Abstract
1. The gating properties of two types of Na+ channels were studied in neurones isolated from rat dorsal root ganglia using the whole cell variation of the patch electrode voltage-clamp technique. 2. Two types of Na+ currents (INa) were identified on the basis of their sensitivity to tetrodotoxin (TTX). One type was insensitive to TTX (up to 0.1 mM), while the other type was blocked by 1 nM of TTX. Whereas they were both insensitive to 50 microM Cd2+, a high concentration (2 mM) of Co2+ selectively inhibited the TTX-insensitive type. 3. The activation thresholds were about -60 and -40 mV for the TTX-sensitive and the TTX-insensitive INa, respectively. Activation of the TTX-sensitive INa developed with a sigmoidal time course which was described by m3 kinetics, whereas the activation of the TTX-insensitive INa was described by a single exponential function. A deactivation process, as measured by the tail current upon repolarization, followed an exponential decay in either type of INa. 4. The rate constant of activation indicated that under comparable membrane potential conditions, the TTX-insensitive channels open 4-5 times slower than the TTX-sensitive ones upon depolarization. Likewise, the rate constant of inactivation indicated that the TTX-insensitive channels inactivate 3-7 times more slowly than the TTX-sensitive ones upon repolarization. 5. The steady-state activation curve for the TTX-insensitive INa was shifted about 20 mV in the positive direction from that for the TTX-sensitive INa. 6. The steady-state inactivation curve for the TTX-insensitive INa as obtained with a 0.5 s prepulse was shifted about 26 mV in the positive