Abstract
1. The chemical changes during contractile activity were separated from recovery metabolism in the forearm flexor musculature in normal human subjects using 31P nuclear magnetic resonance (NMR) spectroscopy. Percutaneous, supramaximal twitch stimulation of the median and ulnar nerves was used in combination with temporary ischaemia of the forearm to characterize the summed ATPase activity. The recovery following restoration of blood flow provided a measure of oxidative ATP synthesis activity. These processes were measured based on the dynamics of creatine phosphate (PCr) content. 2. Muscle oxygen stores were depleted using ischaemia without stimulation as indicated by PCr breakdown after 250 +/- 33 s (mean +/- S.D.; n = 5), which provided a measure of the basal metabolic rate (0.008 +/- 0.002 mM s-1, n = 5). 3. The PCr breakdown rate during twitch stimulation of the oxygen-depleted muscle was constant at 1 Hz at 0.15 +/- 0.03 mM PCr per second or per twitch (n = 8). A constant cost per twitch was found from 0.5 to 2 Hz stimulation (depletion of PCr per twitch = 0.15 mM per twitch). 4. No net anaerobic recovery of PCr was found during a 2 min post-stimulation ischaemia. 5. Upon restoration of blood flow, PCr recovery followed an exponential time course with a time constant of 63 +/- 14 s (n = 8). From these recovery rates, the capacity for oxidative phosphorylation was estimated to be 0.4 mM s-1. 