Abstract
Extracts of Chlorella pyrenoidosa, Euglena gracilis var. bacillaris, spinach, barley, Dictyostelium discoideum and Escherichia coli form an unknown compound enzymically from adenosine 5′-phosphosulphate in the presence of ammonia. This unknown compound shares the following properties with adenosine 5′-phosphoramidate: molar proportions of constituent parts (1 adenine:1 ribose:1 phosphate:1 ammonia released at low pH), co-electrophoresis in all buffers tested including borate, formation of AMP at low pH through release of ammonia, mass and i.r. spectra and conversion into 5′-AMP by phosphodiesterase. This unknown compound therefore appears to be identical with adenosine 5′-phosphoramidate. The enzyme that catalyses the formation of adenosine 5′-phosphoramidate from ammonia and adenosine 5′-phosphosulphate was purified 1800-fold (to homogeneity) from Chlorella by using (NH4)2SO4 precipitation and DEAE-cellulose, Sephadex and Reactive Blue 2–agarose chromatography. The purified enzyme shows one band of protein, coincident with activity, at a position corresponding to 60000–65000 molecular weight, on polyacrylamide-gel electrophoresis, and yields three subunits on sodium dodecyl sulphate/polyacrylamide-gel electrophoresis of 26000, 21000 and 17000 molecular weight, consistent with a molecular weight of 64000 for the native enzyme. Isoelectrofocusing yields one band of pI4.2. The pH optimum of the enzyme-catalysed reaction is 8.8. ATP, ADP