direction from that for the TTX-sensitive INa, indicating a greater availability for the TTX-insensitive INa in depolarized membrane. However, on increasing the duration of prepulse, the inactivation curve for the TTX-insensitive INa, but not for the TTX-sensitive INa, shifted in the negative direction due to an extremely slow inactivation process in the TTX-insensitive INa. Consequently, an overlap between the activation and inactivation curves which causes a steady influx of Na+ (window current) became progressively reduce. 7. The time course of INa decay was best described by a single exponential process in either the TTX-sensitive or TTX-insensitive INa, whereas the development of inactivation and the recovery from inactivation, which were measured by a conventional double-pulse protocol, followed a second order process in either channel type.(ABSTRACT TRUNCATED AT 400 WORDS)
Full text
PDF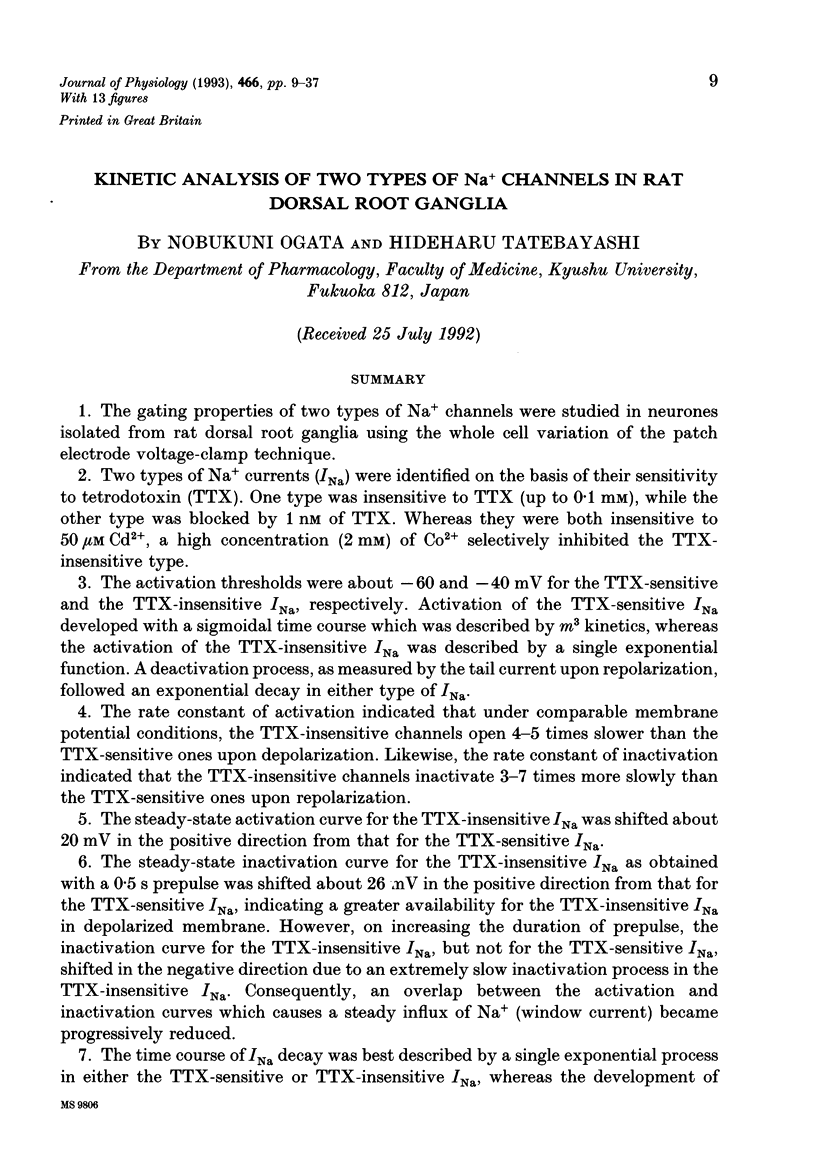
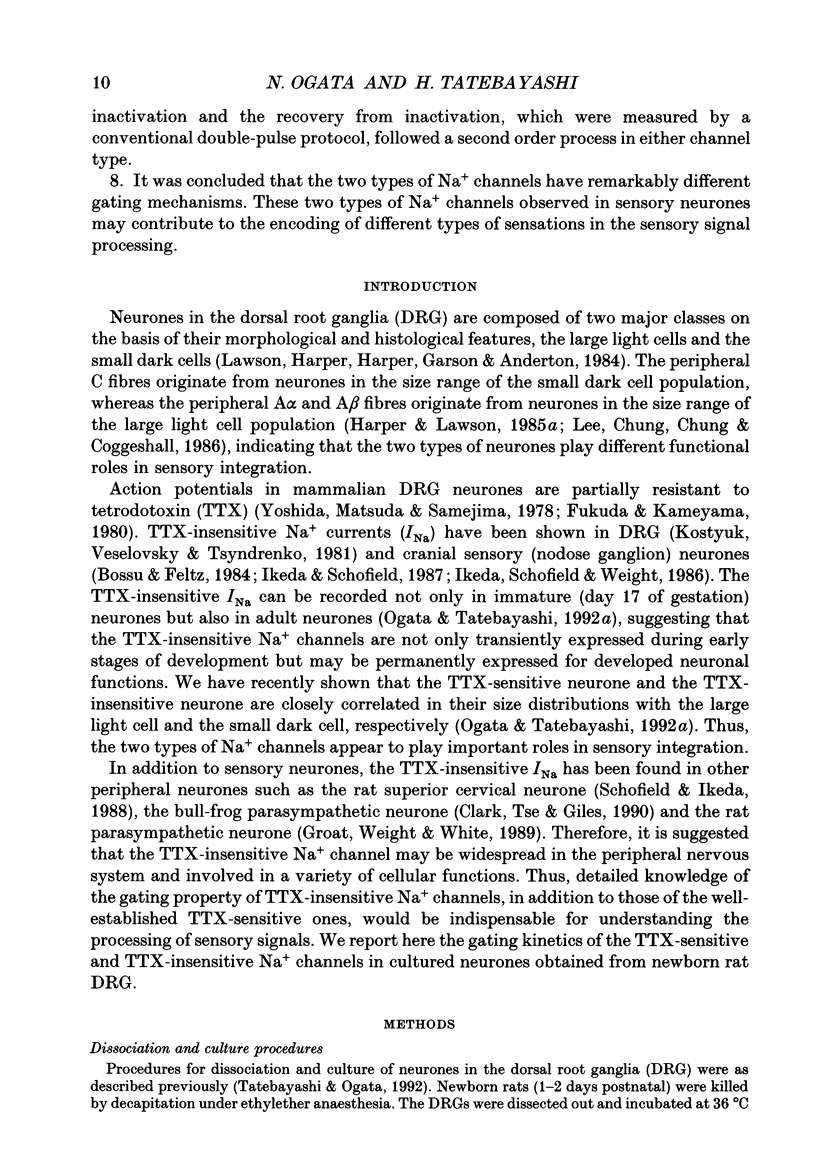
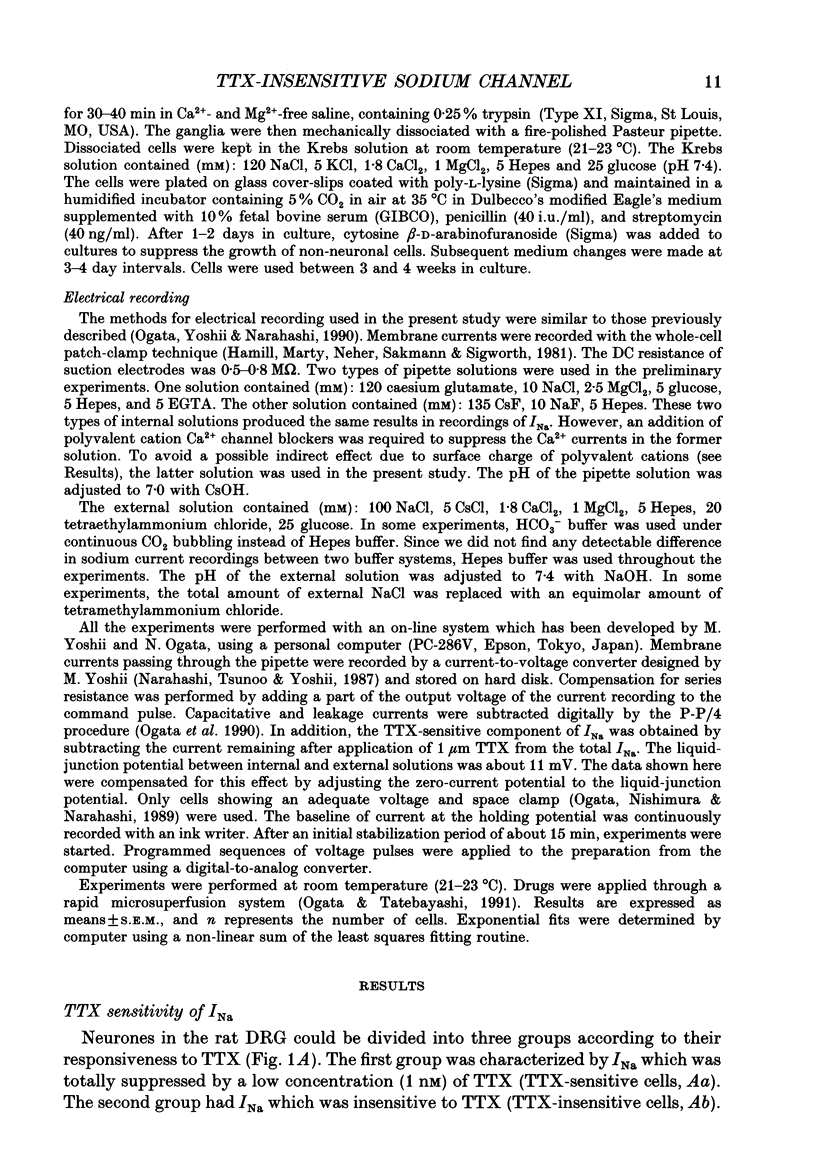
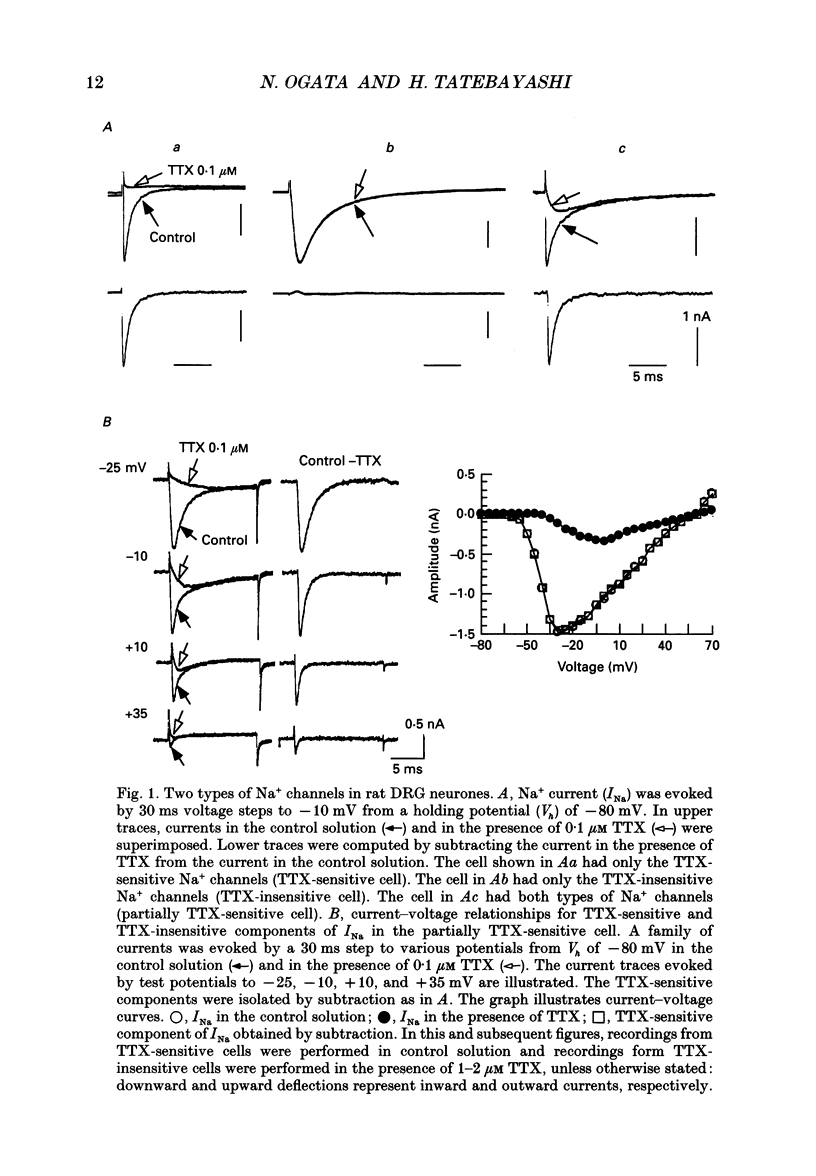
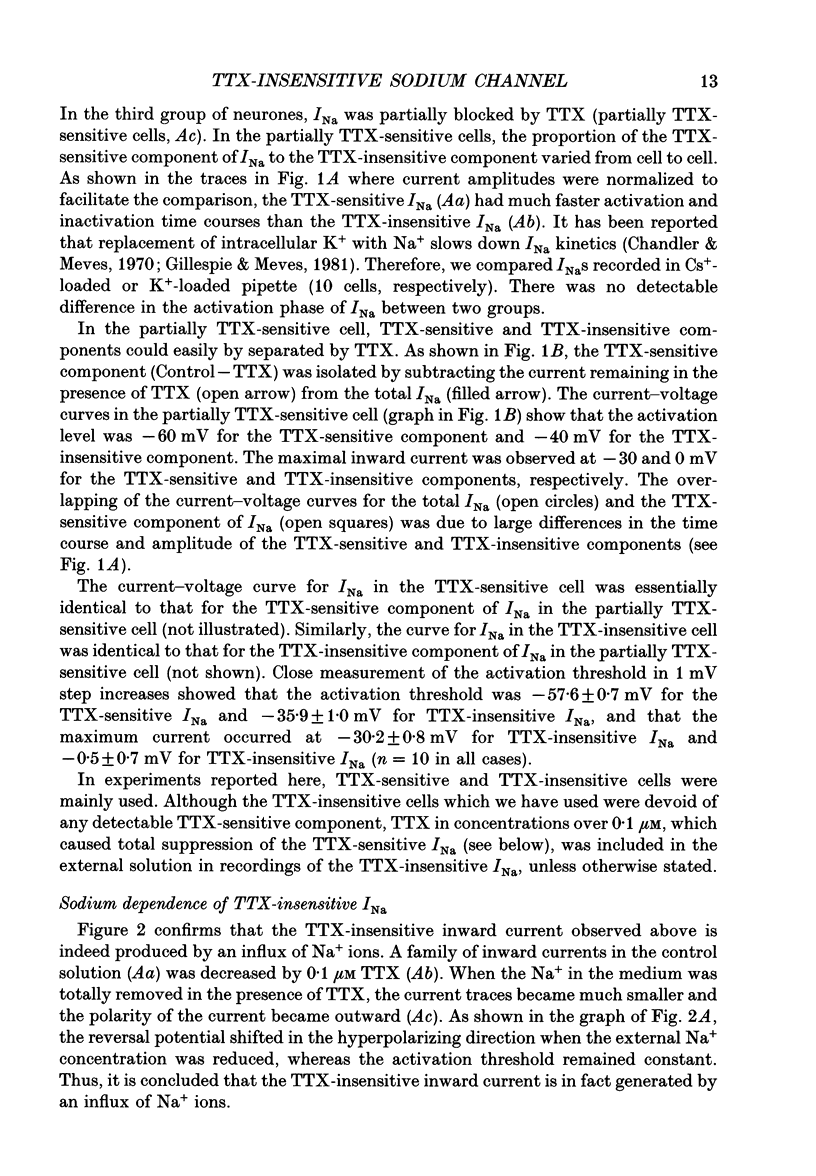