6. This experimental approach defines a non-invasive and quantitative measure of human muscle ATPase rate and ATP synthetase rate.
Full text
PDF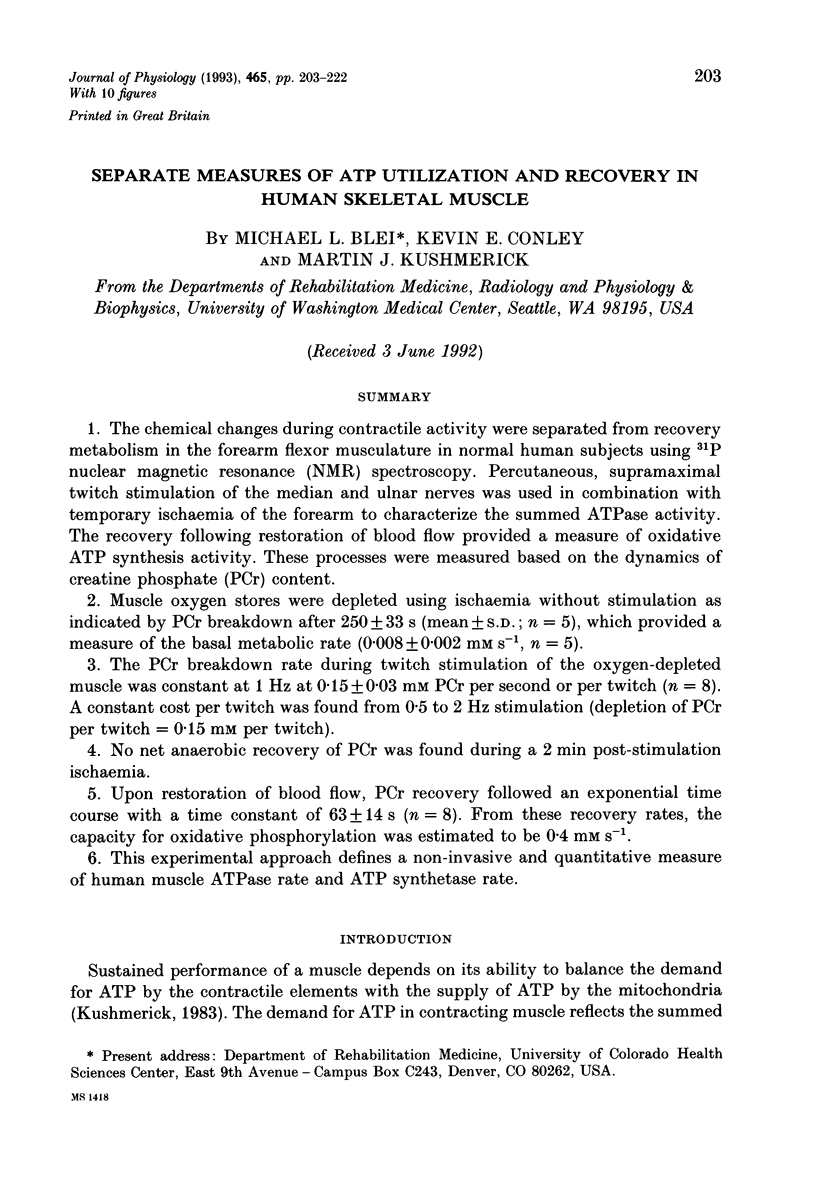
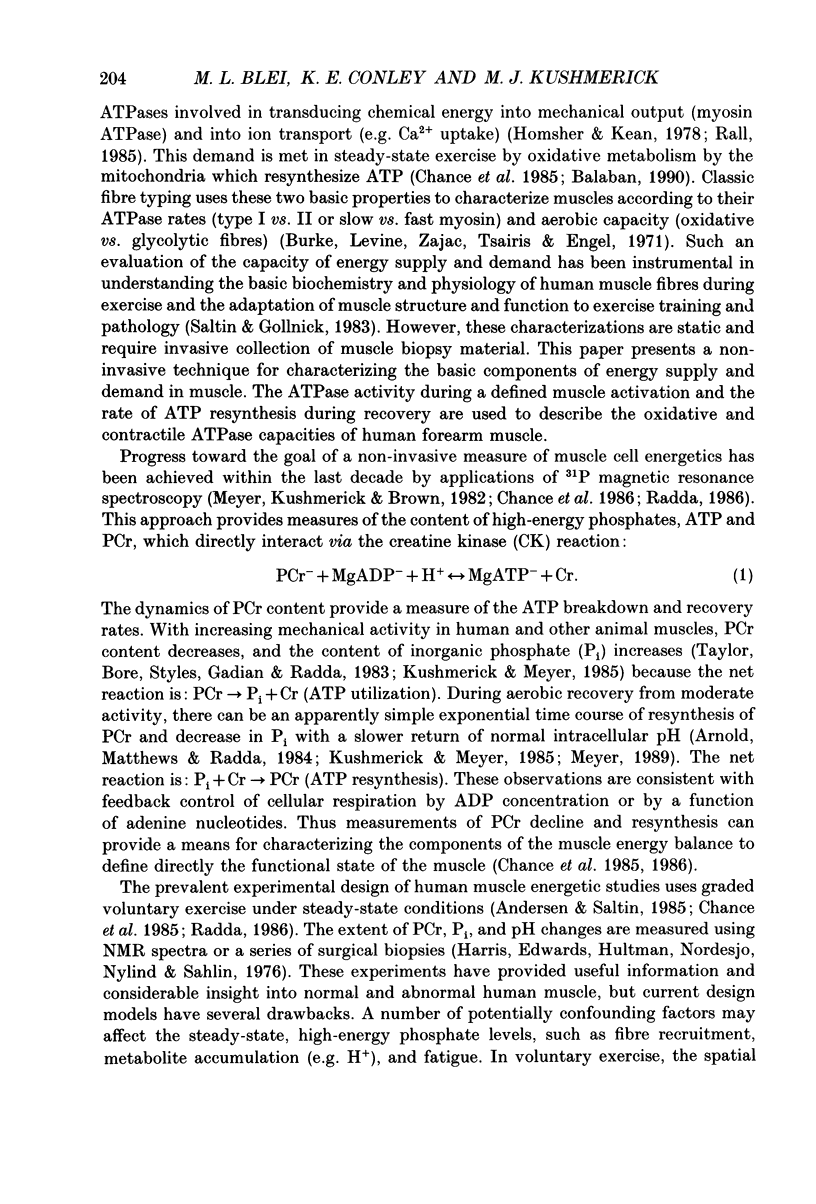
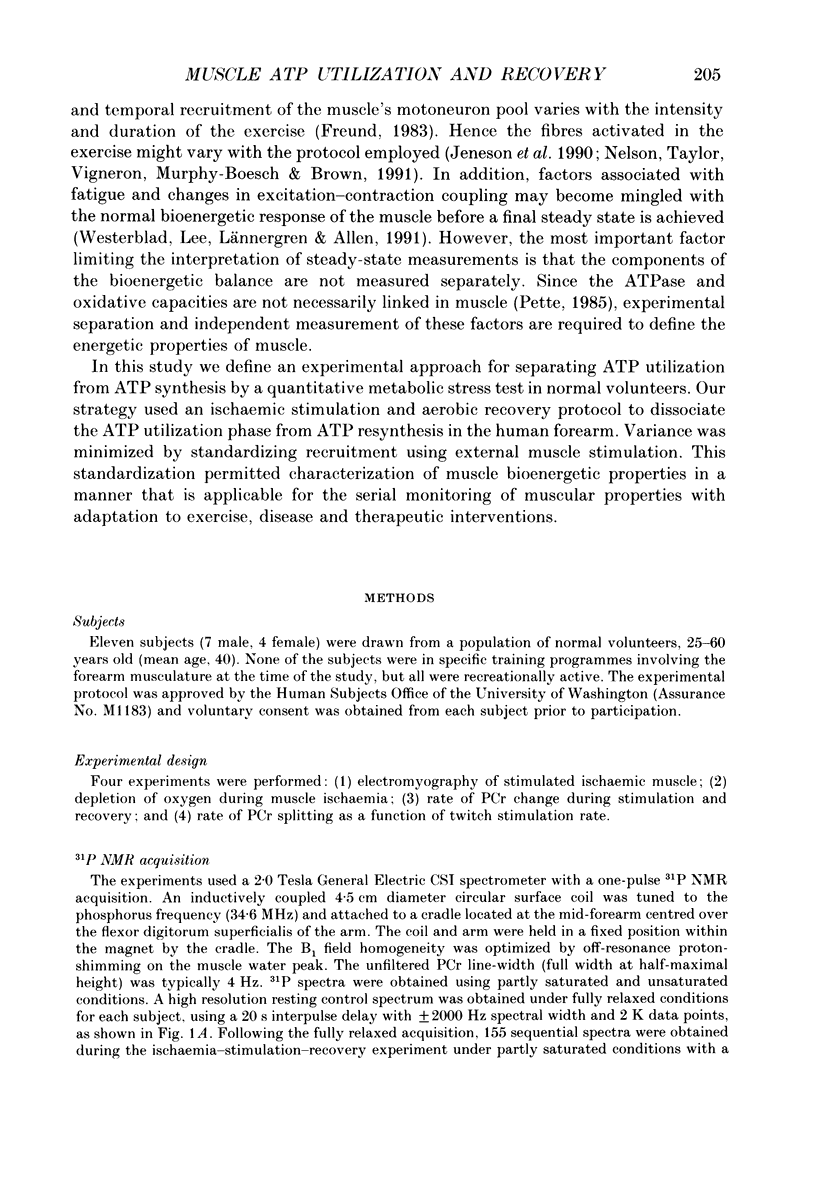
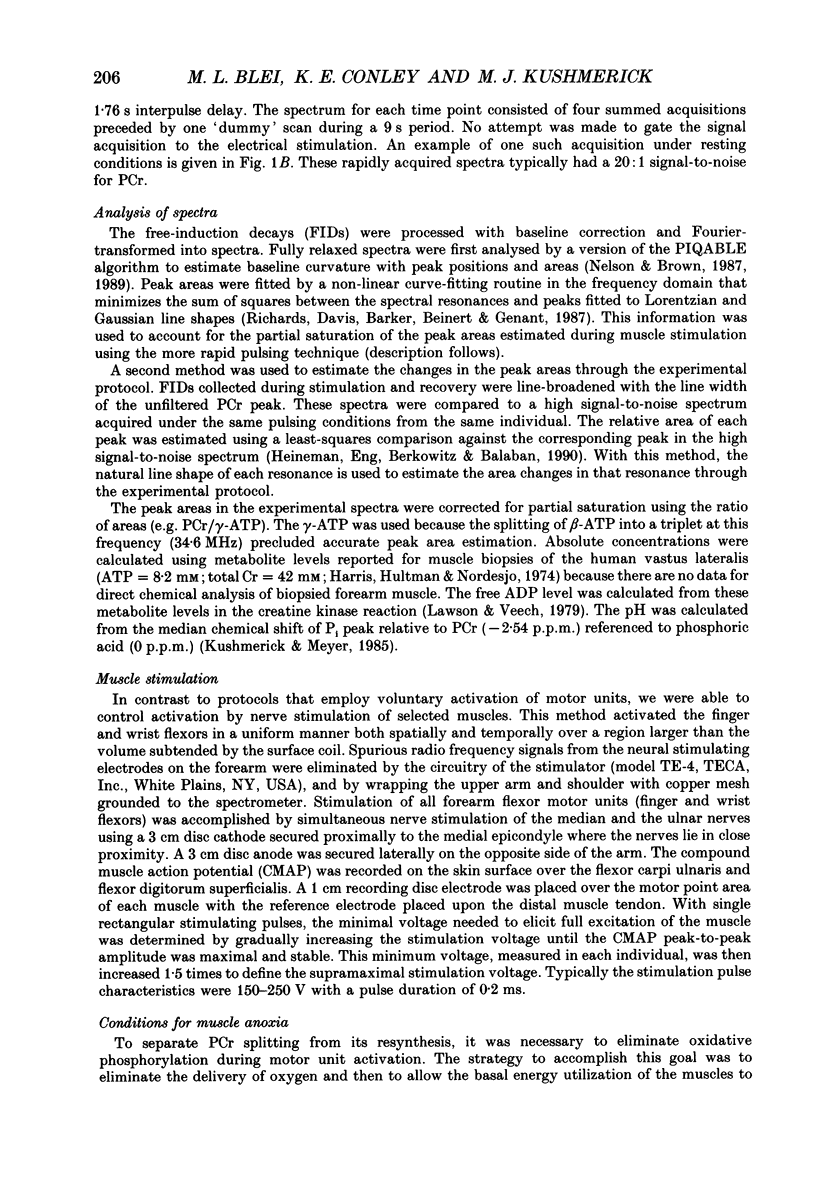
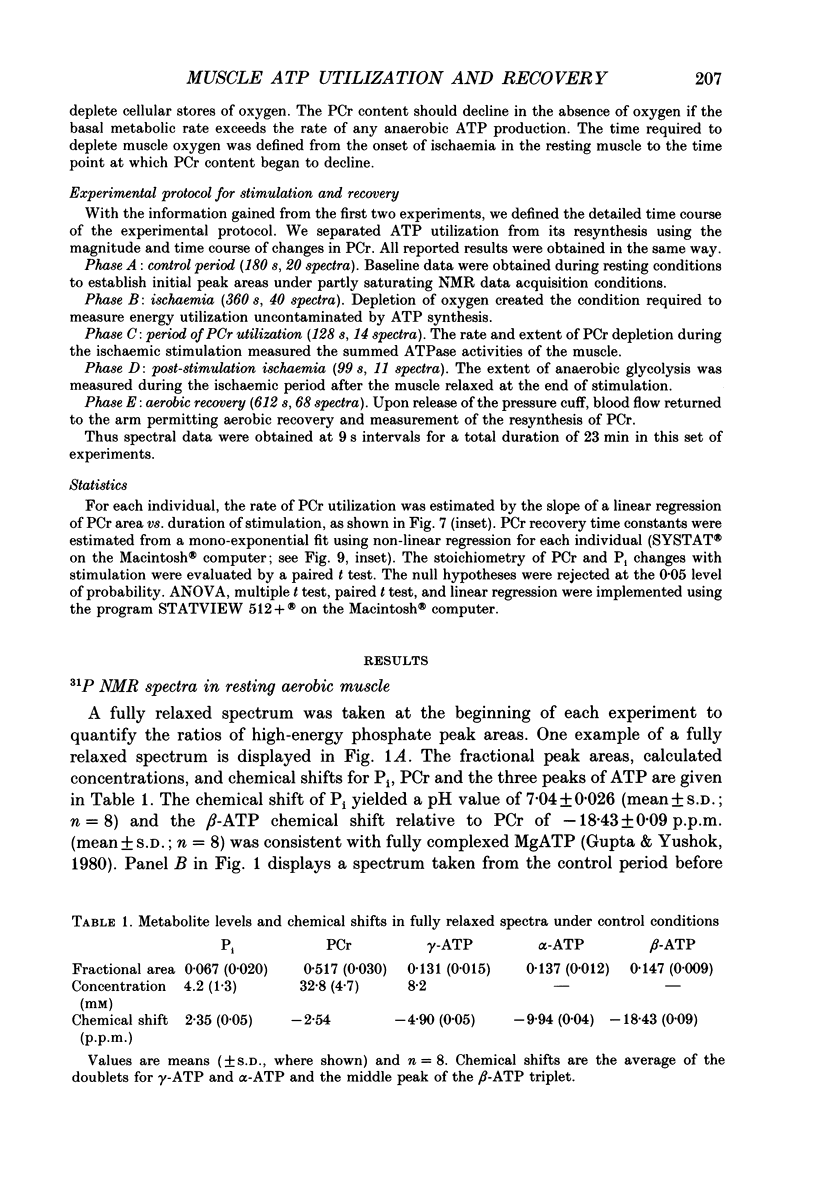
