Abstract
1. The apparent Michaelis constants of the glutamate dehydrogenase (EC 1.4.1.3), the glutamate-oxaloacetate transaminase (EC 2.6.1.1) and the glutaminase (EC 3.5.1.2) of rat brain mitochondria derived from non-synaptic (M) and synaptic (SM2) sources were studied. 2. The kinetics of oxygen uptake of both populations of mitochondria in the presence of a fixed concentration of malate and various concentrations of glutamate or glutamine were investigated. 3. In both mitochondrial populations, glutamate-supported respiration in the presence of 2.5 mM-malate appears to be biphasic, one system (B) having an apparent Km for glutamate of 0.25 +/- 0.04 mM (n=7) and the other (A) of 1.64 +/- 0.5 mM (n=7) [when corrected for low-Km process, Km=2.4 +/- 0.75 mM (n=7)]. Aspartate production in these experiments followed kinetics of a single process with an apparent Km for glutamate of 1.8-2 mM, approximating to the high-Km process. 4. Oxygen-uptake measurement with both mitochondrial populations in the presence of malate and various glutamate concentrations in which amino-oxyacetate was present showed kinetics approximating only to the low-Km process (apparent Km for glutamate approximately 0.2 mM). Similar experiments in the presence of glutamate alone showed kinetics approximating only to the high-Km process (apparent Km for glutamate approximately 1-1.3 mM). 