Abstract
1. The effects of global ischaemia on phosphorus metabolites, intracellular pH (pHi) and developed pressure were measured in isolated whole ferret hearts using 31P nuclear magnetic resonance (NMR) spectroscopy. 2. Brief (10 min) periods of global ischaemia reduced left ventricular developed pressure (LVDP) to undetectable levels. This fall in LVDP was accompanied by a fall in the intracellular concentration of phosphocreatine (PCr) and increases in the concentrations of inorganic phosphate (Pi) and phosphomonoesters. There was no change in the intracellular ATP concentration ([ATP]i). pHi fell approximately linearly at a rate of 0.04 pH units min-1. 3. When ferret hearts were exposed to cyanide (CN-) in the presence of alpha-cyano-4-hydroxycinnamate (CHC), a blocker of lactate efflux, the changes in pHi and [Pi]i which occurred were similar to those observed during global ischaemia. However, developed pressure only fell to around 15% of the control value. 4. Removing the intracellular acidosis (by reducing the CO2 level of the gas with which the perfusate was equilibrated) during exposure to CN- and CHC caused an increase in developed pressure, consistent with the fall in pHi being responsible for a substantial fraction of the fall in developed pressure. 5. Taken together, these results suggest that most, but not all, of the fall in developed pressure during ischaemia can be explained by the effects of the changes in pHi and [Pi]i on the contractile apparatus. 