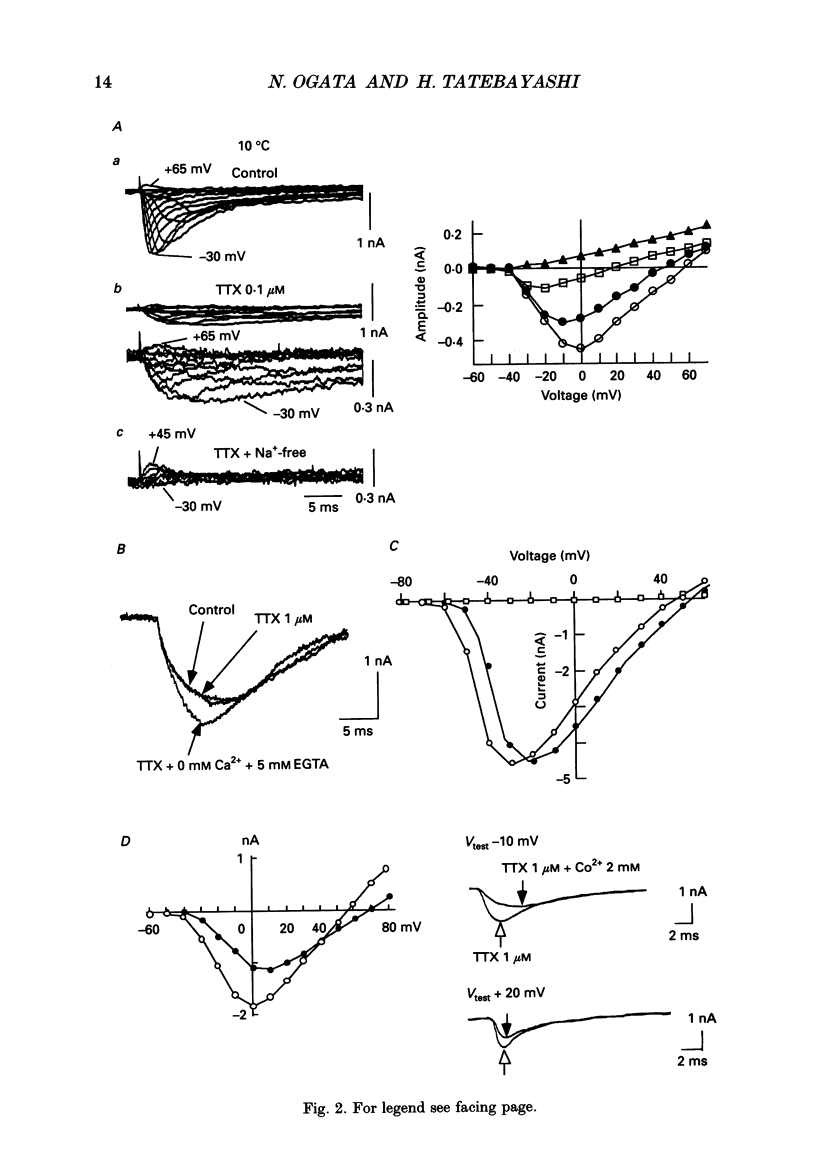
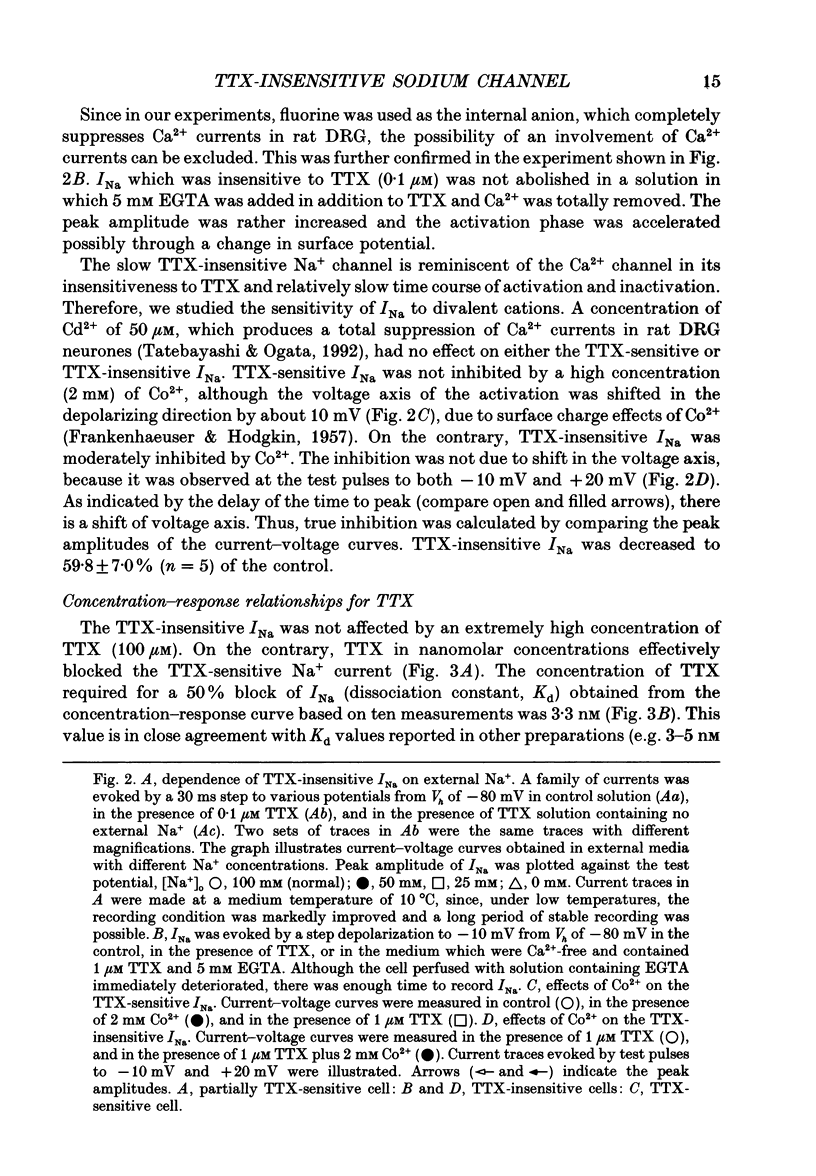
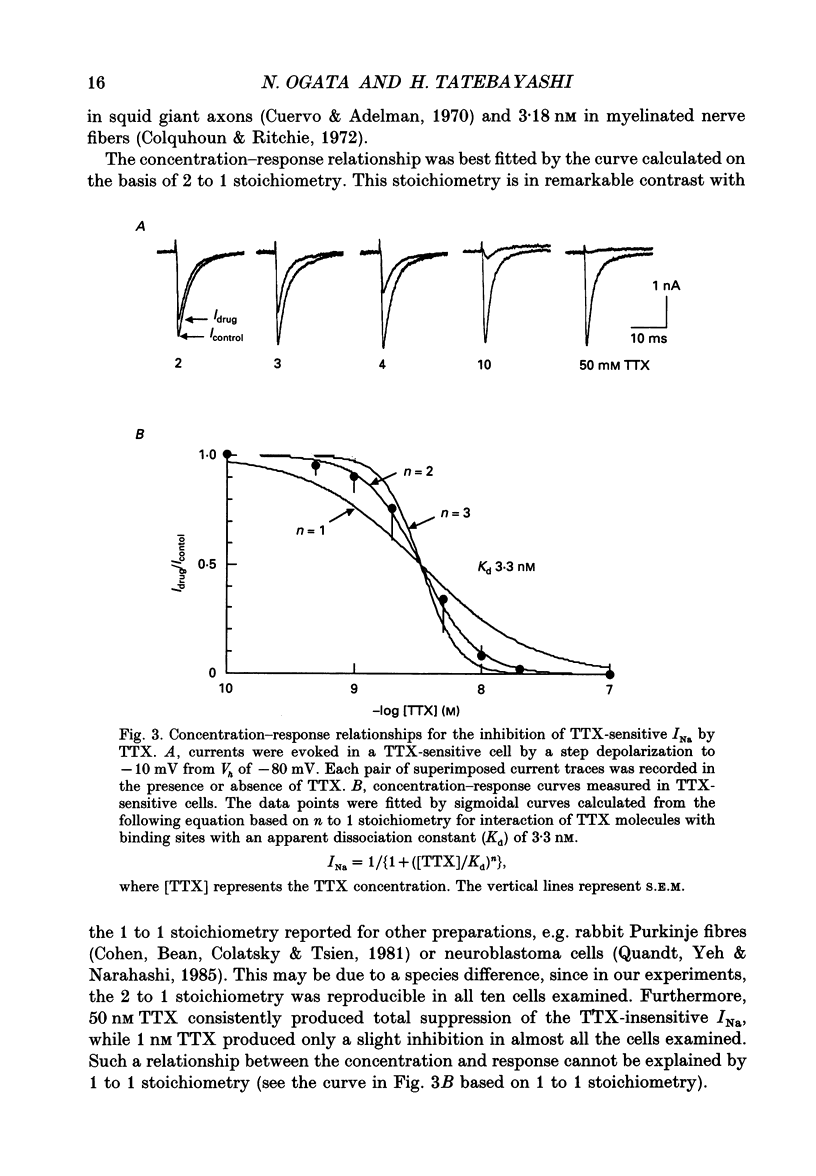
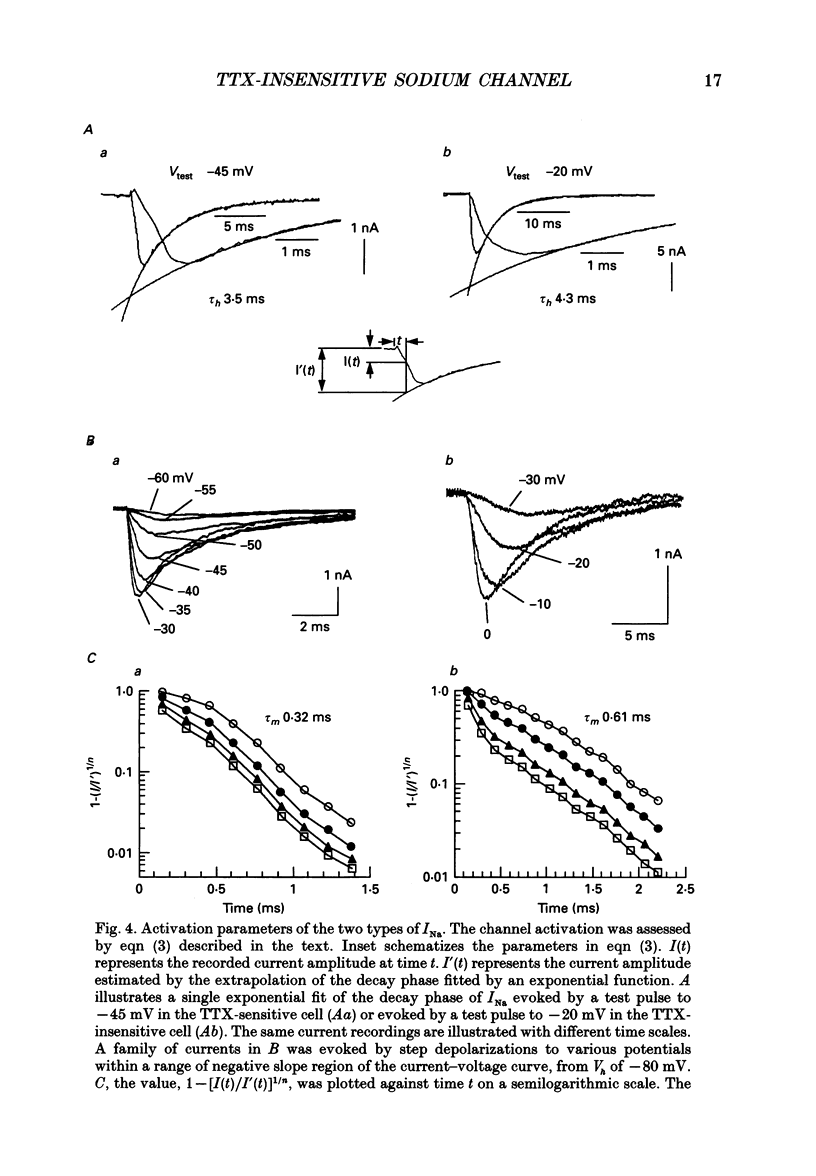
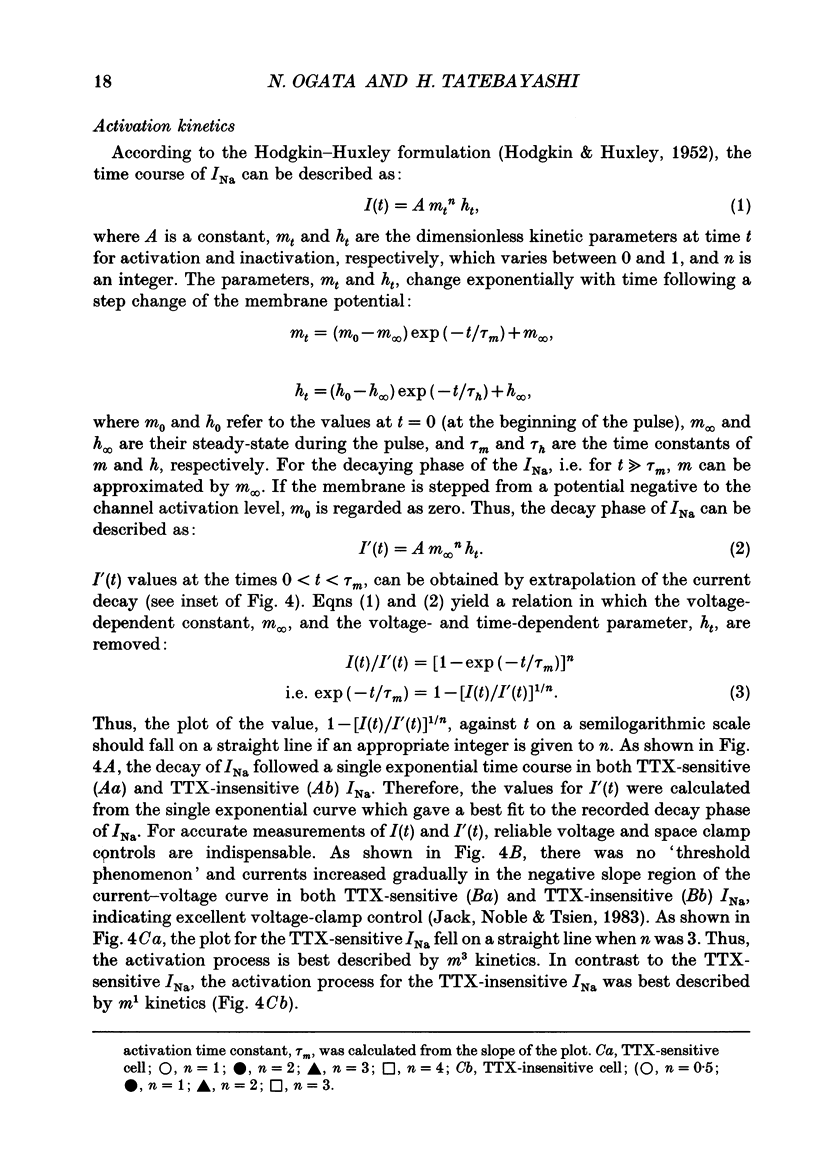
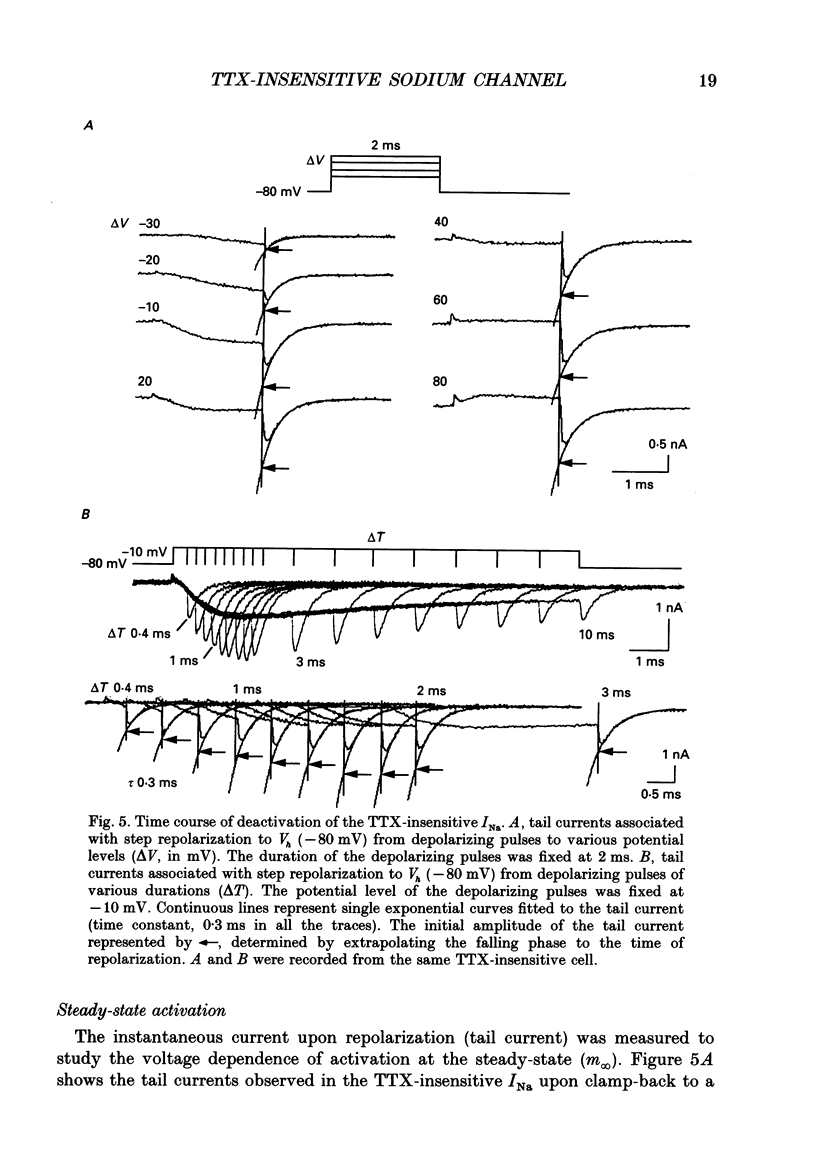
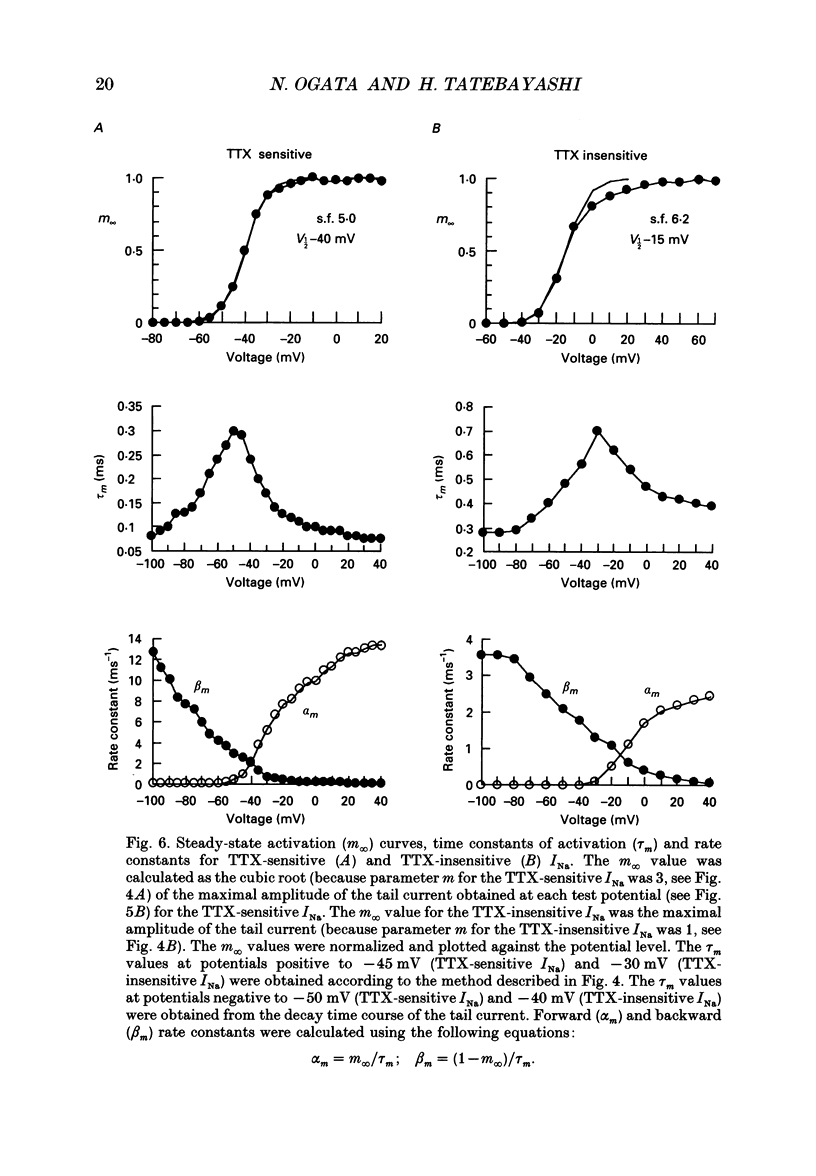
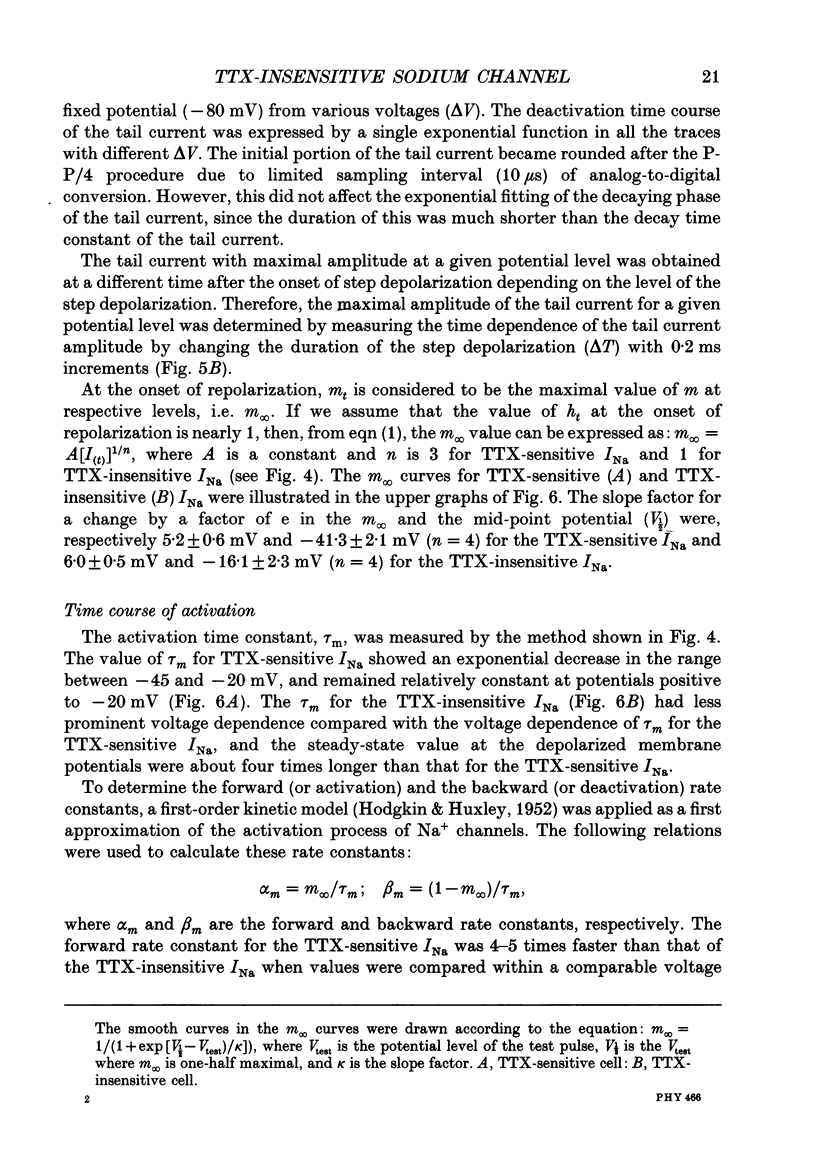
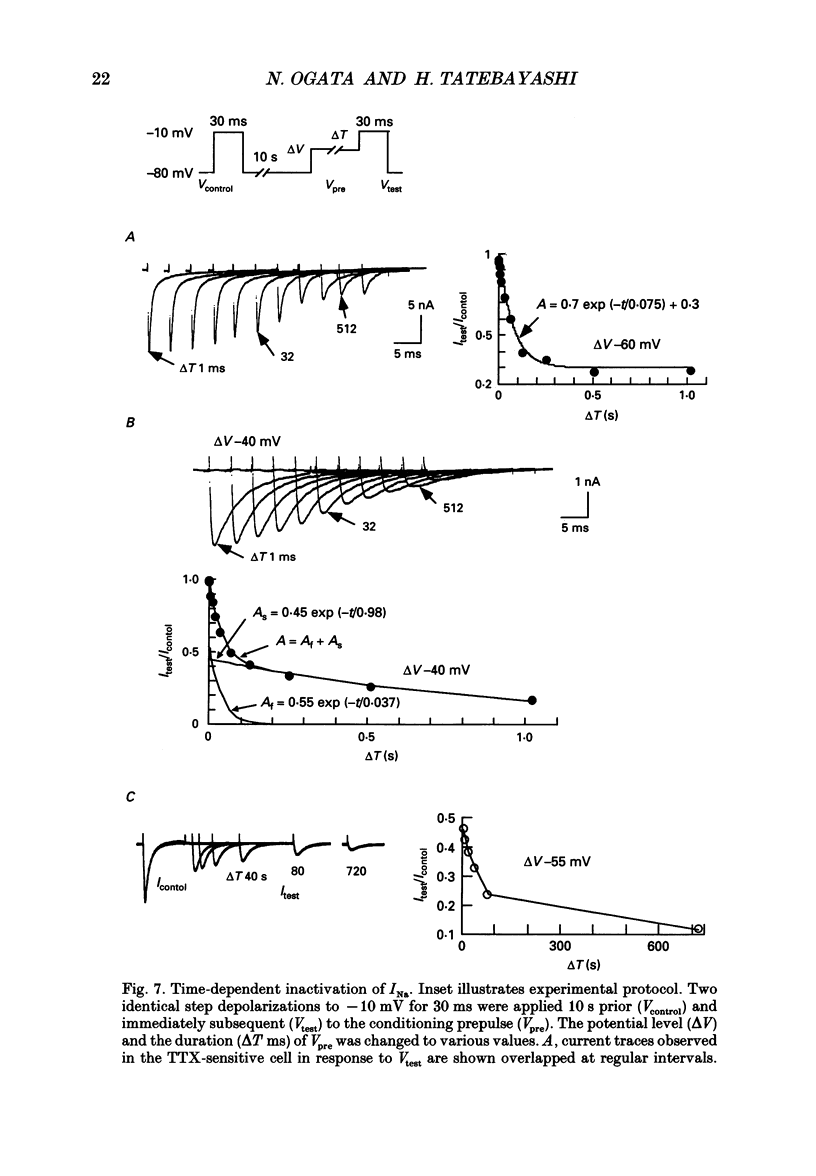
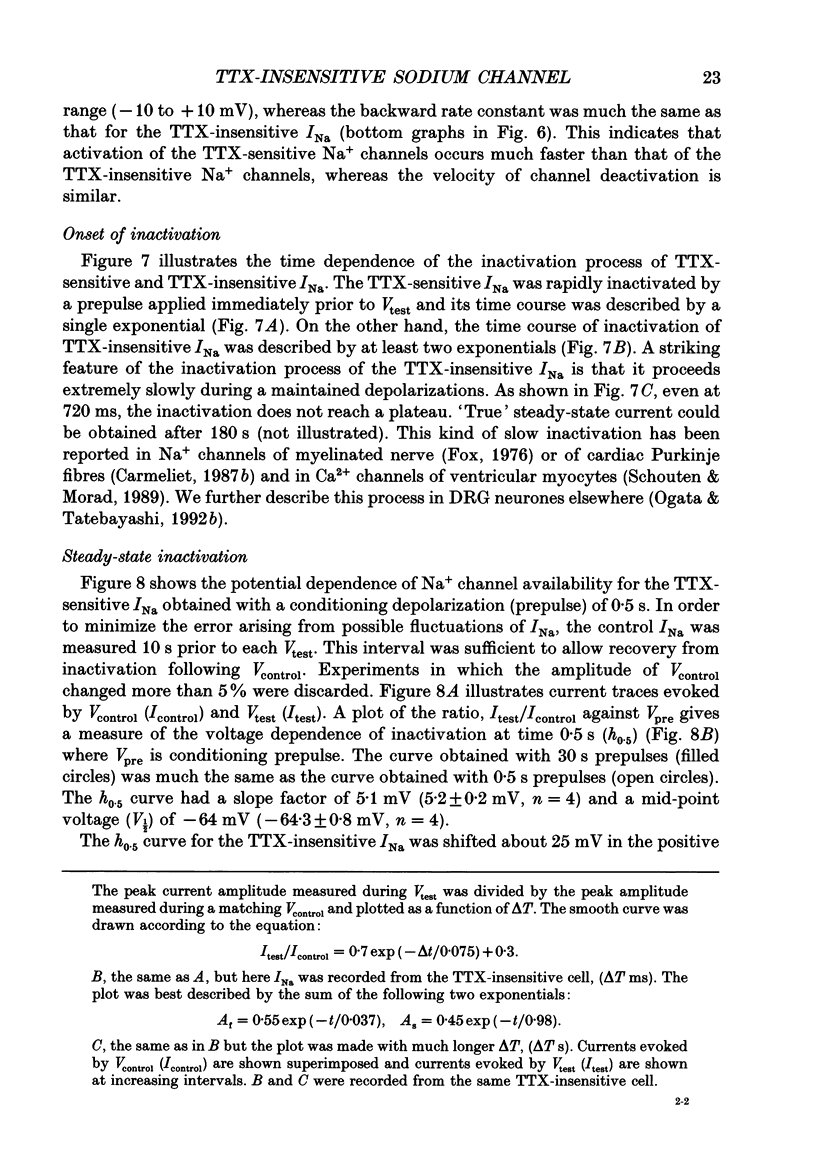
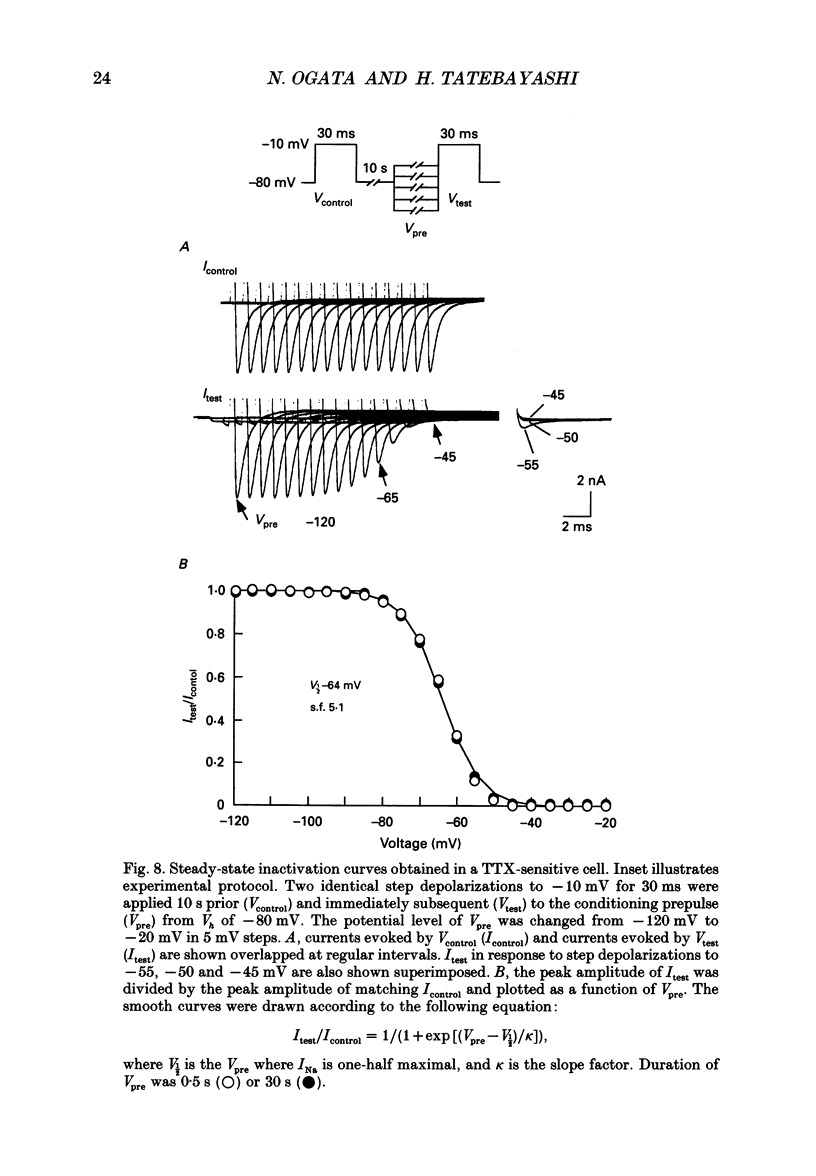
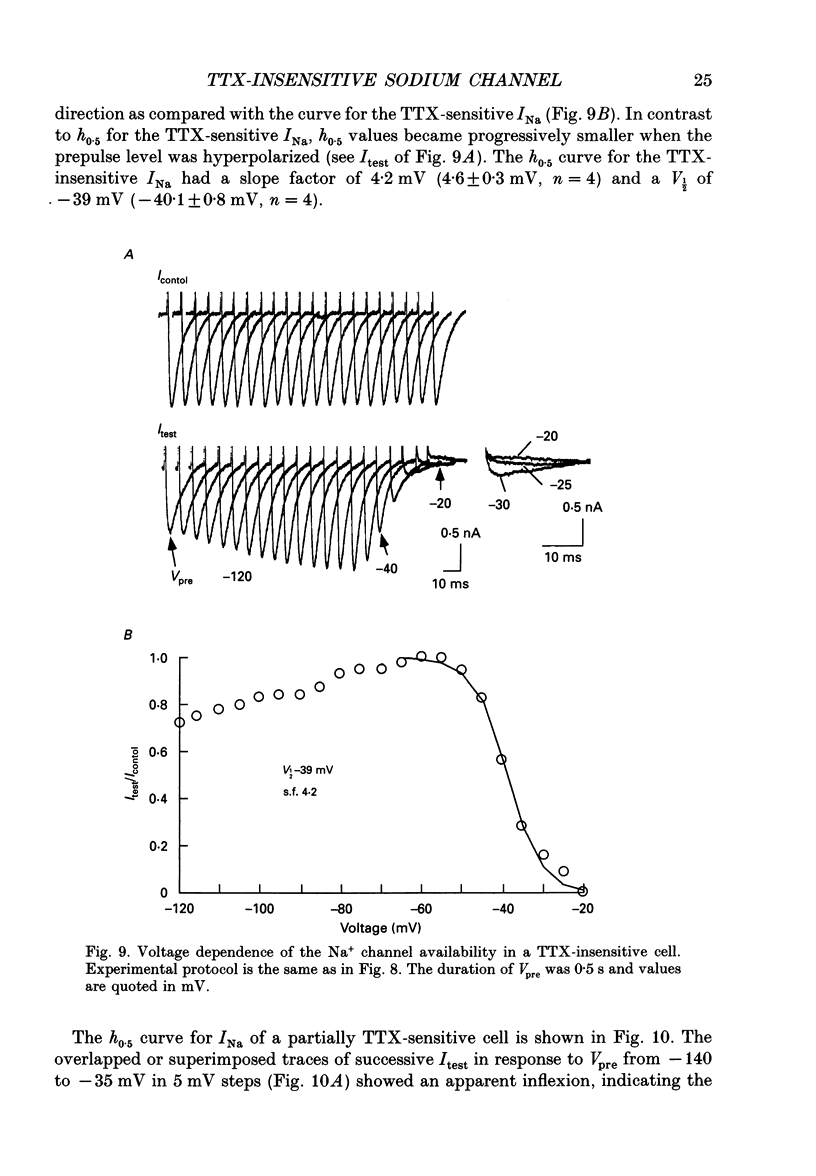
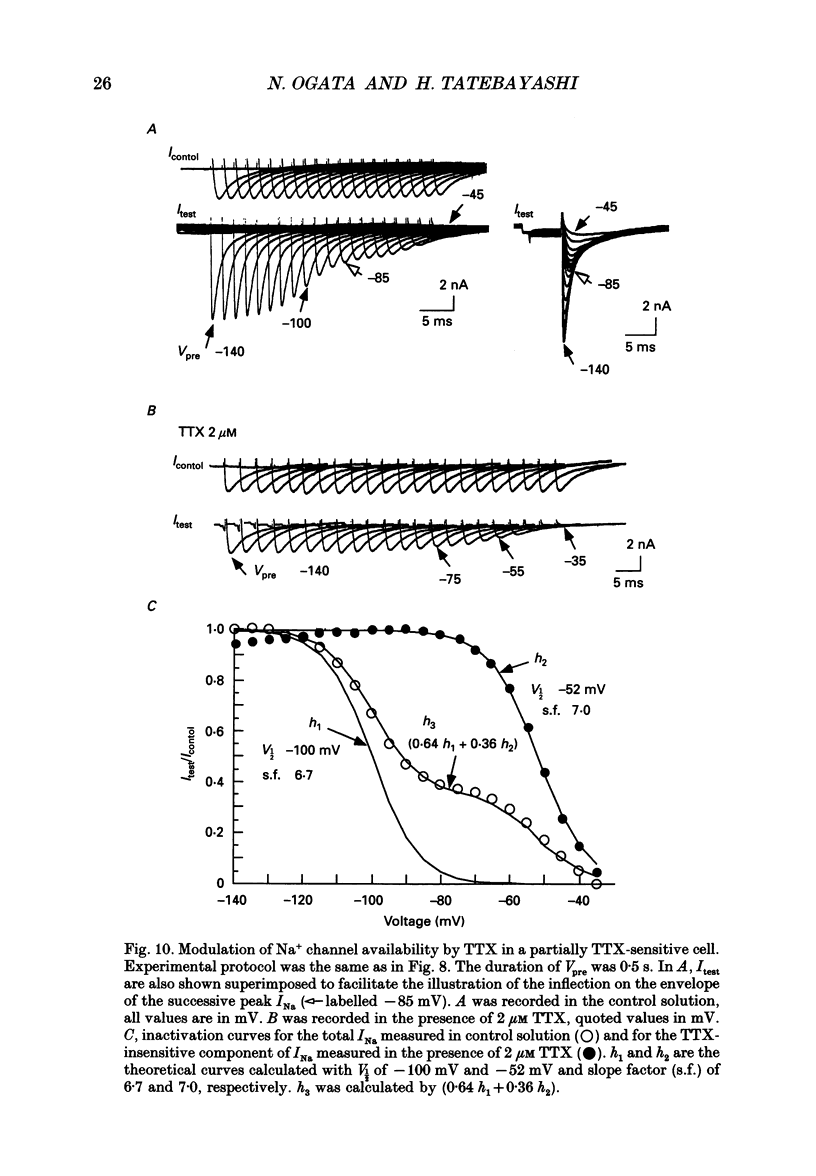
