–486. doi: 10.1016/0304-4165(76)90016-7. [DOI] [PubMed] [Google Scholar]
- Sketcher R. D., Fern E. B., James W. P. The adaptation in muscle oxidation of leucine to dietary protein and energy intake. Br J Nutr. 1974 May;31(3):333–342. doi: 10.1079/bjn19740041. [DOI] [PubMed] [Google Scholar]
- Sullivan S. G., Dancis J., Cox R. P. Transient and long-term differential modulations of branched-chain alpha-keto acid decarboxylase activity in hypophysectomized rats. Biochim Biophys Acta. 1978 Mar 1;539(2):135–141. doi: 10.1016/0304-4165(78)90001-6. [DOI] [PubMed] [Google Scholar]
- Wieland O., Siess E. Interconversion of phospho- and dephospho- forms of pig heart pyruvate dehydrogenase. Proc Natl Acad Sci U S A. 1970 Apr;65(4):947–954. doi: 10.1073/pnas.65.4.947. [DOI] [PMC free article] [PubMed] [Google Scholar]
- Wohlhueter R. M., Harper A. E. Coinduction of rat liver branched chain alpha-keto acid dehydrogenase activities. J Biol Chem. 1970 May 10;245(9):2391–2401. [PubMed] [Google Scholar]