5. Oxygen uptake supported by glutamine (0-3 mM) and malate (2.5 mM) by the free (M) mitochondrial population, however, showed single-phase kinetics with an apparent Km for glutamine of 0.28 mM. 6. Aspartate and 2-oxoglutarate accumulation was measured in 'free' nonsynaptic (M) brain mitochondria oxidizing various concentrations of glutamate at a fixed malate concentration. Over a 30-fold increase in glutamate concentration, the flux through the glutamate-oxaloacetate transaminase increased 7--8-fold, whereas the flux through 2-oxoglutarate dehydrogenase increased about 2.5-fold. 7. The biphasic kinetics of glutamate-supported respiration by brain mitochondria in the presence of malate are interpreted as reflecting this change in the relative fluxes through transamination and 2-oxoglutarate metabolism.
Full text
PDF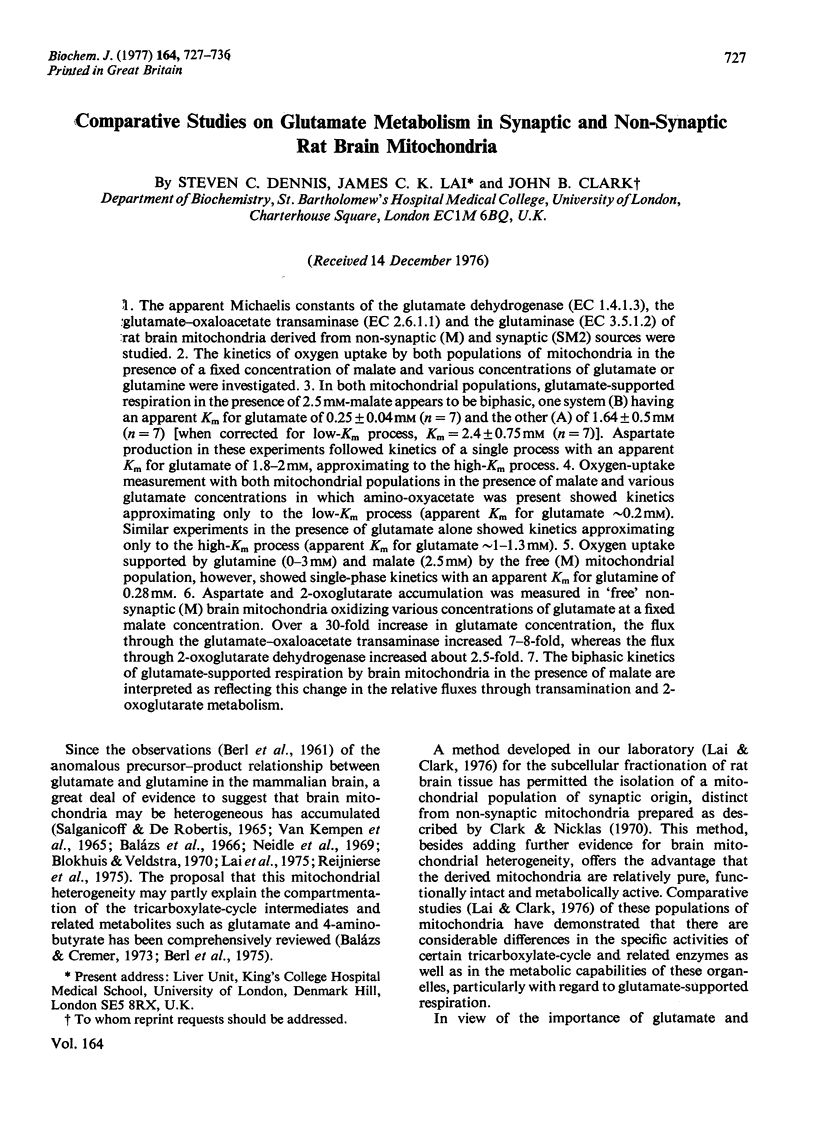
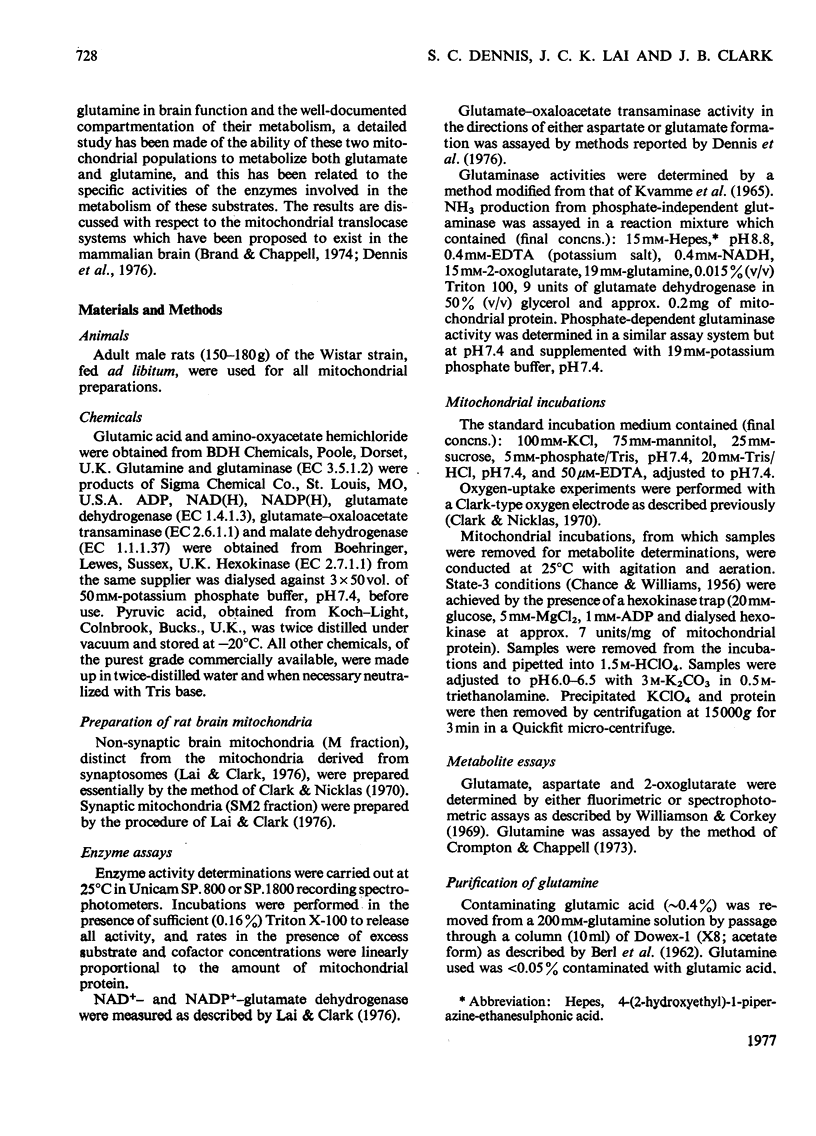