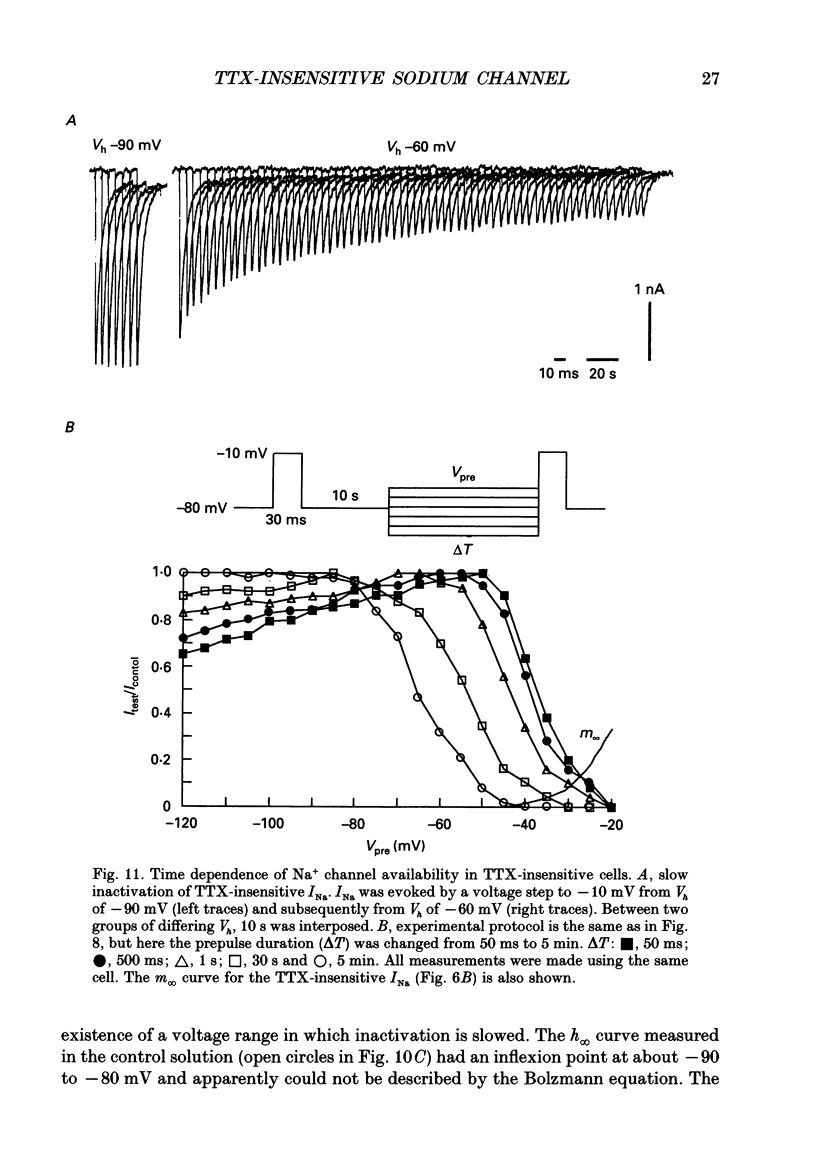
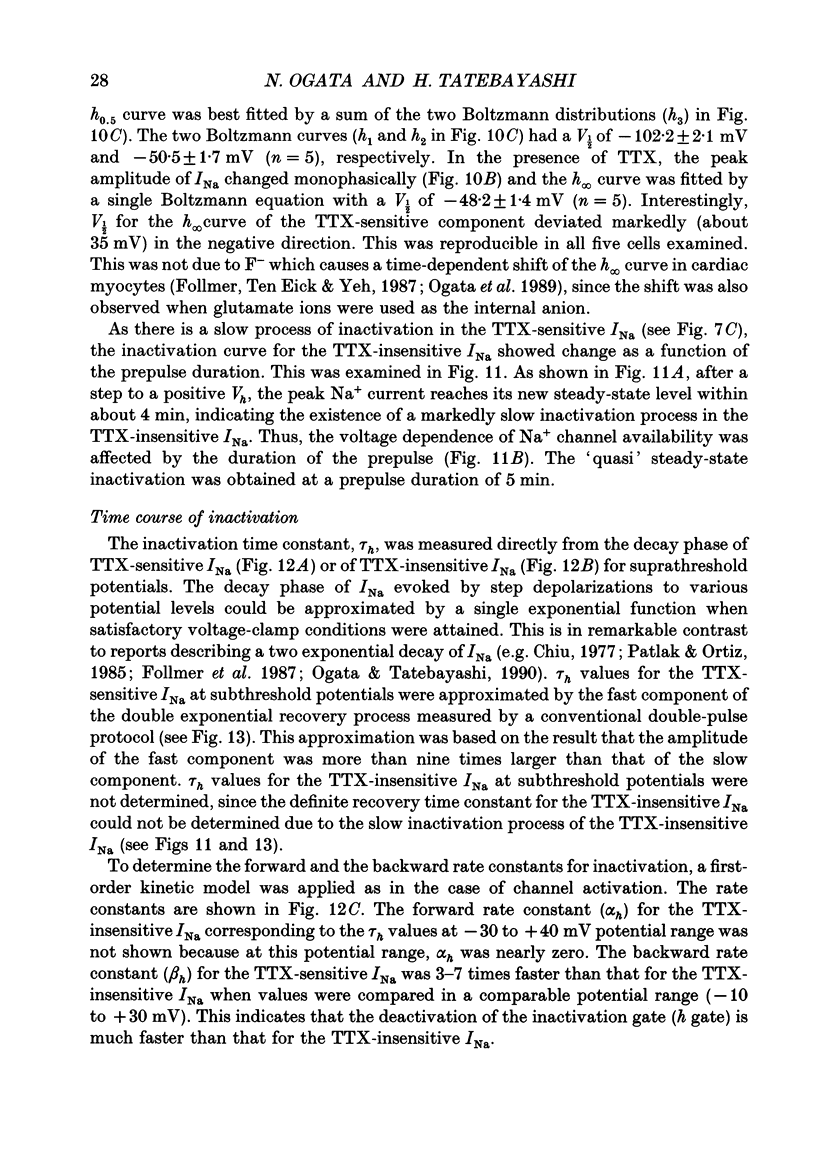
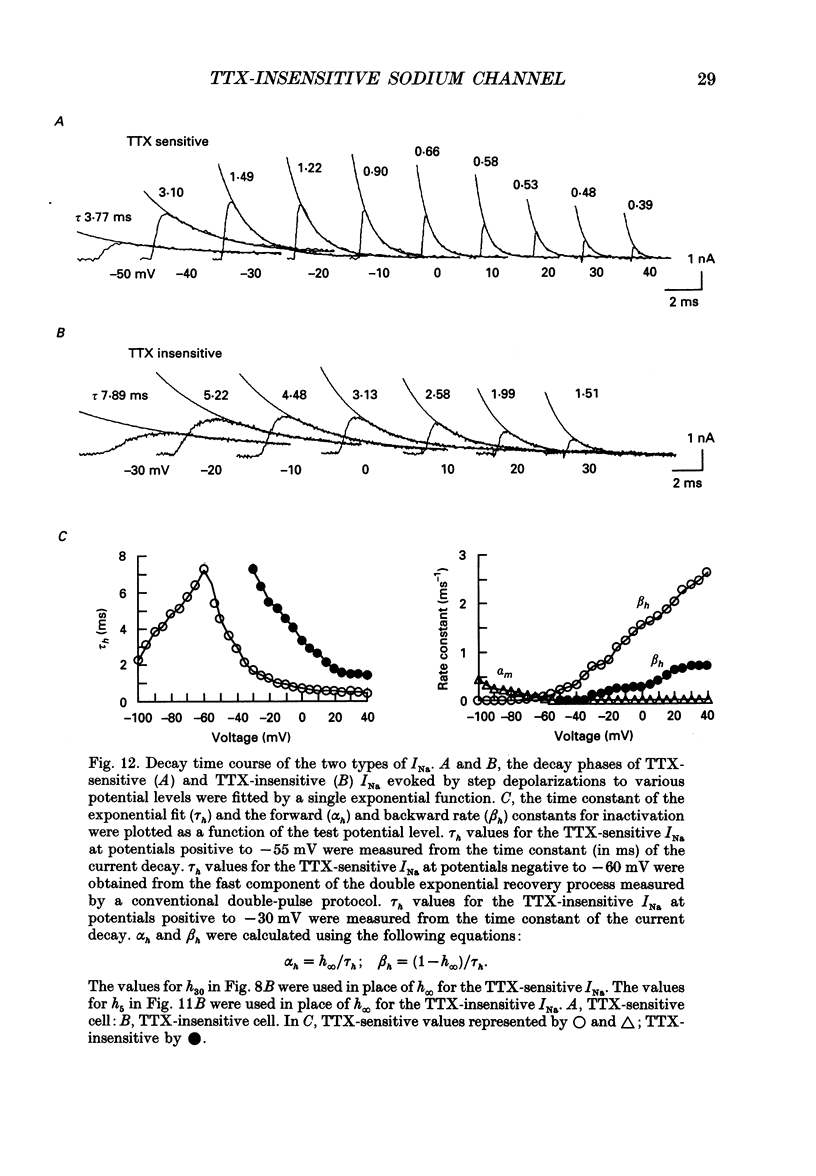
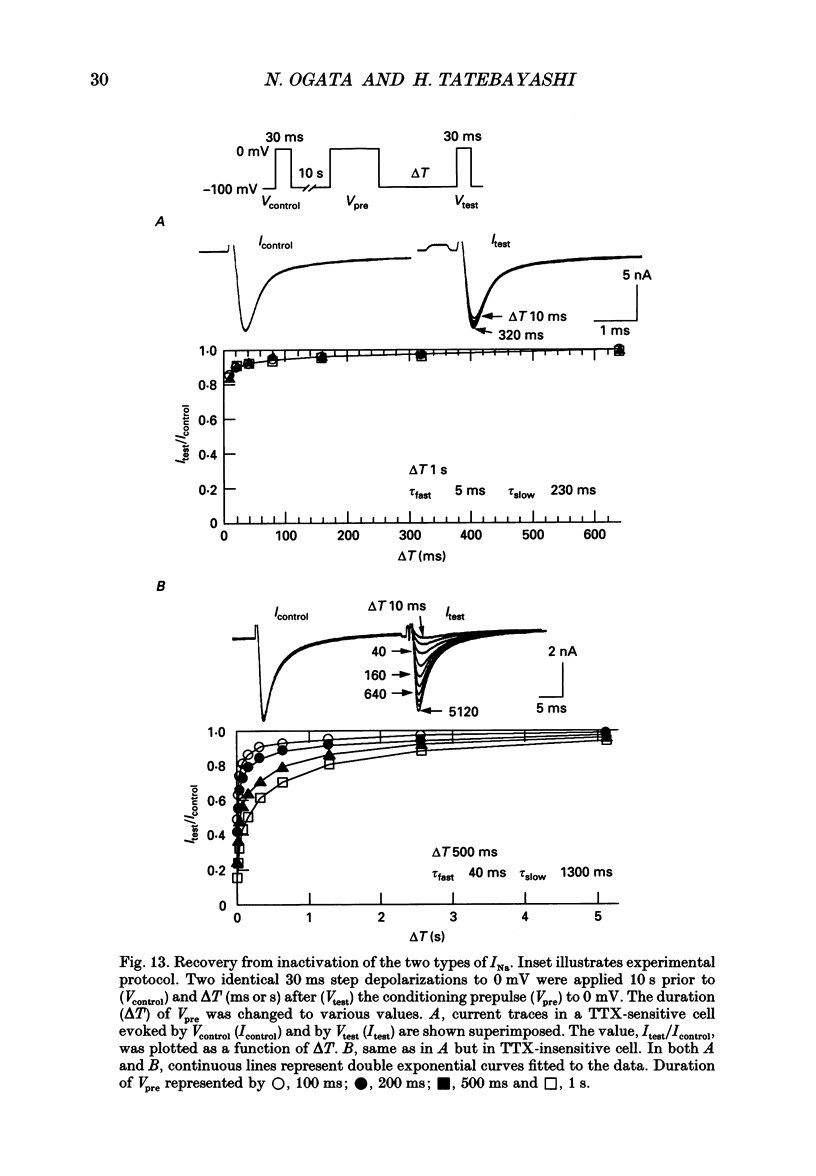
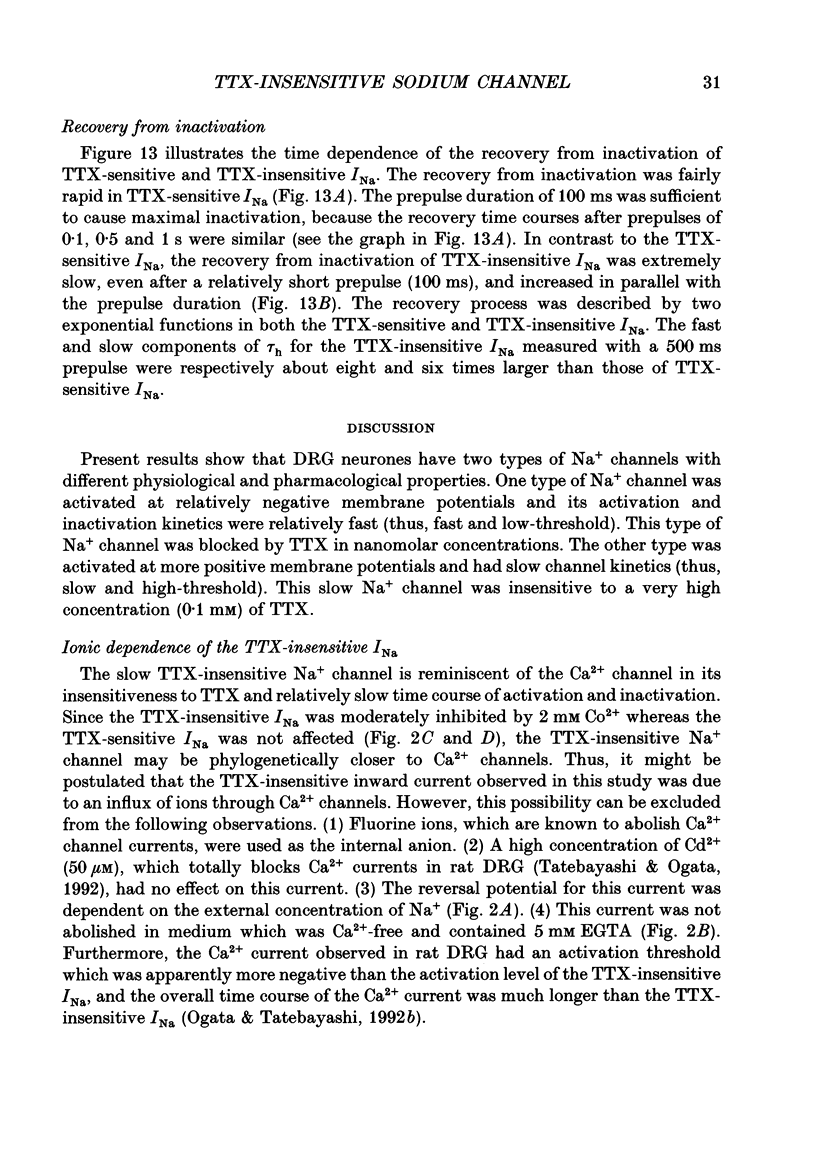
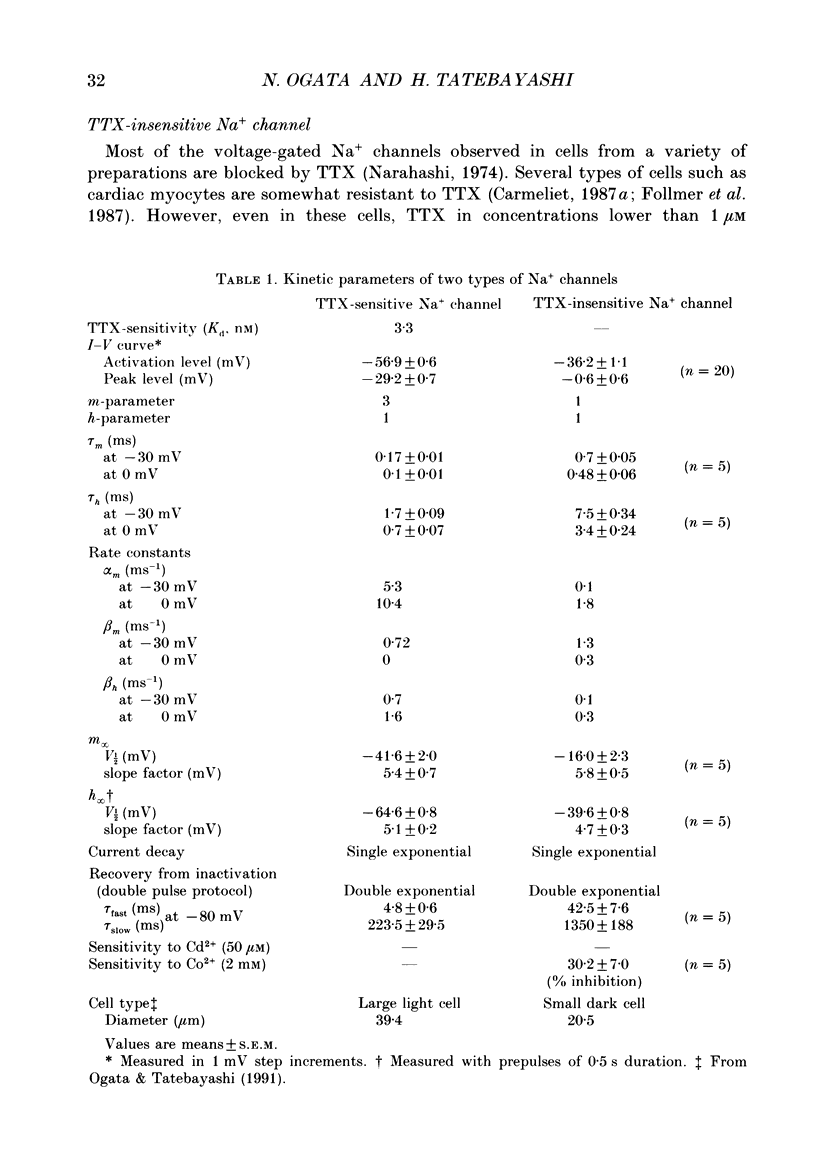
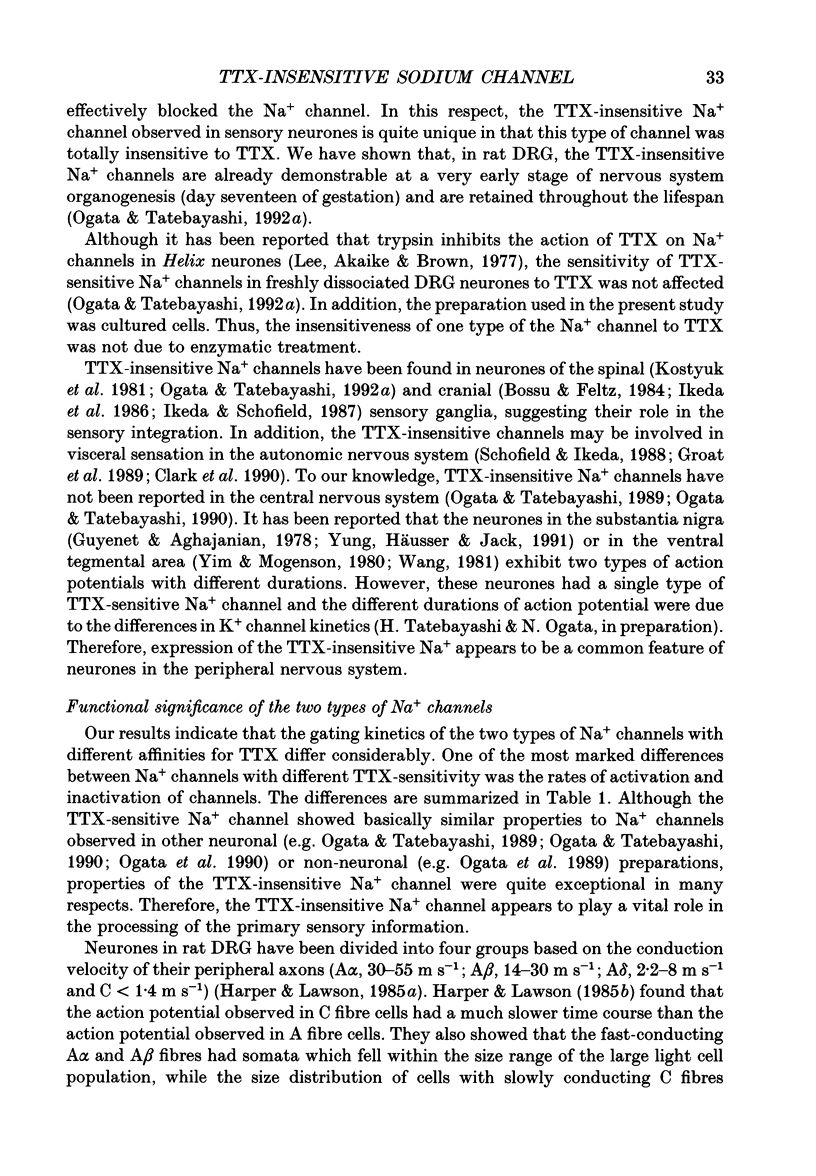
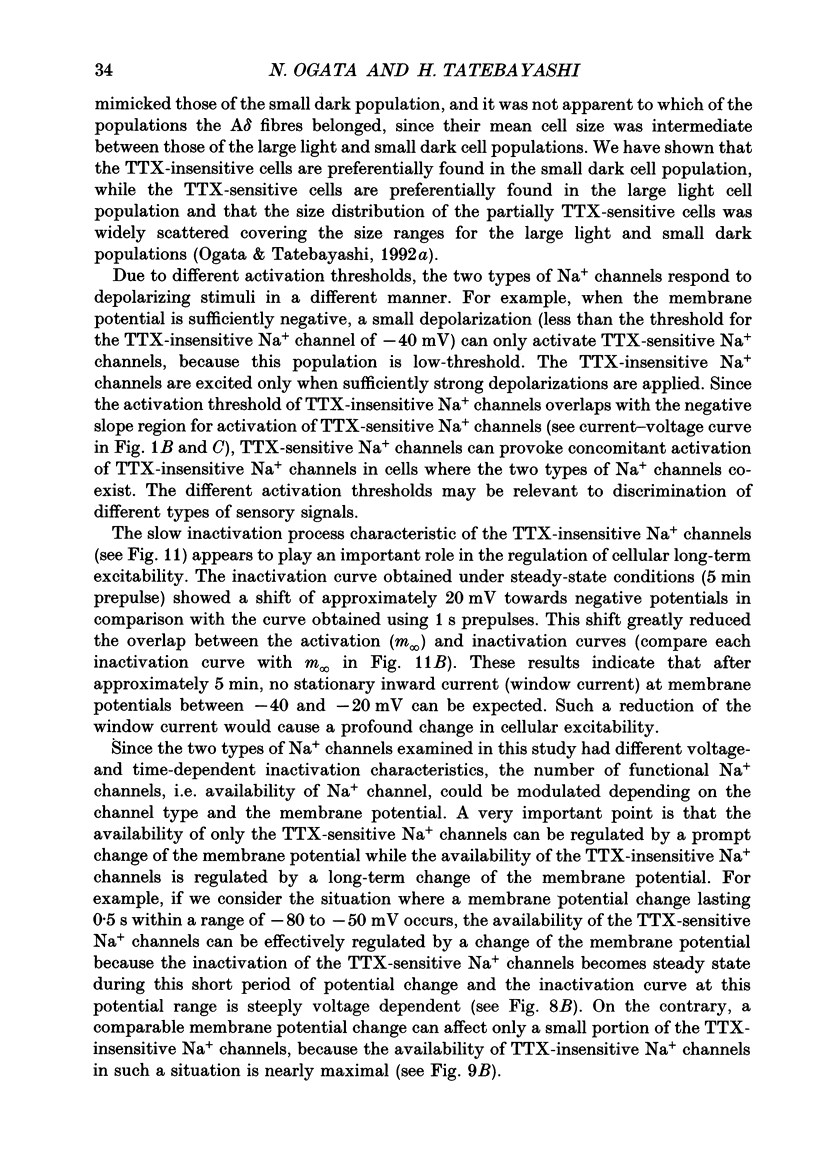
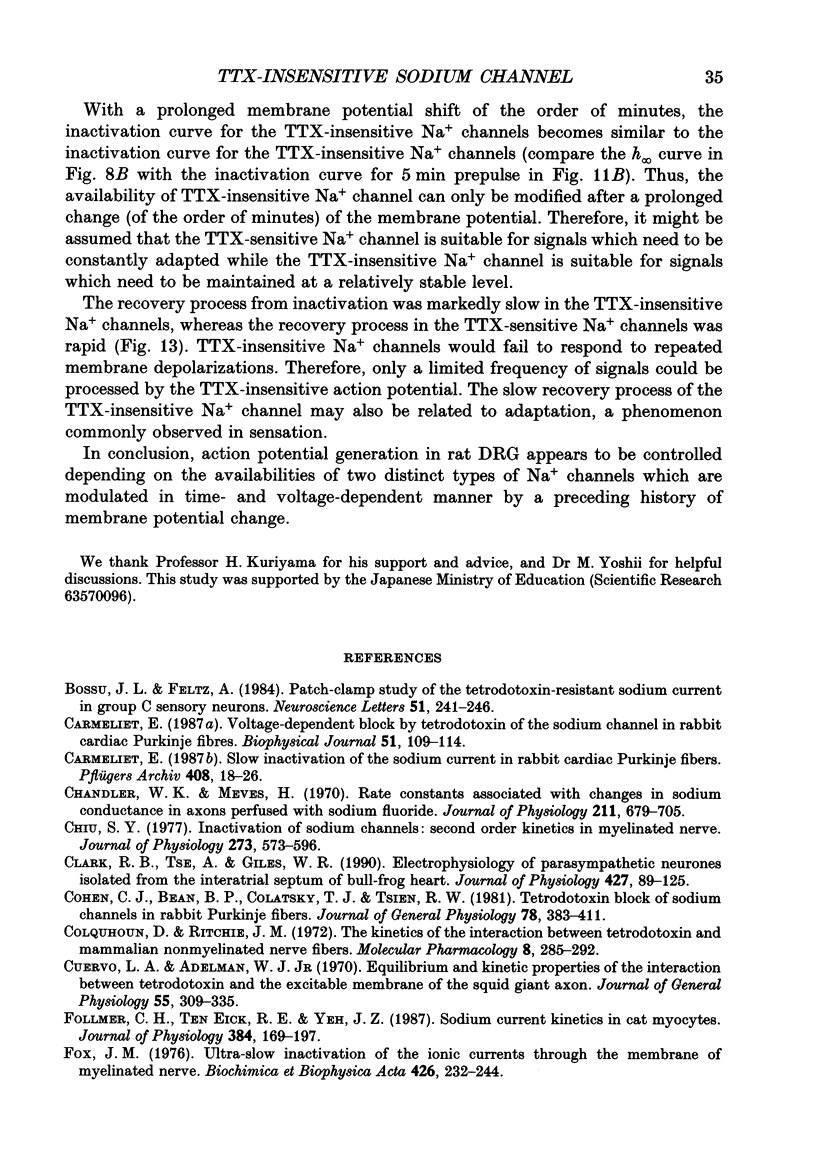
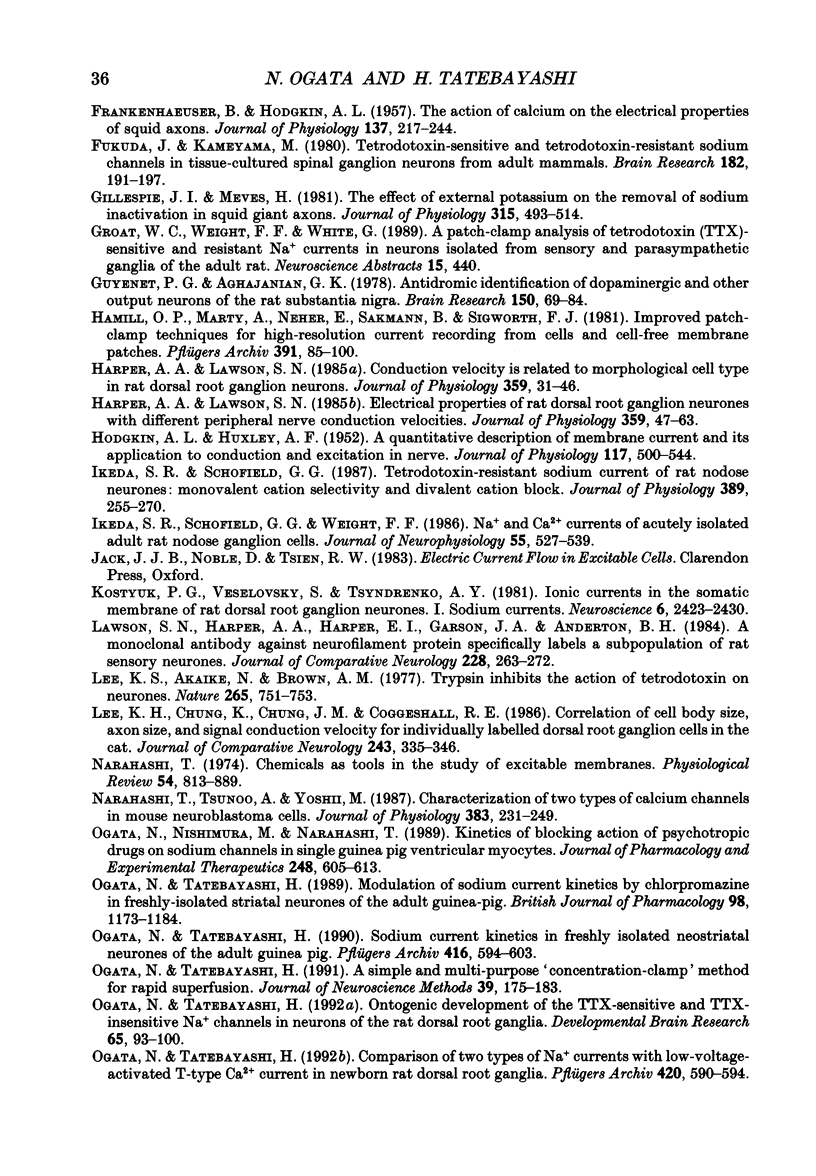
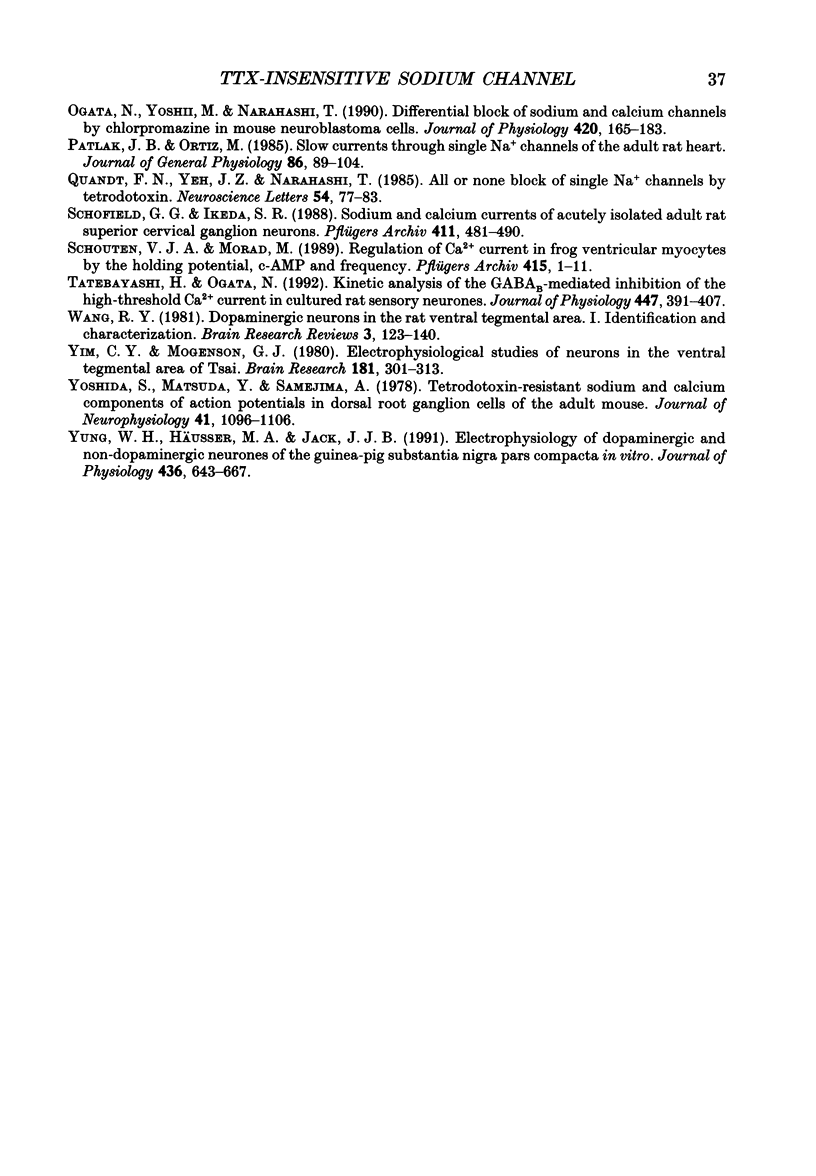
Selected References
These references are in PubMed. This may not be the complete list of references from this article.