or adenosine 3′-phosphate 5′-phosphosulphate will not replace adenosine 5′-phosphosulphate, and the apparent Km for the last-mentioned compound is 0.82mm. The apparent Km for ammonia (assuming NH3 to be the active species) is about 10mm. A large variety of primary, secondary and tertiary amines or amides will not replace ammonia. One mol.prop. of adenosine 5′-phosphosulphate reacts with 1 mol.prop. of ammonia to yield 1 mol.prop. each of adenosine 5′-phosphoramidate and sulphate; no AMP is found. The highly purified enzyme does not catalyse any of the known reactions of adenosine 5′-phosphosulphate, including those catalysed by ATP sulphurylase, adenosine 5′-phosphosulphate kinase, adenosine 5′-phosphosulphate sulphotransferase or ADP sulphurylase. Adenosine 5′-phosphoramidate is found in old samples of the ammonium salt of adenosine 5′-phosphosulphate and can be formed non-enzymically if adenosine 5′-phosphosulphate and ammonia are boiled. In the non-enzymic reaction both adenosine 5′-phosphoramidate and AMP are formed. Thus the enzyme forms adenosine 5′-phosphoramidate by selectively speeding up an already favoured reaction.
Full text
PDF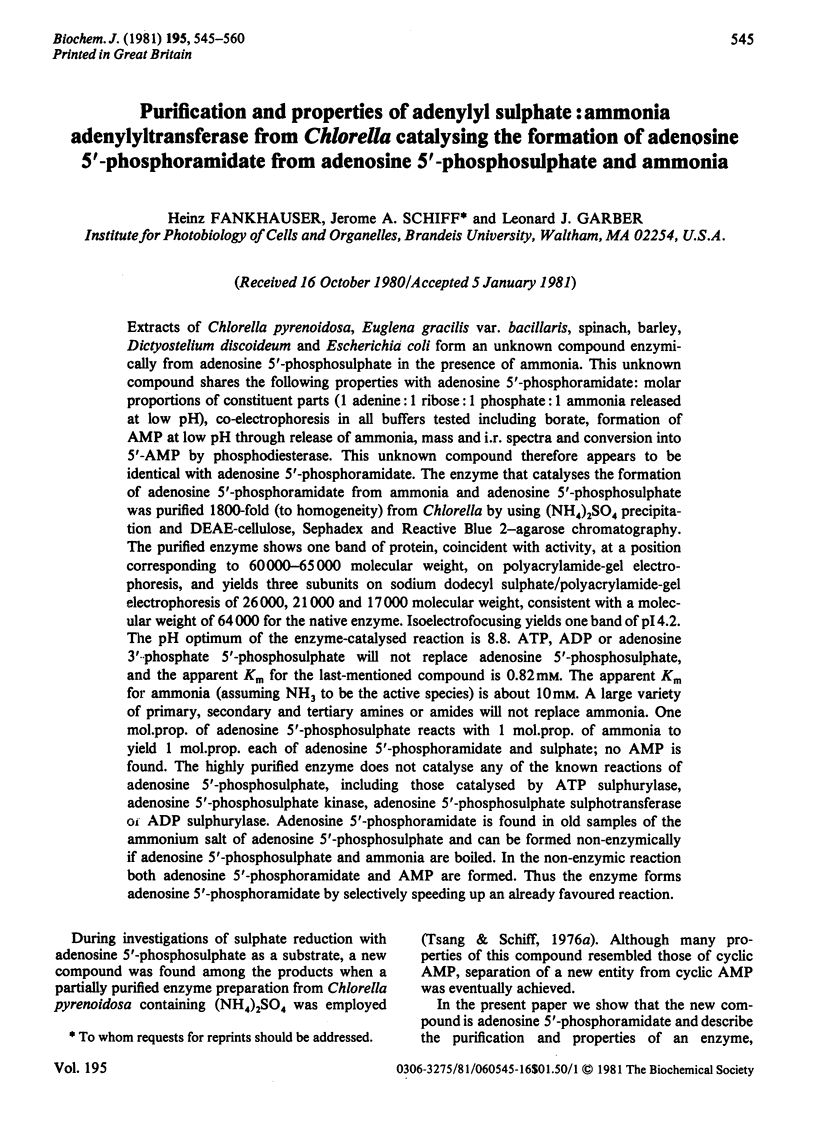
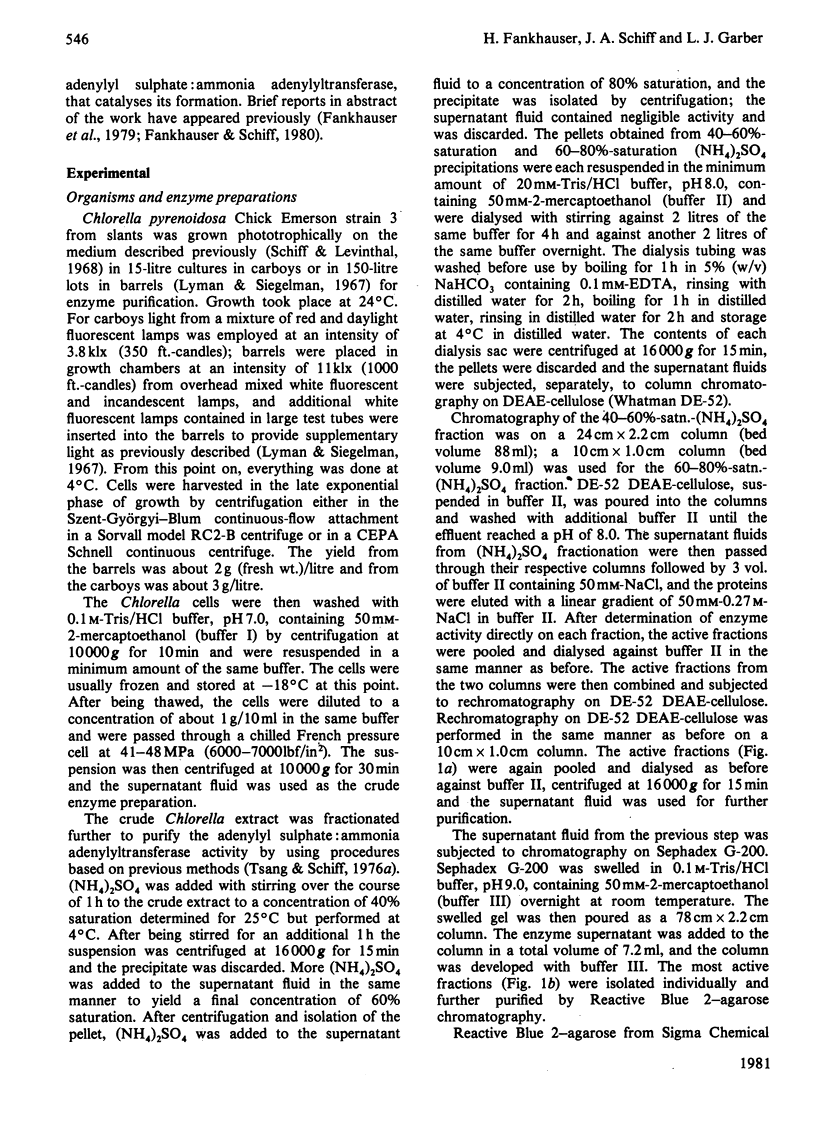
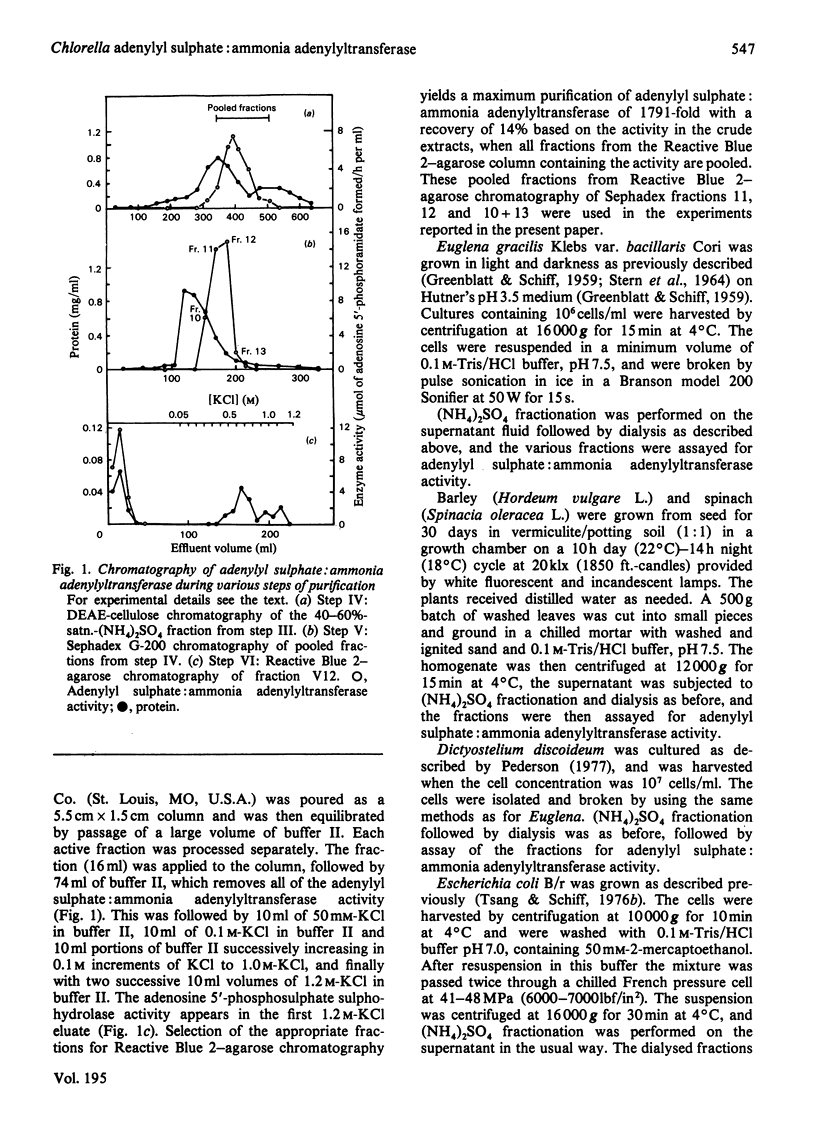
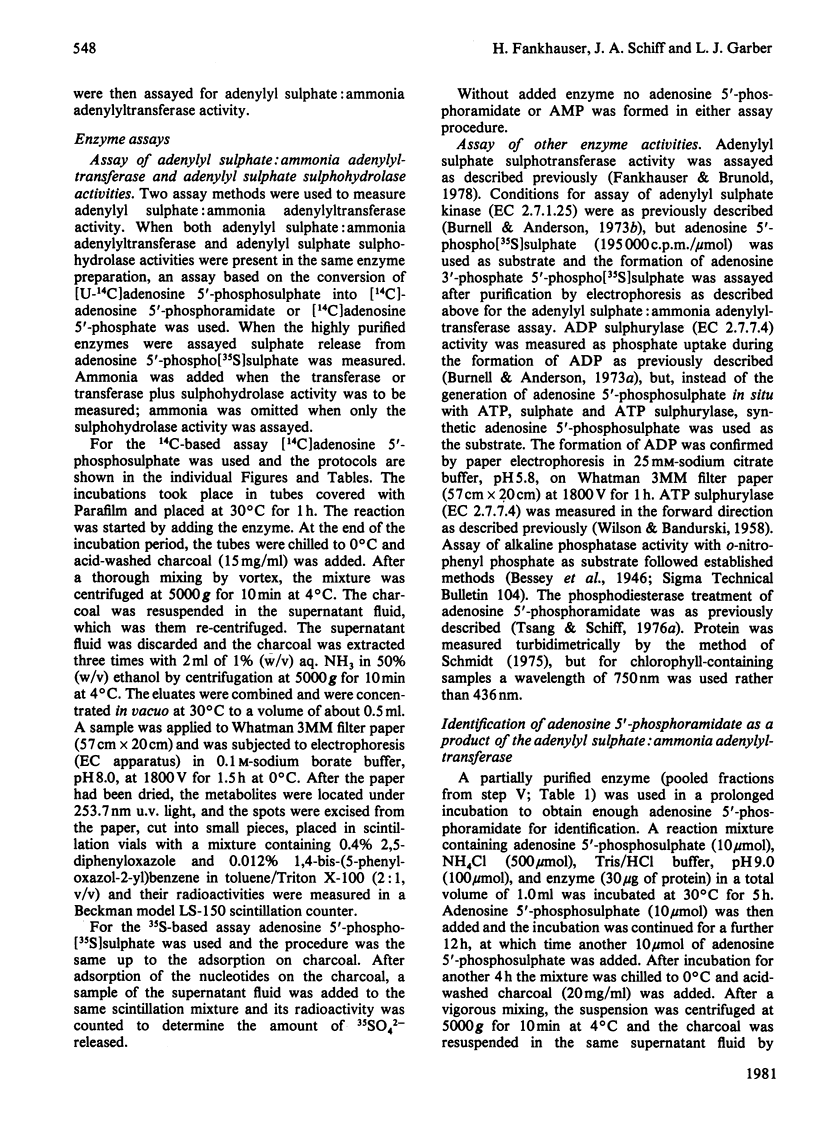
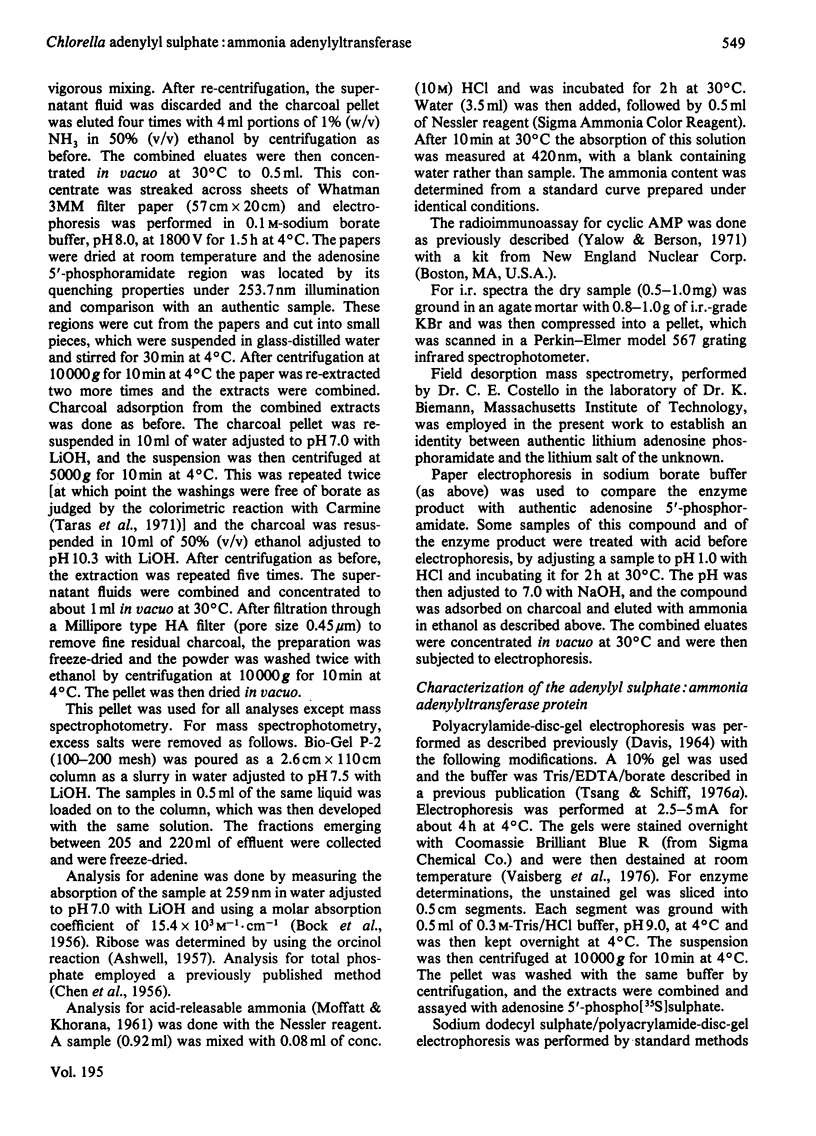
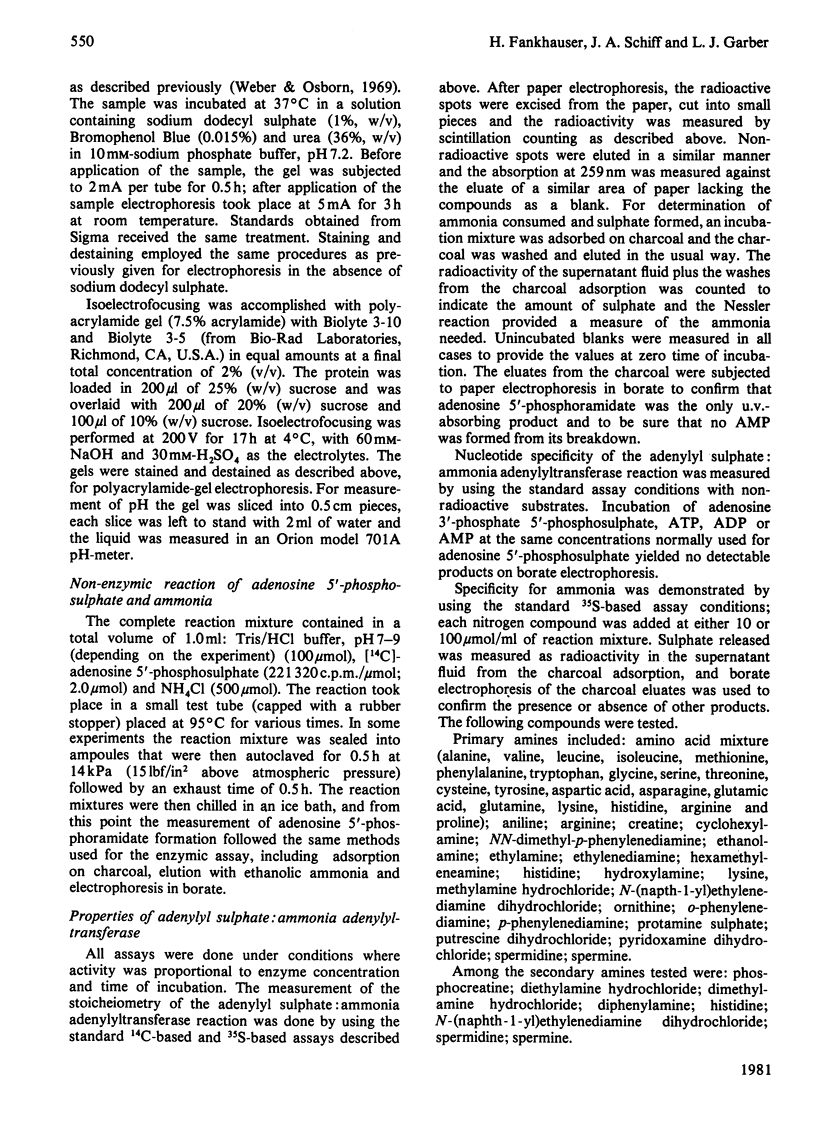
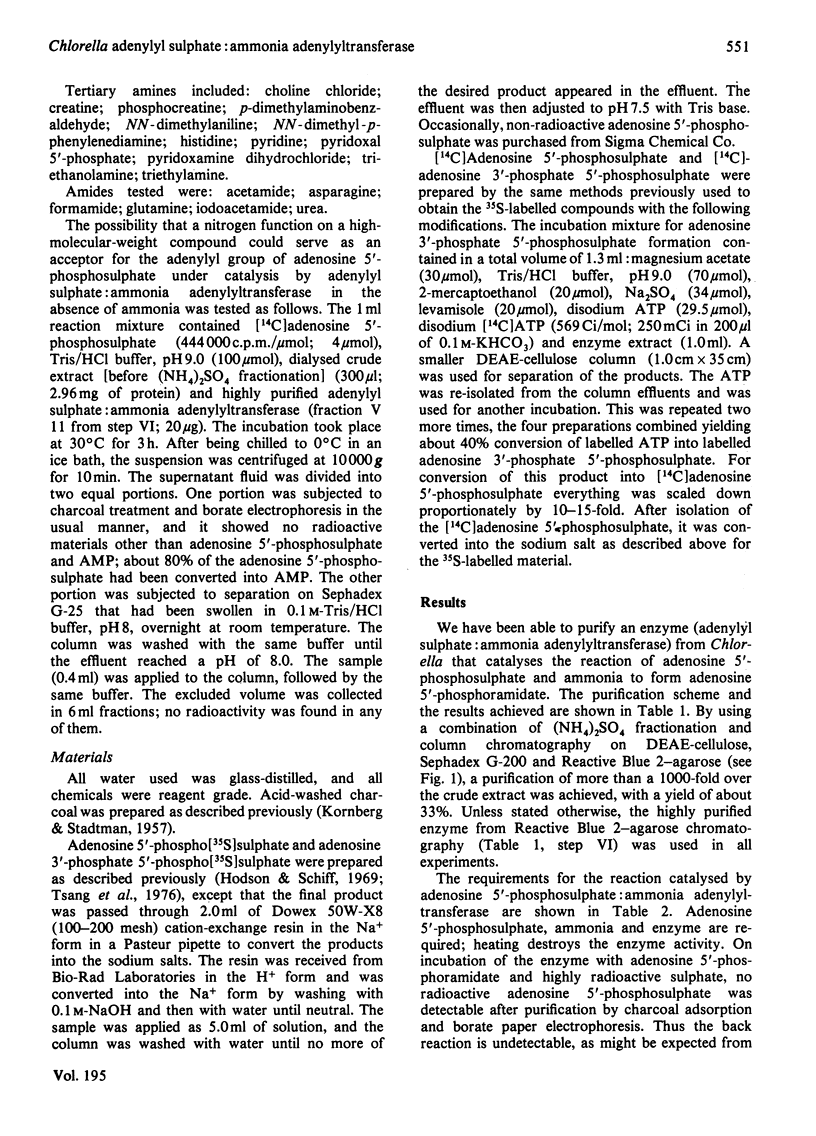
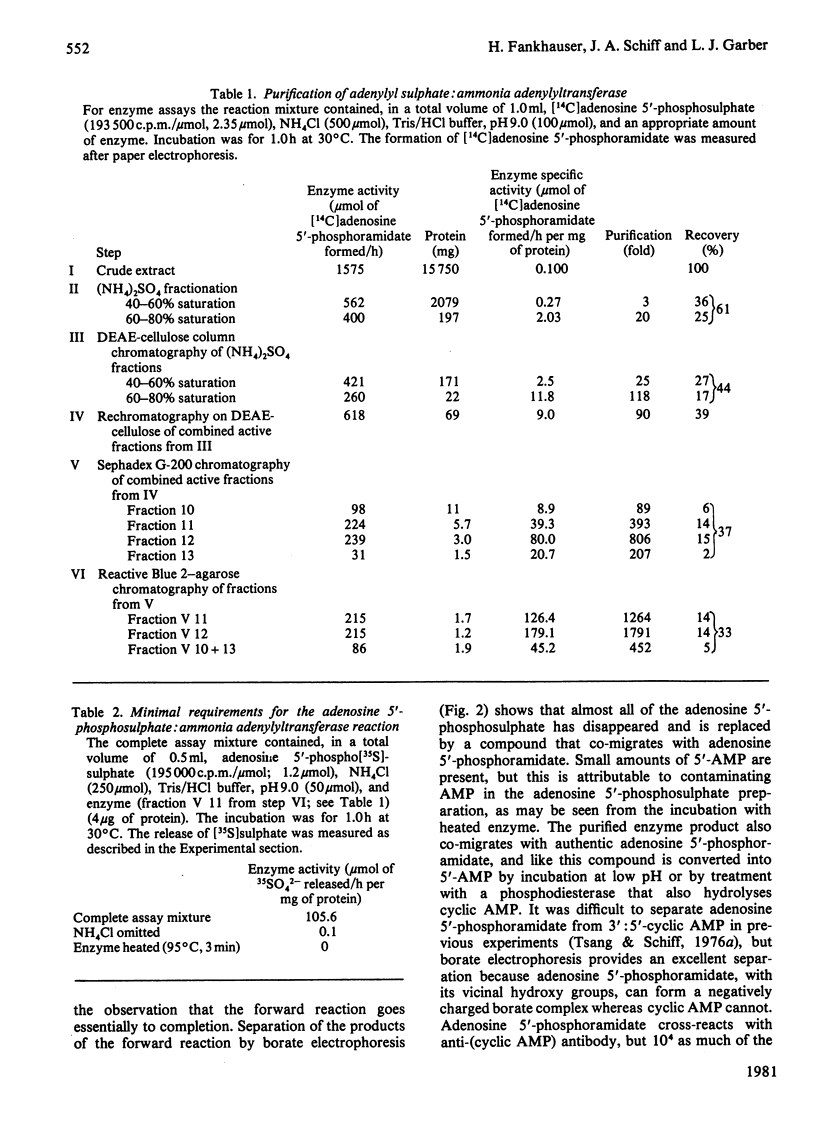