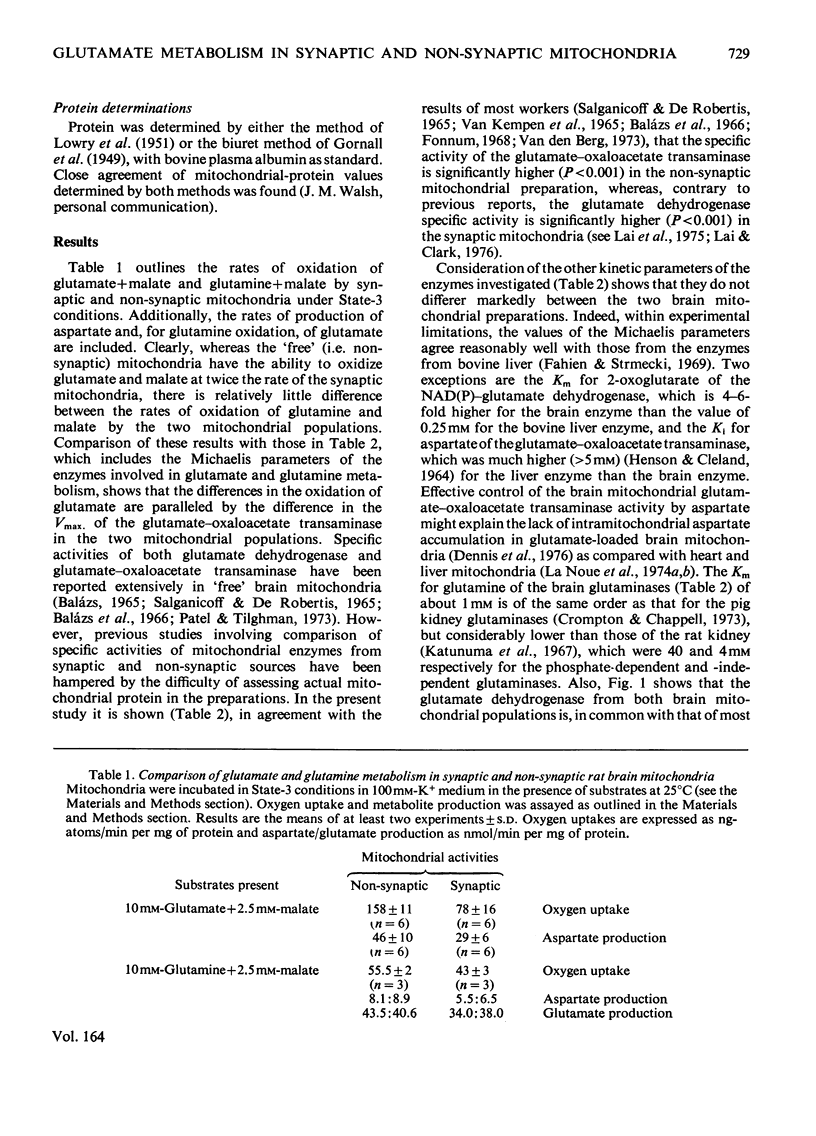
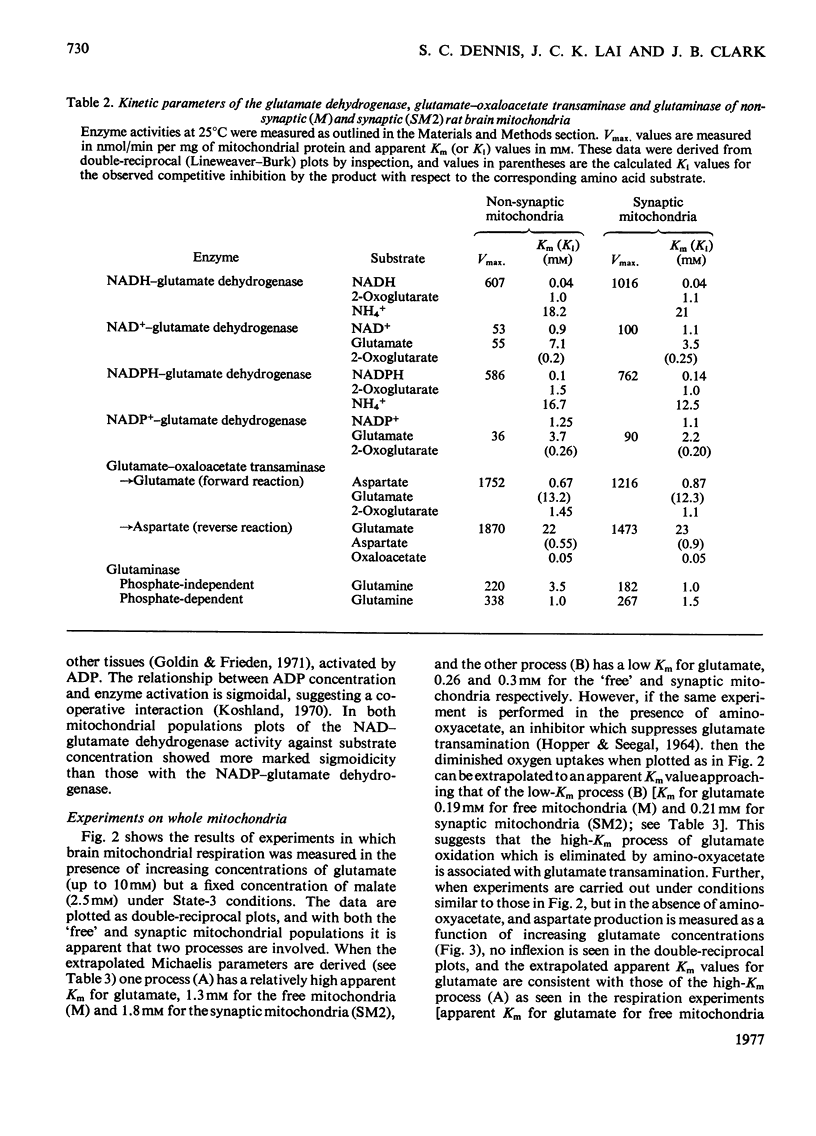
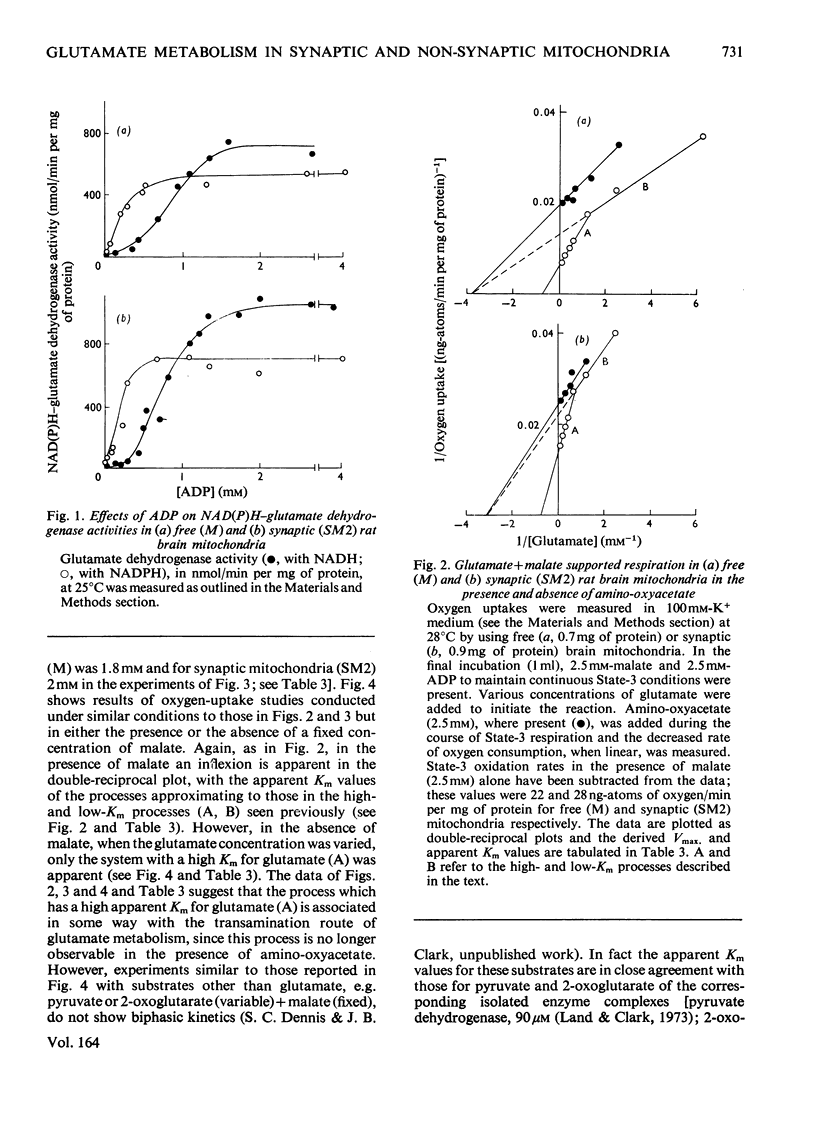
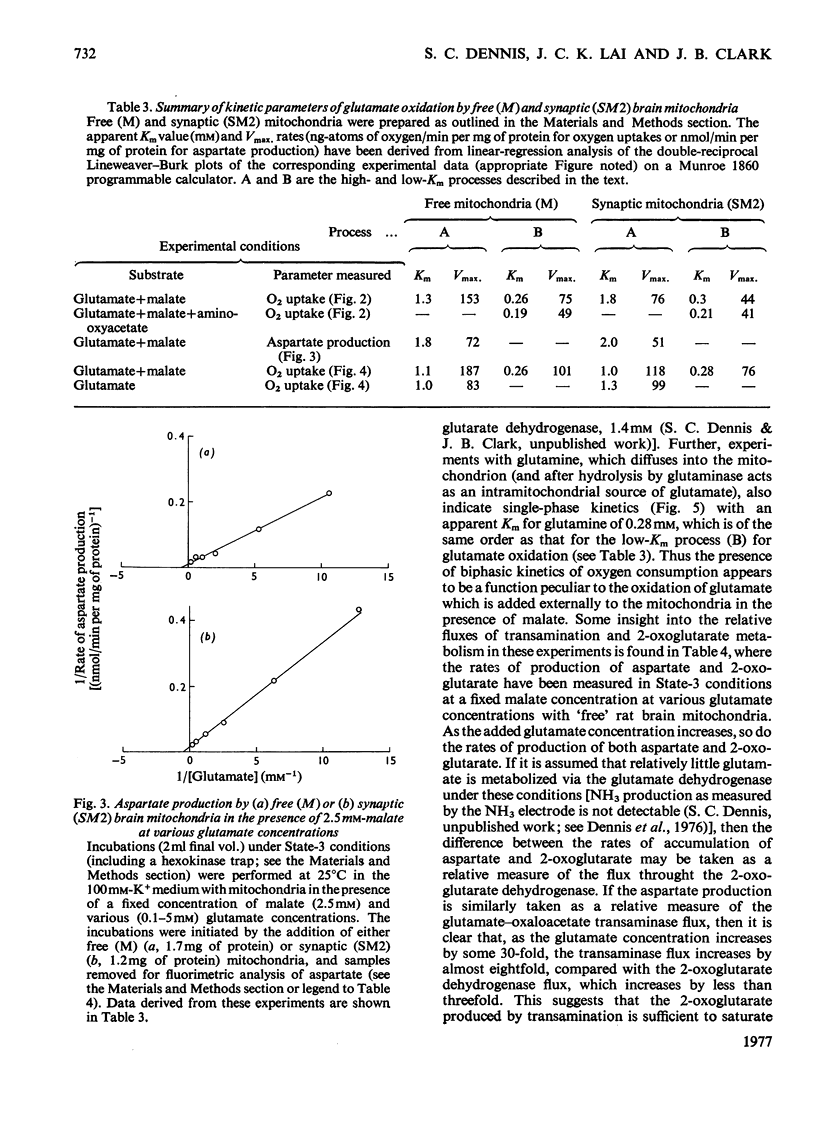
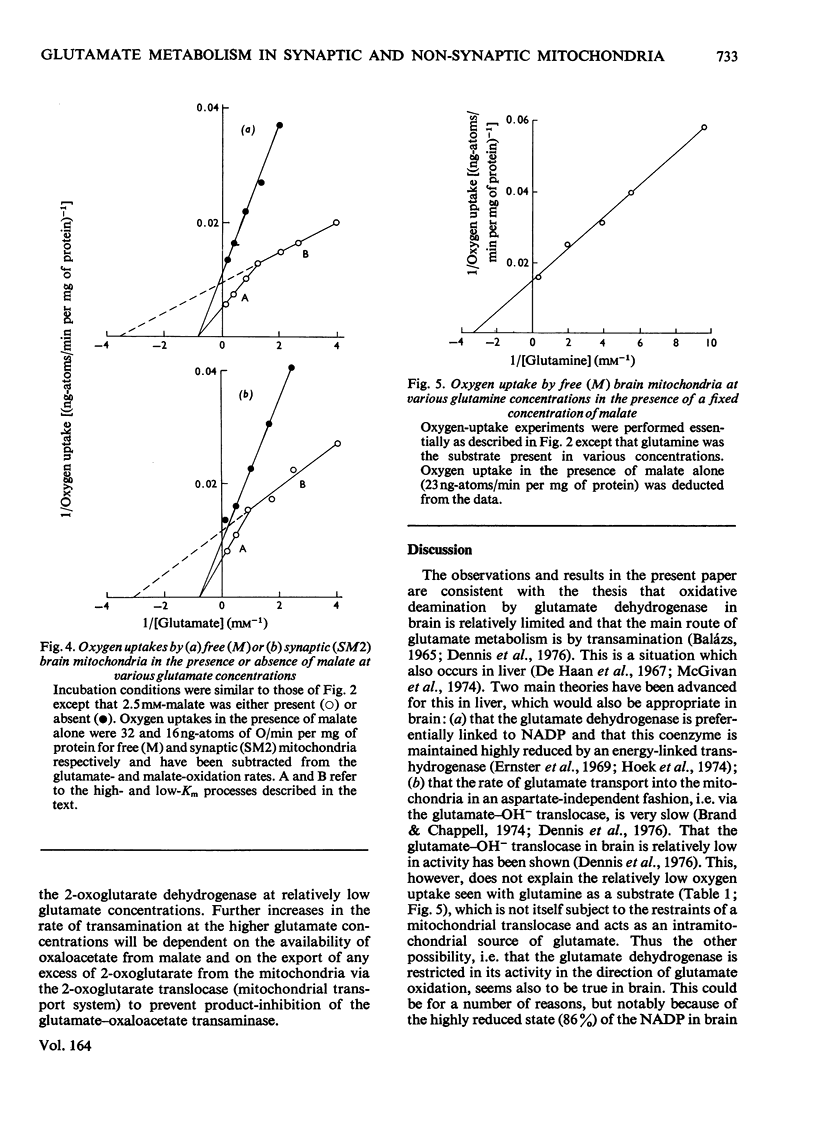
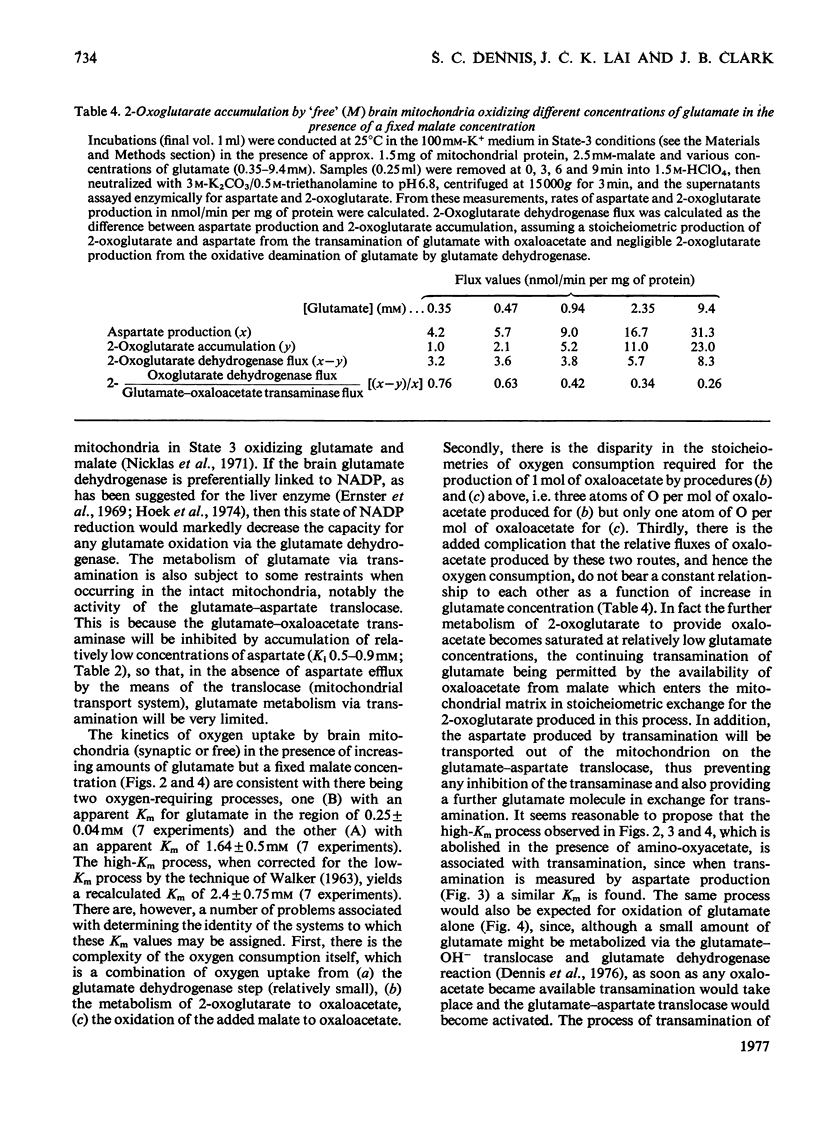
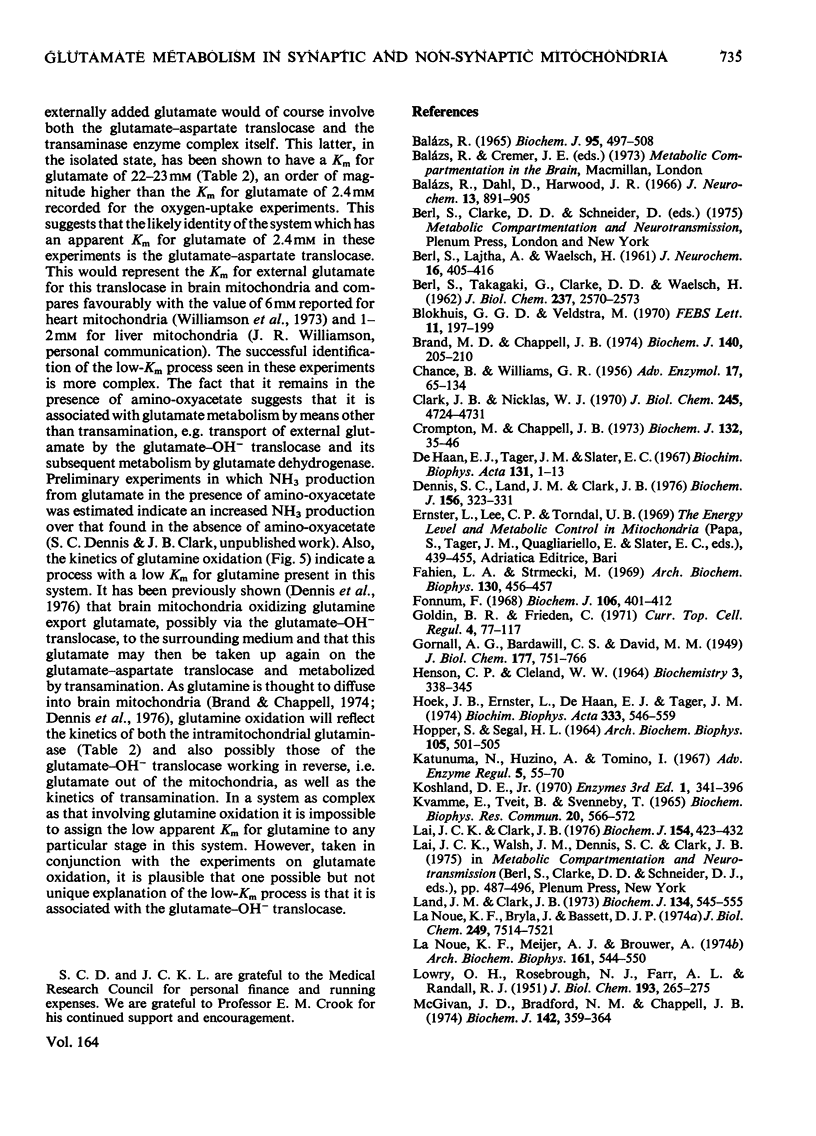
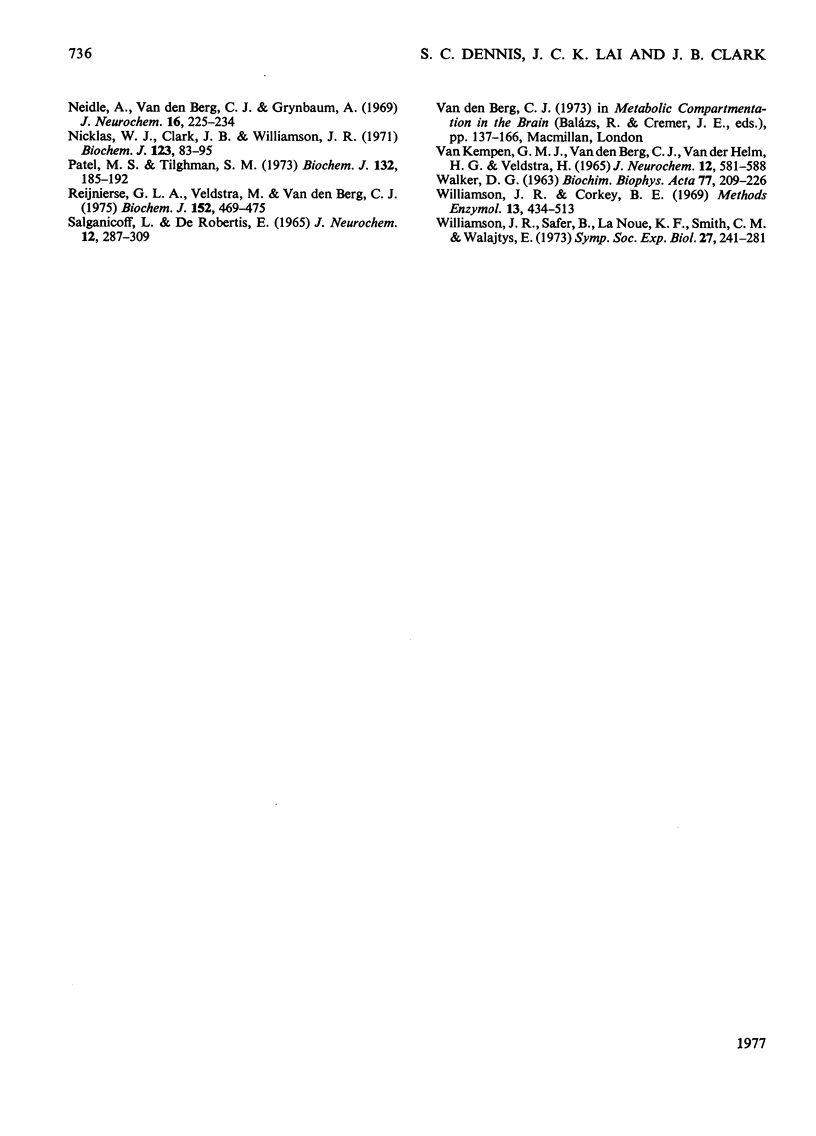
Selected References
These references are in PubMed. This may not be the complete list of references from this article.