- Bossu J. L., Feltz A. Patch-clamp study of the tetrodotoxin-resistant sodium current in group C sensory neurones. Neurosci Lett. 1984 Oct 12;51(2):241–246. doi: 10.1016/0304-3940(84)90558-5. [DOI] [PubMed] [Google Scholar]
- Carmeliet E. Slow inactivation of the sodium current in rabbit cardiac Purkinje fibres. Pflugers Arch. 1987 Jan;408(1):18–26. doi: 10.1007/BF00581835. [DOI] [PubMed] [Google Scholar]
- Carmeliet E. Voltage-dependent block by tetrodotoxin of the sodium channel in rabbit cardiac Purkinje fibers. Biophys J. 1987 Jan;51(1):109–114. doi: 10.1016/S0006-3495(87)83315-5. [DOI] [PMC free article] [PubMed] [Google Scholar]
- Chandler W. K., Meves H. Rate constants associated with changes in sodium conductance in axons perfused with sodium fluoride. J Physiol. 1970 Dec;211(3):679–705. doi: 10.1113/jphysiol.1970.sp009299. [DOI] [PMC free article] [PubMed] [Google Scholar]
- Chiu S. Y. Inactivation of sodium channels: second order kinetics in myelinated nerve. J Physiol. 1977 Dec;273(3):573–596. doi: 10.1113/jphysiol.1977.sp012111. [DOI] [PMC free article] [PubMed] [Google Scholar]
- Clark R. B., Tse A., Giles W. R. Electrophysiology of parasympathetic neurones isolated from the interatrial septum of bull-frog heart. J Physiol. 1990 Aug;427:89–125. doi: 10.1113/jphysiol.1990.sp018163. [DOI] [PMC free article] [PubMed] [Google Scholar]
- Cohen C. J., Bean B. P., Colatsky T. J., Tsien R. W. Tetrodotoxin block of sodium channels in rabbit Purkinje fibers. Interactions between toxin binding and channel gating. J Gen Physiol. 1981 Oct;78(4):383–411. doi: 10.1085/jgp.78.4.383. [DOI] [PMC free article] [PubMed] [Google Scholar]
- Colquhoun D., Ritchie J. M. The kinetics of the interaction between tetrodotoxin and mammalian nonmyelinated nerve fibers. Mol Pharmacol. 1972 May;8(3):285–292. [PubMed] [Google Scholar]
- Cuervo L. A., Adelman W. J., Jr Equilibrium and kinetic properties of the interaction between tetrodotoxin and the excitable membrane of the squid giant axon. J Gen Physiol. 1970 Mar;55(3):309–335. doi: 10.1085/jgp.55.3.309. [DOI] [PMC free article] [PubMed] [Google Scholar]
- FRANKENHAEUSER B., HODGKIN A. L. The action of calcium on the electrical properties of squid axons. J Physiol. 1957 Jul 11;137(2):218–244. doi: 10.1113/jphysiol.1957.sp005808. [DOI] [PMC free article] [PubMed] [Google Scholar]
- Follmer C. H., ten Eick R. E., Yeh J. Z. Sodium current kinetics in cat atrial myocytes. J Physiol. 1987 Mar;384:169–197. doi: 10.1113/jphysiol.1987.sp016449. [DOI] [PMC free article] [PubMed] [Google Scholar]
- Fox J. M. Ultra-slow inactivation of the ionic currents through the membrane of myelinated nerve. Biochim Biophys Acta. 1976 Mar 5;426(2):232–244. doi: 10.1016/0005-2736(76)90334-5. [DOI] [PubMed] [Google Scholar]
- Fukuda J., Kameyama M. Tetrodotoxin-sensitive and tetrodotoxin-resistant sodium channels in tissue-cultured spinal ganglion neurons from adult mammals. Brain Res. 1980 Jan 20;182(1):191–197. doi: 10.1016/0006-8993(80)90844-6. [DOI] [PubMed] [Google Scholar]
- Gillespie J. I., Meves H. The effect of external potassium on the removal of sodium inactivation in squid giant axons. J Physiol. 1981 Jun;315:493–514. doi: 10.1113/jphysiol.1981.sp013760. [DOI] [PMC free article] [PubMed] [Google Scholar]
- Guyenet P. G., Aghajanian G. K. Antidromic identification of dopaminergic and other output neurons of the rat substantia nigra. Brain Res. 1978 Jul 7;150(1):69–84. doi: 10.1016/0006-8993(78)90654-6. [DOI] [PubMed] [Google Scholar]
- HODGKIN A. L., HUXLEY A. F. A quantitative description of membrane current and its application to conduction and excitation in nerve. J Physiol. 1952 Aug;117(4):500–544. doi: 10.1113/jphysiol.1952.sp004764. [DOI] [PMC free article] [PubMed] [Google Scholar]
- Hamill O. P., Marty A., Neher E., Sakmann B., Sigworth F. J. Improved patch-clamp techniques for high-resolution current recording from cells and cell-free membrane patches. Pflugers Arch. 1981 Aug;391(2):85–100. doi: 10.1007/BF00656997. [DOI] [PubMed] [Google Scholar]
- Harper A. A., Lawson S. N. Conduction velocity is related to morphological cell type in rat dorsal root ganglion neurones. J Physiol. 1985 Feb;359:31–46. doi: 10.1113/jphysiol.1985.sp015573. [DOI] [PMC free article] [PubMed] [Google Scholar]
- Harper A. A., Lawson S. N. Electrical properties of rat dorsal root ganglion neurones with different peripheral nerve conduction velocities. J Physiol. 1985 Feb;359:47–63. doi: 10.1113/jphysiol.1985.sp015574. [DOI] [PMC free article] [PubMed] [Google Scholar]
- Ikeda S. R., Schofield G. G. Tetrodotoxin-resistant sodium current of rat nodose neurones: monovalent cation selectivity and divalent cation block. J Physiol. 1987 Aug;389:255–270. doi: 10.1113/jphysiol.1987.sp016656. [DOI] [PMC free article] [PubMed] [Google Scholar]
- Ikeda S. R., Schofield G. G., Weight F. F. Na+ and Ca2+ currents of acutely isolated adult rat nodose ganglion cells. J Neurophysiol. 1986 Mar;55(3):527–539. doi: 10.1152/jn.1986.55.3.527. [DOI] [PubMed] [Google Scholar]
- Kostyuk P. G., Veselovsky N. S., Tsyndrenko A. Y. Ionic currents in the somatic membrane of rat dorsal root ganglion neurons-I. Sodium currents. Neuroscience. 1981;6(12):2423–2430. doi: 10.1016/0306-4522(81)90088-9. [DOI] [PubMed] [Google Scholar]
- Lawson S. N., Harper A. A., Harper E. I., Garson J. A., Anderton B. H. A monoclonal antibody against neurofilament protein specifically labels a subpopulation of rat sensory neurones. J Comp Neurol. 1984 Sep 10;228(2):263–272. doi: 10.1002/cne.902280211. [DOI] [PubMed] [Google Scholar]
- Lee K. H., Chung K., Chung J. M., Coggeshall R. E. Correlation of cell body size, axon size, and signal conduction velocity for individually labelled dorsal root ganglion cells in the cat. J Comp Neurol. 1986 Jan 15;243(3):335–346. doi: 10.1002/cne.902430305. [DOI] [PubMed] [Google Scholar]
- Lee K. S., Akaike N., Brown A. M. Trypsin inhibits the action of tetrodotoxin on neurones. Nature. 1977 Feb 24;265(5596):751–753. doi: 10.1038/265751a0. [DOI] [PubMed] [Google Scholar]
- Narahashi T. Chemicals as tools in the study of excitable membranes. Physiol Rev. 1974 Oct;54(4):813–889. doi: 10.1152/physrev.1974.54.4.813. [DOI] [PubMed] [Google Scholar]
- Narahashi T., Tsunoo A., Yoshii M. Characterization of two types of calcium channels in mouse neuroblastoma cells. J Physiol. 1987 Feb;383:231–249. doi: 10.1113/jphysiol.1987.sp016406. [DOI] [PMC free article] [PubMed] [Google Scholar]
- Ogata N., Nishimura M., Narahashi T. Kinetics of chlorpromazine block of sodium channels in single guinea pig cardiac myocytes. J Pharmacol Exp Ther. 1989 Feb;248(2):605–613. [PubMed] [Google Scholar]
- Ogata N., Tatebayashi H. A simple and multi-purpose "concentration-clamp" method for rapid superfusion. J Neurosci Methods. 1991 Sep;39(2):175–183. doi: 10.1016/0165-0270(91)90083-c. [DOI] [PubMed] [Google Scholar]
- Ogata N., Tatebayashi H. Comparison of two types of Na+ currents with low-voltage-activated T-type Ca2+ current in newborn rat dorsal root ganglia. Pflugers Arch. 1992 Apr;420(5-6):590–594. doi: 10.1007/BF00374638. [DOI] [PubMed] [Google Scholar]
- Ogata N., Tatebayashi H. Modulation of sodium current kinetics by chlorpromazine in freshly-isolated striatal neurones of the adult guinea-pig. Br J Pharmacol. 1989 Dec;98(4):1173–1184. doi: 10.1111/j.1476-5381.1989.tb12662.x. [DOI] [PMC free article] [PubMed] [Google Scholar]
- Ogata N., Tatebayashi H. Ontogenic development of the TTX-sensitive and TTX-insensitive Na+ channels in neurons of the rat dorsal root ganglia. Brain Res Dev Brain Res. 1992 Jan 17;65(1):93–100. doi: 10.1016/0165-3806(92)90012-l. [DOI] [PubMed] [Google Scholar]
- Ogata N., Tatebayashi H. Sodium current kinetics in freshly isolated neostriatal neurones of the adult guinea pig. Pflugers Arch. 1990 Jul;416(5):594–603. doi: 10.1007/BF00382695. [DOI] [PubMed] [Google Scholar]
- Ogata N., Yoshii M., Narahashi T. Differential block of sodium and calcium channels by chlorpromazine in mouse neuroblastoma cells. J Physiol. 1990 Jan;420:165–183. doi: 10.1113/jphysiol.1990.sp017906. [DOI] [PMC free article] [PubMed] [Google Scholar]
- Patlak J. B., Ortiz M. Slow currents through single sodium channels of the adult rat heart. J Gen Physiol. 1985 Jul;86(1):89–104. doi: 10.1085/jgp.86.1.89. [DOI] [PMC free article] [PubMed] [Google Scholar]
- Quandt F. N., Yeh J. Z., Narahashi T. All or none block of single Na+ channels by tetrodotoxin. Neurosci Lett. 1985 Feb 28;54(1):77–83. doi: 10.1016/s0304-3940(85)80121-x. [DOI] [PubMed] [Google Scholar]
- Schofield G. G., Ikeda S. R. Sodium and calcium currents of acutely isolated adult rat superior cervical ganglion neurons. Pflugers Arch. 1988 May;411(5):481–490. doi: 10.1007/BF00582368. [DOI] [PubMed] [Google Scholar]
- Schouten V. J., Morad M. Regulation of Ca2+ current in frog ventricular myocytes by the holding potential, c-AMP and frequency. Pflugers Arch. 1989 Oct;415(1):1–11. doi: 10.1007/BF00373135. [DOI] [PubMed] [Google Scholar]
- Tatebayashi H., Ogata N. Kinetic analysis of the GABAB-mediated inhibition of the high-threshold Ca2+ current in cultured rat sensory neurones. J Physiol. 1992 Feb;447:391–407. doi: 10.1113/jphysiol.1992.sp019008. [DOI] [PMC free article] [PubMed] [Google Scholar]
- Yim C. Y., Mogenson G. J. Electrophysiological studies of neurons in the ventral tegmental area of Tsai. Brain Res. 1980 Jan 13;181(2):301–313. doi: 10.1016/0006-8993(80)90614-9. [DOI] [PubMed] [Google Scholar]
- Yoshida S., Matsuda Y., Samejima A. Tetrodotoxin-resistant sodium and calcium components of action potentials in dorsal root ganglion cells of the adult mouse. J Neurophysiol. 1978 Sep;41(5):1096–1106. doi: 10.1152/jn.1978.41.5.1096. [DOI] [PubMed] [Google Scholar]
- Yung W. H., Häusser M. A., Jack J. J. Electrophysiology of dopaminergic and non-dopaminergic neurones of the guinea-pig substantia nigra pars compacta in vitro. J Physiol. 1991 May;436:643–667. doi: 10.1113/jphysiol.1991.sp018571. [DOI] [PMC free article] [PubMed] [Google Scholar]