Abstract
1. A voltage-dependent gating mechanism for the fast Cl- channel was developed from the analysis of single-channel current records obtained with the patch clamp technique from primary cultures of rat skeletal muscle. Up to 10(6) open and shut intervals were analysed from each of five different excised patches of membrane containing a single fast Cl- channel. 2. Rate constants for a kinetic scheme with six closed and two open states (scheme I) were estimated at a given voltage by maximum likelihood fitting of open and closed dwell-time distributions obtained at that voltage. This procedure was then repeated for data obtained at each of three to eight different membrane potentials for each channel. 3. Plots of the estimated rate constants against membrane potential typically appeared linear on semilogarithmic co-ordinates, consistent with rate constants that are exponentially dependent on voltage. 4. Regression analysis of these plots yielded two parameters for each rate constant: the value of the rate constant at -50 mV (B) and its voltage sensitivity (A). The dwell-time distributions predicted with these parameters and scheme I gave a good description of the experimental dwell-time distributions at all the studied voltages, lending further support for an exponential dependence of rate constants on membrane potential. 5. Estimates of A and B were also obtained by simultaneously fitting dwell-time distributions obtained at three to eight different voltages, in order to better define these parameters. Predicted dwell-time distributions obtained with these estimates and scheme I could approach the theoretical best description of the data for discrete-state Markov models. 