- BALAZS R. CONTROL OF GLUTAMATE OXIDATION IN BRAIN AND LIVER MITOCHONDRIAL SYSTEMS. Biochem J. 1965 May;95:497–508. doi: 10.1042/bj0950497. [DOI] [PMC free article] [PubMed] [Google Scholar]
- BERL S., TAKAGAKI G., CLARKE D. D., WAELSCH H. Carbon dioxide fixation in the brain. J Biol Chem. 1962 Aug;237:2570–2573. [PubMed] [Google Scholar]
- Balázs R., Dahl D., Harwood J. R. Subcellular distribution of enzymes of glutamate metabolism in rat brain. J Neurochem. 1966 Oct;13(10):897–905. doi: 10.1111/j.1471-4159.1966.tb10285.x. [DOI] [PubMed] [Google Scholar]
- Berl S., Frigyesi T. L. Comparison of cerebral regional metabolism of [14C]leucine following third ventricle and intravenous administration in the cat. J Neurochem. 1969 Mar;16(3):405–415. doi: 10.1111/j.1471-4159.1969.tb10381.x. [DOI] [PubMed] [Google Scholar]
- Blokhuis G. G.D., Veldstra H. Heterogeneity of mitochondria in rat brain. FEBS Lett. 1970 Dec;11(3):197–199. doi: 10.1016/0014-5793(70)80527-0. [DOI] [PubMed] [Google Scholar]
- Brand M. D., Chappell J. B. Glutamate and aspartate transport in rat brain mitochondria. Biochem J. 1974 May;140(2):205–210. doi: 10.1042/bj1400205. [DOI] [PMC free article] [PubMed] [Google Scholar]
- CHANCE B., WILLIAMS G. R. The respiratory chain and oxidative phosphorylation. Adv Enzymol Relat Subj Biochem. 1956;17:65–134. doi: 10.1002/9780470122624.ch2. [DOI] [PubMed] [Google Scholar]
- Clark J. B., Nicklas W. J. The metabolism of rat brain mitochondria. Preparation and characterization. J Biol Chem. 1970 Sep 25;245(18):4724–4731. [PubMed] [Google Scholar]
- Crompton M., Chappell J. B. Transport of glutamine and glutamate in kidney mitochondria in relation to glutamine deamidation. Biochem J. 1973 Jan;132(1):35–46. doi: 10.1042/bj1320035. [DOI] [PMC free article] [PubMed] [Google Scholar]
- Dennis S. C., Land J. M., Clark J. B. Glutamate metabolism and transport in rat brain mitochondria. Biochem J. 1976 May 15;156(2):323–331. doi: 10.1042/bj1560323. [DOI] [PMC free article] [PubMed] [Google Scholar]
- Fahien L. A., Strmecki M. Studies on gluconeogenic mitochondrial enzymes. II. The conversion of glutamate to alpha-ketoglutarate by bovine liver mitochondrial glutamate dehydrogenase and glutamate-oxaloacetate transaminase. Arch Biochem Biophys. 1969 Mar;130(1):456–467. doi: 10.1016/0003-9861(69)90058-7. [DOI] [PubMed] [Google Scholar]
- Fonnum F. The distribution of glutamate decarboxylase and aspartate transaminase in subcellular fractions of rat and guinea-pig brain. Biochem J. 1968 Jan;106(2):401–412. doi: 10.1042/bj1060401. [DOI] [PMC free article] [PubMed] [Google Scholar]
- HENSON C. P., CLELAND W. W. KINETIC STUDIES OF GLUTAMIC OXALOACETIC TRANSAMINASE ISOZYMES. Biochemistry. 1964 Mar;3:338–345. doi: 10.1021/bi00891a007. [DOI] [PubMed] [Google Scholar]
- HOPPER S., SEGAL H. L. COMPARATIVE PROPERTIES OF GLUTAMIC-ALANINE TRANSAMINASE FROM SEVERAL SOURCES. Arch Biochem Biophys. 1964 Jun;105:501–505. doi: 10.1016/0003-9861(64)90042-6. [DOI] [PubMed] [Google Scholar]
- Hoek J. B., Ernster L., de Haan E. J., Tager J. M. The nicotinamide nucleotide specificity of glutamate dehydrogenase in intact rat-liver mitochondria. Biochim Biophys Acta. 1974 Mar 26;333(3):546–559. doi: 10.1016/0005-2728(74)90138-8. [DOI] [PubMed] [Google Scholar]
- Katunuma N., Huzino A., Tomino I. Organ specific control of glutamine metabolism. Adv Enzyme Regul. 1967;5:55–69. doi: 10.1016/0065-2571(67)90008-8. [DOI] [PubMed] [Google Scholar]
- Kvamme E., Tveit B., Svenneby G. Glutaminase from pig kidney, an allosteric protein. Biochem Biophys Res Commun. 1965 Sep 8;20(5):566–572. doi: 10.1016/0006-291x(65)90436-5. [DOI] [PubMed] [Google Scholar]