6. Action potential recordings made with a suction electrode during short periods of global ischaemia showed that there was no decrease in action potential duration over the period when developed pressure was falling, eliminating action potential shortening as a possible cause of the fall in developed pressure. 7. In hearts in which the rate of glycolysis had been reduced by glycogen depletion, global ischaemia led to a marked shortening of the action potential. NMR experiments showed that under these conditions [ATP]i decreased by around 50% over the first 10 jin of ischaemia, while the intracellular acidosis which occurred was smaller than that in a control ischaemic period. 8. The time course of the decline of [ATP]i was examined in several hearts during long (45 min and over) ischaemic periods without prior glycogen depletion. After 45 min of ischaemia [ATP]i fell to around two-thirds of the control value, while pHi declined to approximately 6.1. Resting pressure did not increase. On reperfusion pHi recovered rapidly to control levels. [ATP]i, however, did not recover. 9. If ischaemia was prolonged further, [ATP]i eventually became undetectable after 70-90 min.(ABSTRACT TRUNCATED AT 400 WORDS)
Full text
PDF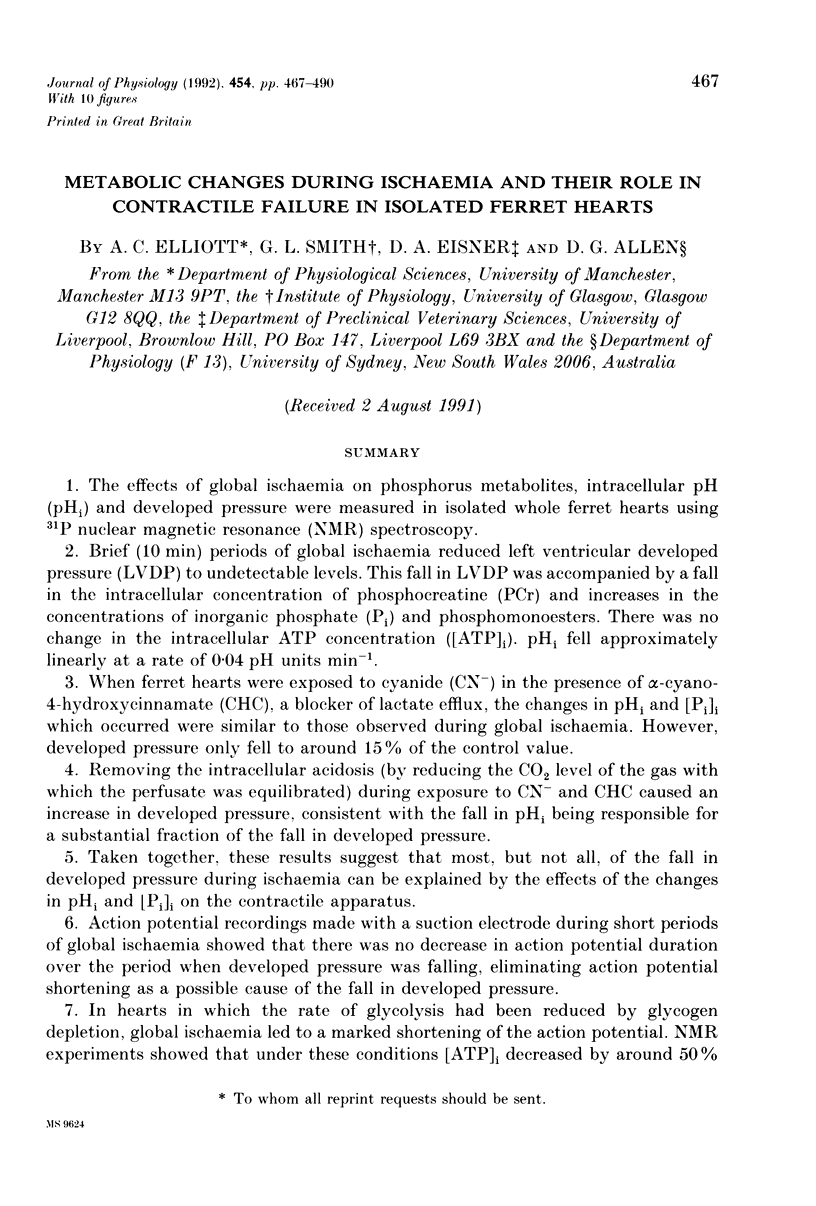
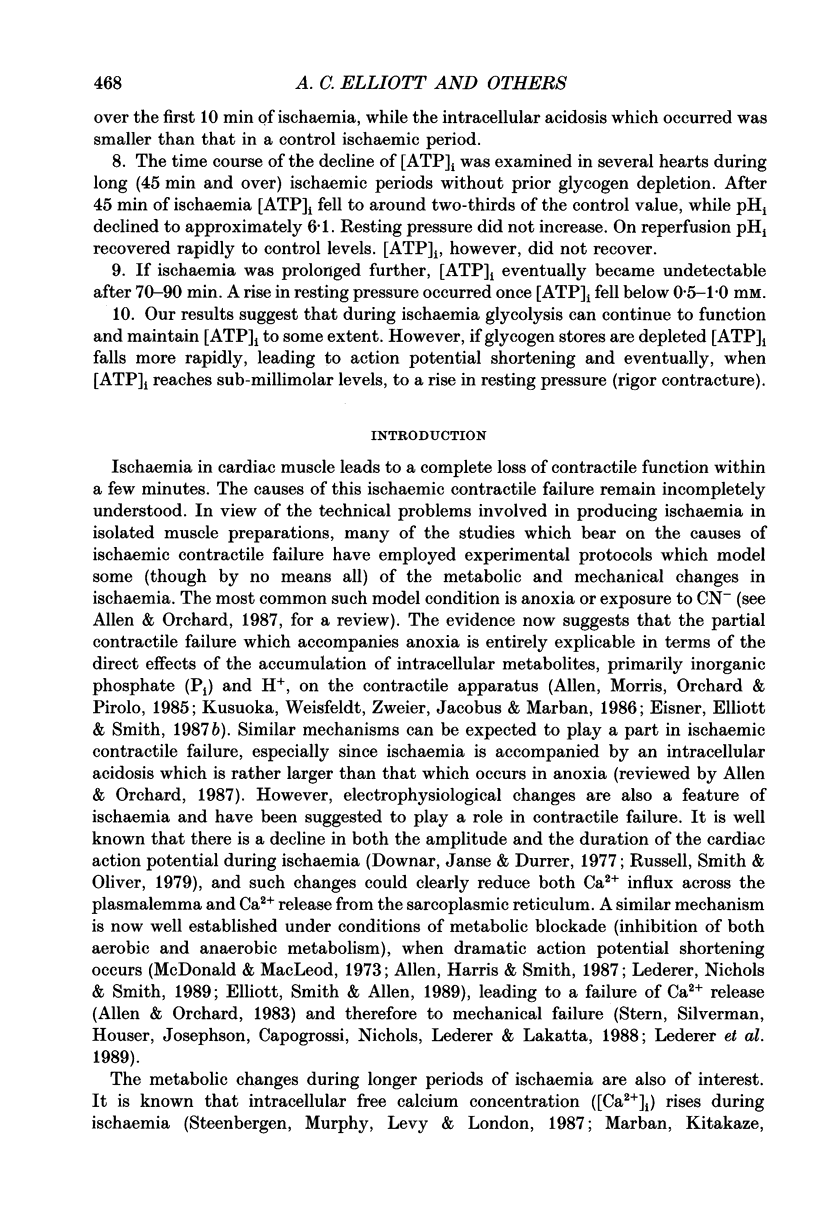
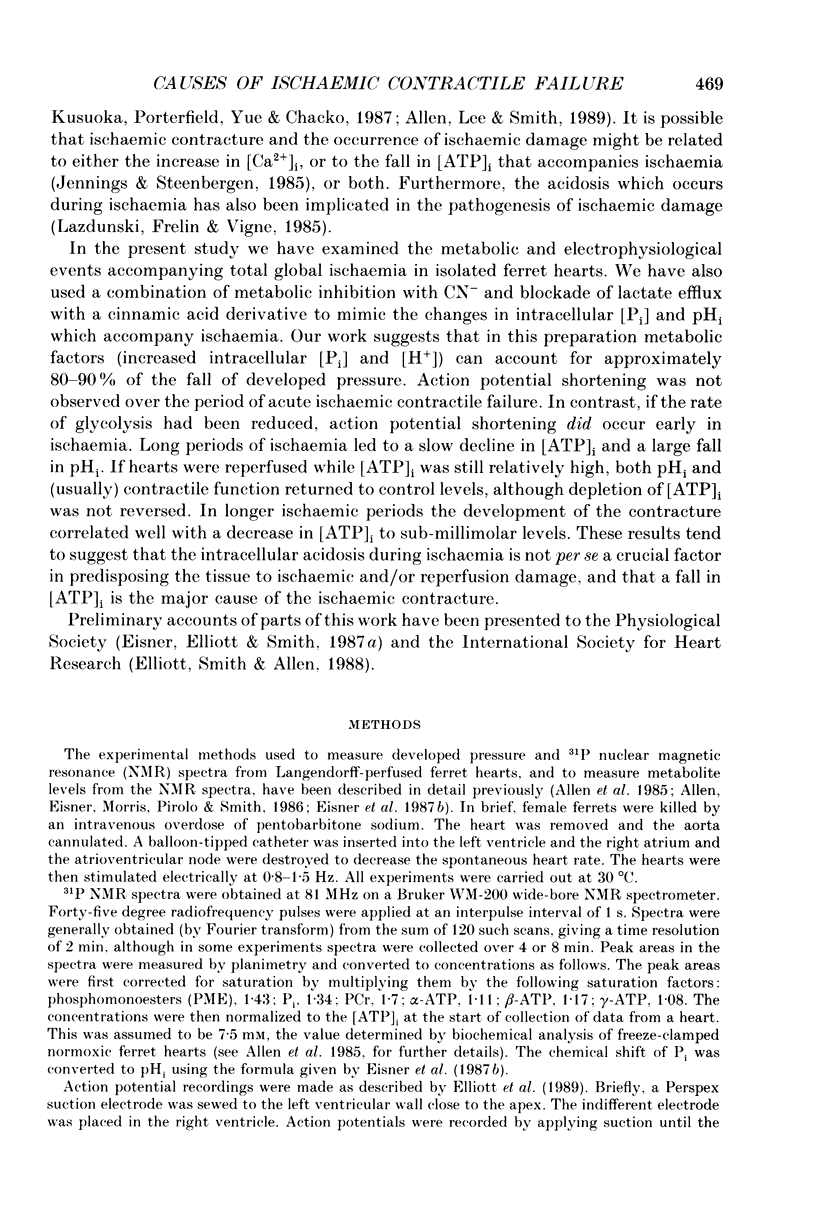
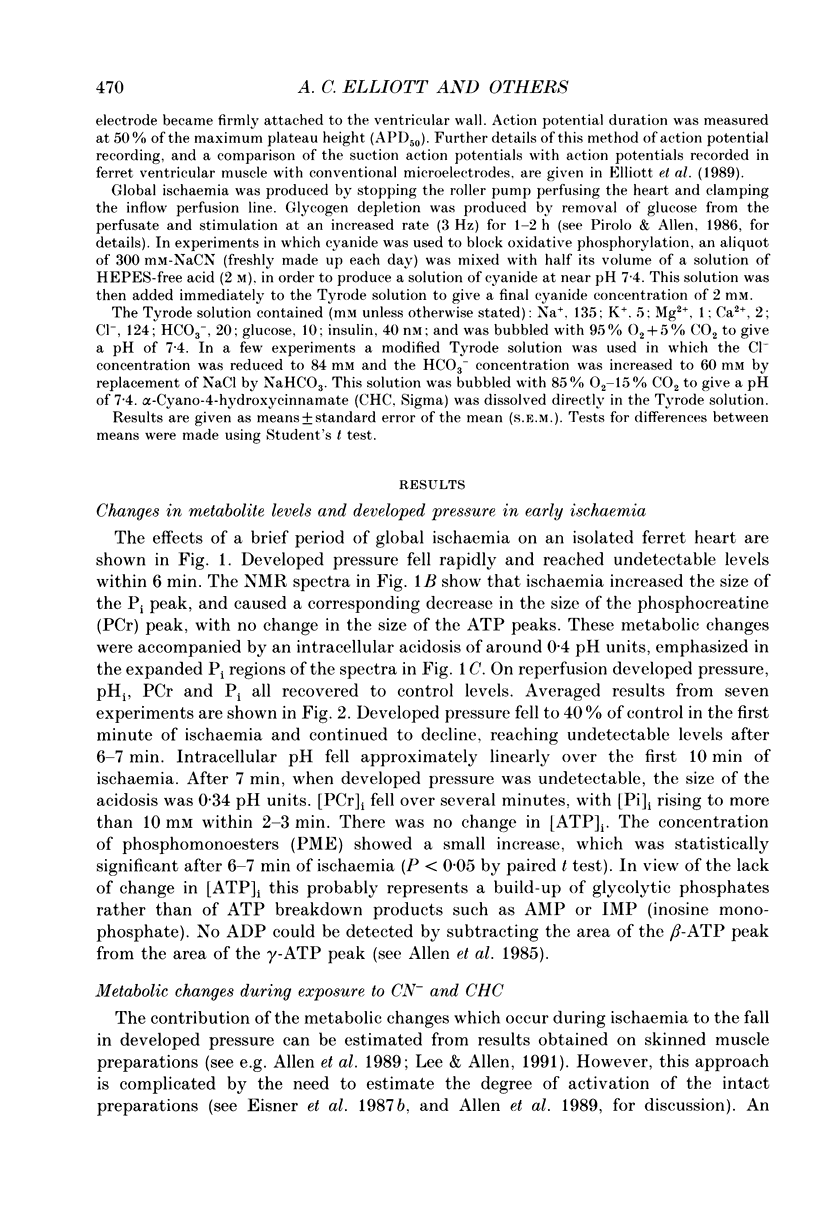