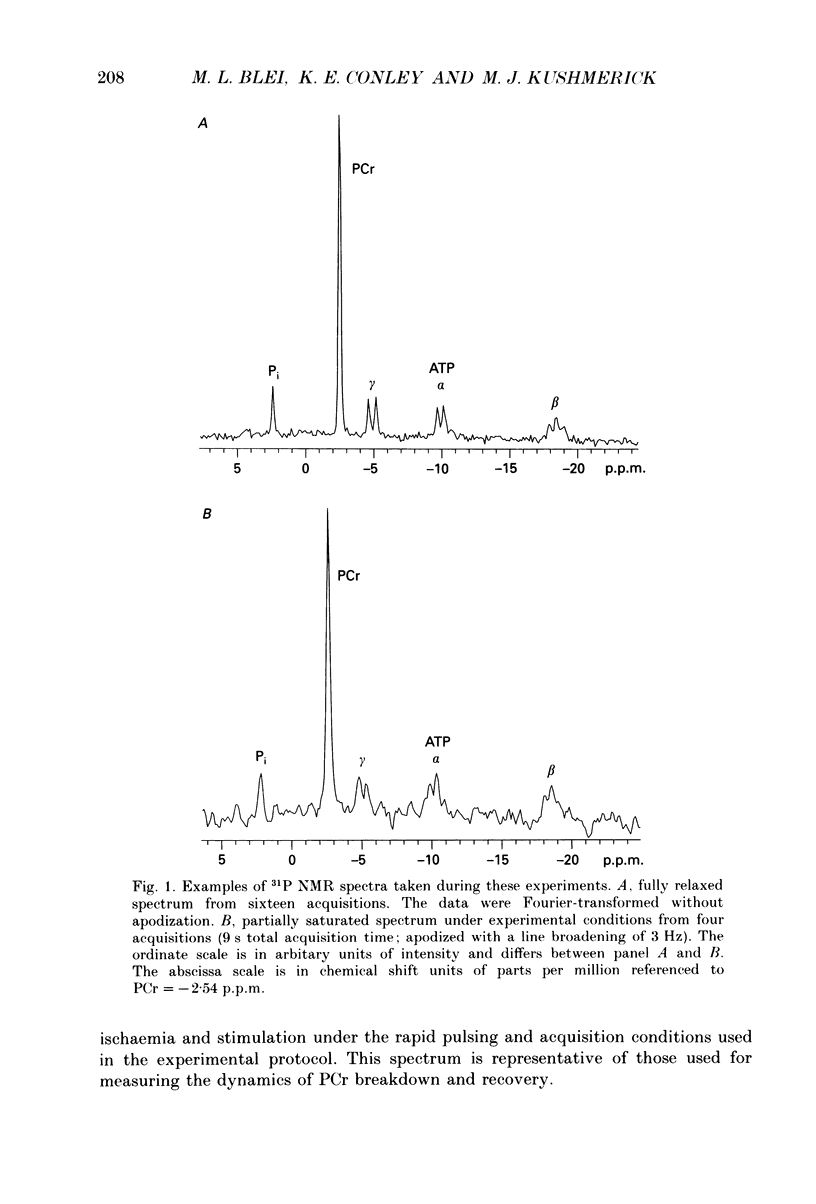
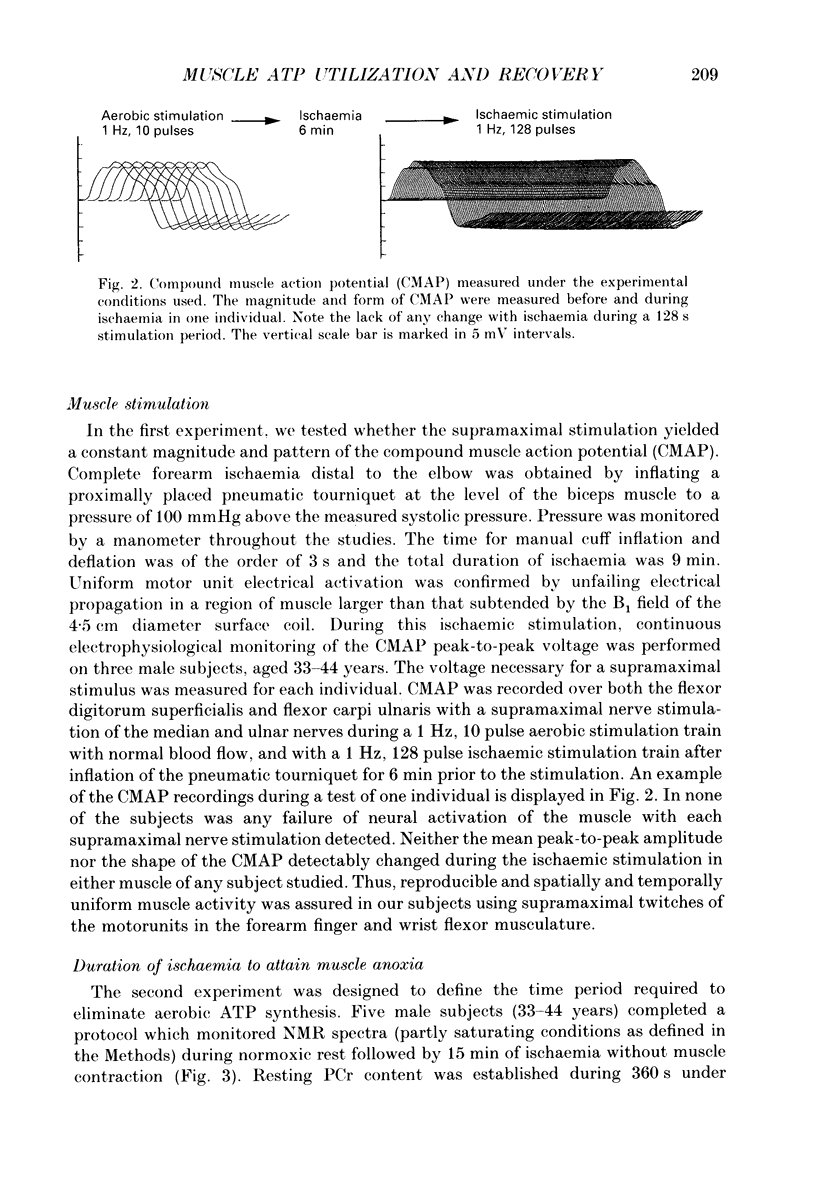
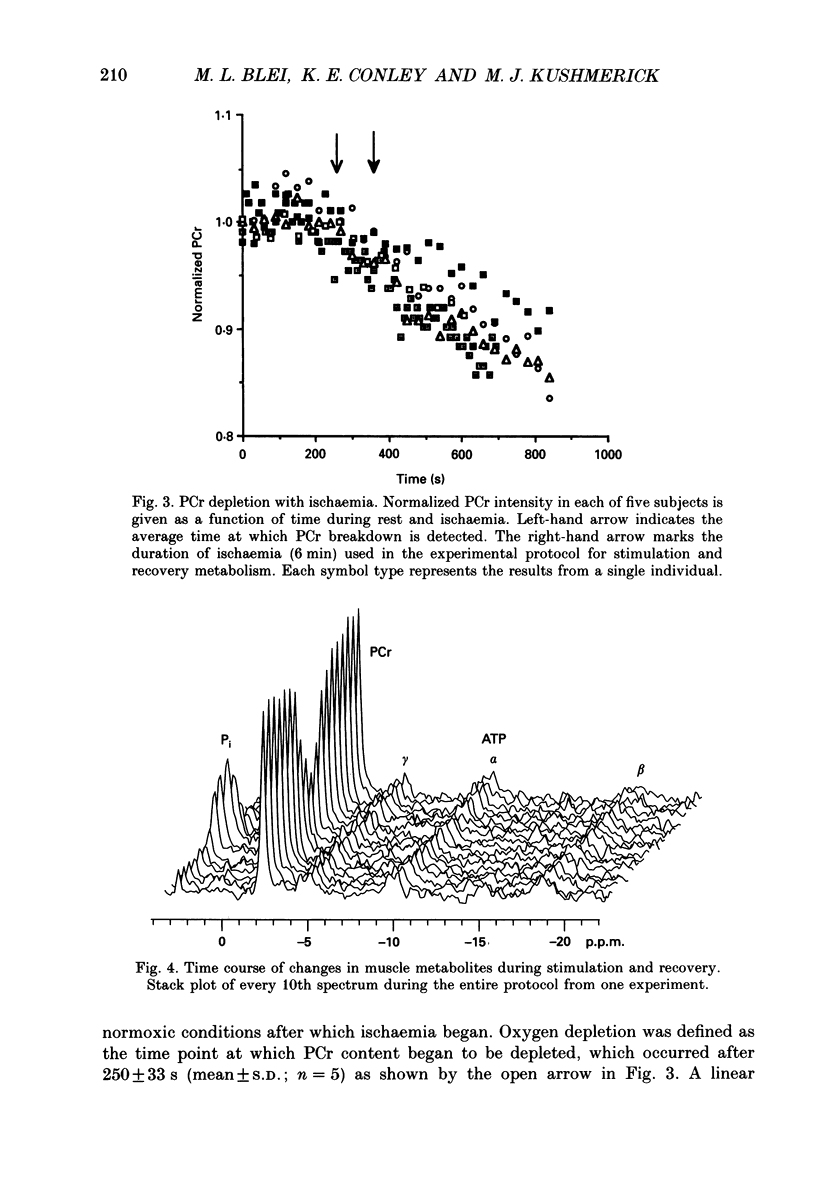
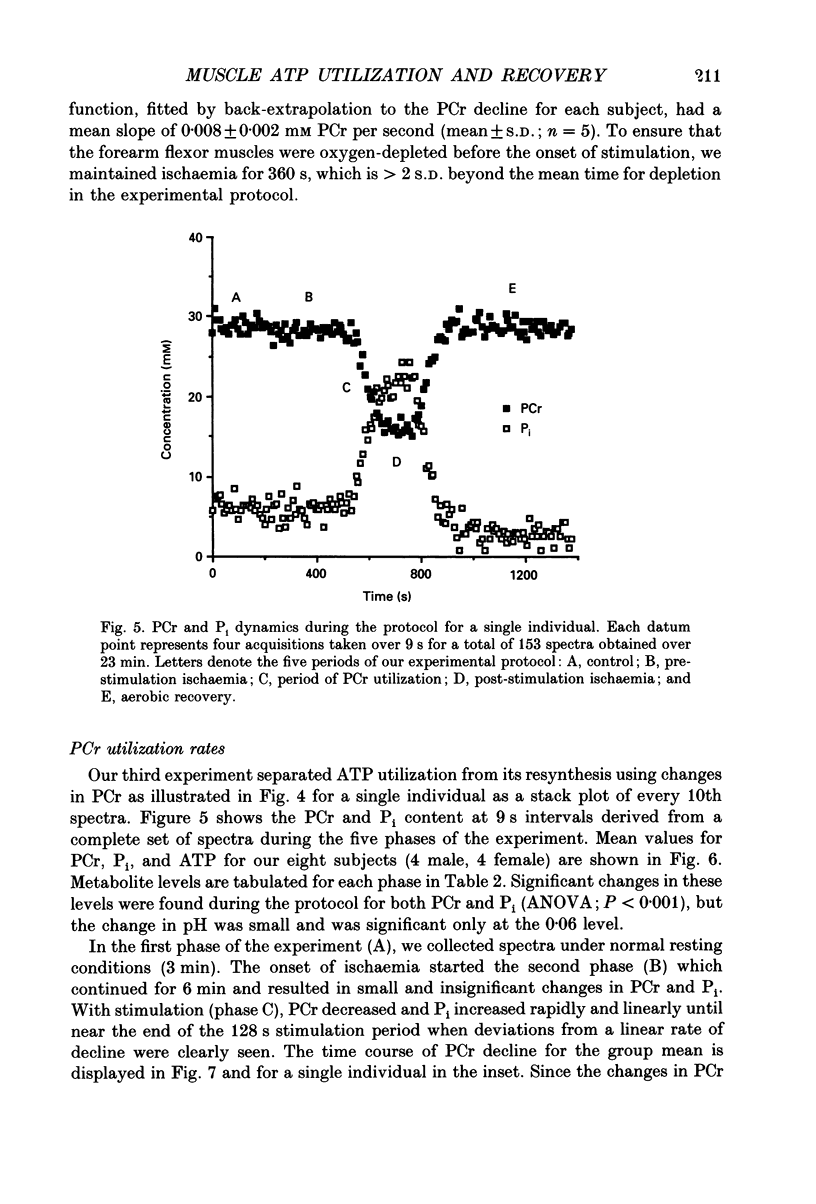
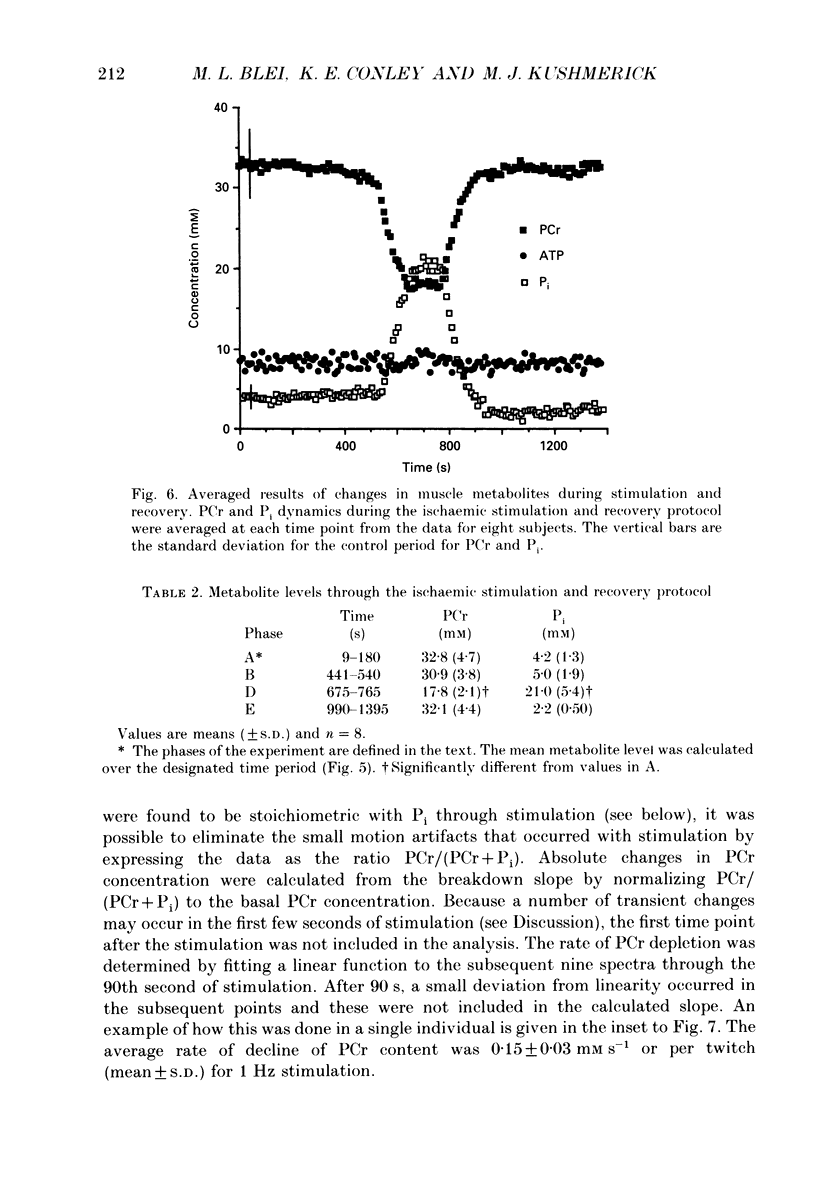
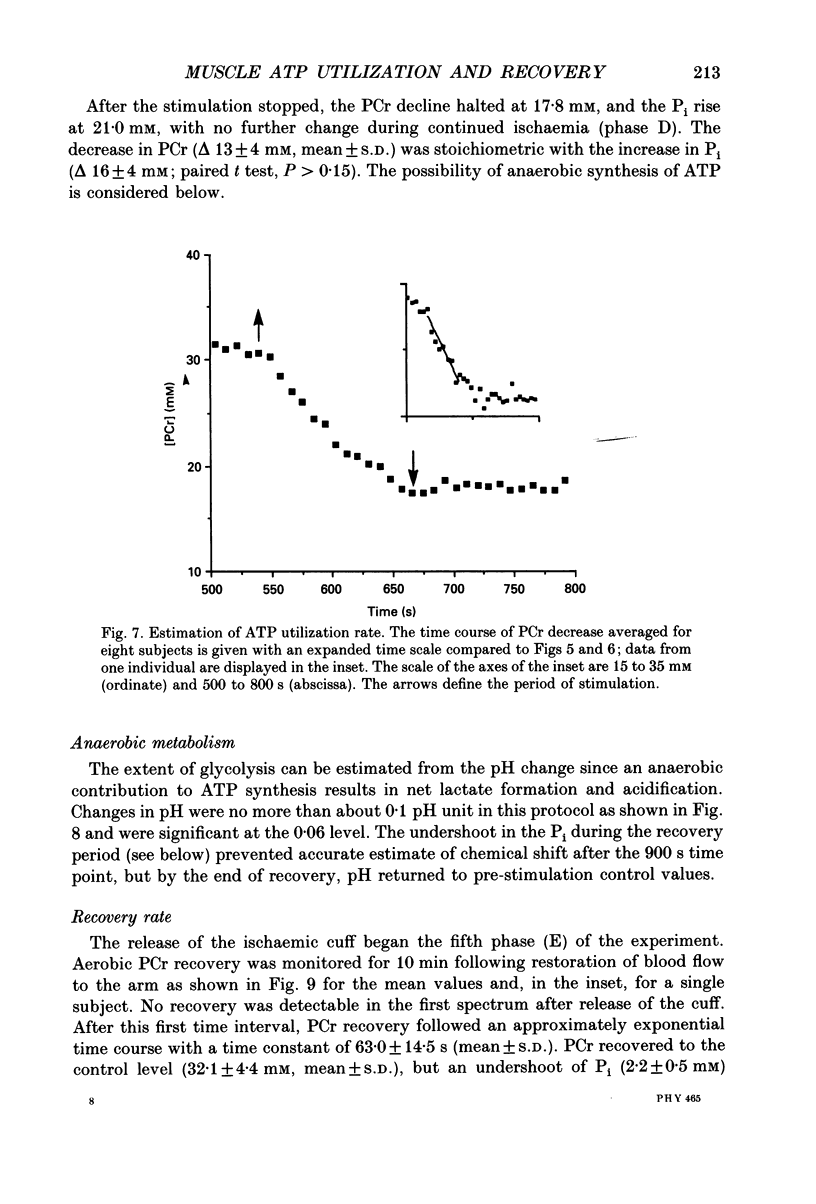
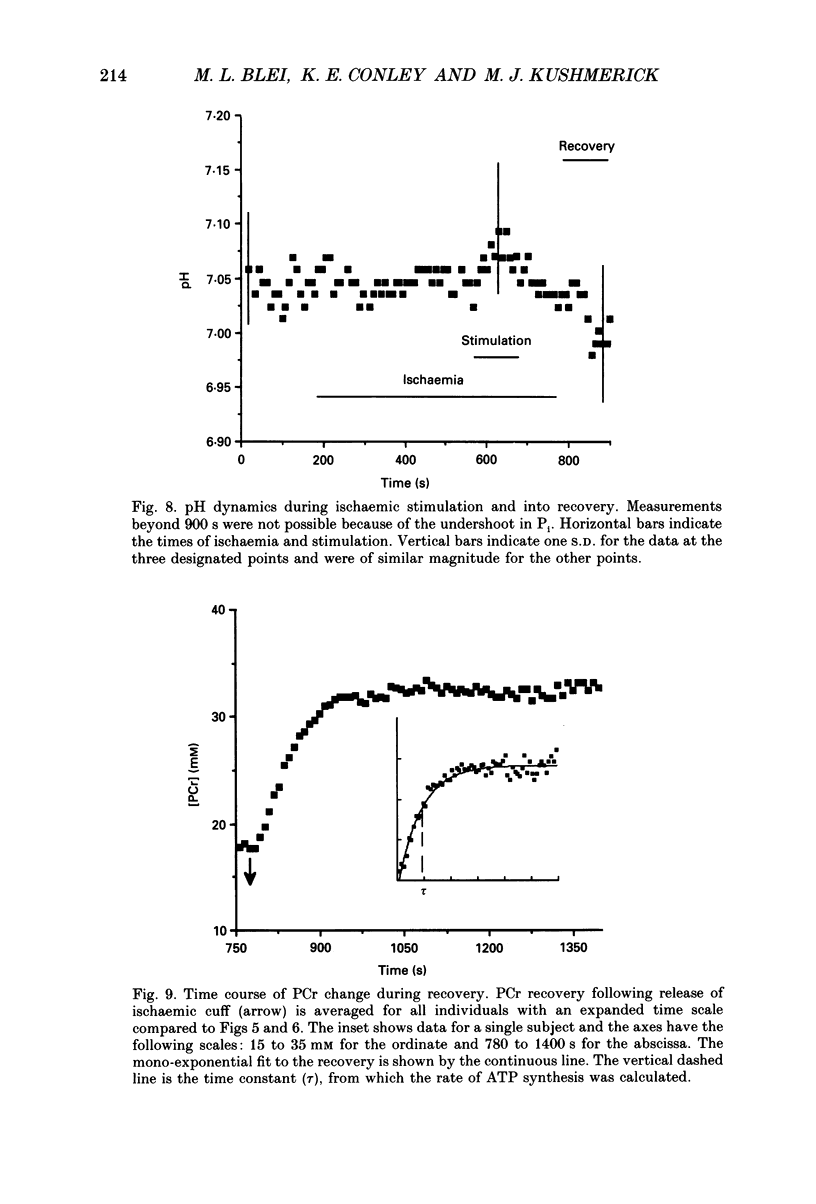
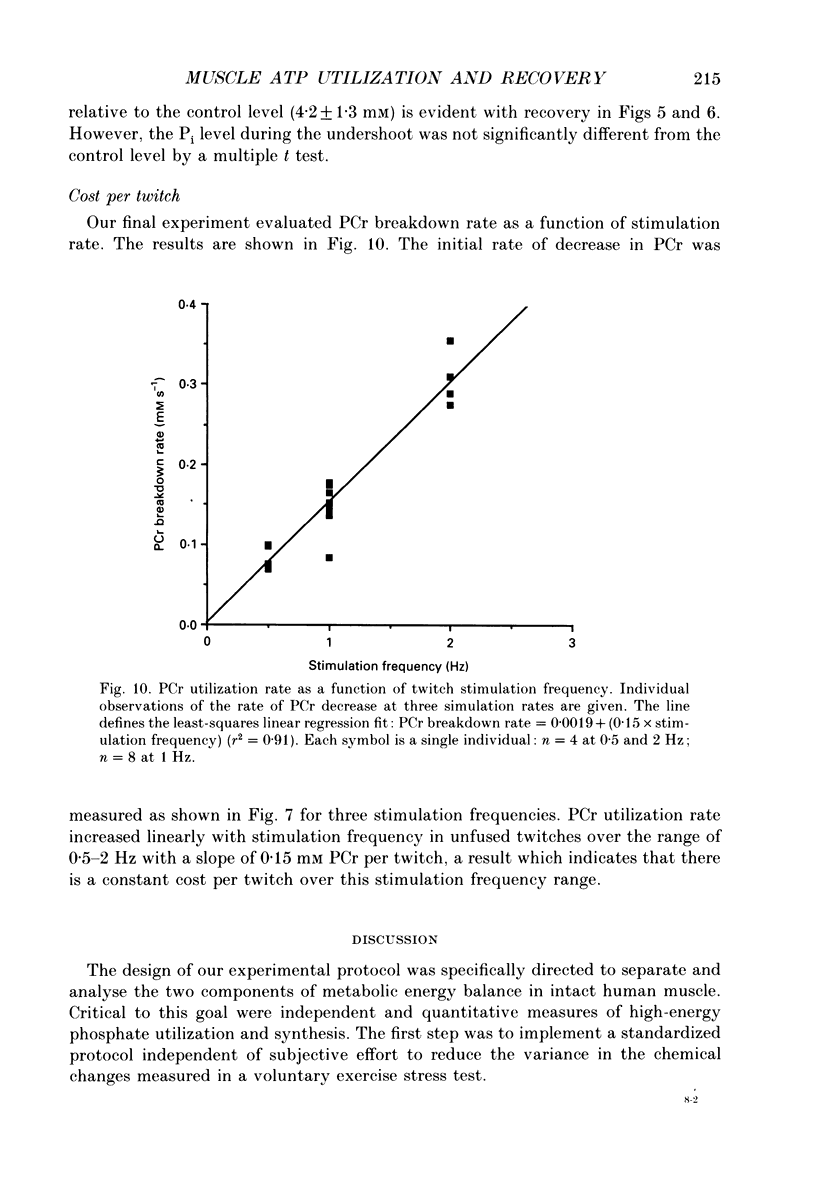
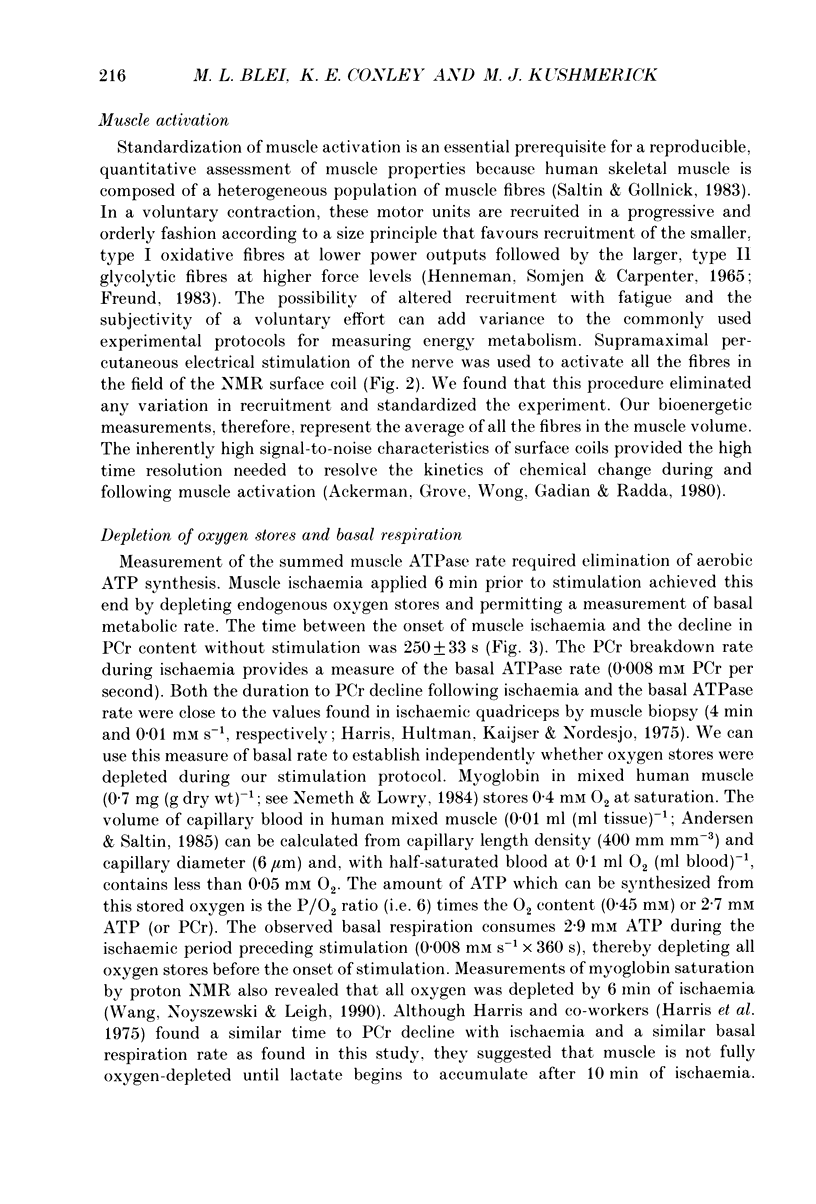