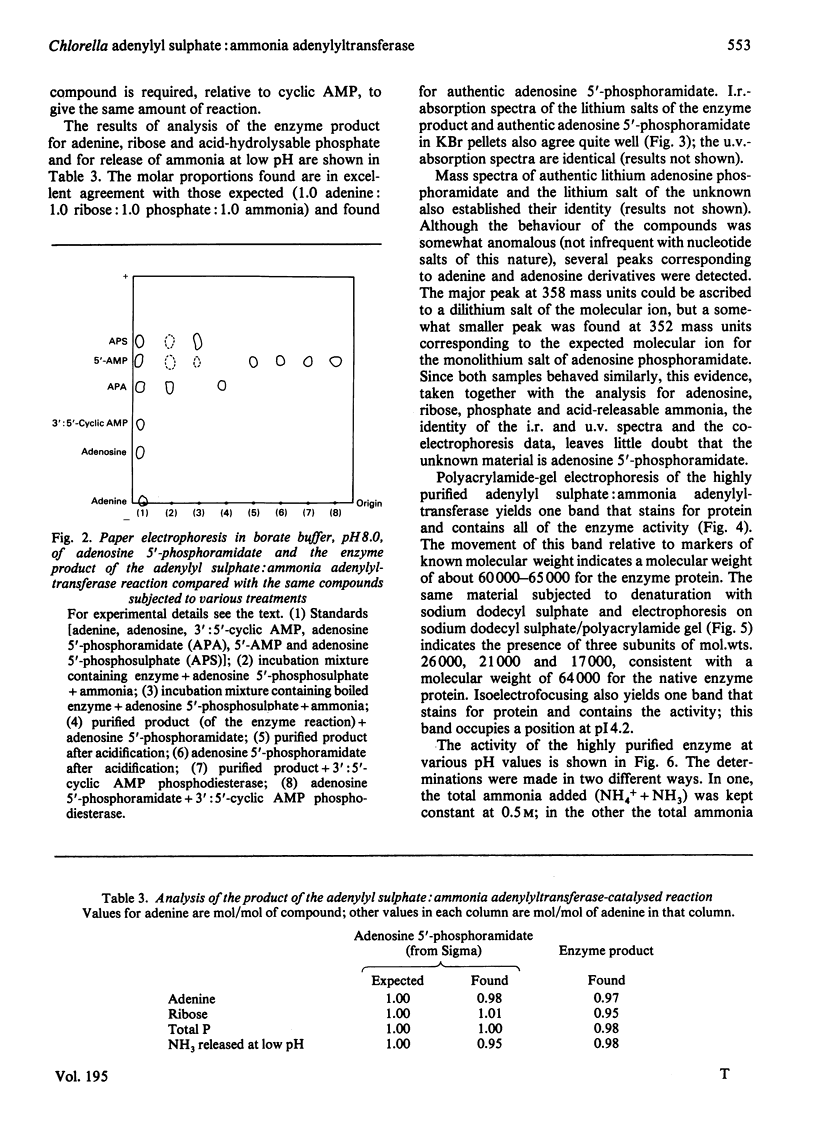
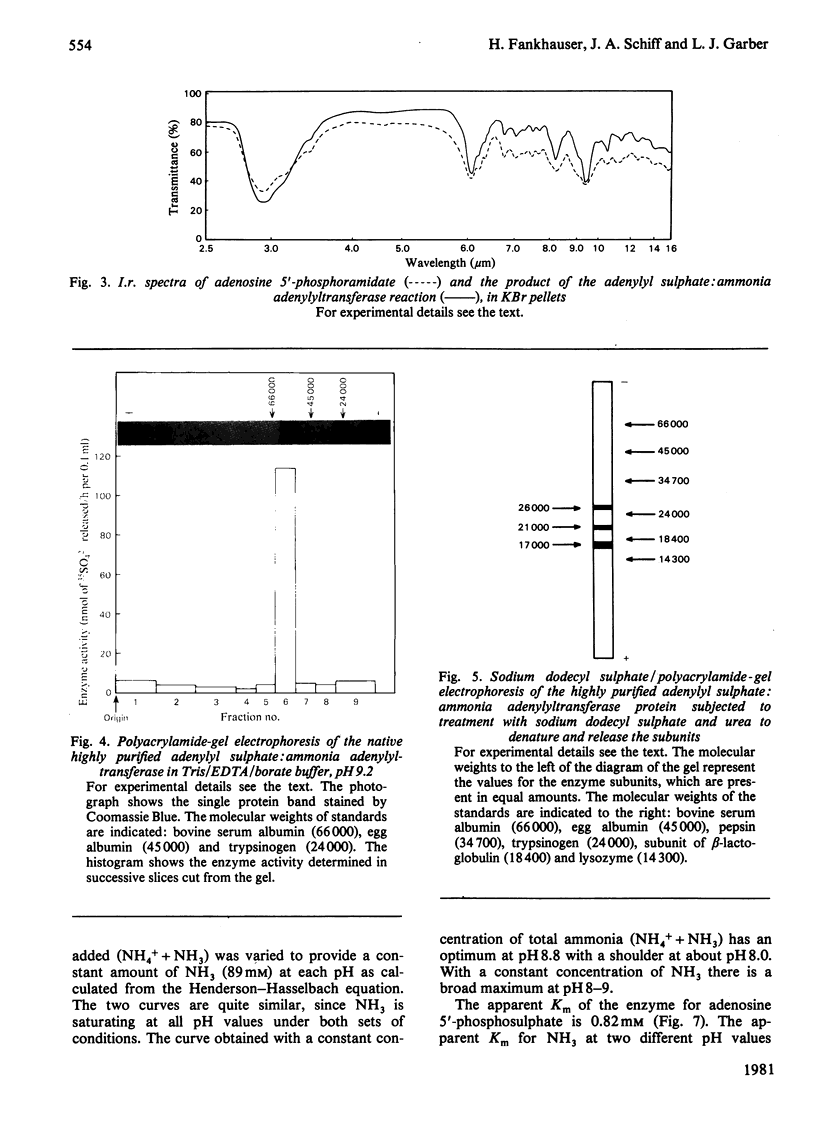
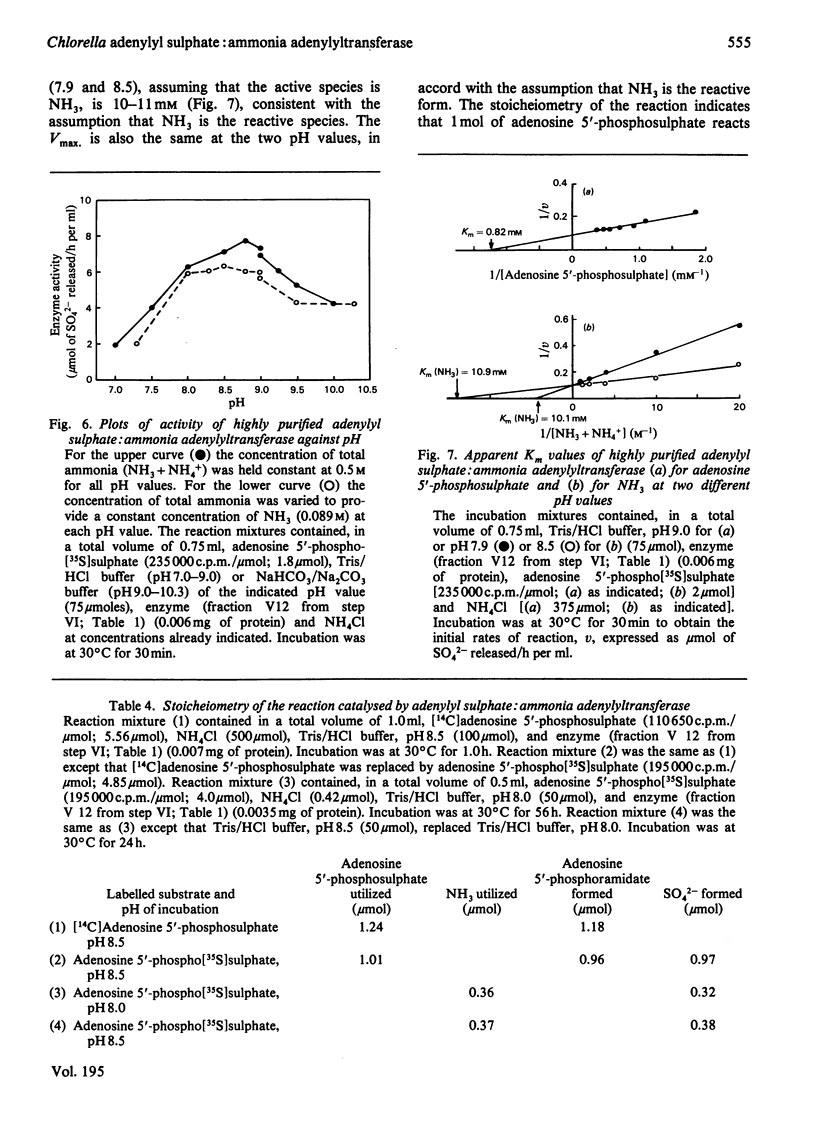
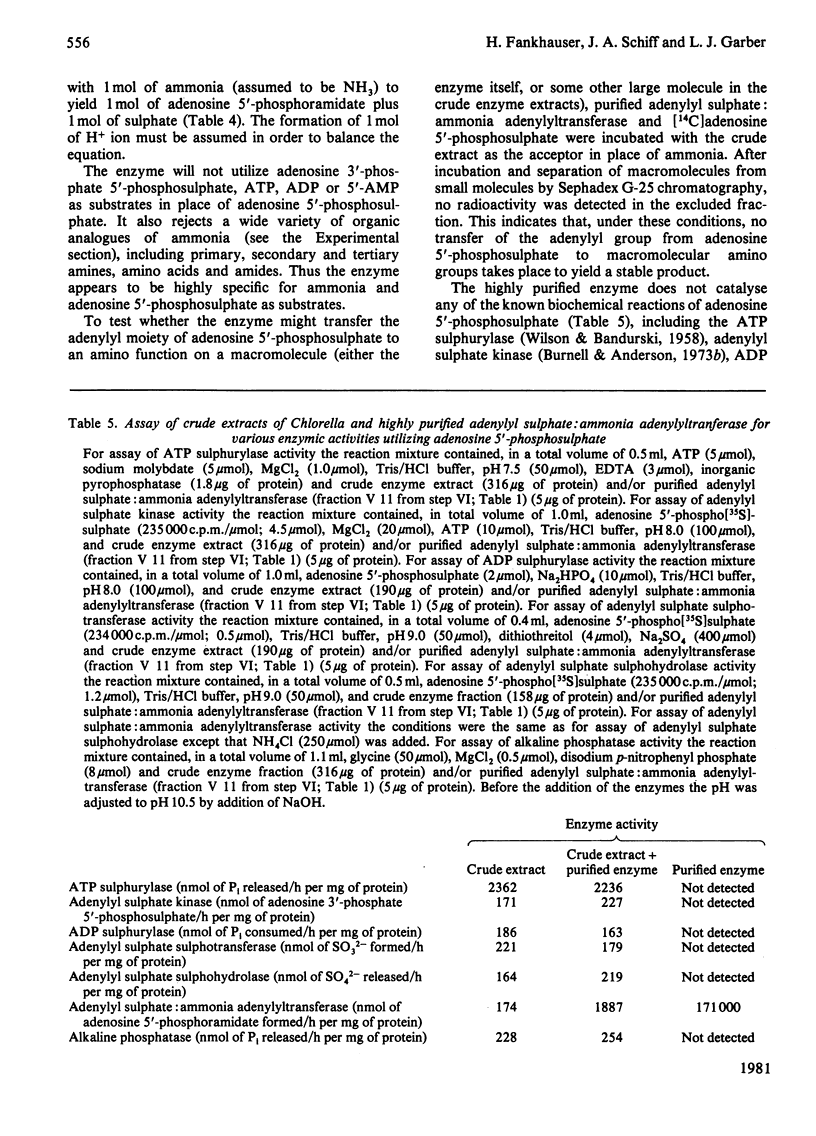
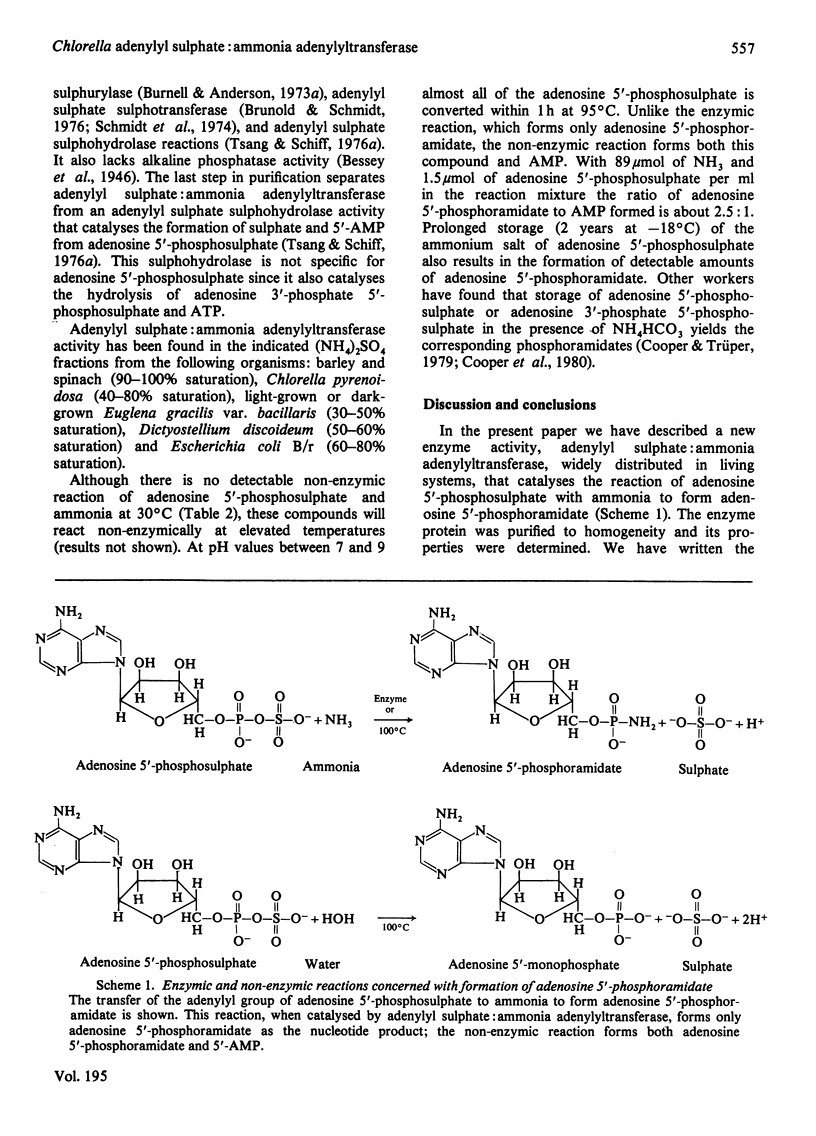
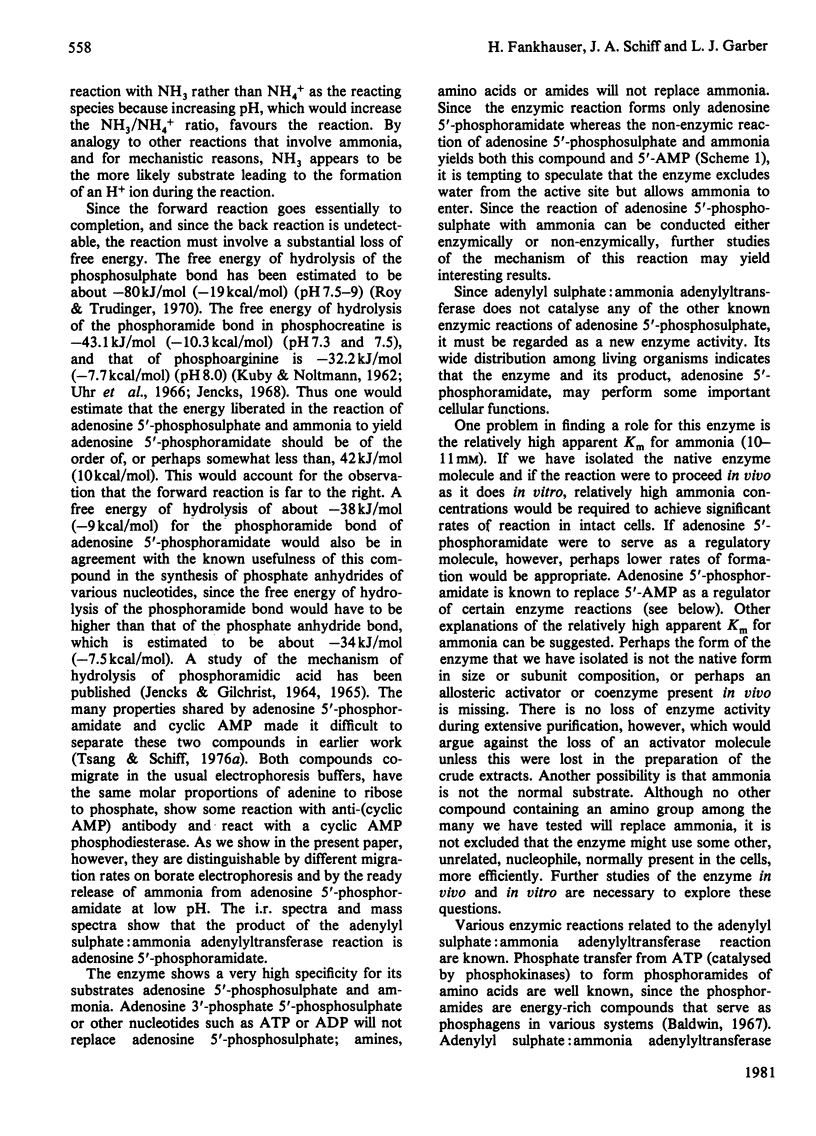
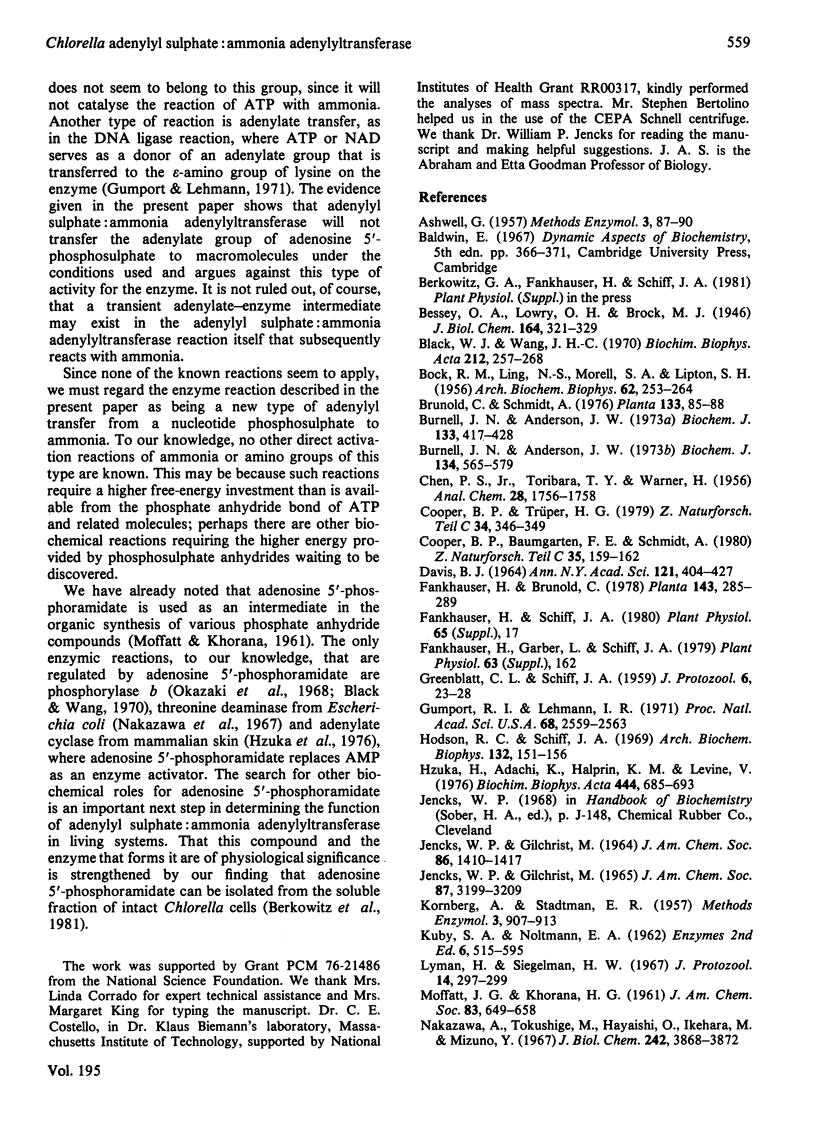
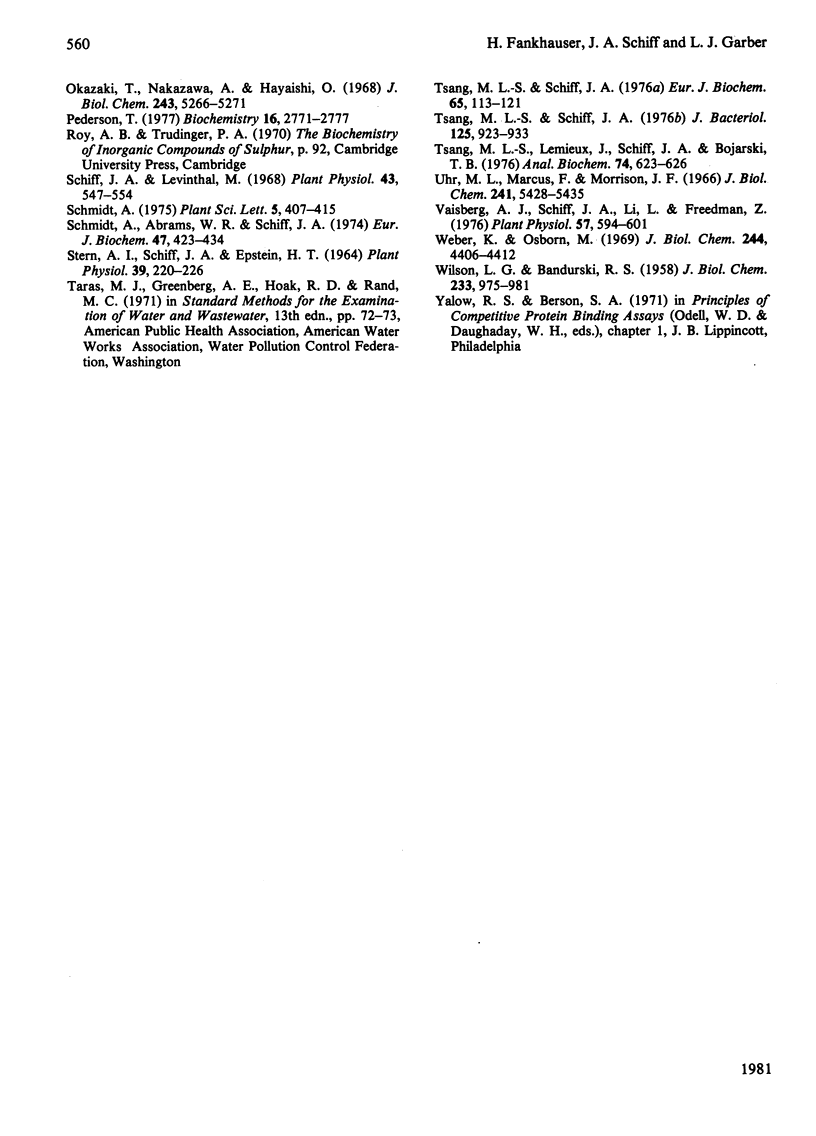
Images in this article
Selected References
These references are in PubMed. This may not be the complete list of references from this article.