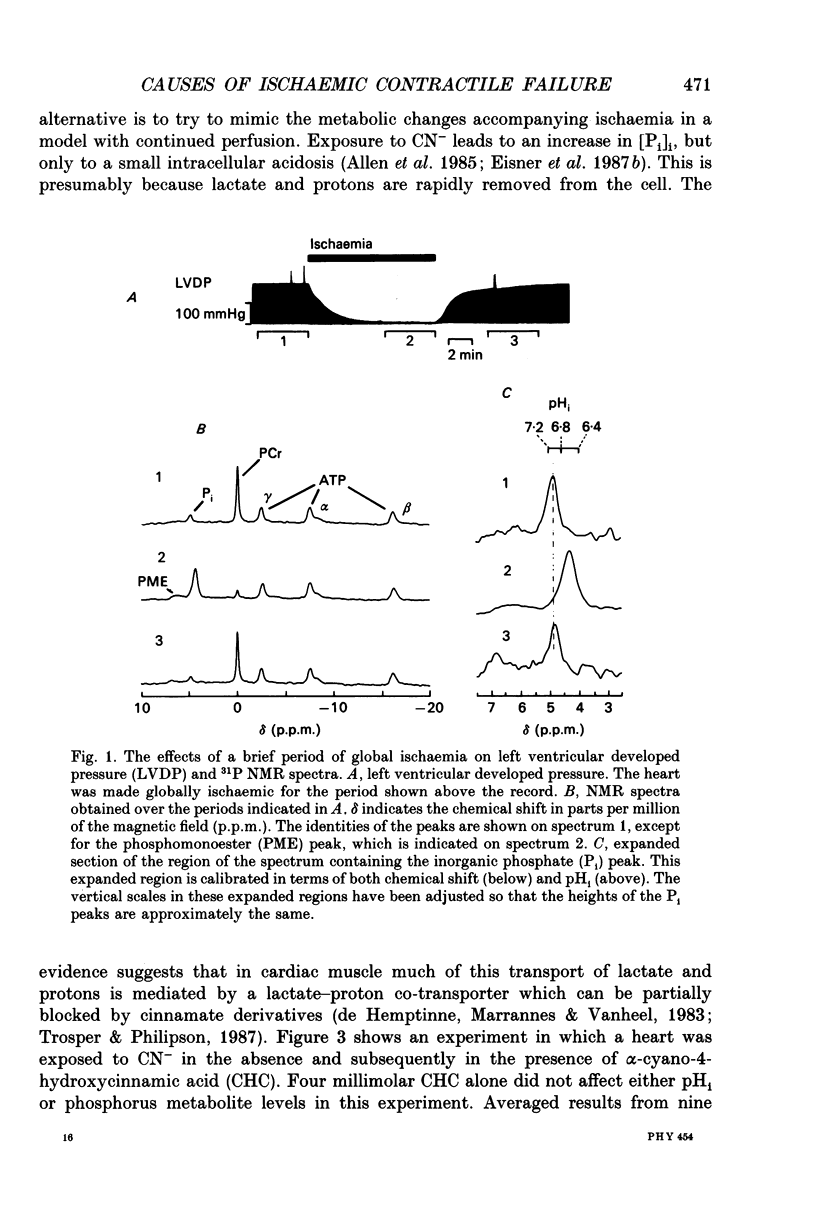
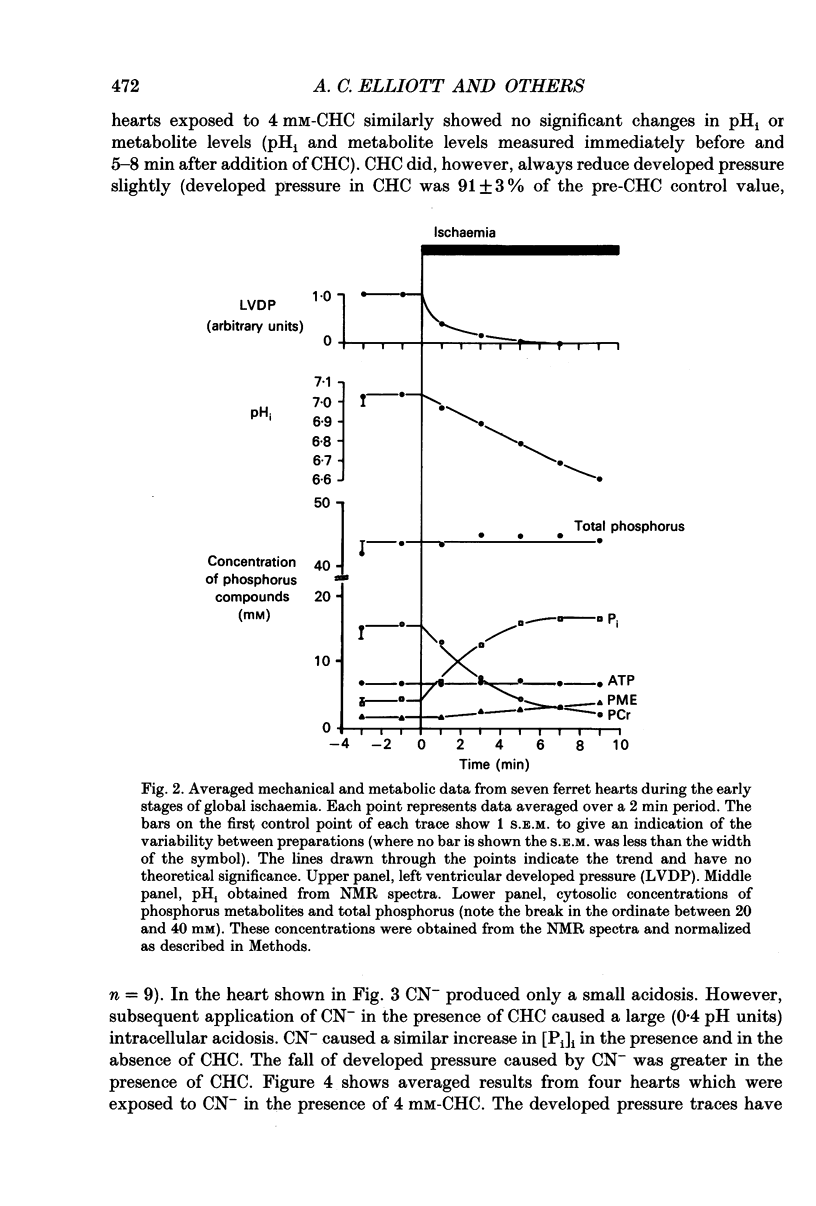
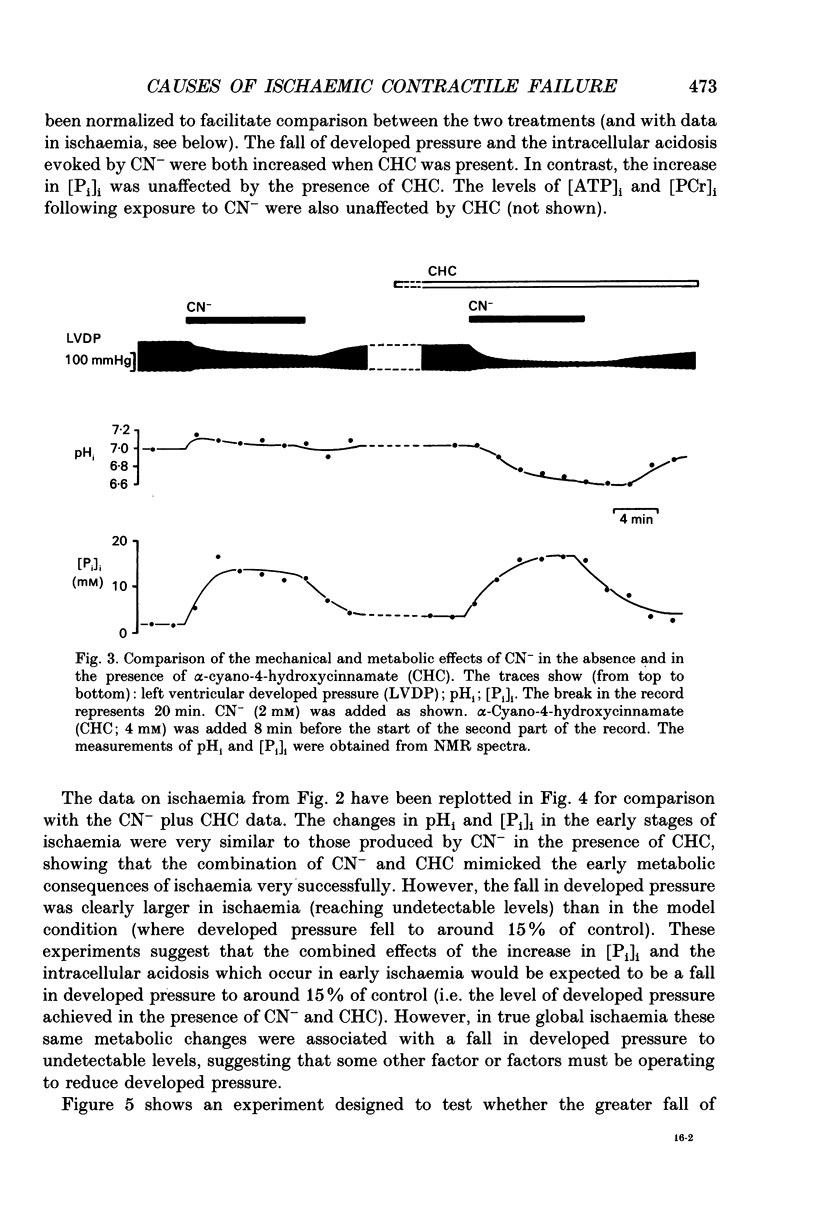
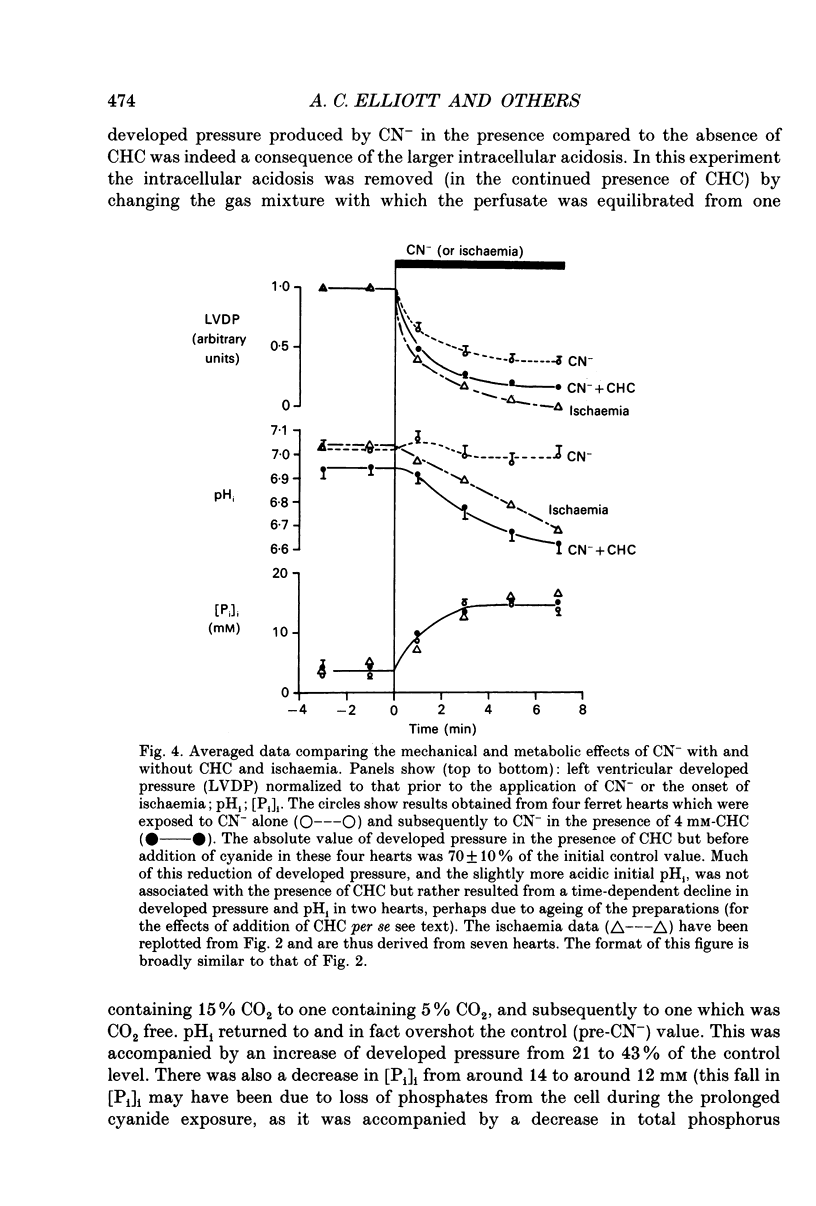
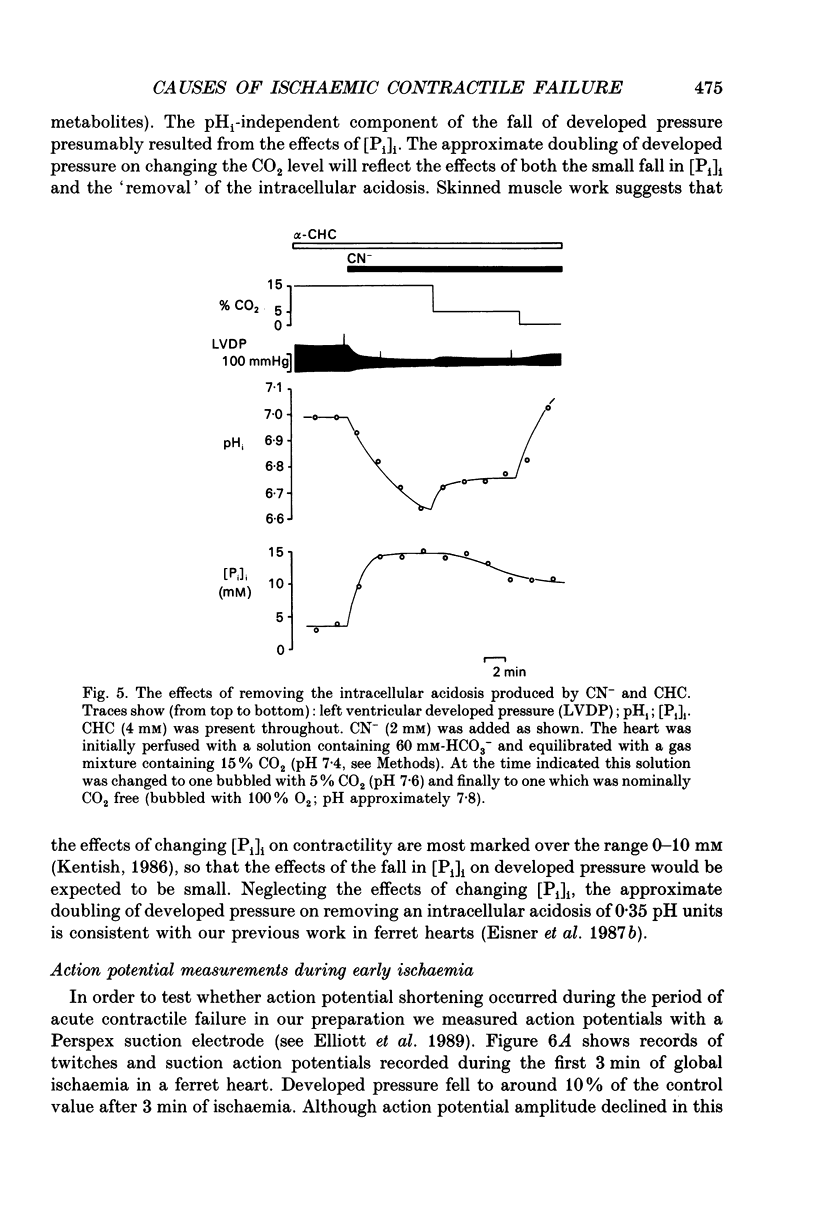
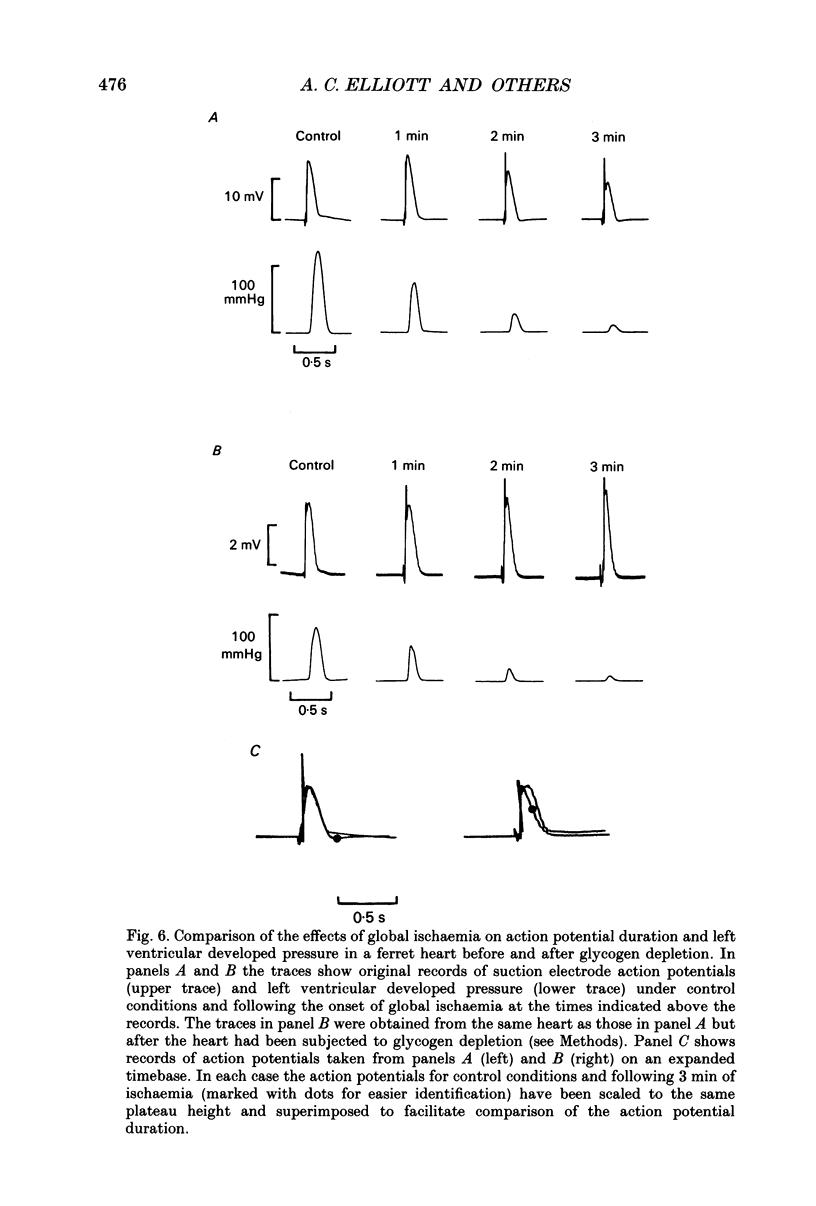
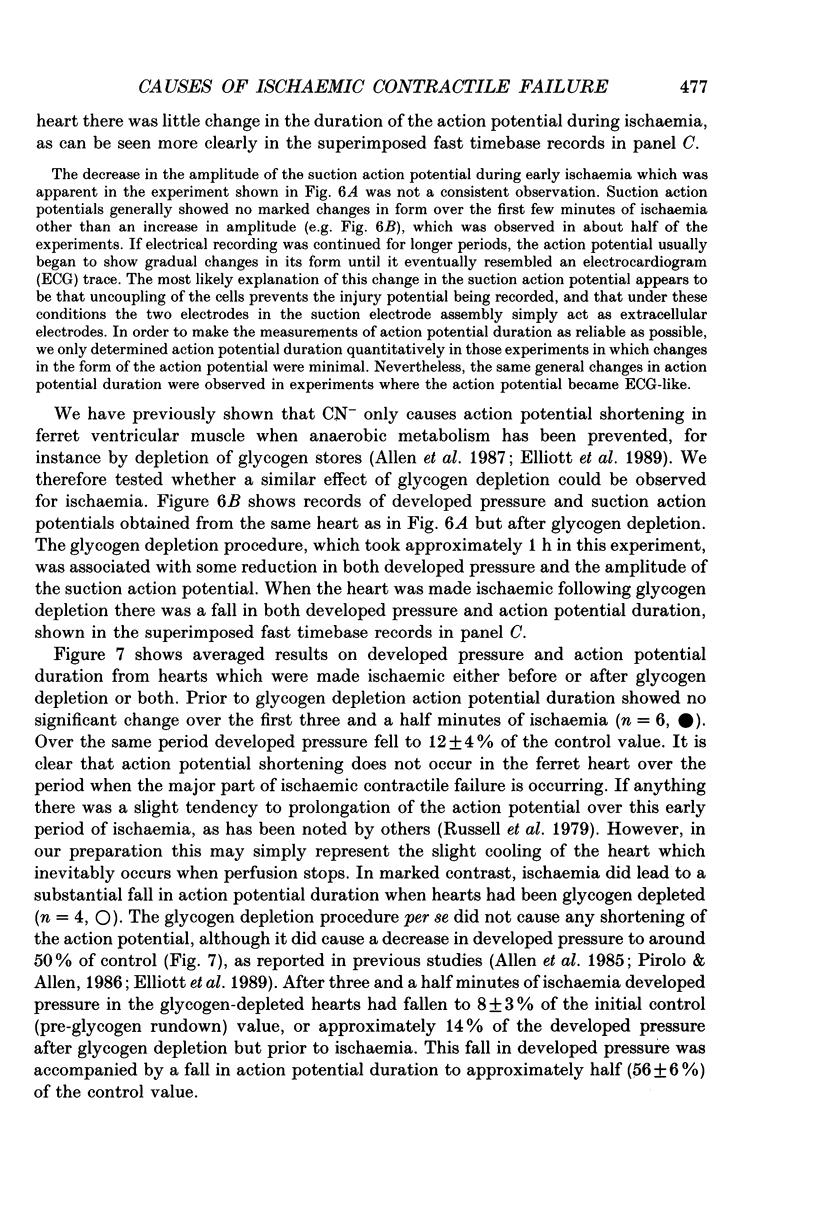
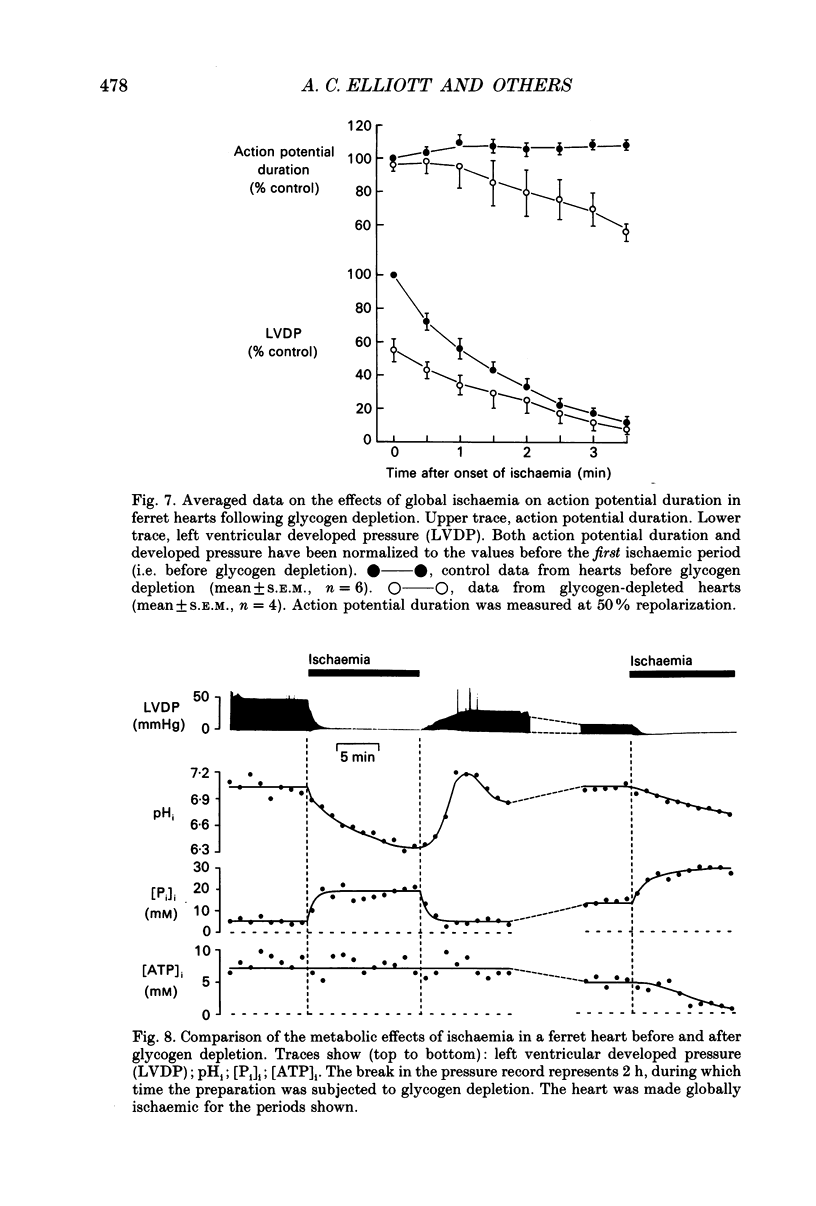
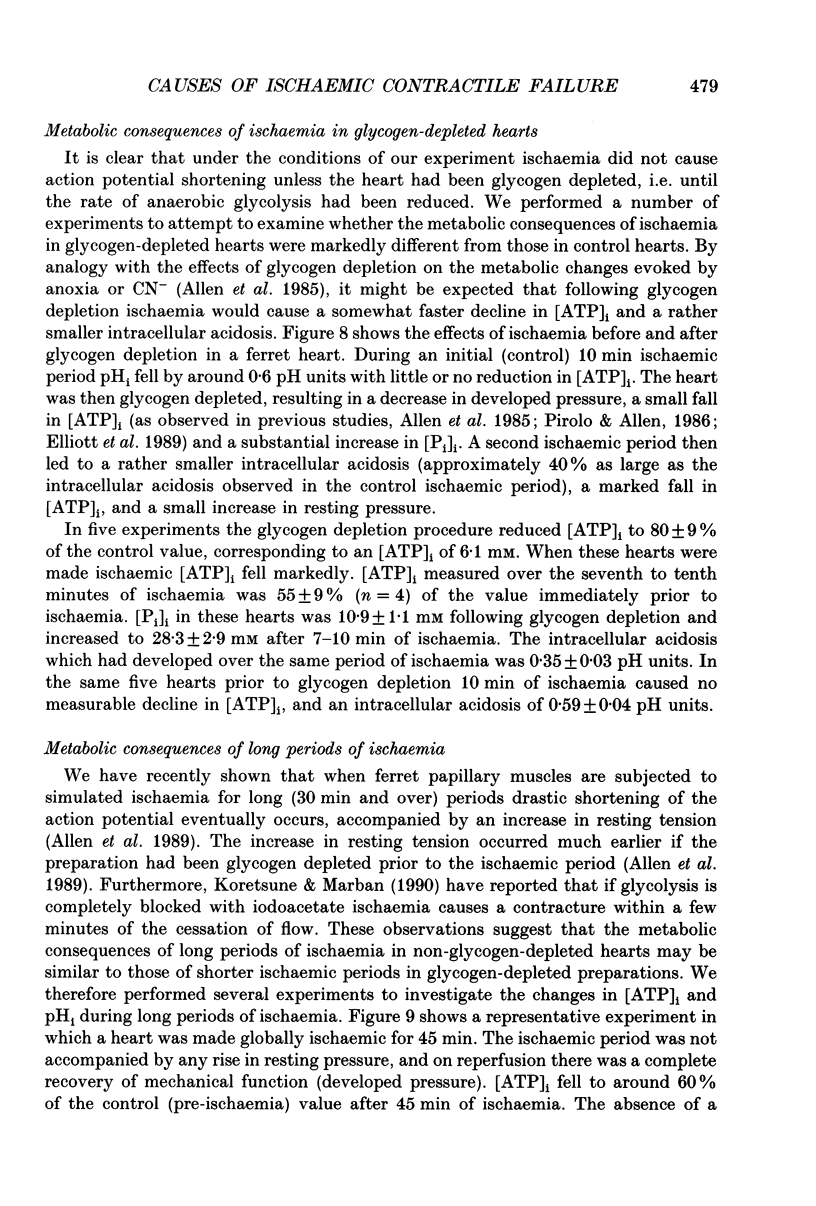
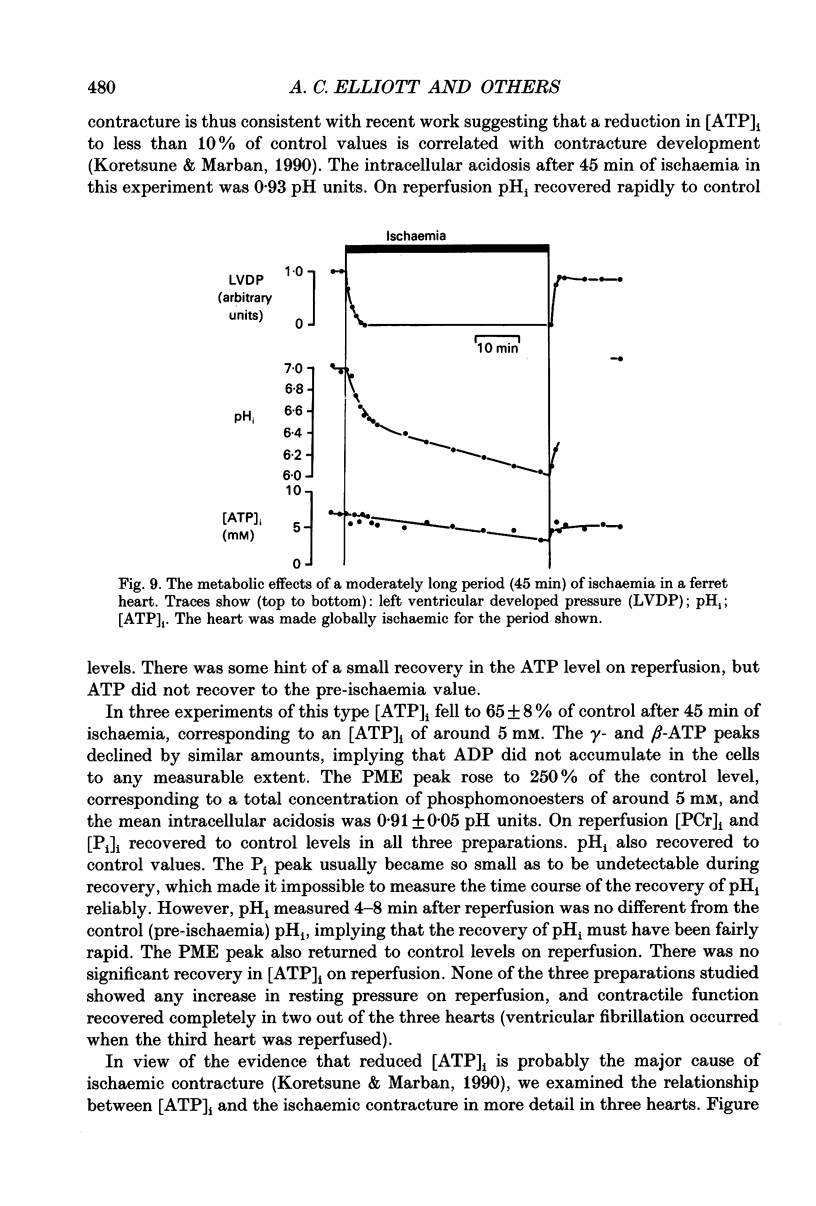
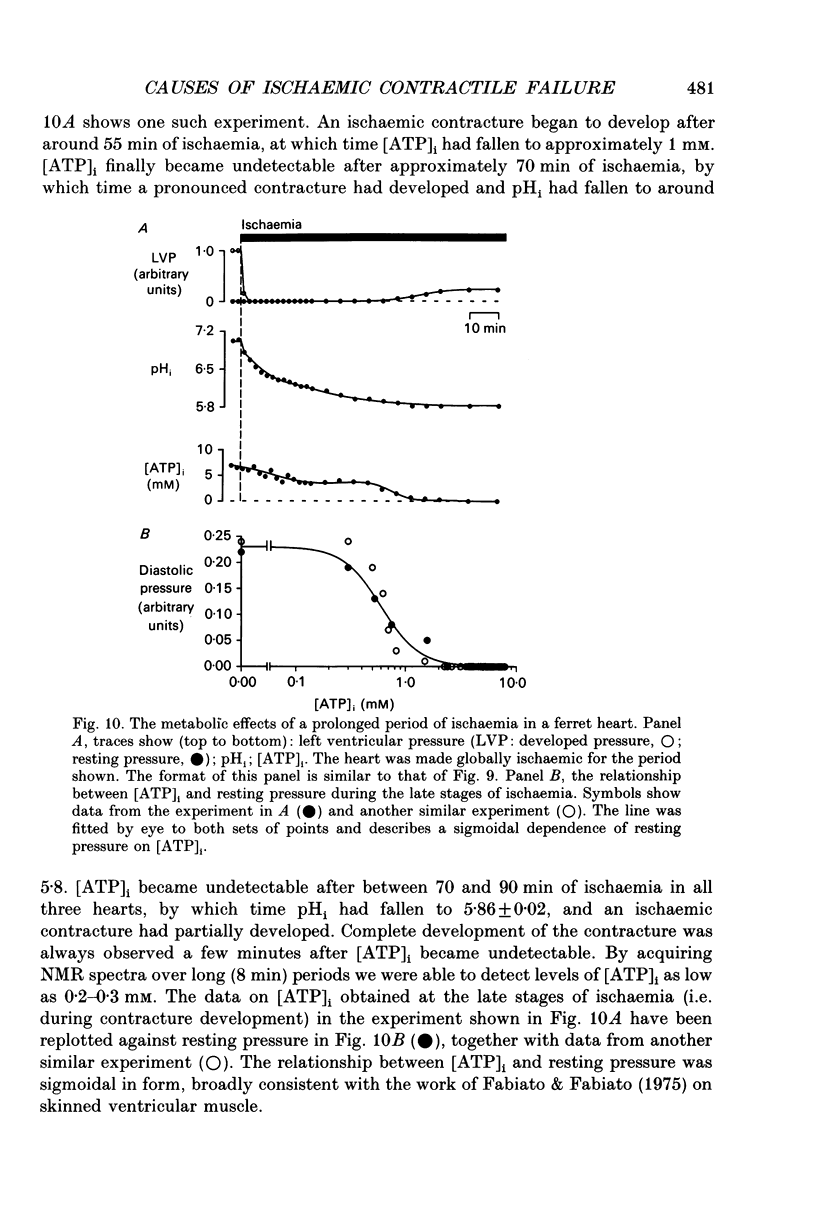
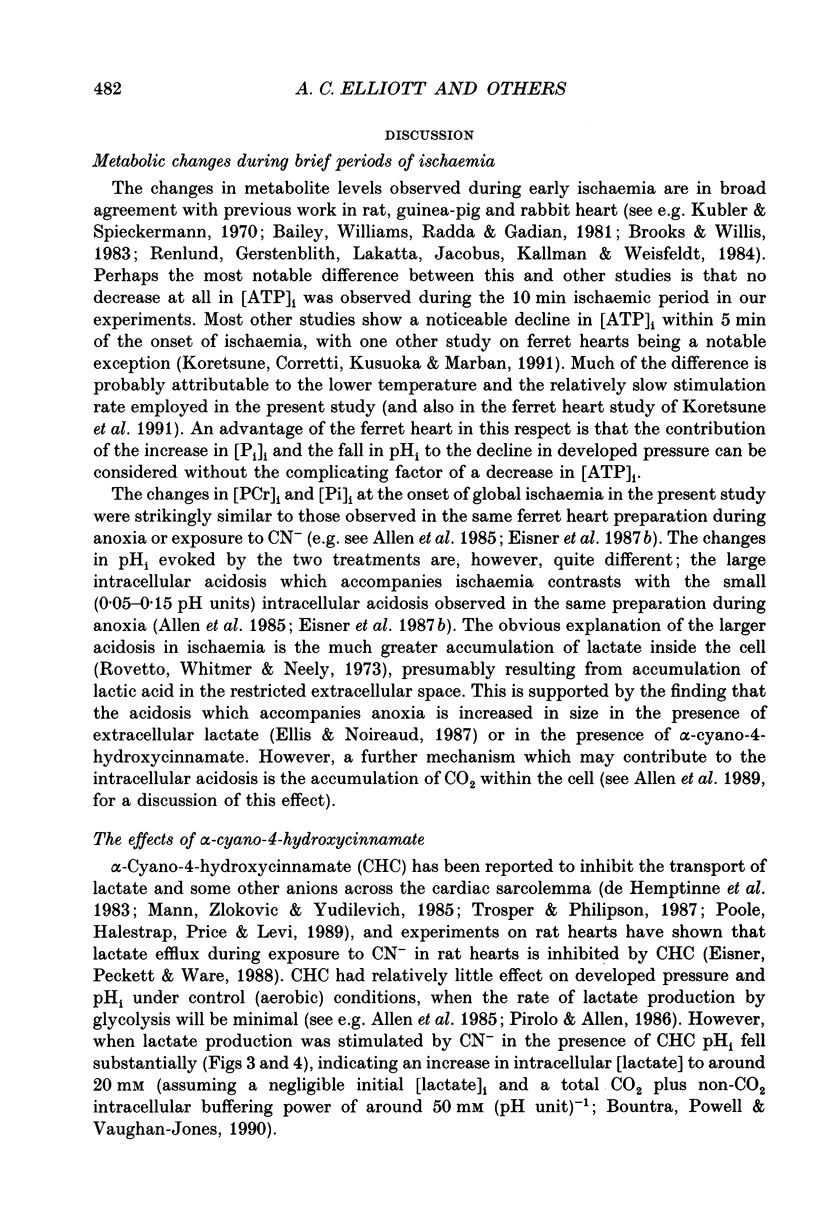
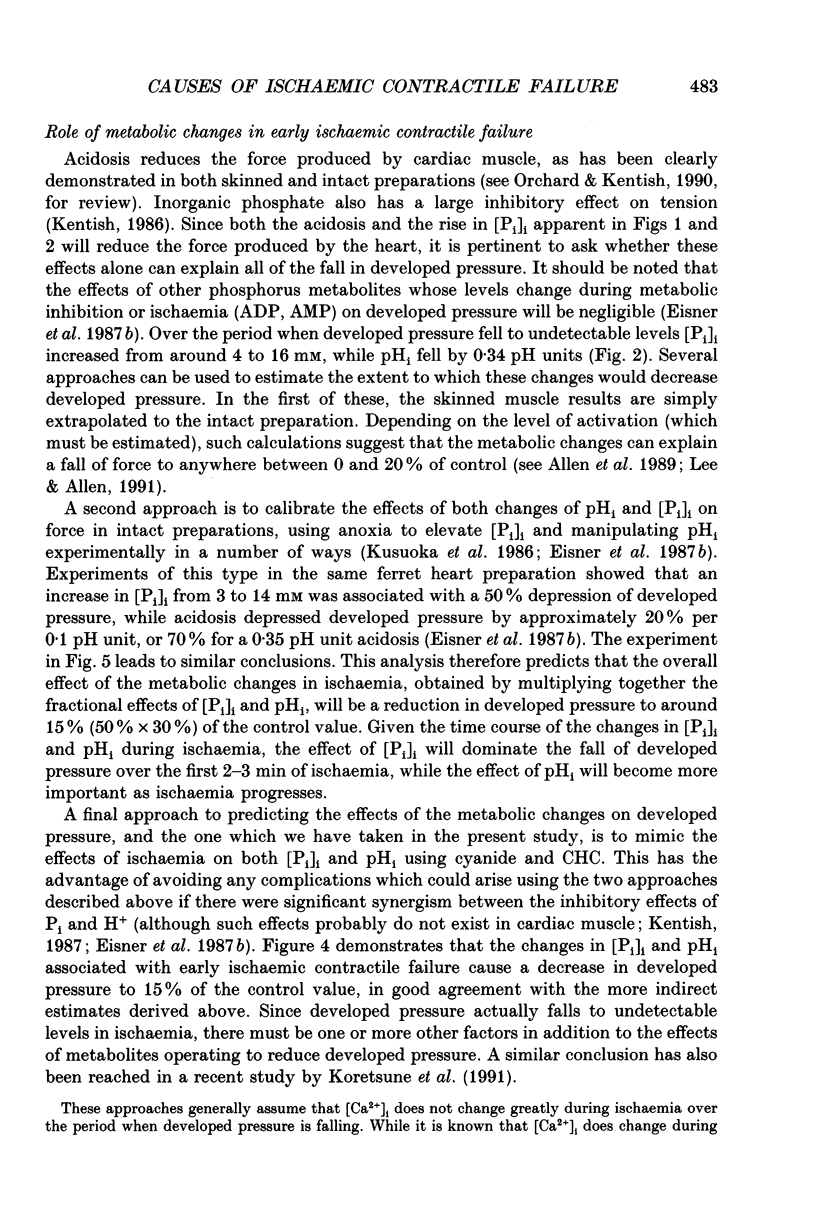
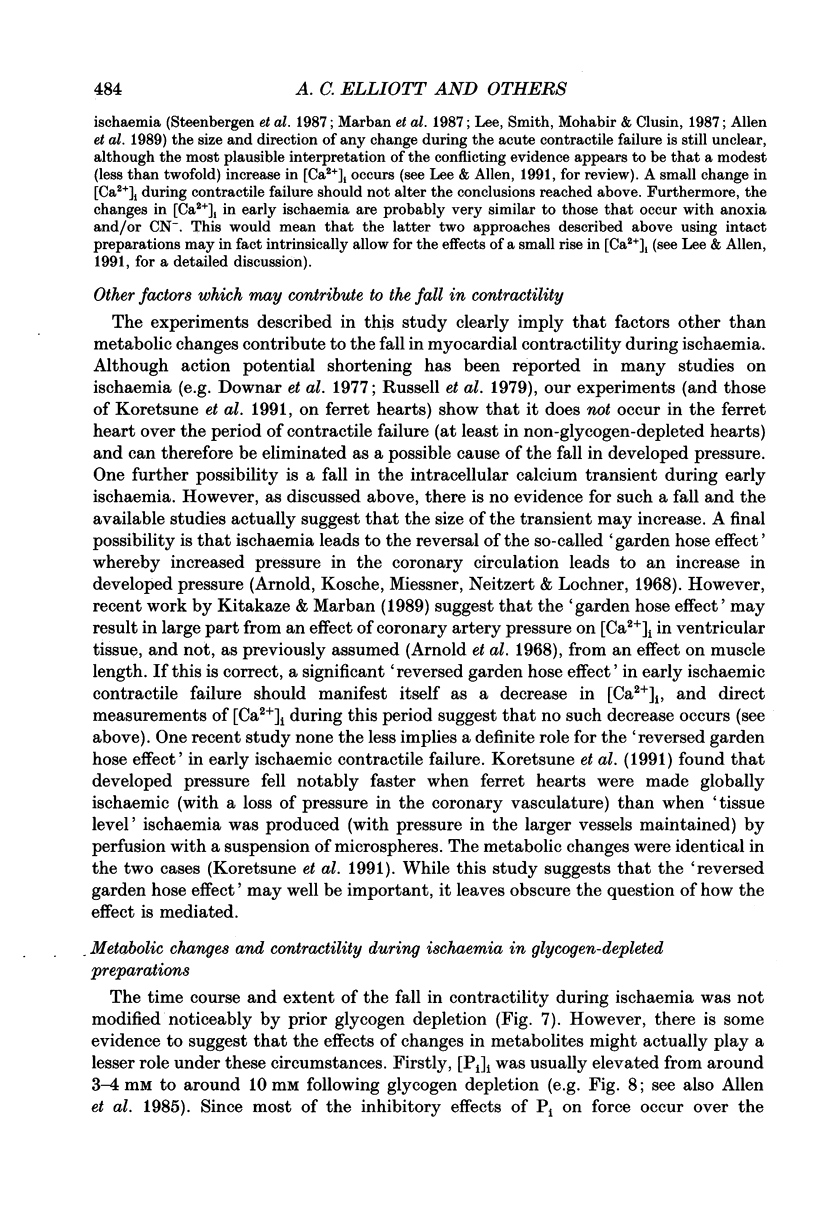
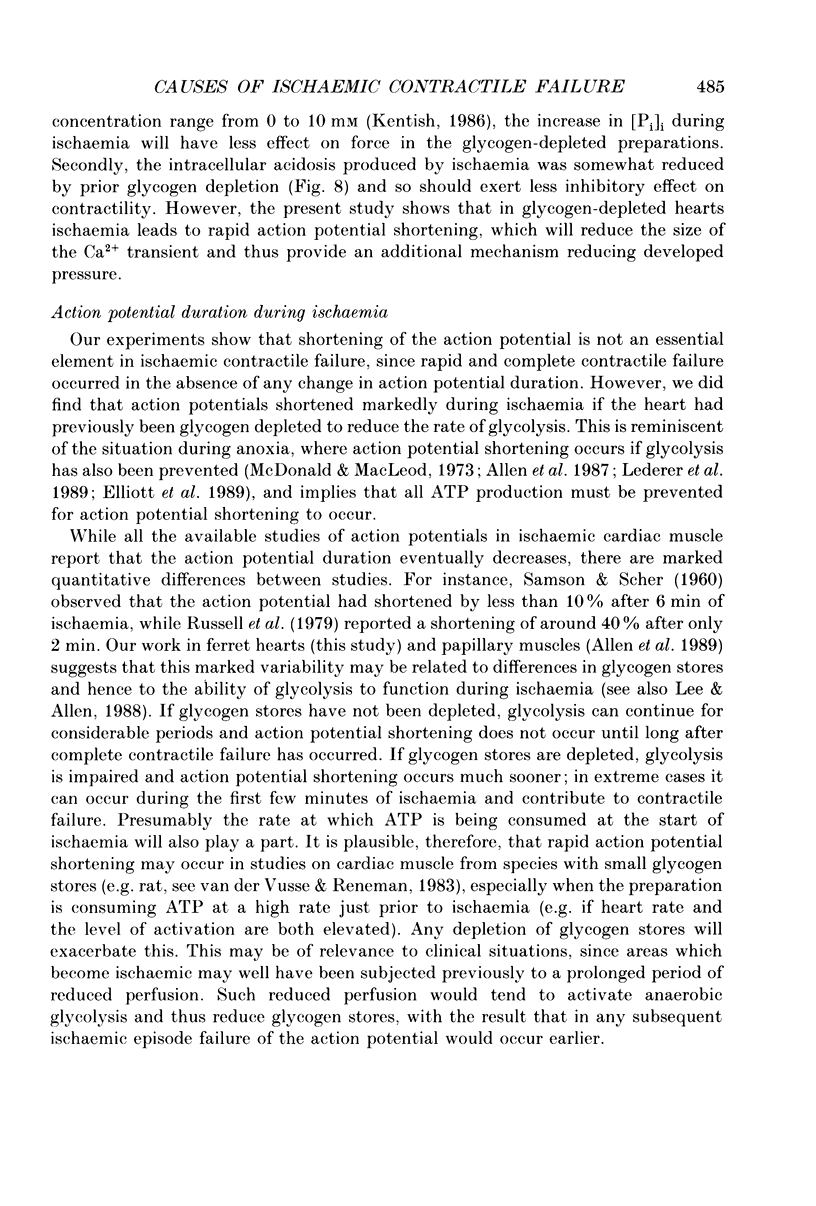
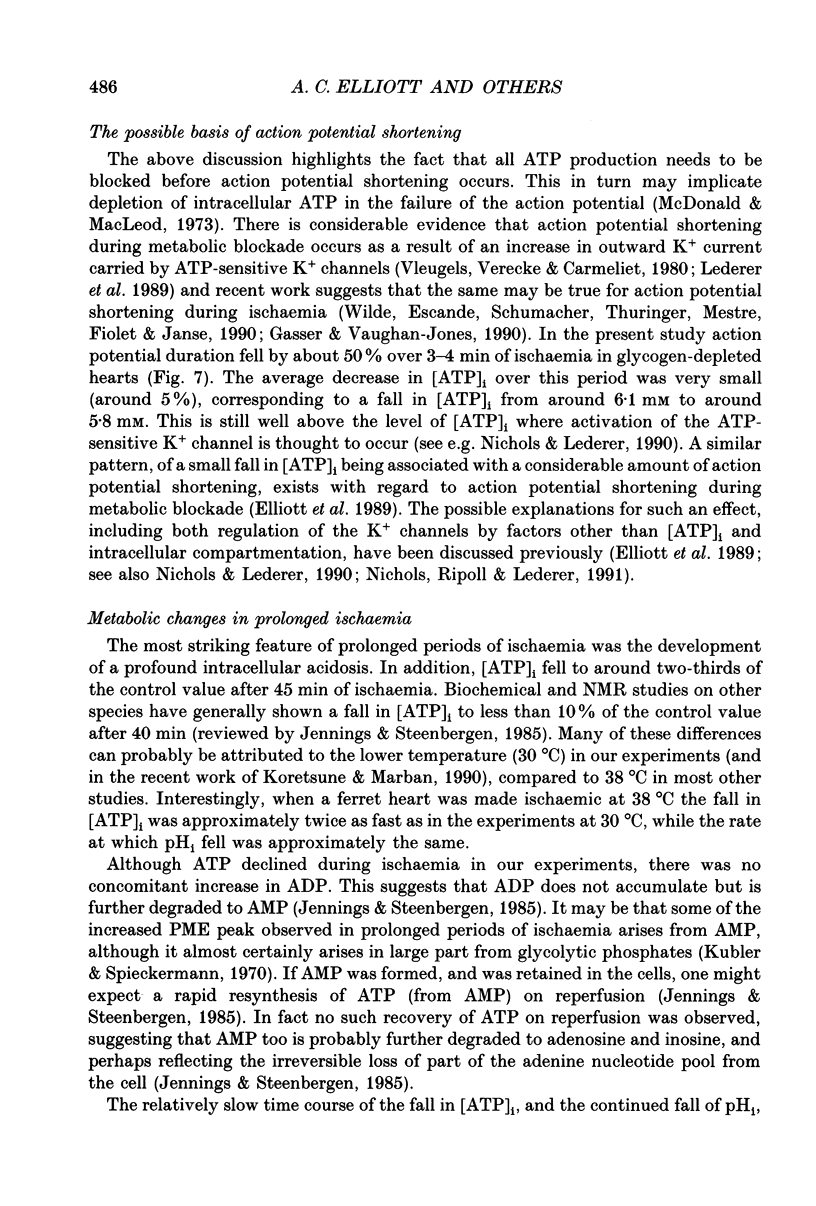
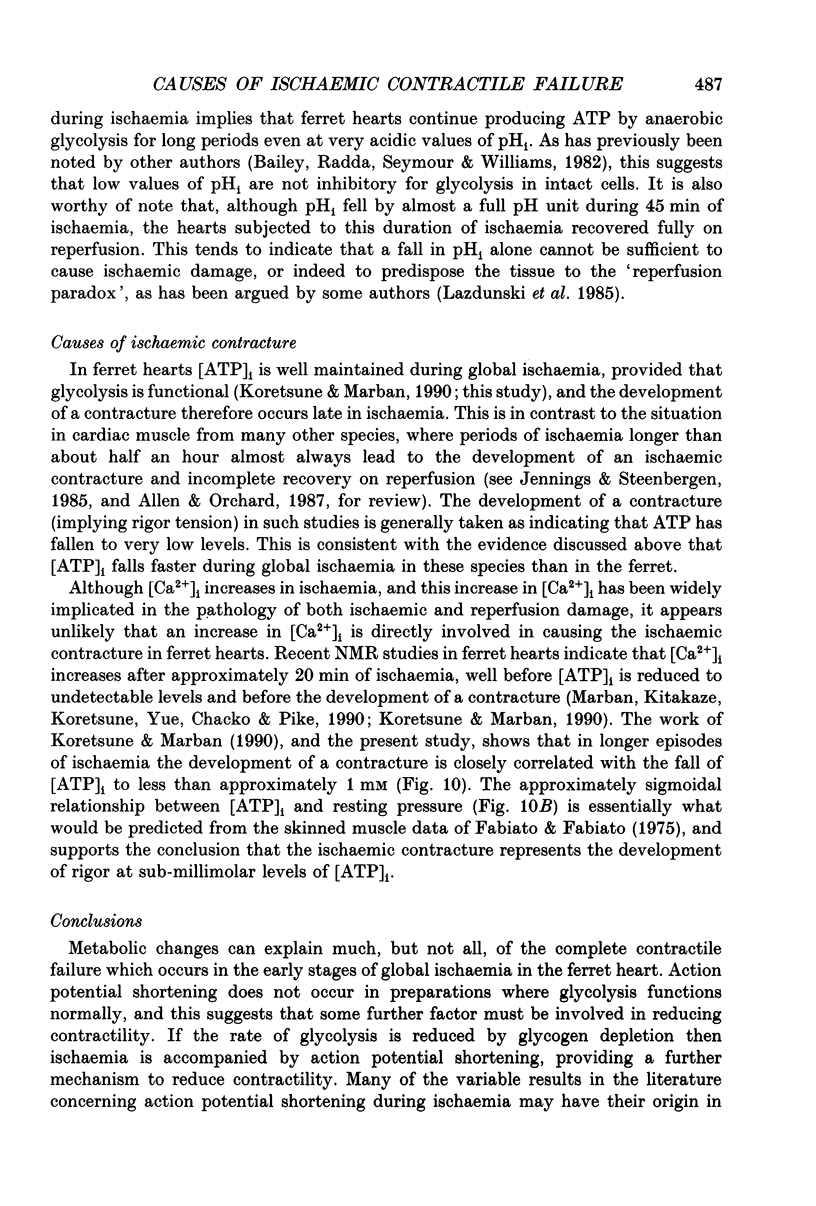