6. Eight to twelve of the fourteen rate constants in scheme I appeared voltage sensitive, with effective gating charges ranging from about -1.5 to +1.0 units of electronic charge. 7. The estimated rate constants and their voltage sensitivities for the five analysed channels were generally similar, but showed some heterogeneity. 8. Gating mechanisms which had fewer kinetic states than scheme I, or equal and opposite effective gating charges over each transition barrier, or four or five identical and independent voltage-dependent subunits, all gave poorer descriptions of the data than scheme I. These simpler mechanisms were also ranked below scheme I by the Schwarz criterion, which applies a heavy penalty for additional free parameters. 9. These findings indicate that the voltage dependence of the fast Cl- channel is consistent with a kinetic scheme with six closed and two open states, in which a majority of transitions among the states are voltage dependent.(ABSTRACT TRUNCATED AT 400 WORDS)
Full text
PDF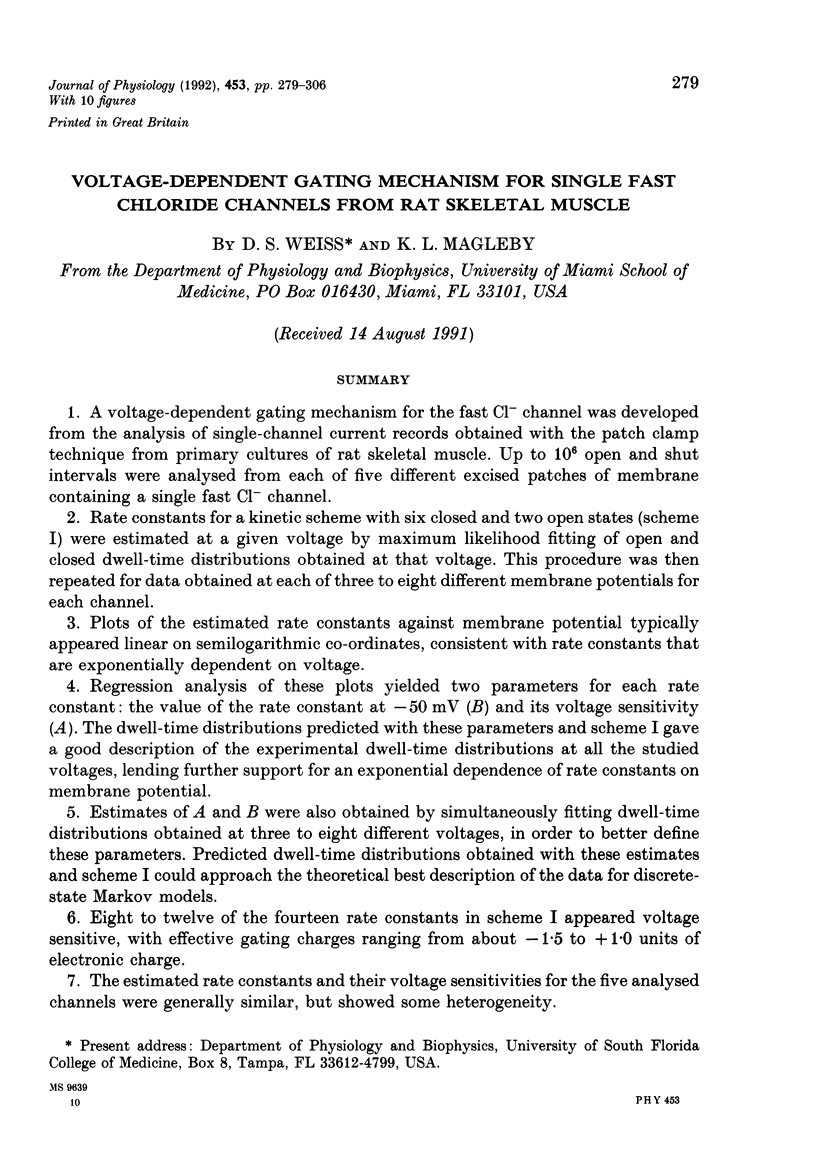
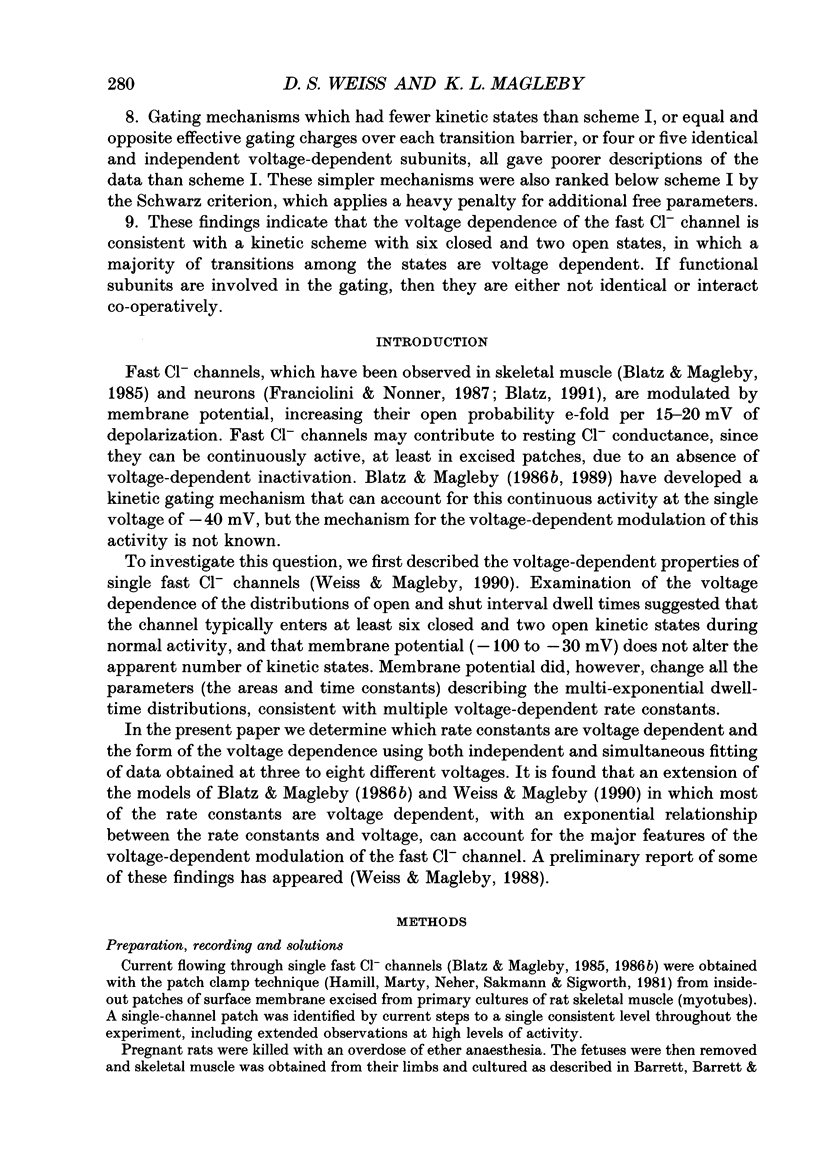
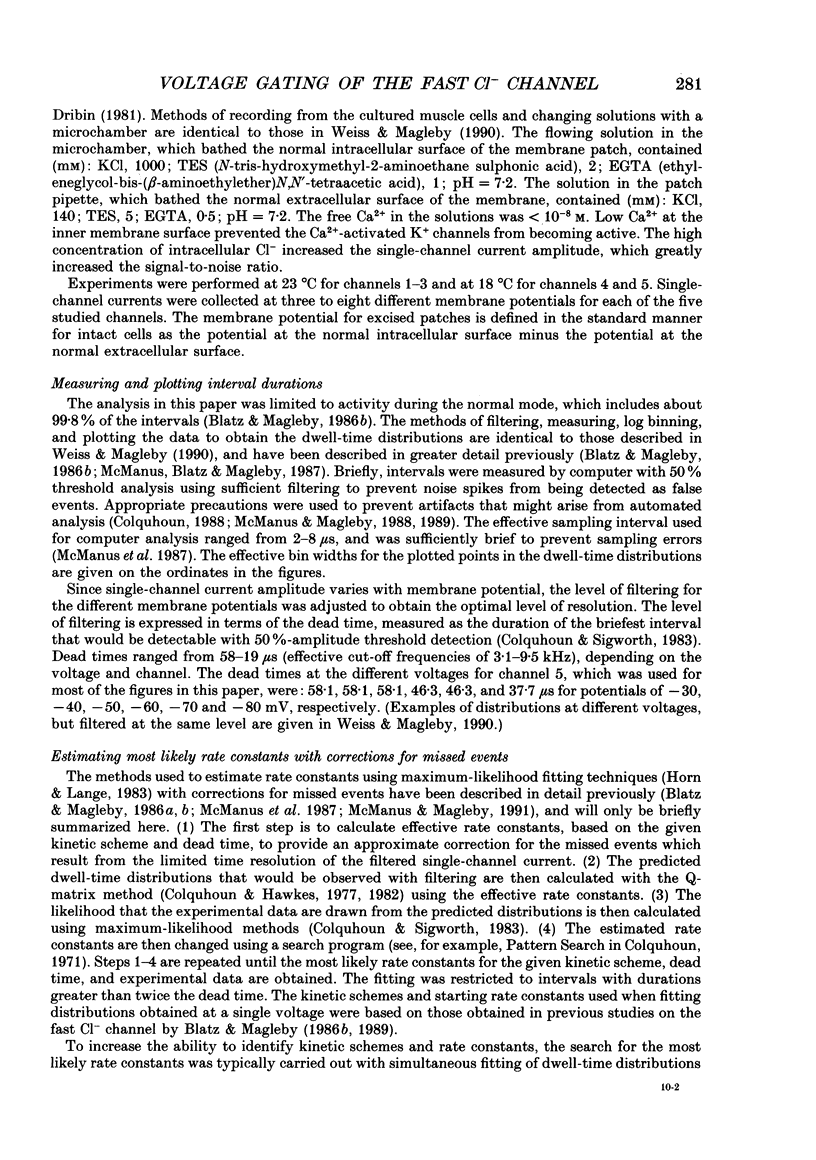