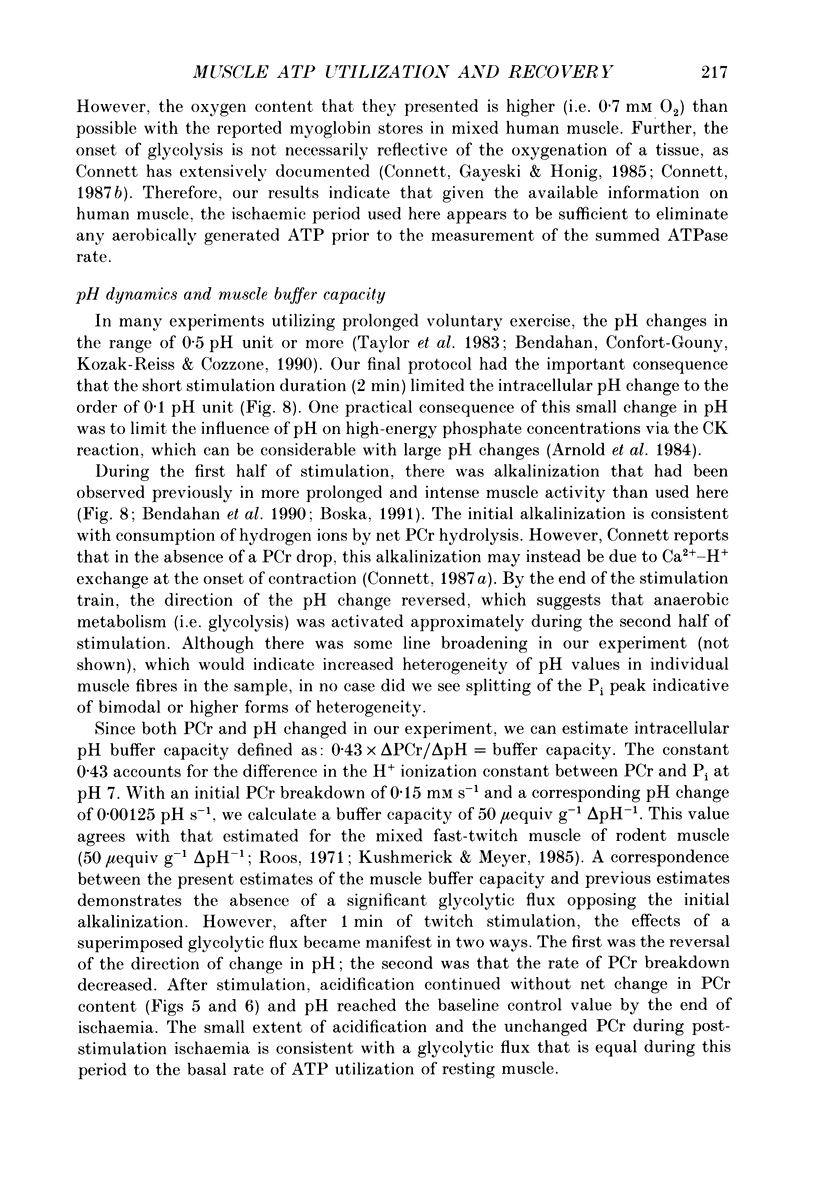
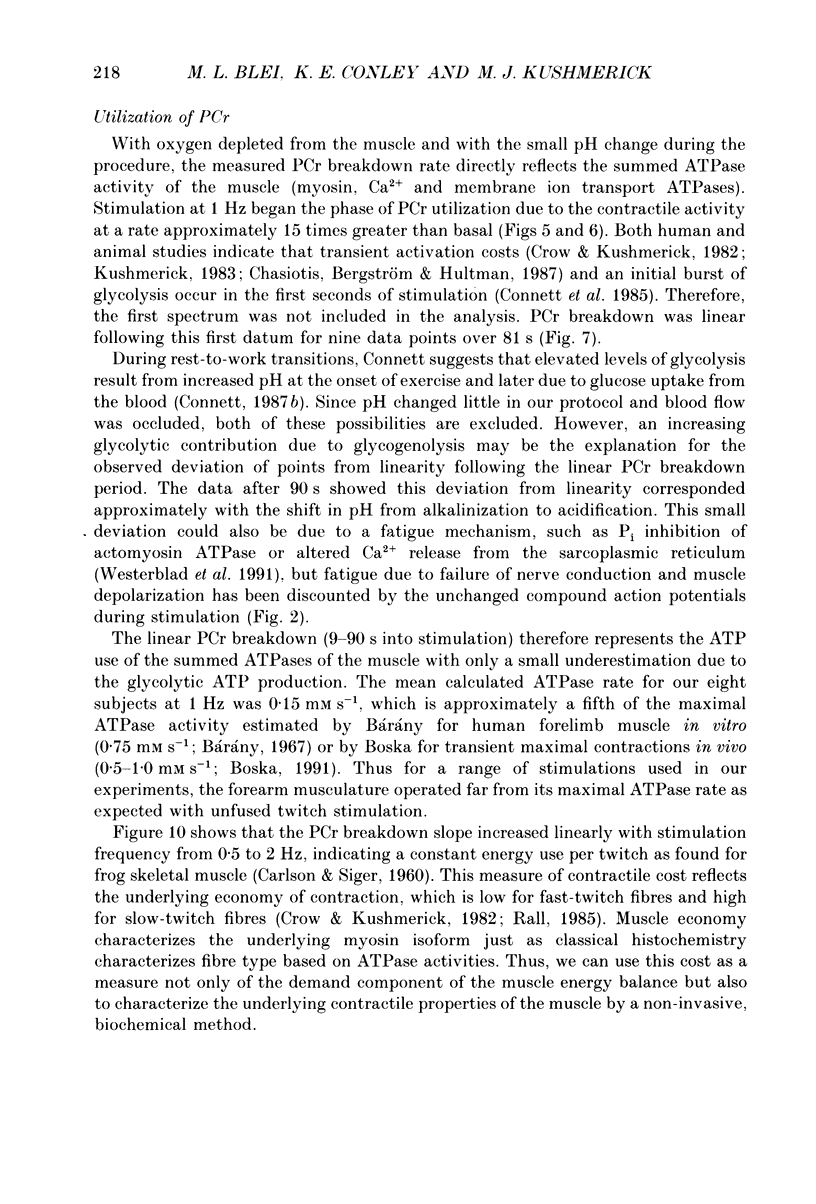
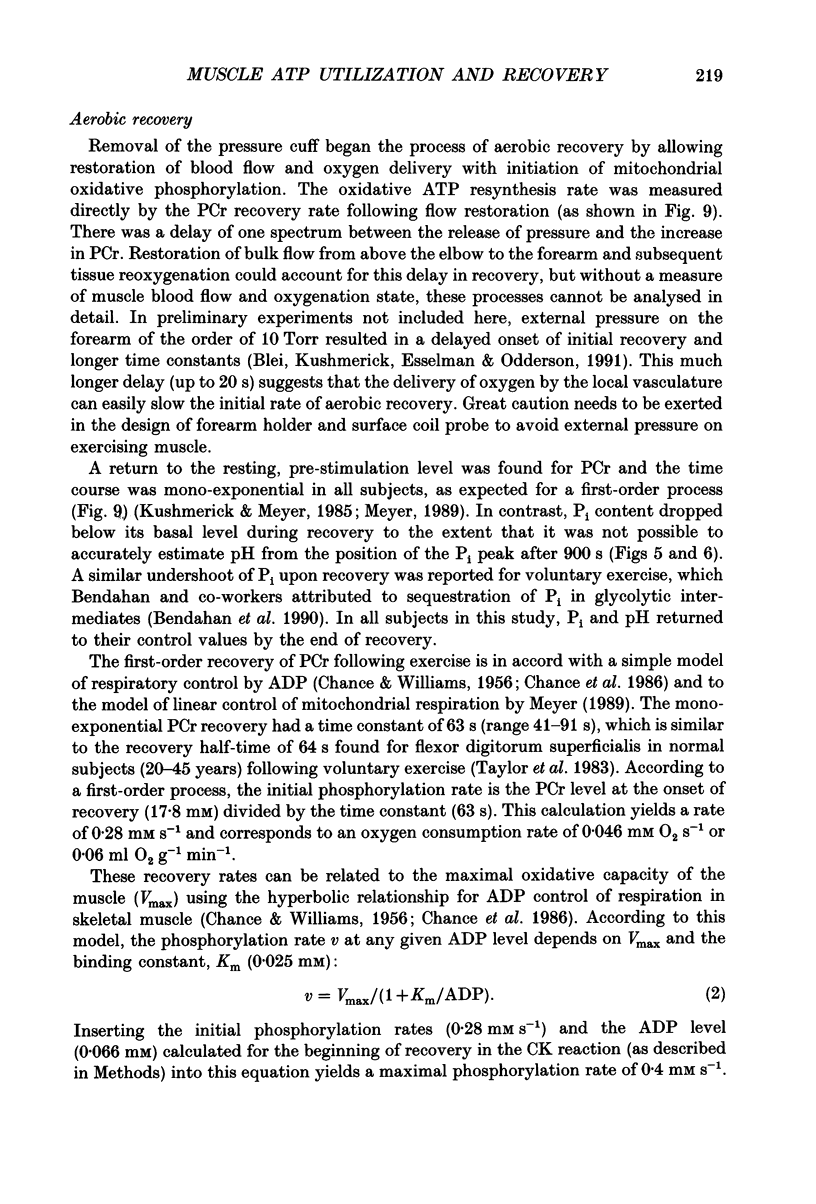
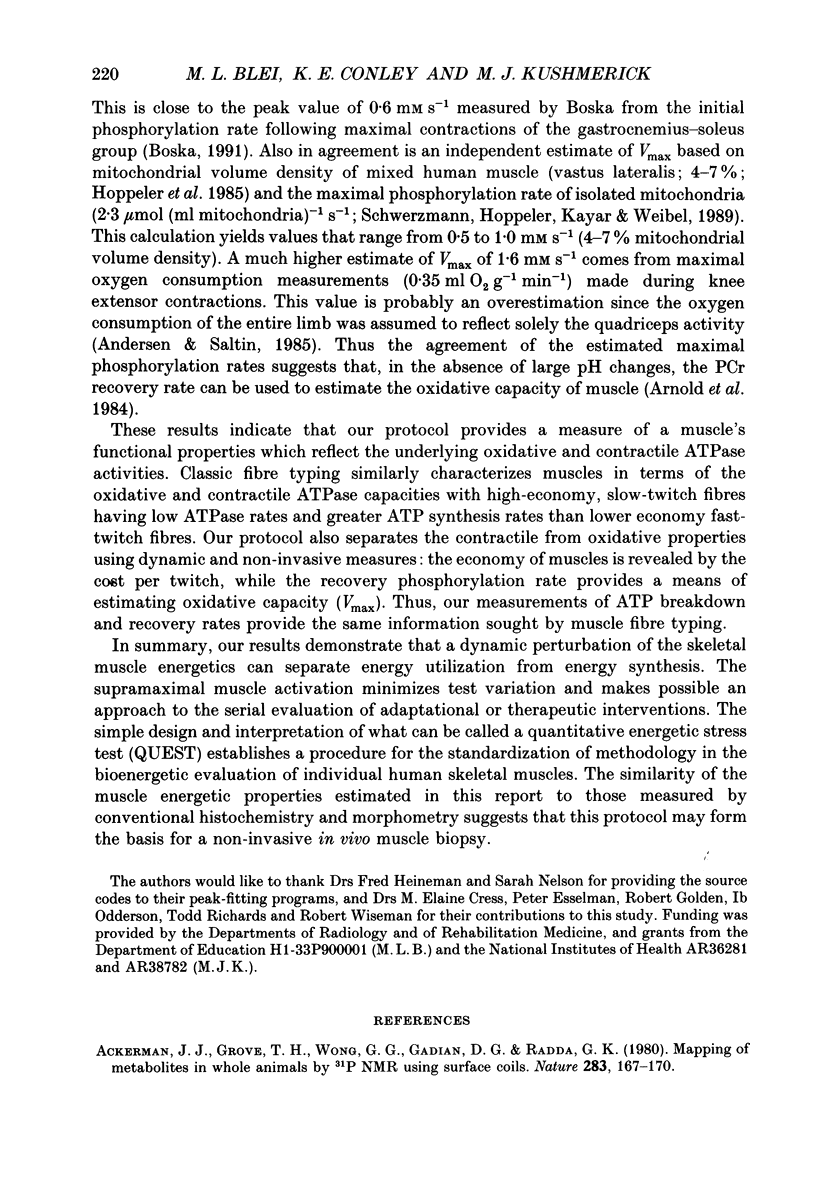
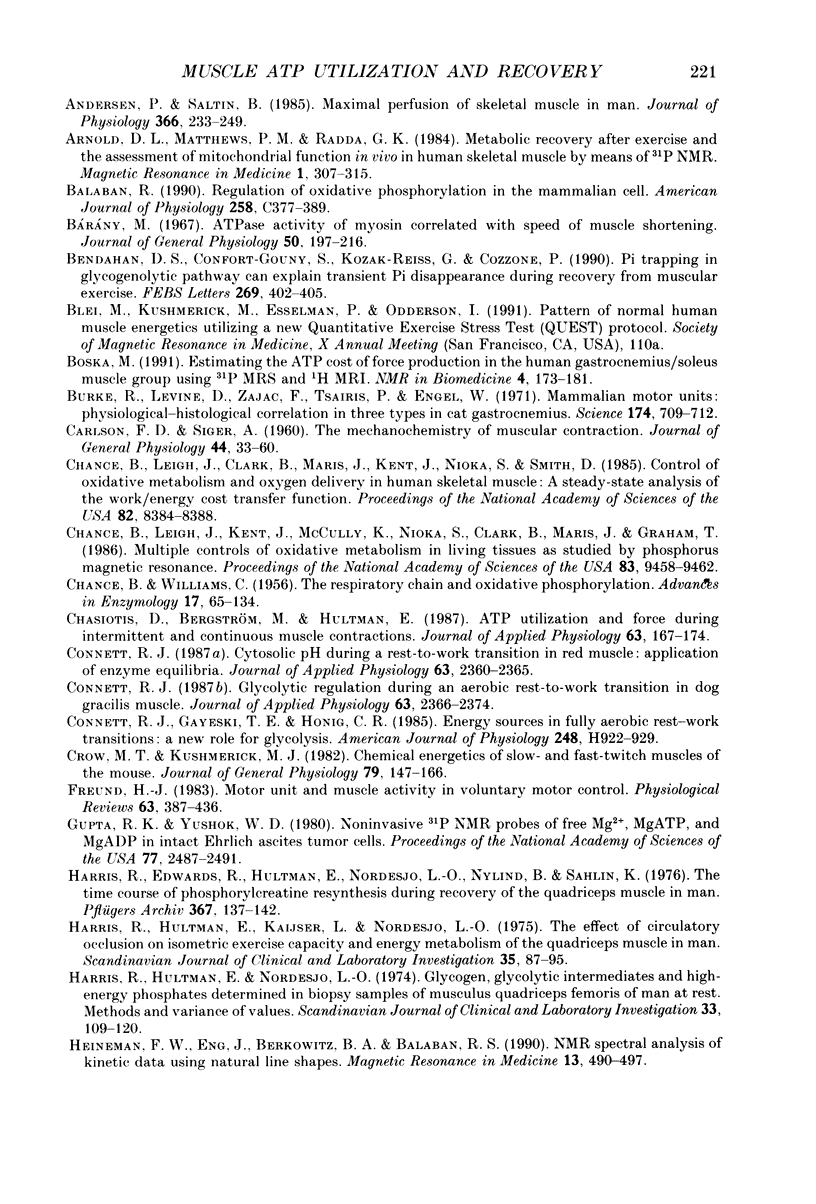
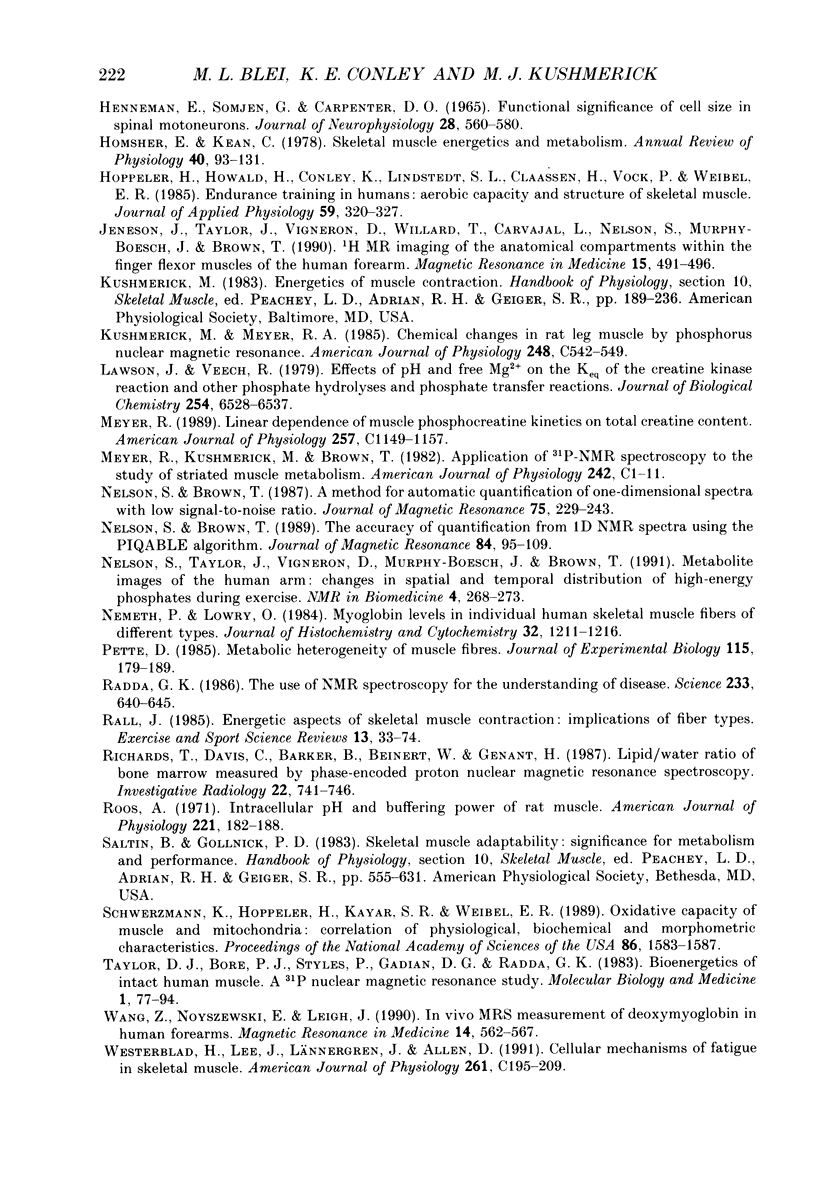
Selected References
These references are in PubMed. This may not be the complete list of references from this article.