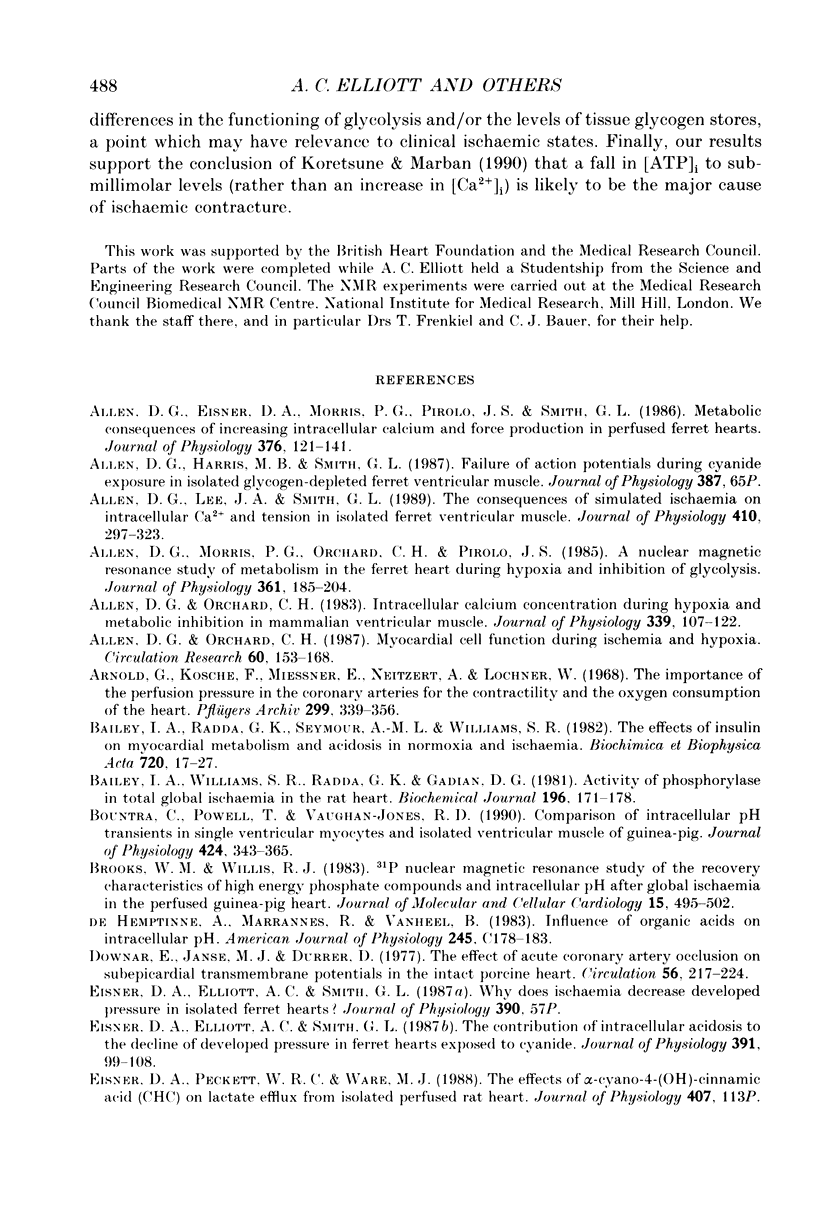
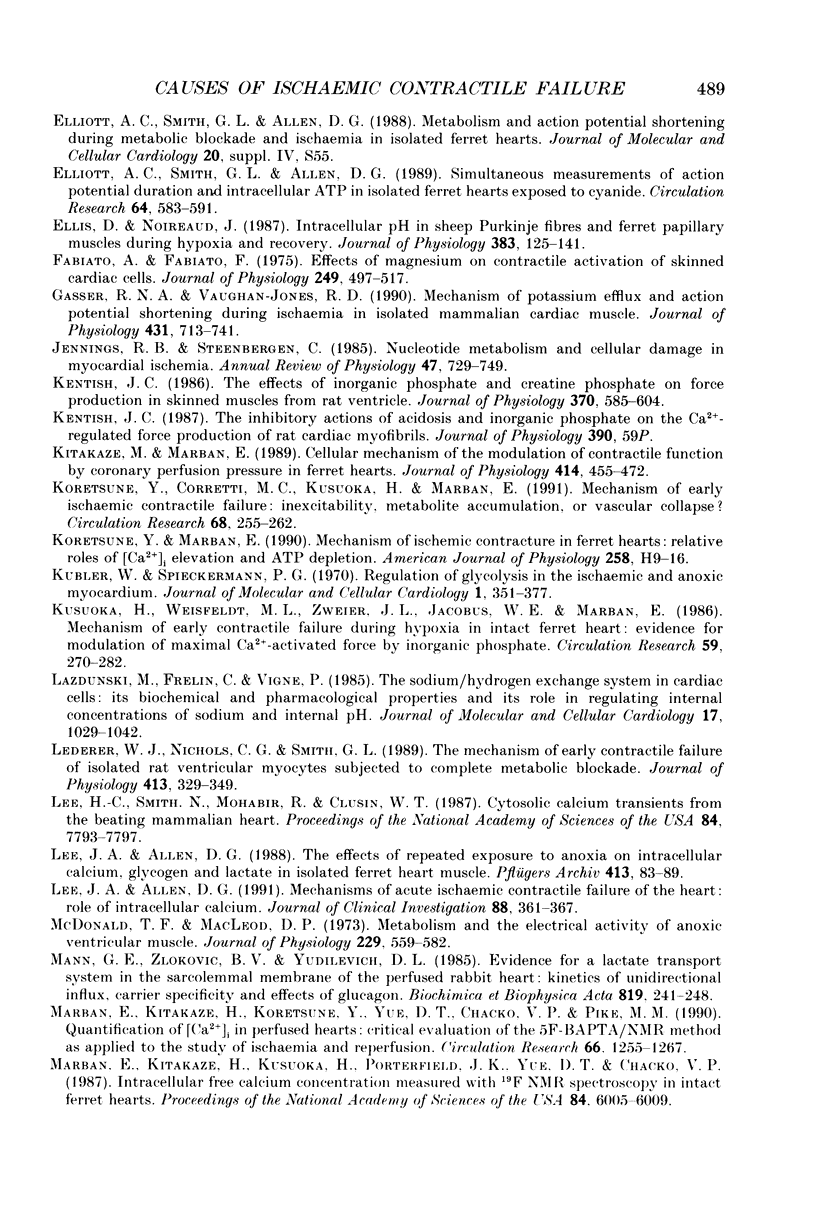
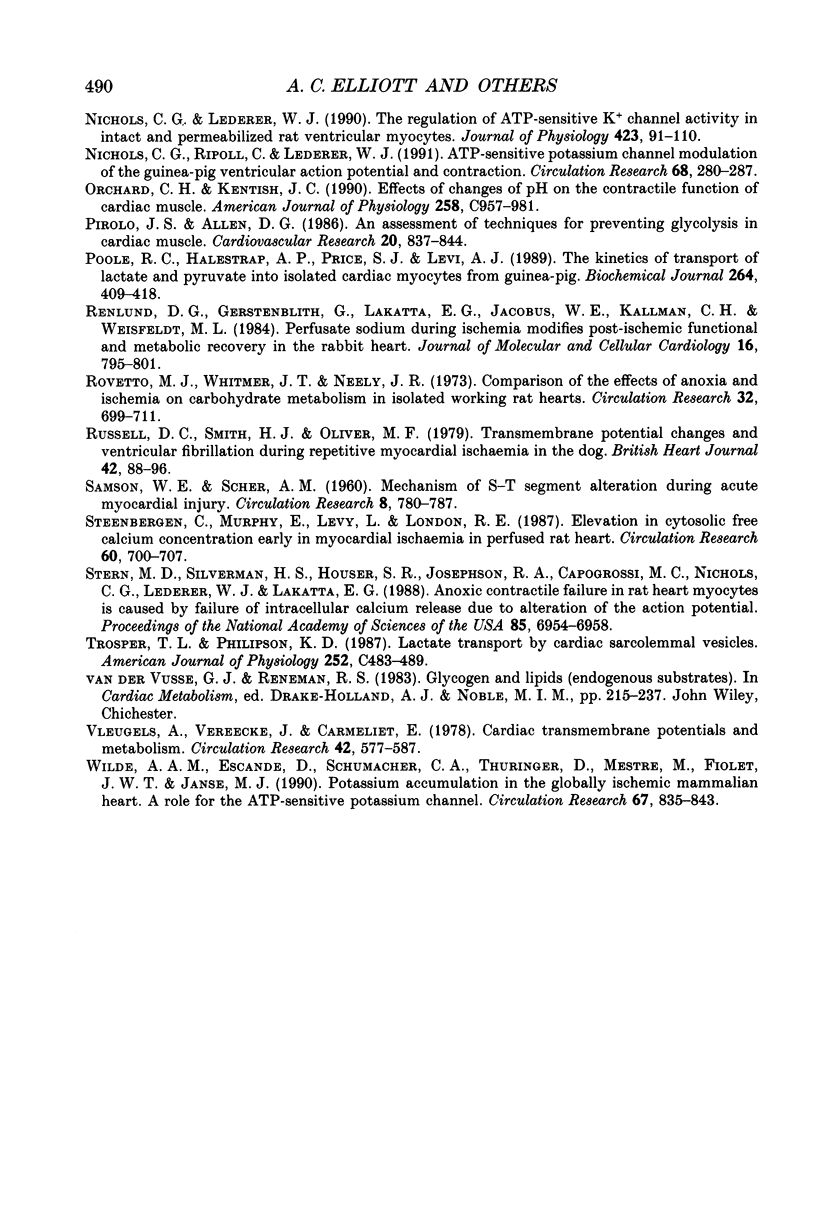
Selected References
These references are in PubMed. This may not be the complete list of references from this article.
- Allen D. G., Eisner D. A., Morris P. G., Pirolo J. S., Smith G. L. Metabolic consequences of increasing intracellular calcium and force production in perfused ferret hearts. J Physiol. 1986 Jul;376:121–141. doi: 10.1113/jphysiol.1986.sp016145. [DOI] [PMC free article] [PubMed] [Google Scholar]
- Allen D. G., Lee J. A., Smith G. L. The consequences of simulated ischaemia on intracellular Ca2+ and tension in isolated ferret ventricular muscle. J Physiol. 1989 Mar;410:297–323. doi: 10.1113/jphysiol.1989.sp017534. [DOI] [PMC free article] [PubMed] [Google Scholar]