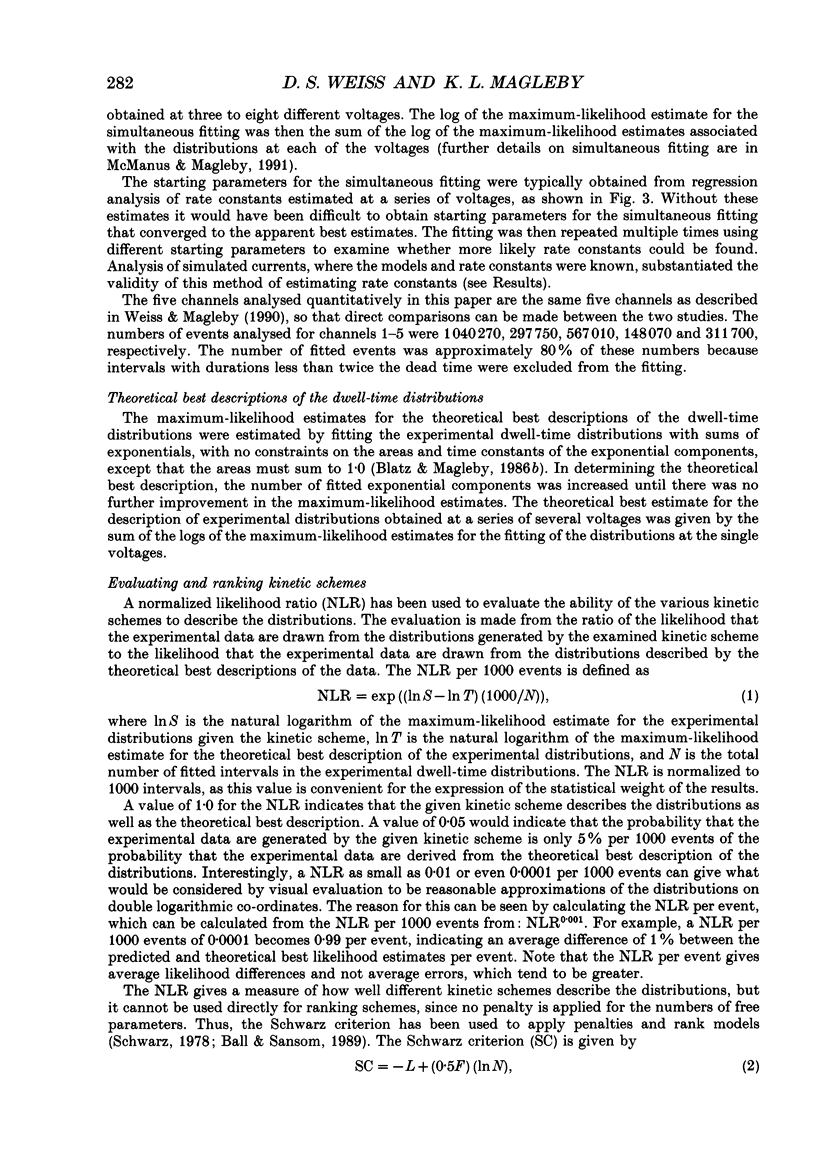
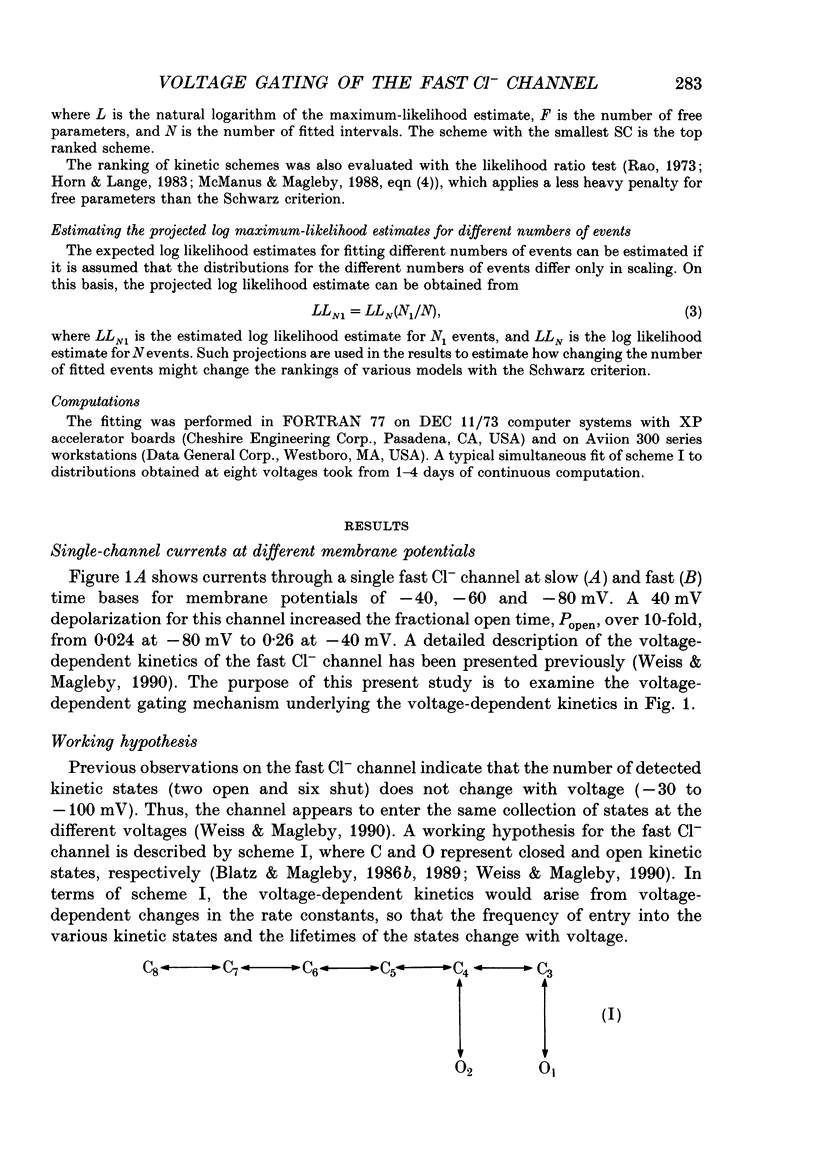
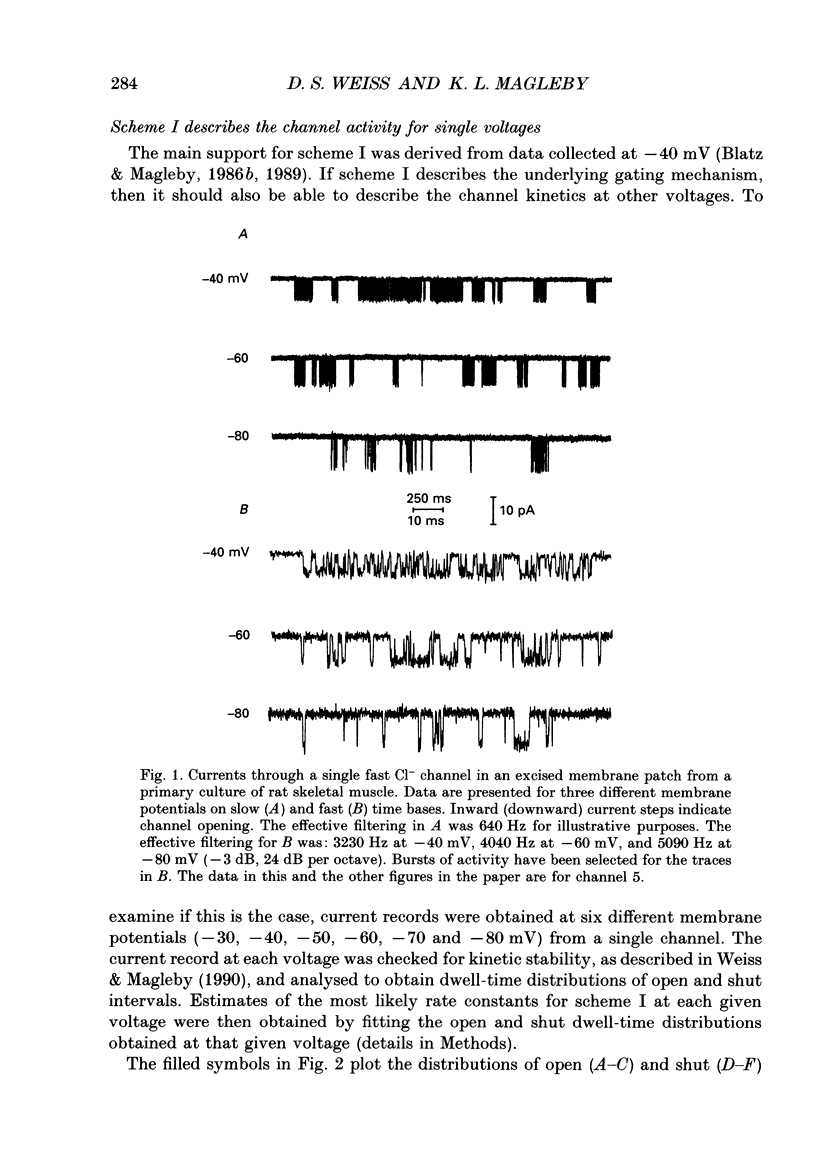
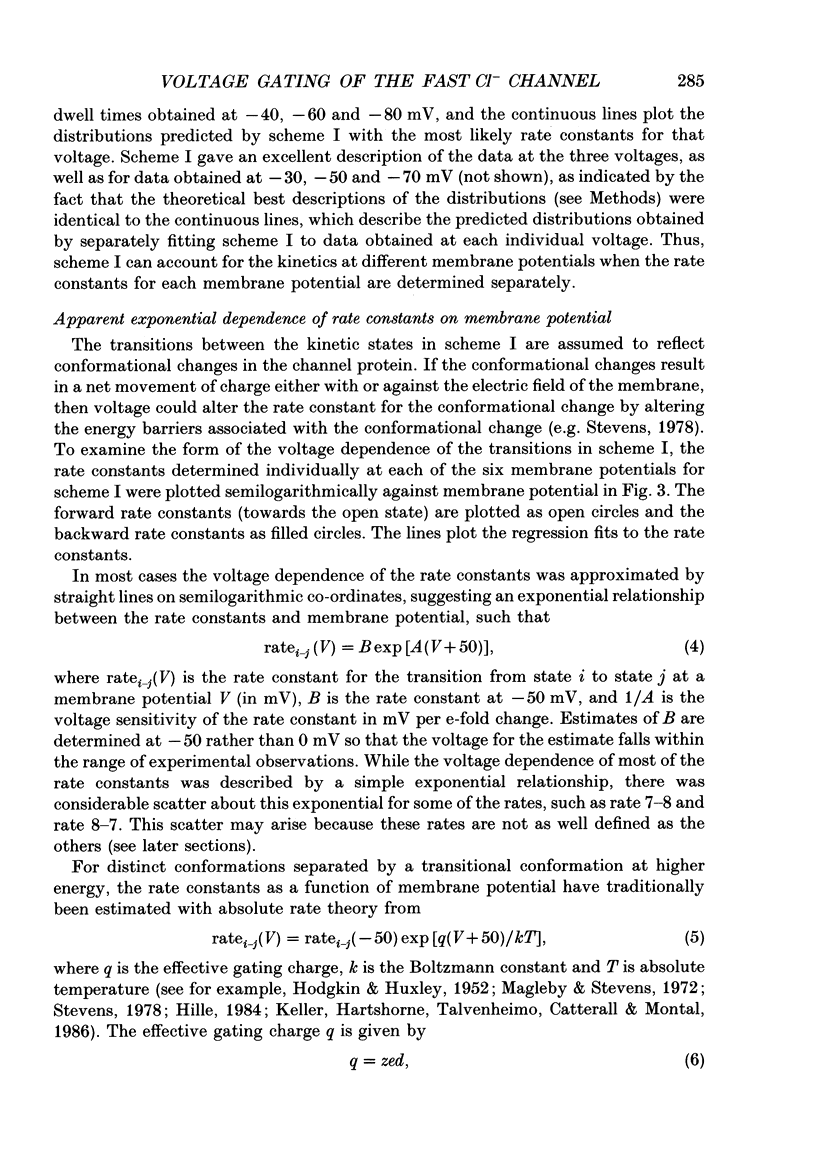
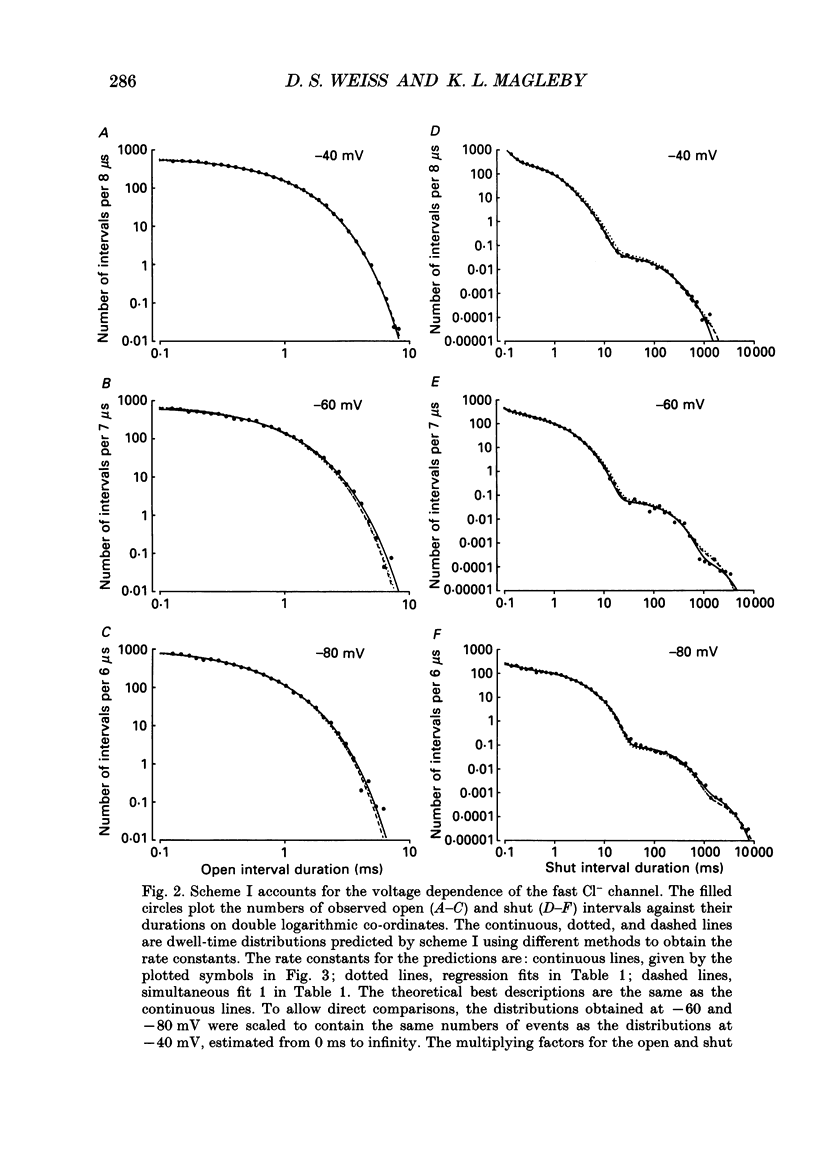
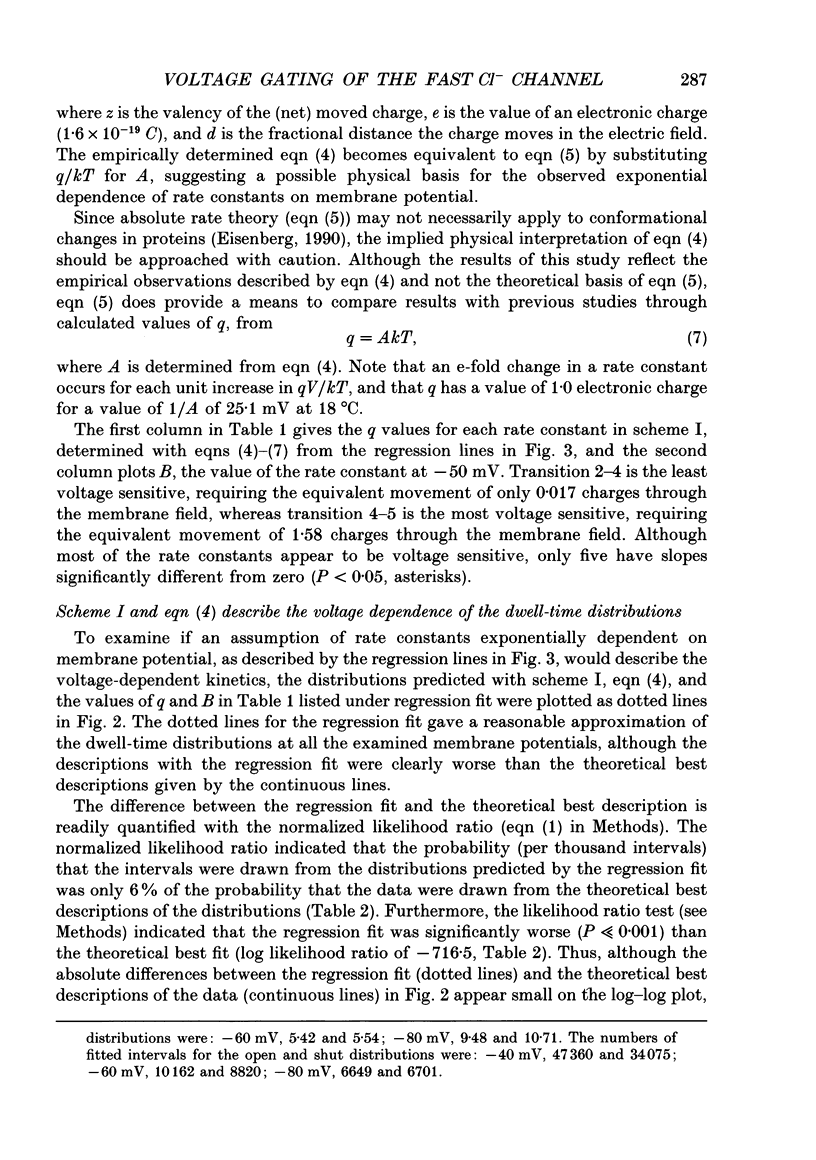
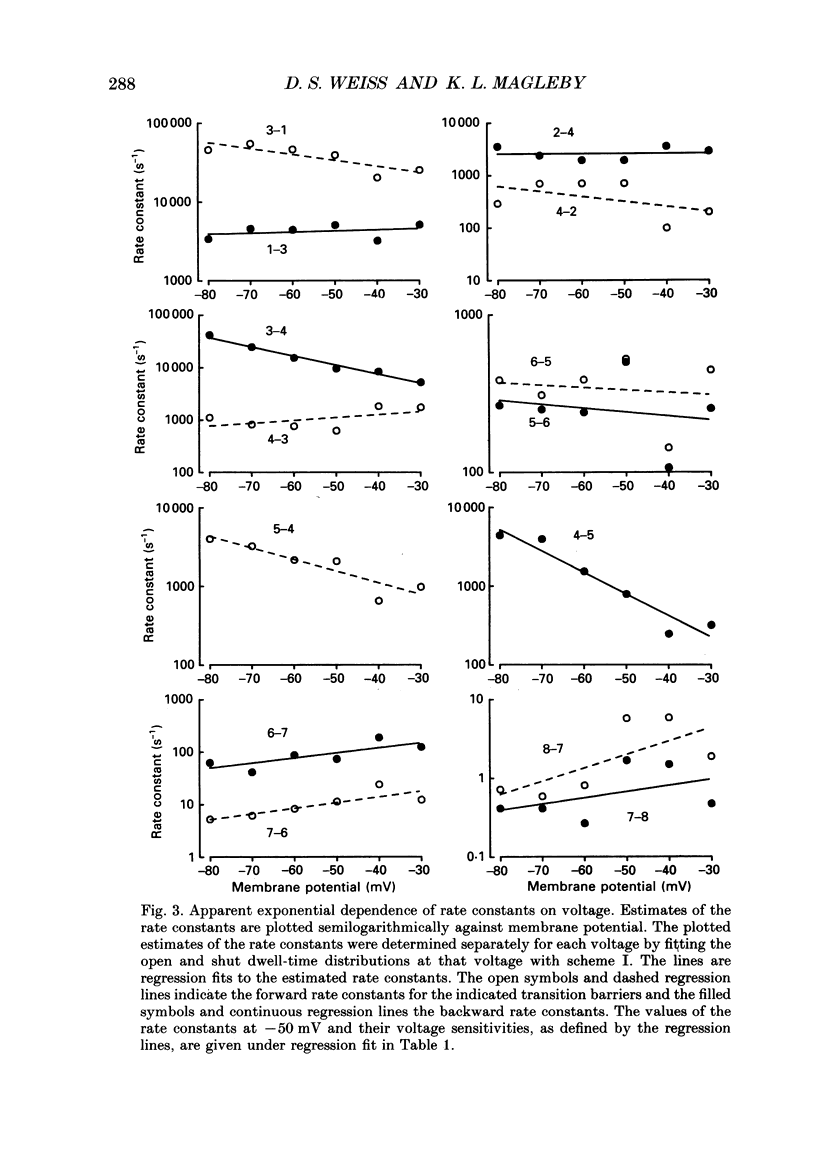
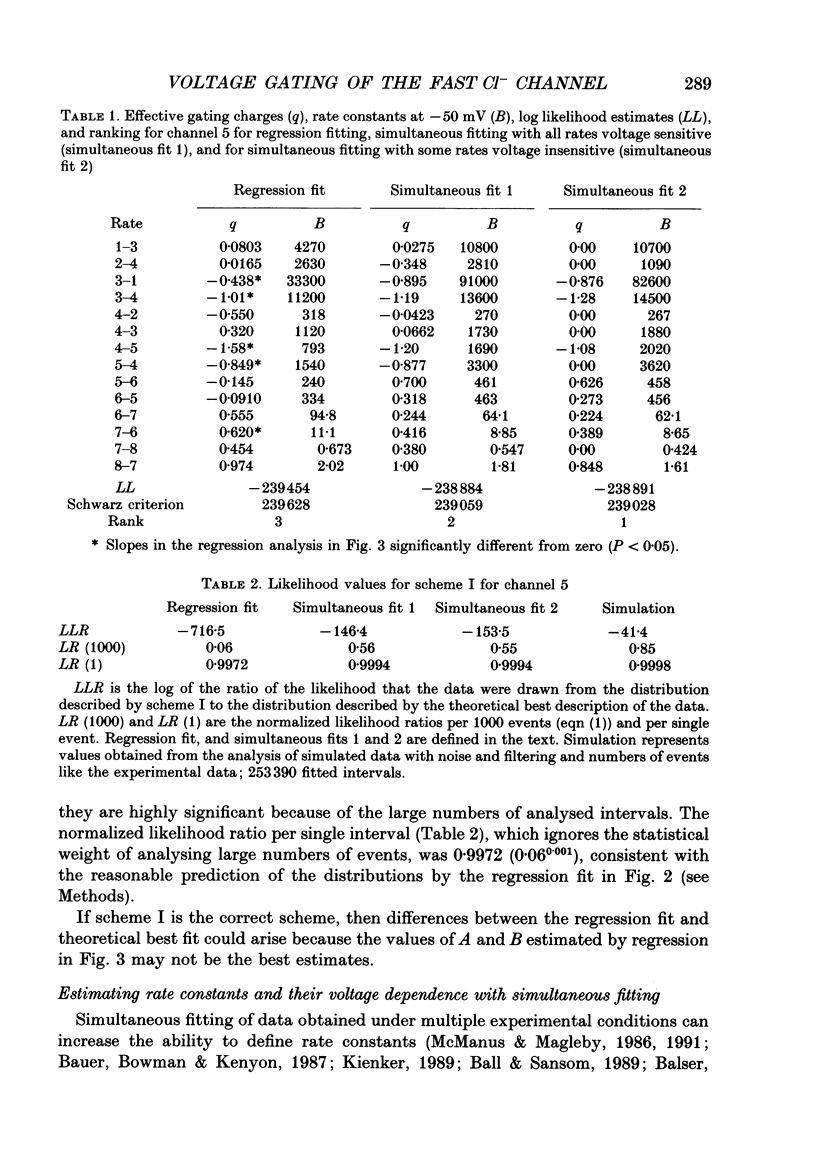
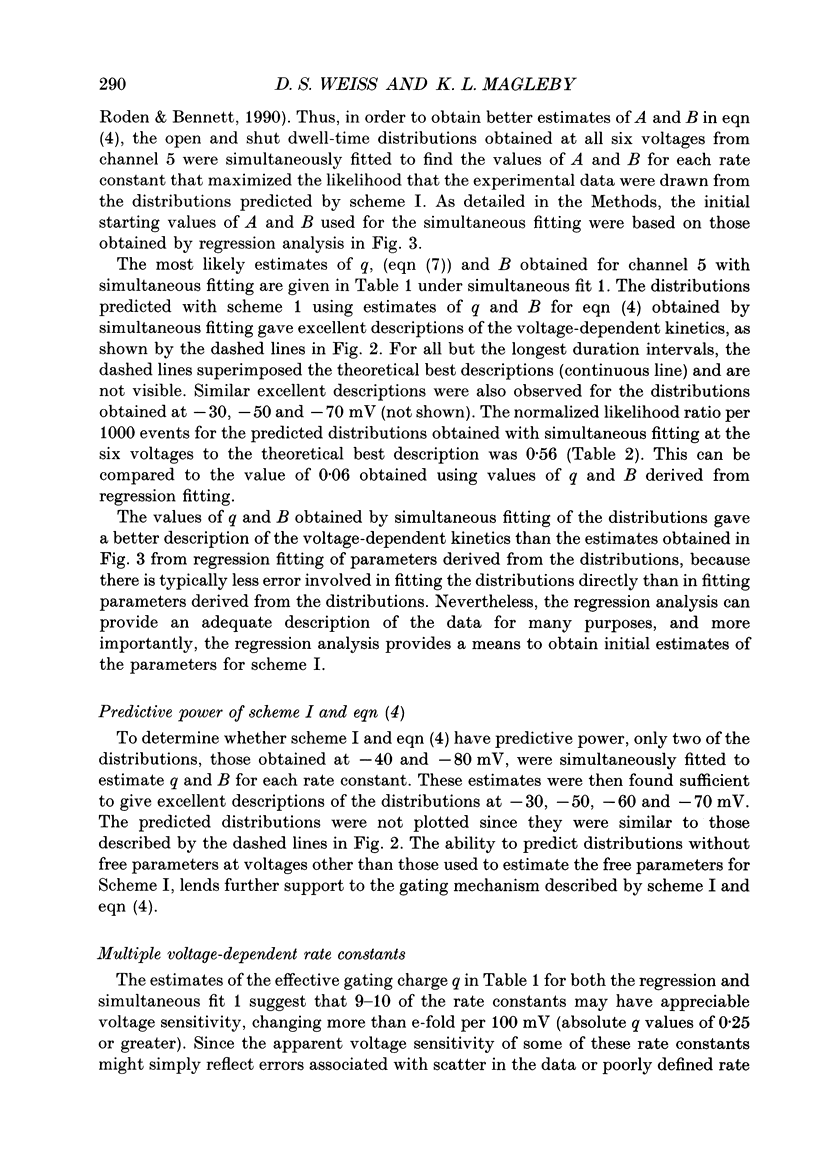
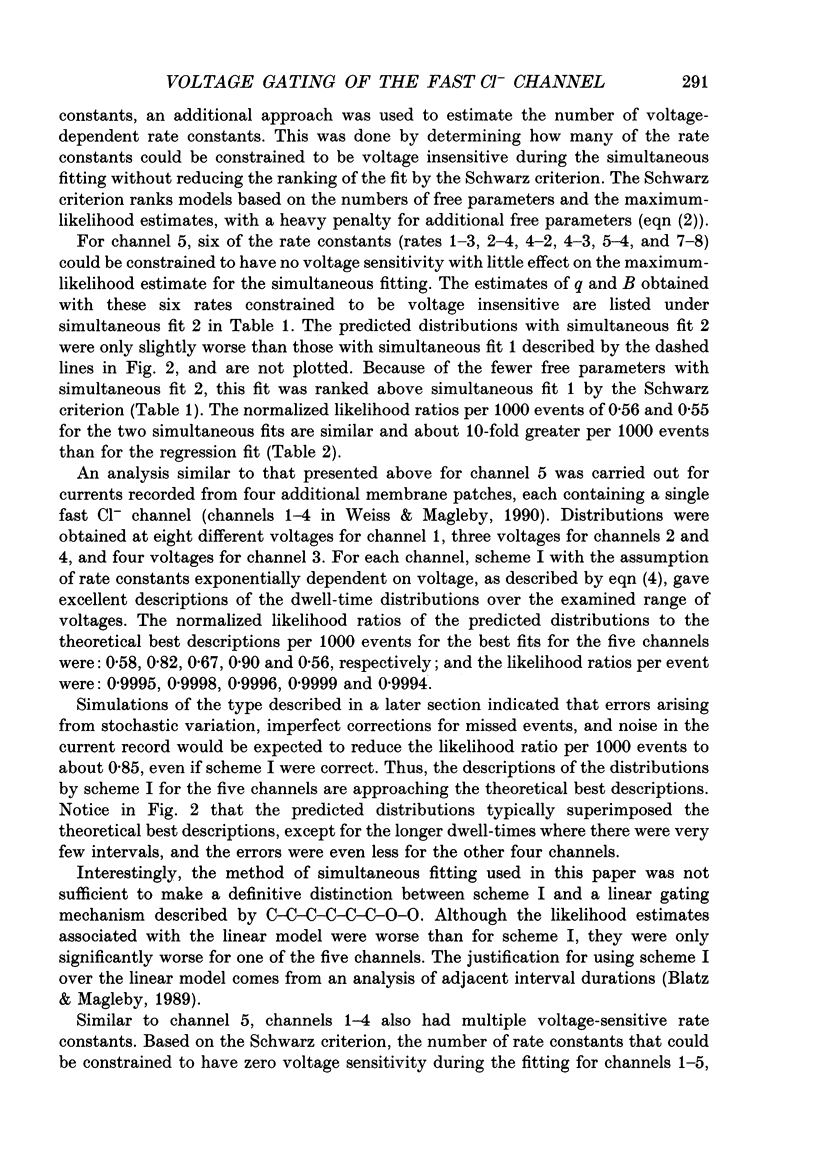
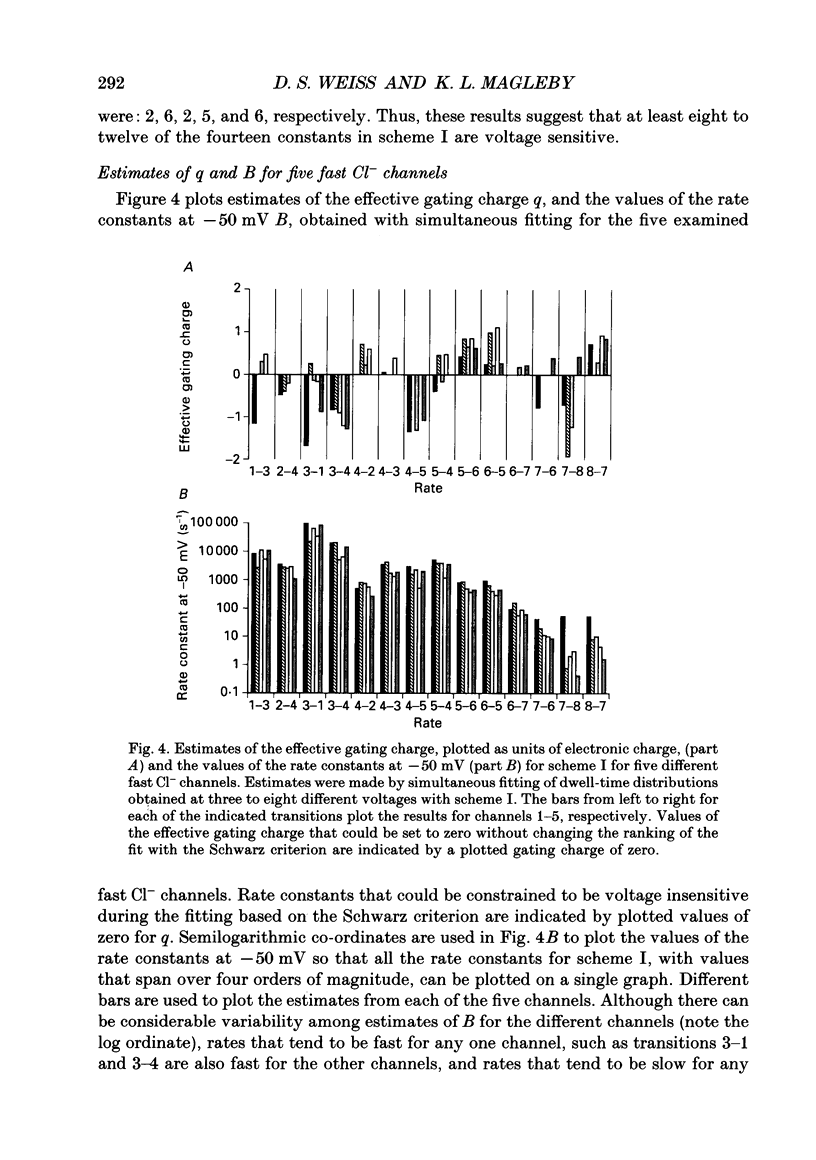
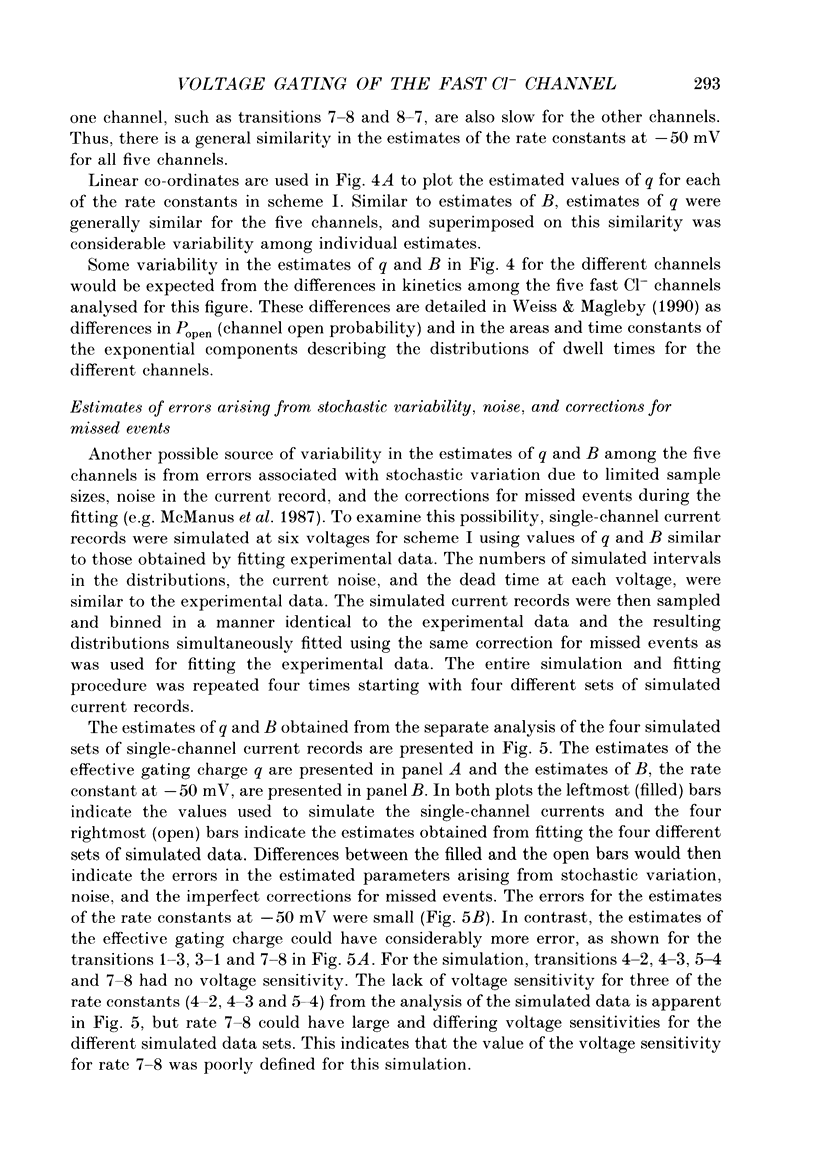
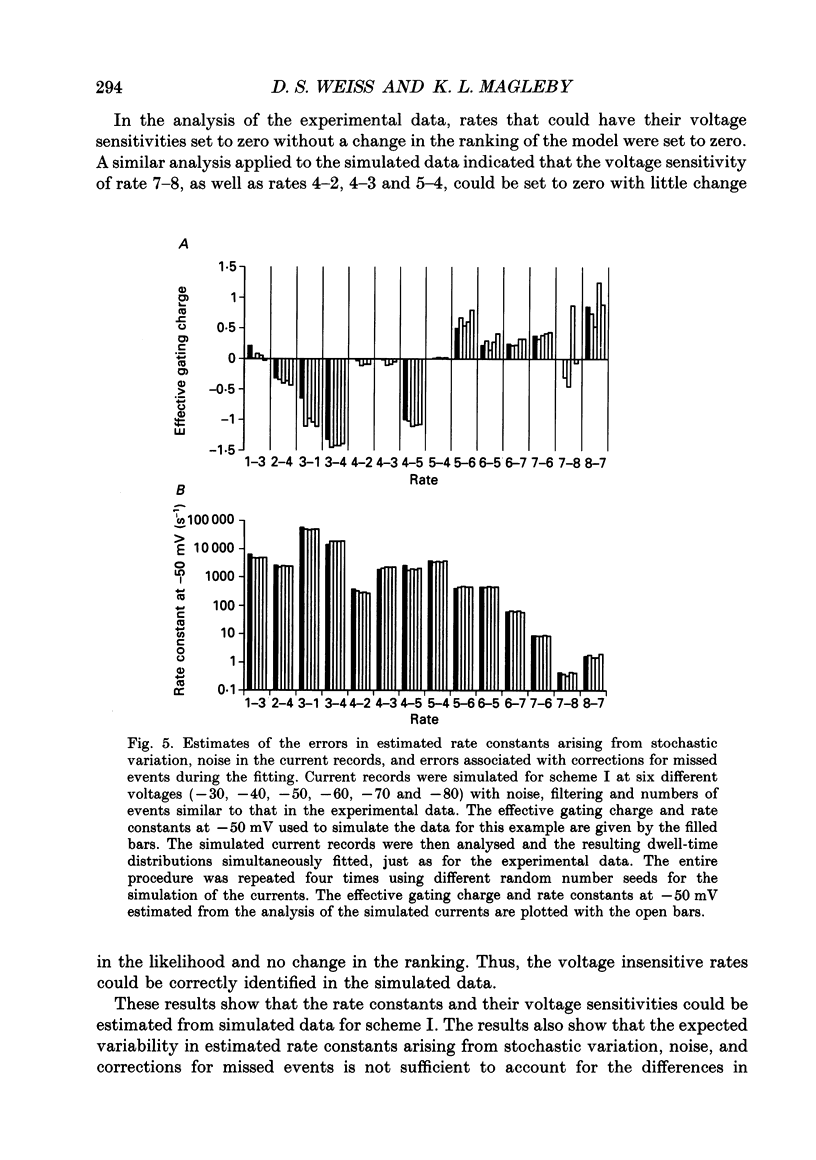
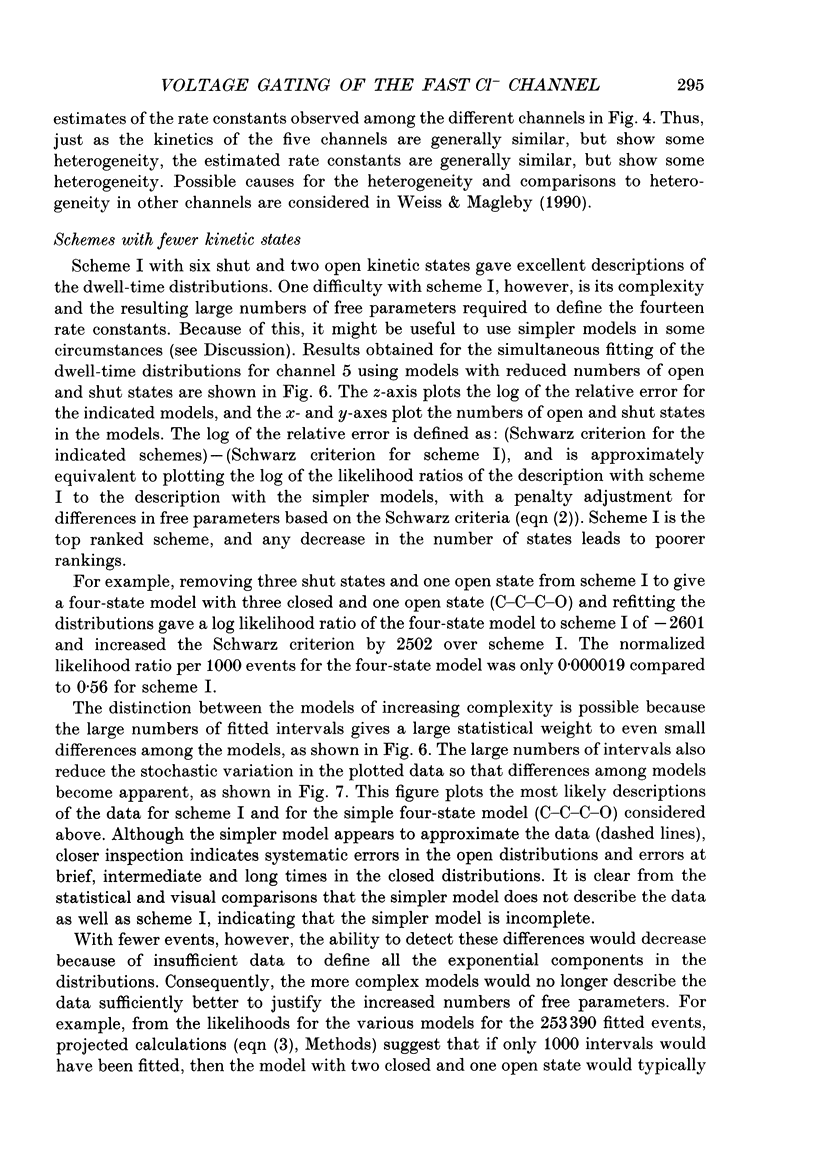