- LOWRY O. H., ROSEBROUGH N. J., FARR A. L., RANDALL R. J. Protein measurement with the Folin phenol reagent. J Biol Chem. 1951 Nov;193(1):265–275. [PubMed] [Google Scholar]
- LaNoue K. F., Bryla J., Bassett D. J. Energy-driven aspartate efflux from heart and liver mitochondria. J Biol Chem. 1974 Dec 10;249(23):7514–7521. [PubMed] [Google Scholar]
- LaNoue K. F., Meijer A. J., Brouwer A. Evidence for electrogenic aspartate transport in rat liver mitochondria. Arch Biochem Biophys. 1974 Apr 2;161(2):544–550. doi: 10.1016/0003-9861(74)90337-3. [DOI] [PubMed] [Google Scholar]
- Lai J. C., Clark J. B. Preparation and properties of mitochondria derived from synaptosomes. Biochem J. 1976 Feb 15;154(2):423–432. doi: 10.1042/bj1540423. [DOI] [PMC free article] [PubMed] [Google Scholar]
- Land J. M., Clark J. B. Effect of phenylpyruvate on enzymes involved in fatty acid synthesis in rat brain. Biochem J. 1973 Jun;134(2):545–555. doi: 10.1042/bj1340545. [DOI] [PMC free article] [PubMed] [Google Scholar]
- McGivan J. D., Bradford N. M., Chappell J. B. Adaptive changes in the capacity of systems used for the synthesis of citrulline in rat liver mitochondria in response to high- and-low-protein diets. Biochem J. 1974 Aug;142(2):359–364. doi: 10.1042/bj1420359. [DOI] [PMC free article] [PubMed] [Google Scholar]
- Neidle A., van den Berg C. J., Grynbaum A. The heterogeneity of rat brain mitochondria isolated on continuous sucrose gradients. J Neurochem. 1969 Feb;16(2):225–234. doi: 10.1111/j.1471-4159.1969.tb05940.x. [DOI] [PubMed] [Google Scholar]
- Nicklas W. J., Clark J. B., Williamson J. R. Metabolism of rat brain mitochondria. Studies on the potassium ion-stimulated oxidation of pyruvate. Biochem J. 1971 Jun;123(1):83–95. doi: 10.1042/bj1230083. [DOI] [PMC free article] [PubMed] [Google Scholar]
- Patel M. S., Tilghman S. M. Regulation of pyruvate metabolism via pyruvate carboxylase in rat brain mitochondria. Biochem J. 1973 Feb;132(2):185–192. doi: 10.1042/bj1320185. [DOI] [PMC free article] [PubMed] [Google Scholar]
- Reijnierse G. L., Veldstra H., Van den Berg C. J. Subcellular localization of gamma-aminobutyrate transaminase and glutamate dehydrogenase in adult rat brain. Evidence for at least two small glutamate compartments in brain. Biochem J. 1975 Dec;152(3):469–475. doi: 10.1042/bj1520469. [DOI] [PMC free article] [PubMed] [Google Scholar]
- SALGANICOFF L., DEROBERTIS E. SUBCELLULAR DISTRIBUTION OF THE ENZYMES OF THE GLUTAMIC ACID, GLUTAMINE AND GAMMA-AMINOBUTYRIC ACID CYCLES IN RAT BRAIN. J Neurochem. 1965 Apr;12:287–309. doi: 10.1111/j.1471-4159.1965.tb06766.x. [DOI] [PubMed] [Google Scholar]
- WALKER D. G. ON THE PRESENCE OF TWO SOLUBLE GLUCOSE-PHOSPHORYLATING ENZYMES IN ADULT LIVER AND THE DEVELOPMENT OF ONE OF THESE AFTER BIRTH. Biochim Biophys Acta. 1963 Oct 1;77:209–226. doi: 10.1016/0006-3002(63)90494-3. [DOI] [PubMed] [Google Scholar]
- Williamson J. R., Safer B., LaNoue K. F., Smith C. M., Walajtys E. Mitochondrial-cytosolic interactions in cardiac tissue: role of the malate-aspartate cycle in the removal of glycolytic NADH from the cytosol. Symp Soc Exp Biol. 1973;27:241–281. [PubMed] [Google Scholar]
- van Kempen G. M., van den Berg C. J., van der Helm H. J., Veldstra H. Intracellular localization of glutamate decarboxylase, gamma-aminobutyrate transaminase and some other enzymes in brain tissue. J Neurochem. 1965 Jul;12(7):581–588. doi: 10.1111/j.1471-4159.1965.tb04250.x. [DOI] [PubMed] [Google Scholar]