- BOCK R. M., LING N. S., MORELL S. A., LIPTON S. H. Ultraviolet absorption spectra of adenosine-5'-triphosphate and related 5'-ribonucleotides. Arch Biochem Biophys. 1956 Jun;62(2):253–264. doi: 10.1016/0003-9861(56)90123-0. [DOI] [PubMed] [Google Scholar]
- Black W. J., Hsueh-Ching Wang J. Studies on the allosteric activation of glycogen phosphorylase b by nucleotides. II. Nucleotide structure in relation to mechanism of activation. Biochim Biophys Acta. 1970 Aug 15;212(2):257–268. doi: 10.1016/0005-2744(70)90206-8. [DOI] [PubMed] [Google Scholar]
- Burnell J. N., Anderson J. W. Adenosine 5'-sulphatophosphate kinase activity in spinach leaf tissue. Biochem J. 1973 Jun;134(2):565–579. doi: 10.1042/bj1340565. [DOI] [PMC free article] [PubMed] [Google Scholar]
- Burnell J. N., Anderson J. W. Adenosine diphosphate sulphurylase activity in leaf tissue. Biochem J. 1973 Jul;133(3):417–428. doi: 10.1042/bj1330417. [DOI] [PMC free article] [PubMed] [Google Scholar]
- Cooper B. P., Trüper H. G. Improved synthesis and rapid isolation millimole quantities of adenylysulfate. Z Naturforsch C. 1979 May-Jun;34C(5-6):346–349. doi: 10.1515/znc-1979-5-606. [DOI] [PubMed] [Google Scholar]
- DAVIS B. J. DISC ELECTROPHORESIS. II. METHOD AND APPLICATION TO HUMAN SERUM PROTEINS. Ann N Y Acad Sci. 1964 Dec 28;121:404–427. doi: 10.1111/j.1749-6632.1964.tb14213.x. [DOI] [PubMed] [Google Scholar]
- Duke S. O., Hoagland R. E., Elmore C. D. Effects of Glyphosate on Metabolism of Phenolic Compounds: V. l-alpha-AMINOOXY-beta-PHENYLPROPIONIC ACID AND GLYPHOSATE EFFECTS ON PHENYLALANINE AMMONIA-LYASE IN SOYBEAN SEEDLINGS. Plant Physiol. 1980 Jan;65(1):17–21. doi: 10.1104/pp.65.1.17. [DOI] [PMC free article] [PubMed] [Google Scholar]
- Gumport R. I., Lehman I. R. Structure of the DNA ligase-adenylate intermediate: lysine (epsilon-amino)-linked adenosine monophosphoramidate. Proc Natl Acad Sci U S A. 1971 Oct;68(10):2559–2563. doi: 10.1073/pnas.68.10.2559. [DOI] [PMC free article] [PubMed] [Google Scholar]
- Hodson R. C., Schiff J. A. Preparation of adenosine-3'-phosphate-51-phosphosulfate (PAPS): an improved enzymatic method using Chlorella pyrenoidosa. Arch Biochem Biophys. 1969 Jun;132(1):151–156. doi: 10.1016/0003-9861(69)90347-6. [DOI] [PubMed] [Google Scholar]
- Iizuka H., Adachi K., Halprin K. M., Levine V. Adenosine and adenine nucleotides stimulation of skin (epidermal) adenylate cyclase. Biochim Biophys Acta. 1976 Oct 22;444(3):685–693. doi: 10.1016/0304-4165(76)90315-9. [DOI] [PubMed] [Google Scholar]
- Lik-Shing Tsang M., Schiff J. A. Properties of enzyme fraction A from Chlorella and copurification of 3' (2'), 5'-biphosphonucleoside 3' (2')-phosphohydrolase, adenosine 5'phosphosulfate sulfohydrolase and adenosine-5'-phosphosulfate cyclase activities. Eur J Biochem. 1976 May 17;65(1):113–121. doi: 10.1111/j.1432-1033.1976.tb10395.x. [DOI] [PubMed] [Google Scholar]
- Lyman H., Siegelman H. W. Large-scale autotrophic culture of Euglena gracilis. J Protozool. 1967 May;14(2):297–299. doi: 10.1111/j.1550-7408.1967.tb02000.x. [DOI] [PubMed] [Google Scholar]
- Nakazawa A., Tokushige M., Hayaishi O., Ikehara M., Mizuno Y. Studies on the interaction between regulatory enzymes and effectors. I. Effect of adenosine 5'-monophosphate analogues on threonine deaminase. J Biol Chem. 1967 Sep 10;242(17):3868–3872. [PubMed] [Google Scholar]
- Okazaki T., Nakazawa A., Hayaishi O. Studies on the interaction between regulatory enzymes and effectors. II. Effect of adenosine 5'-monophosphate analogues on glycogen phosphorylase B. J Biol Chem. 1968 Oct 25;243(20):5266–5271. [PubMed] [Google Scholar]
- Pederson T. Isolation and characterization of chromatin from the cellular slime mold, Dictyostelium discoideum. Biochemistry. 1977 Jun 14;16(12):2771–2777. doi: 10.1021/bi00631a029. [DOI] [PubMed] [Google Scholar]
- Schiff J. A., Levinthal M. Studies of sulfate utilization by algae. 4. Properties of a cell-free sulfate-reducing system from chlorella. Plant Physiol. 1968 Apr;43(4):547–554. doi: 10.1104/pp.43.4.547. [DOI] [PMC free article] [PubMed] [Google Scholar]
- Schmidt A., Abrams W. R., Schiff J. A. Reduction of adenosine 5'-phosphosulfate to cysteine in extracts from Chlorella and mutants blocked for sulfate reduction. Eur J Biochem. 1974 Sep 16;47(3):423–434. doi: 10.1111/j.1432-1033.1974.tb03709.x. [DOI] [PubMed] [Google Scholar]
- Stern A. I., Schiff J. A., Epstein H. T. Studies of Chloroplast Development in Euglena. V. Pigment Biosynthesis, Photosynthetic Oxygen Evolution and Carbon Dioxide Fixation during Chloroplast Development. Plant Physiol. 1964 Mar;39(2):220–226. doi: 10.1104/pp.39.2.220. [DOI] [PMC free article] [PubMed] [Google Scholar]
- Tsang M. L., Lemieux J., Schiff J. A., Bojarski T. B. Preparation of adenosine 5'-phosphosulfate (APS) from adenosine 3'-phosphate 5'-phosphosulfate (PAPS) prepared by an improved procedure. Anal Biochem. 1976 Aug;74(2):623–626. doi: 10.1016/0003-2697(76)90249-9. [DOI] [PubMed] [Google Scholar]
- Tsang M. L., Schiff J. A. Sulfate-reducing pathway in Escherichia coli involving bound intermediates. J Bacteriol. 1976 Mar;125(3):923–933. doi: 10.1128/jb.125.3.923-933.1976. [DOI] [PMC free article] [PubMed] [Google Scholar]
- Uhr M. L., Marcus F., Morrison J. F. Studies on adenosine triphosphate: arginine phosphotransferase. Purification and reaction mechanism. J Biol Chem. 1966 Nov 25;241(22):5428–5435. [PubMed] [Google Scholar]
- Vaisberg A. J., Schiff J. A., Li L., Freedman Z. Events Surrounding the Early Development of Euglena Chloroplasts: VII. Photocontrol of the Source Of Reducing Power for Chloramphenicol Reduction by the Ferredoxin-NADP Reductase System. Plant Physiol. 1976 Apr;57(4):594–601. doi: 10.1104/pp.57.4.594. [DOI] [PMC free article] [PubMed] [Google Scholar]
- WILSON L. G., BANDURSKI R. S. Enzymatic reactions involving sulfate, sulfite, selenate, and molybdate. J Biol Chem. 1958 Oct;233(4):975–981. [PubMed] [Google Scholar]
- Weber K., Osborn M. The reliability of molecular weight determinations by dodecyl sulfate-polyacrylamide gel electrophoresis. J Biol Chem. 1969 Aug 25;244(16):4406–4412. [PubMed] [Google Scholar]