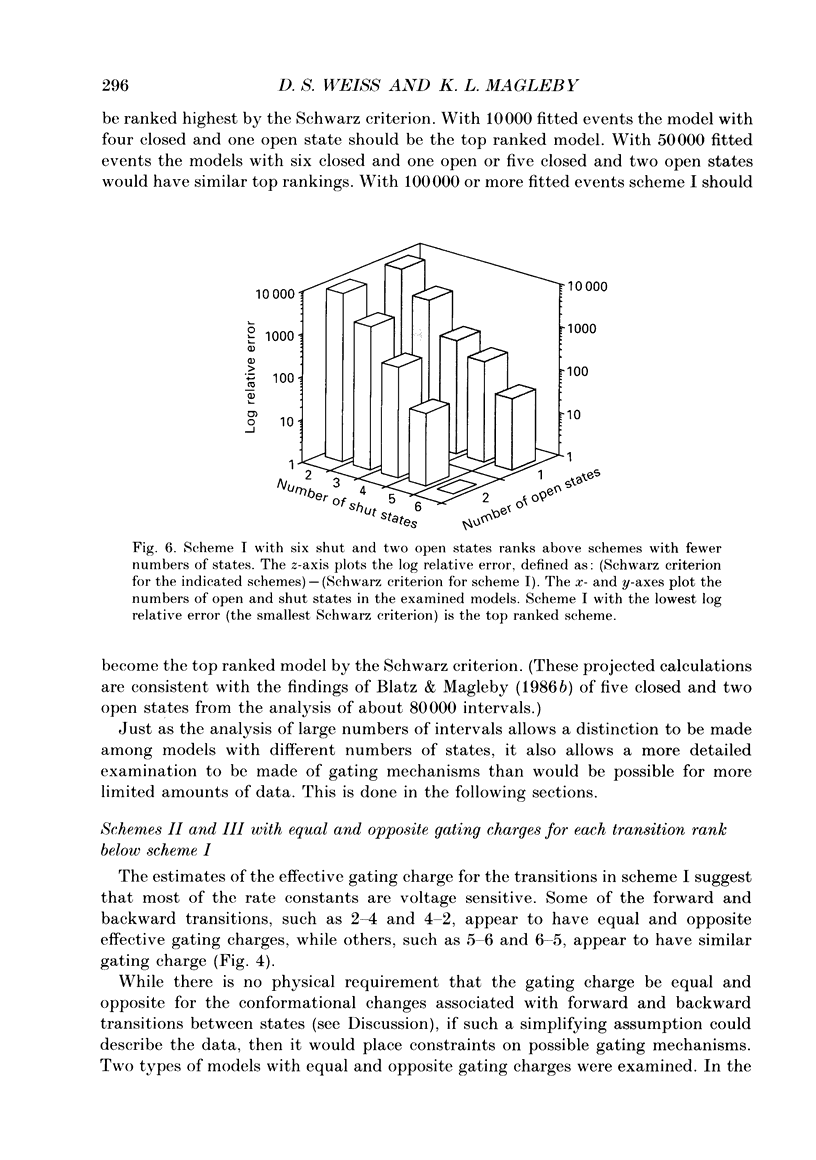
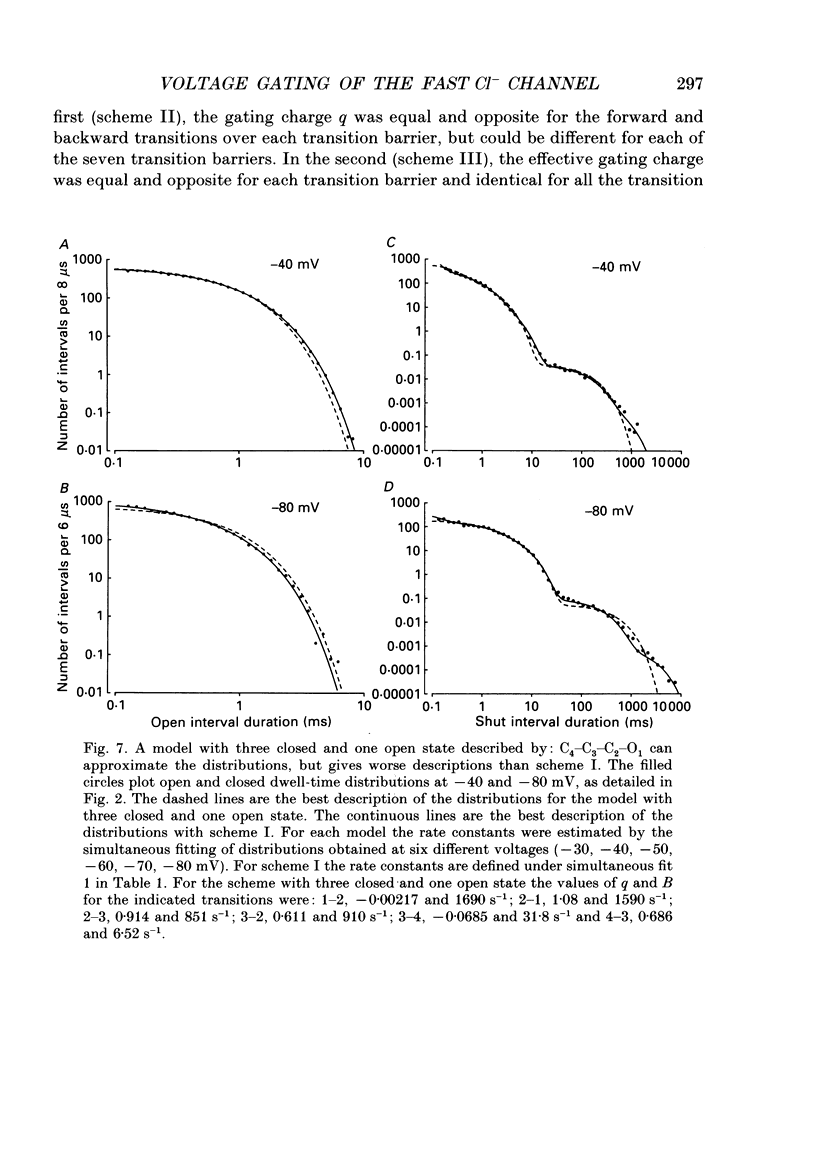
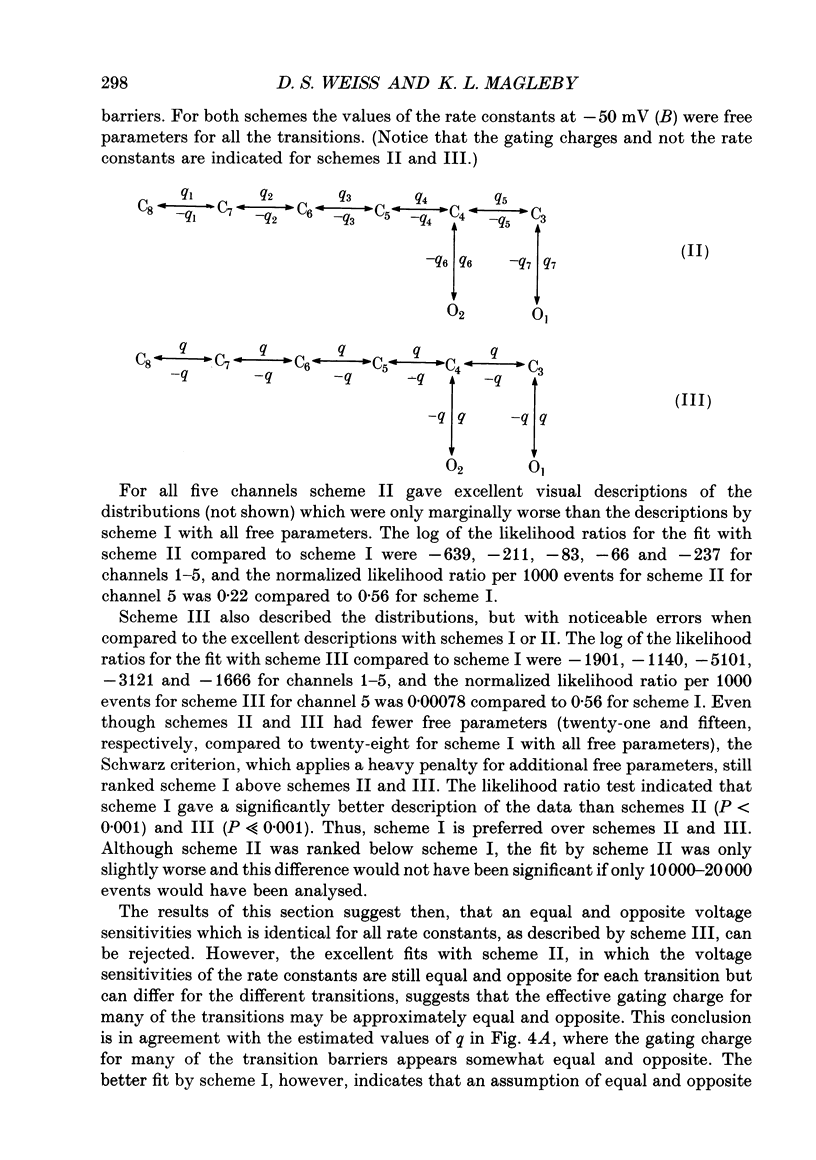
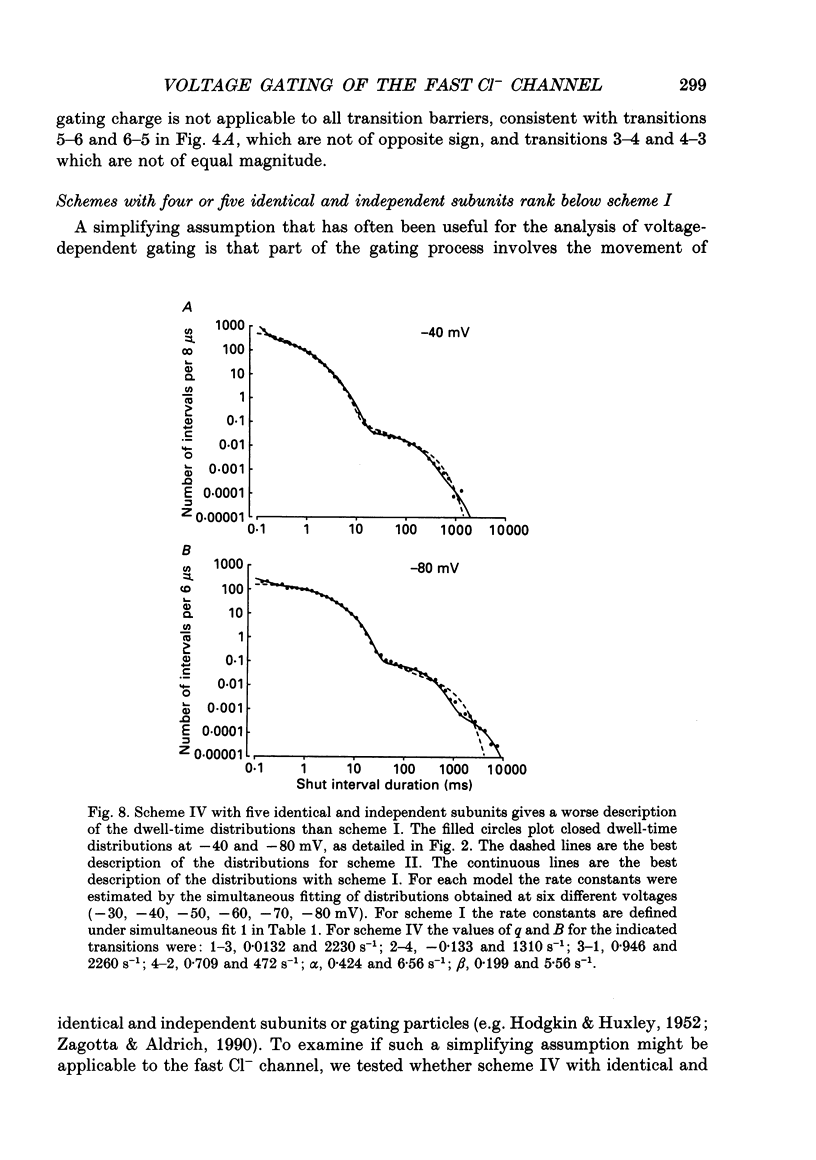
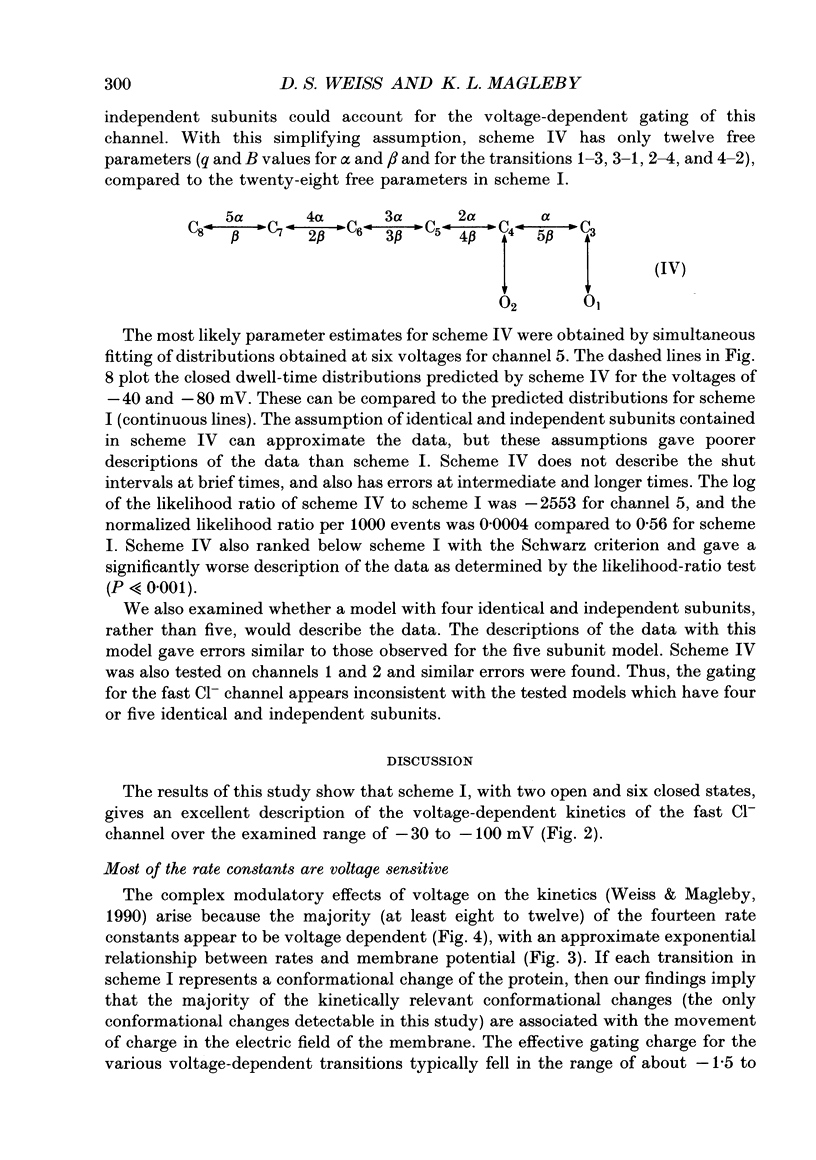
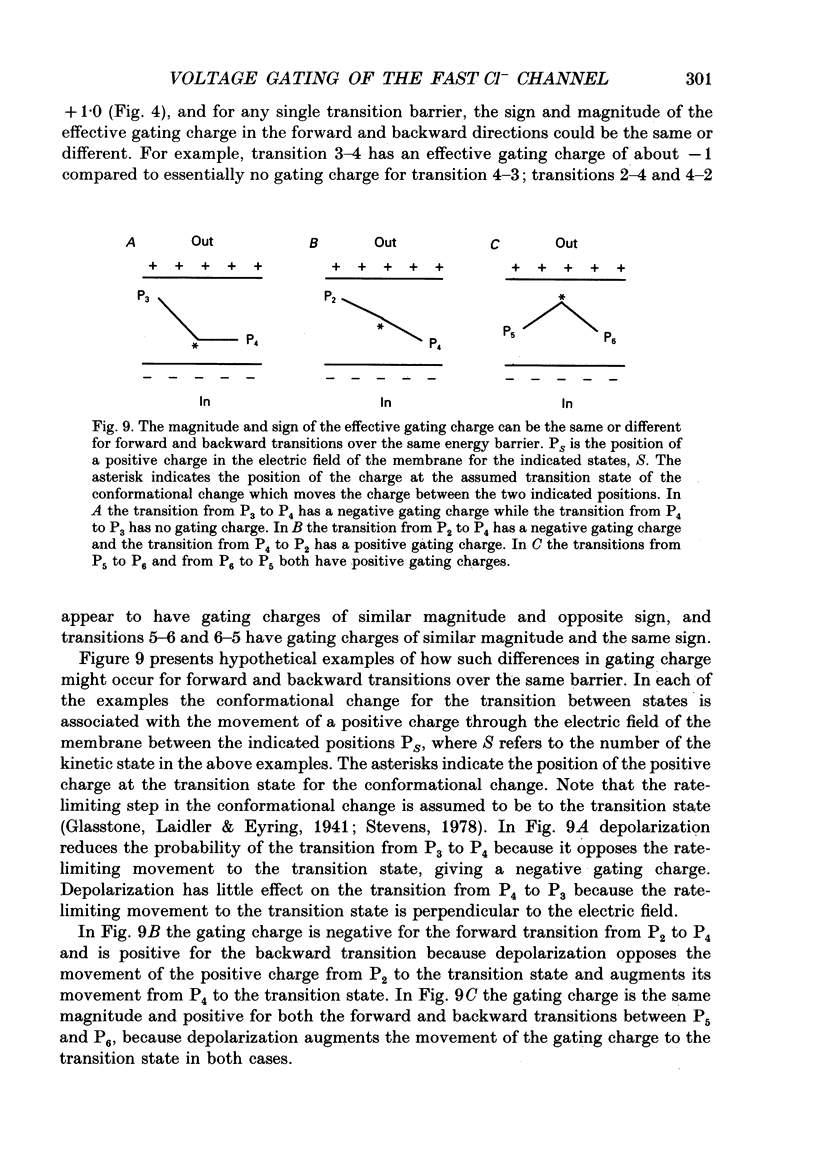
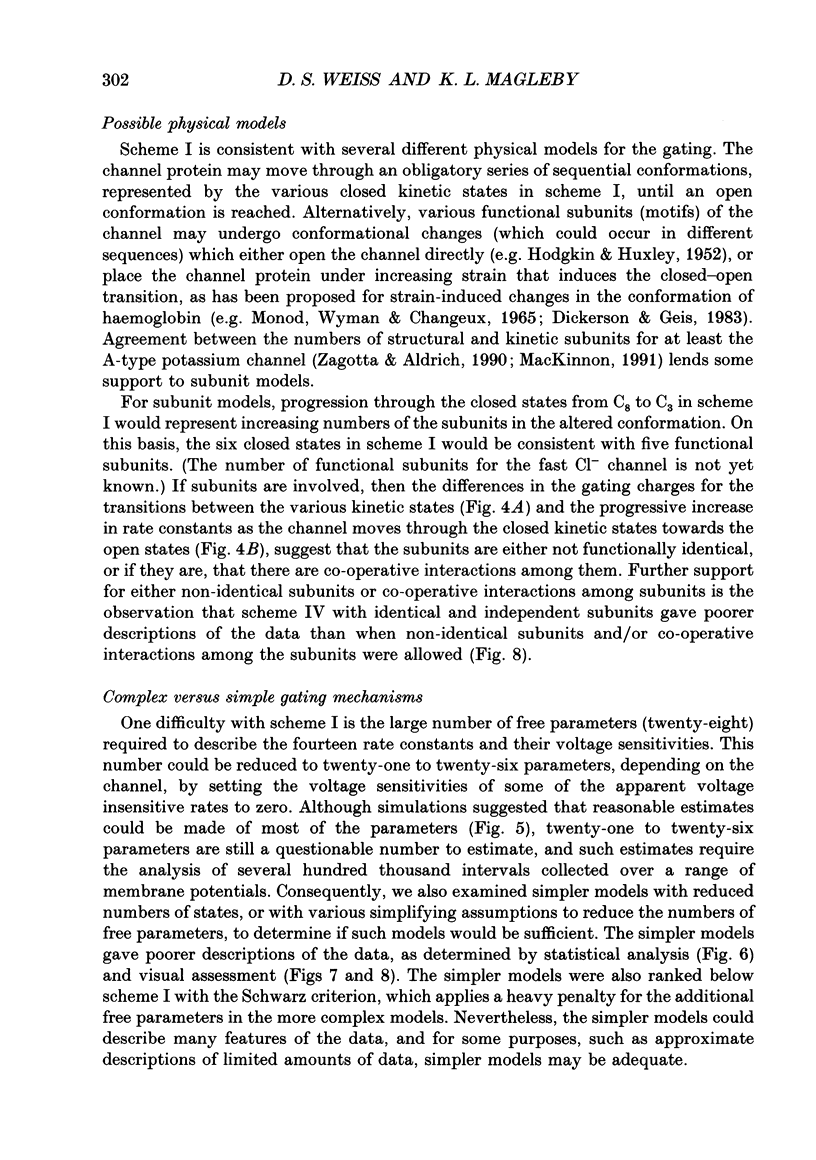
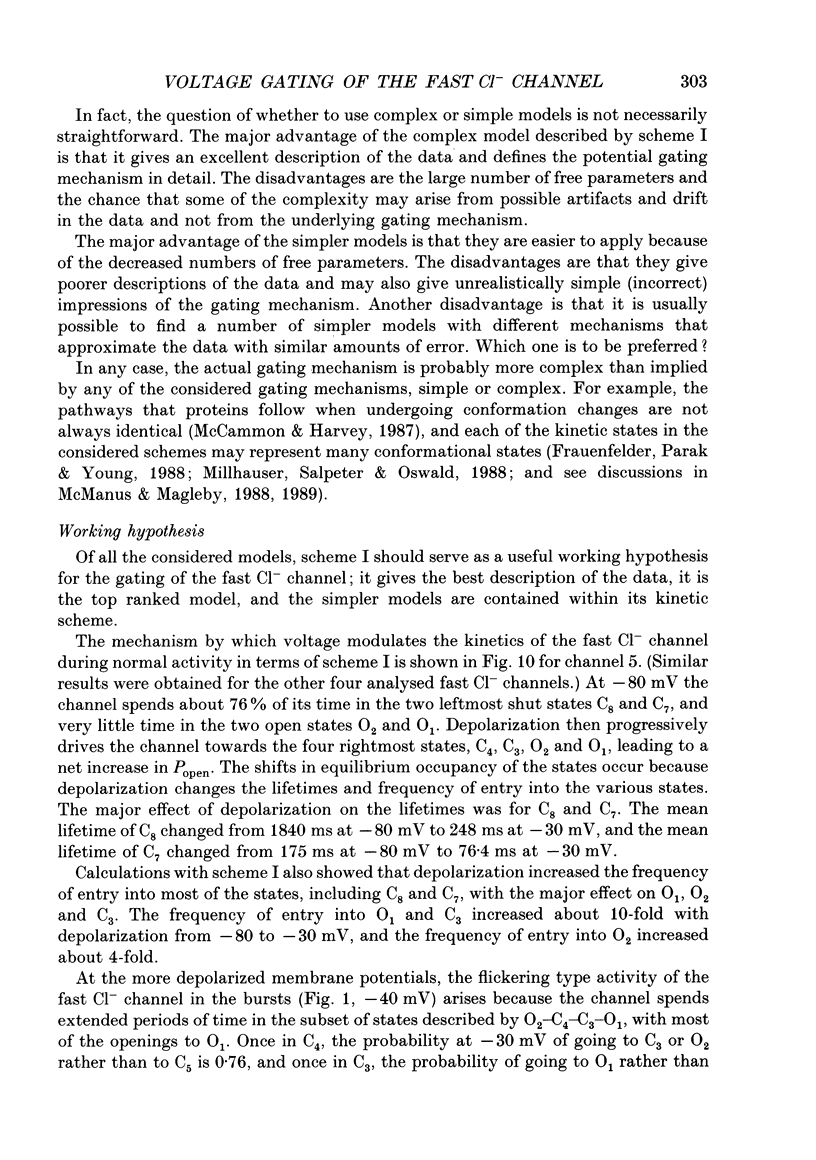
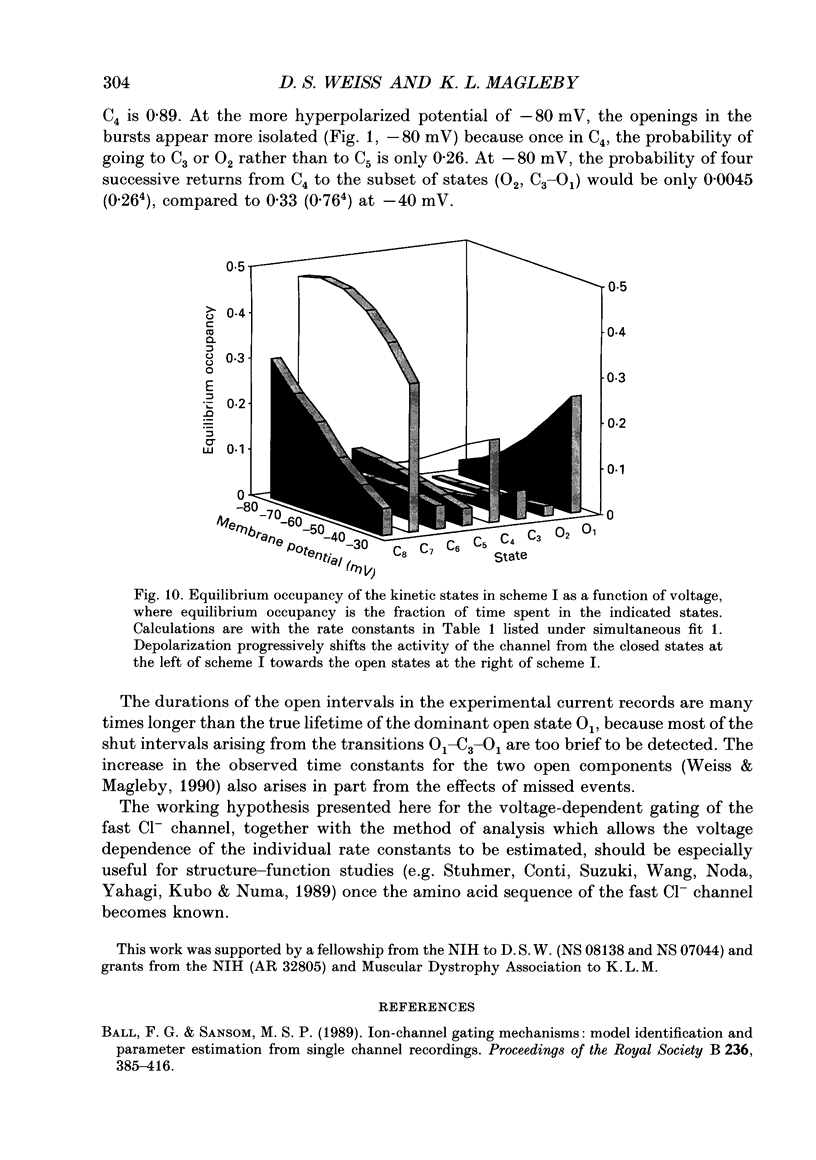
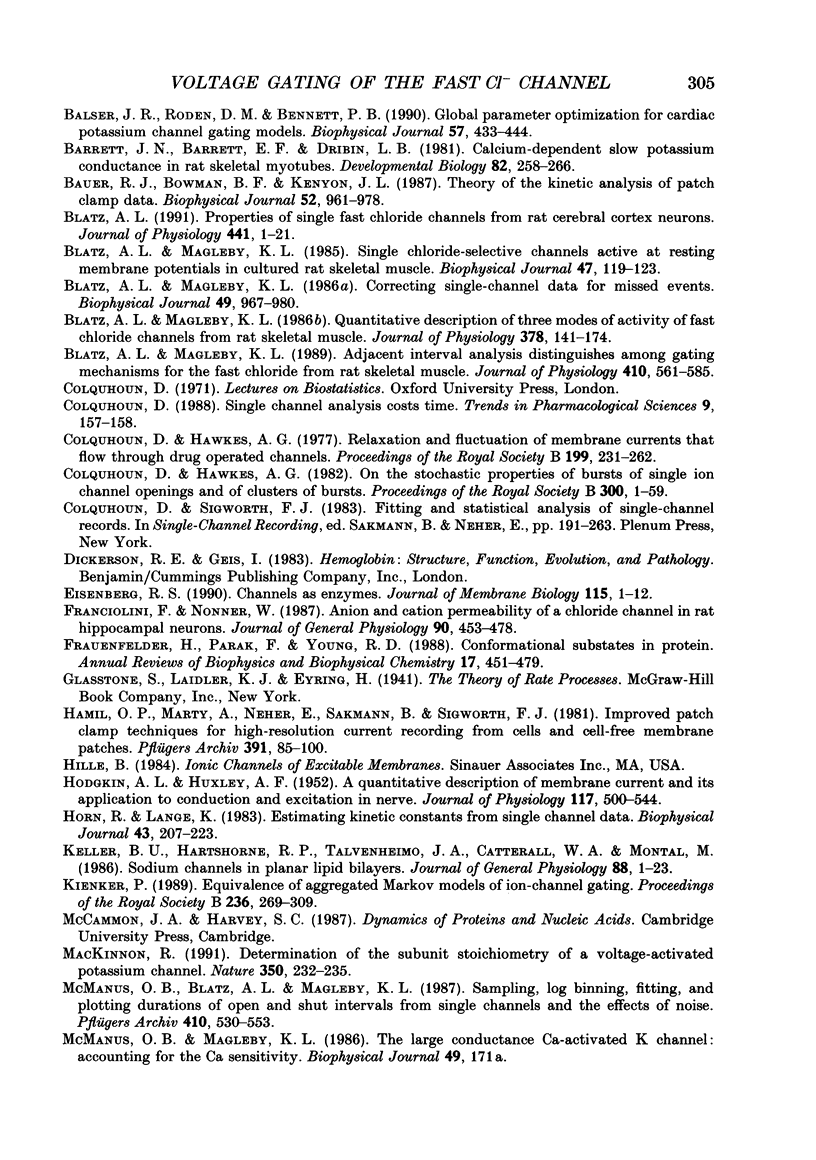
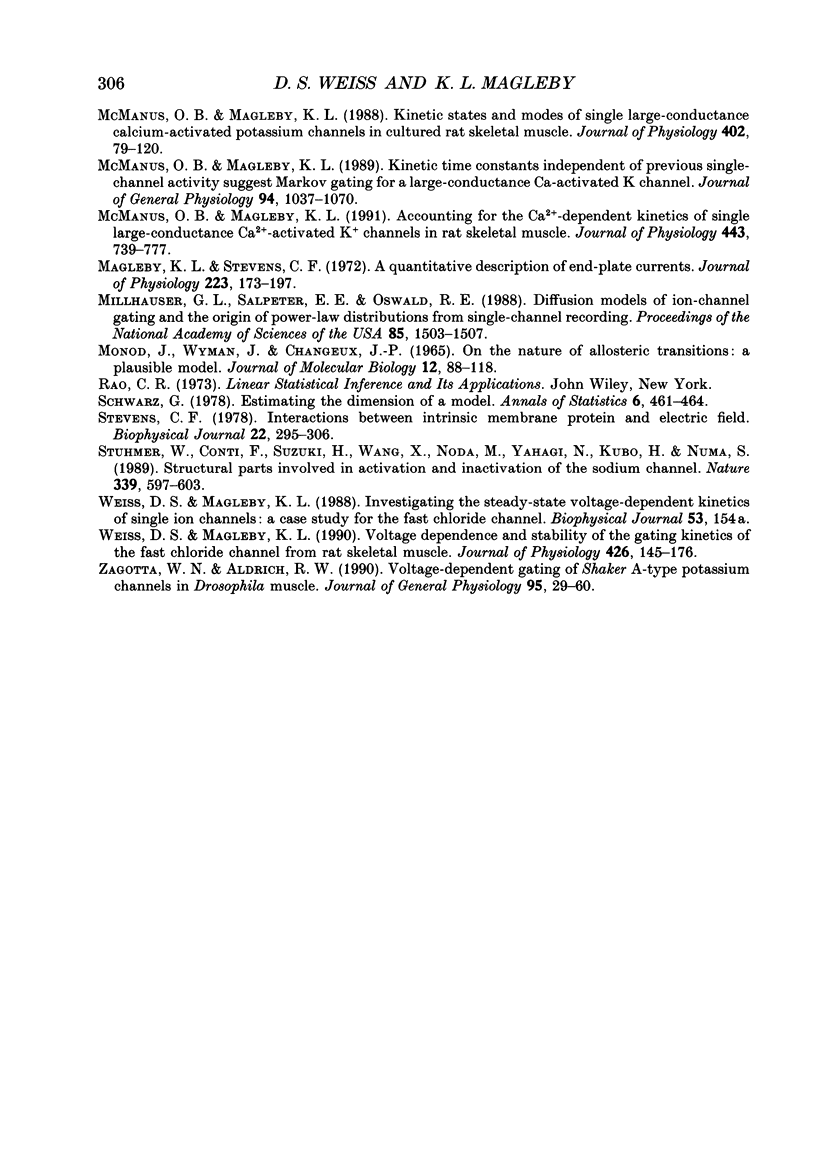
Images in this article
Selected References
These references are in PubMed. This may not be the complete list of references from this article.