- Ackerman J. J., Grove T. H., Wong G. G., Gadian D. G., Radda G. K. Mapping of metabolites in whole animals by 31P NMR using surface coils. Nature. 1980 Jan 10;283(5743):167–170. doi: 10.1038/283167a0. [DOI] [PubMed] [Google Scholar]
- Andersen P., Saltin B. Maximal perfusion of skeletal muscle in man. J Physiol. 1985 Sep;366:233–249. doi: 10.1113/jphysiol.1985.sp015794. [DOI] [PMC free article] [PubMed] [Google Scholar]
- Arnold D. L., Matthews P. M., Radda G. K. Metabolic recovery after exercise and the assessment of mitochondrial function in vivo in human skeletal muscle by means of 31P NMR. Magn Reson Med. 1984 Sep;1(3):307–315. doi: 10.1002/mrm.1910010303. [DOI] [PubMed] [Google Scholar]
- Balaban R. S. Regulation of oxidative phosphorylation in the mammalian cell. Am J Physiol. 1990 Mar;258(3 Pt 1):C377–C389. doi: 10.1152/ajpcell.1990.258.3.C377. [DOI] [PubMed] [Google Scholar]
- Bendahan D., Confort-Gouny S., Kozak-Reiss G., Cozzone P. J. Pi trapping in glycogenolytic pathway can explain transient Pi disappearance during recovery from muscular exercise. A 31P NMR study in the human. FEBS Lett. 1990 Sep 3;269(2):402–405. doi: 10.1016/0014-5793(90)81203-z. [DOI] [PubMed] [Google Scholar]
- Boska M. Estimating the ATP cost of force production in the human gastrocnemius/soleus muscle group using 31P MRS and 1H MRI. NMR Biomed. 1991 Aug;4(4):173–181. doi: 10.1002/nbm.1940040404. [DOI] [PubMed] [Google Scholar]
- Burke R. E., Levine D. N., Zajac F. E., 3rd Mammalian motor units: physiological-histochemical correlation in three types in cat gastrocnemius. Science. 1971 Nov 12;174(4010):709–712. doi: 10.1126/science.174.4010.709. [DOI] [PubMed] [Google Scholar]
- Bárány M. ATPase activity of myosin correlated with speed of muscle shortening. J Gen Physiol. 1967 Jul;50(6 Suppl):197–218. doi: 10.1085/jgp.50.6.197. [DOI] [PMC free article] [PubMed] [Google Scholar]
- CARLSON F. D., SIGER A. The mechanochemistry of muscular contraction. I. The isometric twitch. J Gen Physiol. 1960 Sep;44:33–60. doi: 10.1085/jgp.44.1.33. [DOI] [PMC free article] [PubMed] [Google Scholar]
- CHANCE B., WILLIAMS G. R. The respiratory chain and oxidative phosphorylation. Adv Enzymol Relat Subj Biochem. 1956;17:65–134. doi: 10.1002/9780470122624.ch2. [DOI] [PubMed] [Google Scholar]
- Chance B., Leigh J. S., Jr, Clark B. J., Maris J., Kent J., Nioka S., Smith D. Control of oxidative metabolism and oxygen delivery in human skeletal muscle: a steady-state analysis of the work/energy cost transfer function. Proc Natl Acad Sci U S A. 1985 Dec;82(24):8384–8388. doi: 10.1073/pnas.82.24.8384. [DOI] [PMC free article] [PubMed] [Google Scholar]
- Chance B., Leigh J. S., Jr, Kent J., McCully K., Nioka S., Clark B. J., Maris J. M., Graham T. Multiple controls of oxidative metabolism in living tissues as studied by phosphorus magnetic resonance. Proc Natl Acad Sci U S A. 1986 Dec;83(24):9458–9462. doi: 10.1073/pnas.83.24.9458. [DOI] [PMC free article] [PubMed] [Google Scholar]
- Chasiotis D., Bergström M., Hultman E. ATP utilization and force during intermittent and continuous muscle contractions. J Appl Physiol (1985) 1987 Jul;63(1):167–174. doi: 10.1152/jappl.1987.63.1.167. [DOI] [PubMed] [Google Scholar]
- Connett R. J. Cytosolic pH during a rest-to-work transition in red muscle: application of enzyme equilibria. J Appl Physiol (1985) 1987 Dec;63(6):2360–2365. doi: 10.1152/jappl.1987.63.6.2360. [DOI] [PubMed] [Google Scholar]
- Connett R. J., Gayeski T. E., Honig C. R. Energy sources in fully aerobic rest-work transitions: a new role for glycolysis. Am J Physiol. 1985 Jun;248(6 Pt 2):H922–H929. doi: 10.1152/ajpheart.1985.248.6.H922. [DOI] [PubMed] [Google Scholar]
- Connett R. J. Glycolytic regulation during an aerobic rest-to-work transition in dog gracilis muscle. J Appl Physiol (1985) 1987 Dec;63(6):2366–2374. doi: 10.1152/jappl.1987.63.6.2366. [DOI] [PubMed] [Google Scholar]
- Crow M. T., Kushmerick M. J. Chemical energetics of slow- and fast-twitch muscles of the mouse. J Gen Physiol. 1982 Jan;79(1):147–166. doi: 10.1085/jgp.79.1.147. [DOI] [PMC free article] [PubMed] [Google Scholar]
- Freund H. J. Motor unit and muscle activity in voluntary motor control. Physiol Rev. 1983 Apr;63(2):387–436. doi: 10.1152/physrev.1983.63.2.387. [DOI] [PubMed] [Google Scholar]
- Gupta R. K., Yushok W. D. Noninvasive 31P NMR probes of free Mg2+, MgATP, and MgADP in intact Ehrlich ascites tumor cells. Proc Natl Acad Sci U S A. 1980 May;77(5):2487–2491. doi: 10.1073/pnas.77.5.2487. [DOI] [PMC free article] [PubMed] [Google Scholar]
- HENNEMAN E., SOMJEN G., CARPENTER D. O. FUNCTIONAL SIGNIFICANCE OF CELL SIZE IN SPINAL MOTONEURONS. J Neurophysiol. 1965 May;28:560–580. doi: 10.1152/jn.1965.28.3.560. [DOI] [PubMed] [Google Scholar]
- Harris R. C., Edwards R. H., Hultman E., Nordesjö L. O., Nylind B., Sahlin K. The time course of phosphorylcreatine resynthesis during recovery of the quadriceps muscle in man. Pflugers Arch. 1976 Dec 28;367(2):137–142. doi: 10.1007/BF00585149. [DOI] [PubMed] [Google Scholar]
- Harris R. C., Hultman E., Kaijser L., Nordesjö L. O. The effect of circulatory occlusion on isometric exercise capacity and energy metabolism of the quadriceps muscle in man. Scand J Clin Lab Invest. 1975 Jan;35(1):87–95. [PubMed] [Google Scholar]
- Harris R. C., Hultman E., Nordesjö L. O. Glycogen, glycolytic intermediates and high-energy phosphates determined in biopsy samples of musculus quadriceps femoris of man at rest. Methods and variance of values. Scand J Clin Lab Invest. 1974 Apr;33(2):109–120. [PubMed] [Google Scholar]
- Heineman F. W., Eng J., Berkowitz B. A., Balaban R. S. NMR spectral analysis of kinetic data using natural lineshapes. Magn Reson Med. 1990 Mar;13(3):490–497. doi: 10.1002/mrm.1910130316. [DOI] [PubMed] [Google Scholar]
- Homsher E., Kean C. J. Skeletal muscle energetics and metabolism. Annu Rev Physiol. 1978;40:93–131. doi: 10.1146/annurev.ph.40.030178.000521. [DOI] [PubMed] [Google Scholar]
- Hoppeler H., Howald H., Conley K., Lindstedt S. L., Claassen H., Vock P., Weibel E. R. Endurance training in humans: aerobic capacity and structure of skeletal muscle. J Appl Physiol (1985) 1985 Aug;59(2):320–327. doi: 10.1152/jappl.1985.59.2.320. [DOI] [PubMed] [Google Scholar]
- Jeneson J. A., Taylor J. S., Vigneron D. B., Willard T. S., Carvajal L., Nelson S. J., Murphy-Boesch J., Brown T. R. 1H MR imaging of anatomical compartments within the finger flexor muscles of the human forearm. Magn Reson Med. 1990 Sep;15(3):491–496. doi: 10.1002/mrm.1910150316. [DOI] [PubMed] [Google Scholar]
- Kushmerick M. J., Meyer R. A. Chemical changes in rat leg muscle by phosphorus nuclear magnetic resonance. Am J Physiol. 1985 May;248(5 Pt 1):C542–C549. doi: 10.1152/ajpcell.1985.248.5.C542. [DOI] [PubMed] [Google Scholar]
- Lawson J. W., Veech R. L. Effects of pH and free Mg2+ on the Keq of the creatine kinase reaction and other phosphate hydrolyses and phosphate transfer reactions. J Biol Chem. 1979 Jul 25;254(14):6528–6537. [PubMed] [Google Scholar]
- Meyer R. A. Linear dependence of muscle phosphocreatine kinetics on total creatine content. Am J Physiol. 1989 Dec;257(6 Pt 1):C1149–C1157. doi: 10.1152/ajpcell.1989.257.6.C1149. [DOI] [PubMed] [Google Scholar]
- Nelson S. J., Taylor J. S., Vigneron D. B., Murphy-Boesch J., Brown T. R. Metabolite images of the human arm: changes in spatial and temporal distribution of high energy phosphates during exercise. NMR Biomed. 1991 Dec;4(6):268–273. doi: 10.1002/nbm.1940040604. [DOI] [PubMed] [Google Scholar]
- Nemeth P. M., Lowry O. H. Myoglobin levels in individual human skeletal muscle fibers of different types. J Histochem Cytochem. 1984 Nov;32(11):1211–1216. doi: 10.1177/32.11.6491255. [DOI] [PubMed] [Google Scholar]
- Pette D. Metabolic heterogeneity of muscle fibres. J Exp Biol. 1985 Mar;115:179–189. doi: 10.1242/jeb.115.1.179. [DOI] [PubMed] [Google Scholar]
- Radda G. K. The use of NMR spectroscopy for the understanding of disease. Science. 1986 Aug 8;233(4764):640–645. doi: 10.1126/science.3726553. [DOI] [PubMed] [Google Scholar]
- Rall J. A. Energetic aspects of skeletal muscle contraction: implications of fiber types. Exerc Sport Sci Rev. 1985;13:33–74. [PubMed] [Google Scholar]
- Richards T. L., Davis C. A., Barker B. R., Beinert W. D., Genant H. K. Lipid/water ratio of bone marrow measured by phase-encoded proton nuclear magnetic resonance spectroscopy. Invest Radiol. 1987 Sep;22(9):741–746. doi: 10.1097/00004424-198709000-00008. [DOI] [PubMed] [Google Scholar]
- Roos A. Intracellular pH and buffering power of rat muscle. Am J Physiol. 1971 Jul;221(1):182–188. doi: 10.1152/ajplegacy.1971.221.1.182. [DOI] [PubMed] [Google Scholar]
- Schwerzmann K., Hoppeler H., Kayar S. R., Weibel E. R. Oxidative capacity of muscle and mitochondria: correlation of physiological, biochemical, and morphometric characteristics. Proc Natl Acad Sci U S A. 1989 Mar;86(5):1583–1587. doi: 10.1073/pnas.86.5.1583. [DOI] [PMC free article] [PubMed] [Google Scholar]
- Taylor D. J., Bore P. J., Styles P., Gadian D. G., Radda G. K. Bioenergetics of intact human muscle. A 31P nuclear magnetic resonance study. Mol Biol Med. 1983 Jul;1(1):77–94. [PubMed] [Google Scholar]
- Wang Z. Y., Noyszewski E. A., Leigh J. S., Jr In vivo MRS measurement of deoxymyoglobin in human forearms. Magn Reson Med. 1990 Jun;14(3):562–567. doi: 10.1002/mrm.1910140314. [DOI] [PubMed] [Google Scholar]
- Westerblad H., Lee J. A., Lännergren J., Allen D. G. Cellular mechanisms of fatigue in skeletal muscle. Am J Physiol. 1991 Aug;261(2 Pt 1):C195–C209. doi: 10.1152/ajpcell.1991.261.2.C195. [DOI] [PubMed] [Google Scholar]