- Ball F. G., Sansom M. S. Ion-channel gating mechanisms: model identification and parameter estimation from single channel recordings. Proc R Soc Lond B Biol Sci. 1989 May 22;236(1285):385–416. doi: 10.1098/rspb.1989.0029. [DOI] [PubMed] [Google Scholar]
- Balser J. R., Roden D. M., Bennett P. B. Global parameter optimization for cardiac potassium channel gating models. Biophys J. 1990 Mar;57(3):433–444. doi: 10.1016/S0006-3495(90)82560-1. [DOI] [PMC free article] [PubMed] [Google Scholar]
- Barrett J. N., Barrett E. F., Dribin L. B. Calcium-dependent slow potassium conductance in rat skeletal myotubes. Dev Biol. 1981 Mar;82(2):258–266. doi: 10.1016/0012-1606(81)90450-4. [DOI] [PubMed] [Google Scholar]
- Bauer R. J., Bowman B. F., Kenyon J. L. Theory of the kinetic analysis of patch-clamp data. Biophys J. 1987 Dec;52(6):961–978. doi: 10.1016/S0006-3495(87)83289-7. [DOI] [PMC free article] [PubMed] [Google Scholar]
- Blatz A. L., Magleby K. L. Adjacent interval analysis distinguishes among gating mechanisms for the fast chloride channel from rat skeletal muscle. J Physiol. 1989 Mar;410:561–585. doi: 10.1113/jphysiol.1989.sp017549. [DOI] [PMC free article] [PubMed] [Google Scholar]
- Blatz A. L., Magleby K. L. Correcting single channel data for missed events. Biophys J. 1986 May;49(5):967–980. doi: 10.1016/S0006-3495(86)83725-0. [DOI] [PMC free article] [PubMed] [Google Scholar]
- Blatz A. L., Magleby K. L. Quantitative description of three modes of activity of fast chloride channels from rat skeletal muscle. J Physiol. 1986 Sep;378:141–174. doi: 10.1113/jphysiol.1986.sp016212. [DOI] [PMC free article] [PubMed] [Google Scholar]
- Blatz A. L., Magleby K. L. Single chloride-selective channels active at resting membrane potentials in cultured rat skeletal muscle. Biophys J. 1985 Jan;47(1):119–123. doi: 10.1016/S0006-3495(85)83884-4. [DOI] [PMC free article] [PubMed] [Google Scholar]
- Blatz A. L. Properties of single fast chloride channels from rat cerebral cortex neurons. J Physiol. 1991 Sep;441:1–21. doi: 10.1113/jphysiol.1991.sp018735. [DOI] [PMC free article] [PubMed] [Google Scholar]
- Colquhoun D., Hawkes A. G. On the stochastic properties of bursts of single ion channel openings and of clusters of bursts. Philos Trans R Soc Lond B Biol Sci. 1982 Dec 24;300(1098):1–59. doi: 10.1098/rstb.1982.0156. [DOI] [PubMed] [Google Scholar]
- Colquhoun D., Hawkes A. G. Relaxation and fluctuations of membrane currents that flow through drug-operated channels. Proc R Soc Lond B Biol Sci. 1977 Nov 14;199(1135):231–262. doi: 10.1098/rspb.1977.0137. [DOI] [PubMed] [Google Scholar]
- Colquhoun D. Single channel analysis costs time. Trends Pharmacol Sci. 1988 May;9(5):157–158. doi: 10.1016/0165-6147(88)90027-2. [DOI] [PubMed] [Google Scholar]
- Eisenberg R. S. Channels as enzymes. J Membr Biol. 1990 Apr;115(1):1–12. doi: 10.1007/BF01869101. [DOI] [PubMed] [Google Scholar]
- Franciolini F., Nonner W. Anion and cation permeability of a chloride channel in rat hippocampal neurons. J Gen Physiol. 1987 Oct;90(4):453–478. doi: 10.1085/jgp.90.4.453. [DOI] [PMC free article] [PubMed] [Google Scholar]
- Frauenfelder H., Parak F., Young R. D. Conformational substates in proteins. Annu Rev Biophys Biophys Chem. 1988;17:451–479. doi: 10.1146/annurev.bb.17.060188.002315. [DOI] [PubMed] [Google Scholar]
- HODGKIN A. L., HUXLEY A. F. A quantitative description of membrane current and its application to conduction and excitation in nerve. J Physiol. 1952 Aug;117(4):500–544. doi: 10.1113/jphysiol.1952.sp004764. [DOI] [PMC free article] [PubMed] [Google Scholar]
- Hamill O. P., Marty A., Neher E., Sakmann B., Sigworth F. J. Improved patch-clamp techniques for high-resolution current recording from cells and cell-free membrane patches. Pflugers Arch. 1981 Aug;391(2):85–100. doi: 10.1007/BF00656997. [DOI] [PubMed] [Google Scholar]
- Horn R., Lange K. Estimating kinetic constants from single channel data. Biophys J. 1983 Aug;43(2):207–223. doi: 10.1016/S0006-3495(83)84341-0. [DOI] [PMC free article] [PubMed] [Google Scholar]
- Keller B. U., Hartshorne R. P., Talvenheimo J. A., Catterall W. A., Montal M. Sodium channels in planar lipid bilayers. Channel gating kinetics of purified sodium channels modified by batrachotoxin. J Gen Physiol. 1986 Jul;88(1):1–23. doi: 10.1085/jgp.88.1.1. [DOI] [PMC free article] [PubMed] [Google Scholar]
- Kienker P. Equivalence of aggregated Markov models of ion-channel gating. Proc R Soc Lond B Biol Sci. 1989 Apr 22;236(1284):269–309. doi: 10.1098/rspb.1989.0024. [DOI] [PubMed] [Google Scholar]
- MONOD J., WYMAN J., CHANGEUX J. P. ON THE NATURE OF ALLOSTERIC TRANSITIONS: A PLAUSIBLE MODEL. J Mol Biol. 1965 May;12:88–118. doi: 10.1016/s0022-2836(65)80285-6. [DOI] [PubMed] [Google Scholar]
- MacKinnon R. Determination of the subunit stoichiometry of a voltage-activated potassium channel. Nature. 1991 Mar 21;350(6315):232–235. doi: 10.1038/350232a0. [DOI] [PubMed] [Google Scholar]
- Magleby K. L., Stevens C. F. A quantitative description of end-plate currents. J Physiol. 1972 May;223(1):173–197. doi: 10.1113/jphysiol.1972.sp009840. [DOI] [PMC free article] [PubMed] [Google Scholar]
- McManus O. B., Blatz A. L., Magleby K. L. Sampling, log binning, fitting, and plotting durations of open and shut intervals from single channels and the effects of noise. Pflugers Arch. 1987 Nov;410(4-5):530–553. doi: 10.1007/BF00586537. [DOI] [PubMed] [Google Scholar]
- McManus O. B., Magleby K. L. Accounting for the Ca(2+)-dependent kinetics of single large-conductance Ca(2+)-activated K+ channels in rat skeletal muscle. J Physiol. 1991 Nov;443:739–777. doi: 10.1113/jphysiol.1991.sp018861. [DOI] [PMC free article] [PubMed] [Google Scholar]
- McManus O. B., Magleby K. L. Kinetic states and modes of single large-conductance calcium-activated potassium channels in cultured rat skeletal muscle. J Physiol. 1988 Aug;402:79–120. doi: 10.1113/jphysiol.1988.sp017195. [DOI] [PMC free article] [PubMed] [Google Scholar]
- McManus O. B., Magleby K. L. Kinetic time constants independent of previous single-channel activity suggest Markov gating for a large conductance Ca-activated K channel. J Gen Physiol. 1989 Dec;94(6):1037–1070. doi: 10.1085/jgp.94.6.1037. [DOI] [PMC free article] [PubMed] [Google Scholar]
- Millhauser G. L., Salpeter E. E., Oswald R. E. Diffusion models of ion-channel gating and the origin of power-law distributions from single-channel recording. Proc Natl Acad Sci U S A. 1988 Mar;85(5):1503–1507. doi: 10.1073/pnas.85.5.1503. [DOI] [PMC free article] [PubMed] [Google Scholar]
- Stevens C. F. Interactions between intrinsic membrane protein and electric field. An approach to studying nerve excitability. Biophys J. 1978 May;22(2):295–306. doi: 10.1016/S0006-3495(78)85490-3. [DOI] [PMC free article] [PubMed] [Google Scholar]
- Stühmer W., Conti F., Suzuki H., Wang X. D., Noda M., Yahagi N., Kubo H., Numa S. Structural parts involved in activation and inactivation of the sodium channel. Nature. 1989 Jun 22;339(6226):597–603. doi: 10.1038/339597a0. [DOI] [PubMed] [Google Scholar]
- Weiss D. S., Magleby K. L. Voltage dependence and stability of the gating kinetics of the fast chloride channel from rat skeletal muscle. J Physiol. 1990 Jul;426:145–176. doi: 10.1113/jphysiol.1990.sp018131. [DOI] [PMC free article] [PubMed] [Google Scholar]
- Zagotta W. N., Aldrich R. W. Voltage-dependent gating of Shaker A-type potassium channels in Drosophila muscle. J Gen Physiol. 1990 Jan;95(1):29–60. doi: 10.1085/jgp.95.1.29. [DOI] [PMC free article] [PubMed] [Google Scholar]