- Allen D. G., Morris P. G., Orchard C. H., Pirolo J. S. A nuclear magnetic resonance study of metabolism in the ferret heart during hypoxia and inhibition of glycolysis. J Physiol. 1985 Apr;361:185–204. doi: 10.1113/jphysiol.1985.sp015640. [DOI] [PMC free article] [PubMed] [Google Scholar]
- Allen D. G., Orchard C. H. Intracellular calcium concentration during hypoxia and metabolic inhibition in mammalian ventricular muscle. J Physiol. 1983 Jun;339:107–122. doi: 10.1113/jphysiol.1983.sp014706. [DOI] [PMC free article] [PubMed] [Google Scholar]
- Allen D. G., Orchard C. H. Myocardial contractile function during ischemia and hypoxia. Circ Res. 1987 Feb;60(2):153–168. doi: 10.1161/01.res.60.2.153. [DOI] [PubMed] [Google Scholar]
- Arnold G., Kosche F., Miessner E., Neitzert A., Lochner W. The importance of the perfusion pressure in the coronary arteries for the contractility and the oxygen consumption of the heart. Pflugers Arch Gesamte Physiol Menschen Tiere. 1968;299(4):339–356. doi: 10.1007/BF00602910. [DOI] [PubMed] [Google Scholar]
- Bailey I. A., Radda G. K., Seymour A. M., Williams S. R. The effects of insulin on myocardial metabolism and acidosis in normoxia and ischaemia. A 31P-NMR study. Biochim Biophys Acta. 1982 Feb 10;720(1):17–27. doi: 10.1016/0167-4889(82)90034-9. [DOI] [PubMed] [Google Scholar]
- Bailey I. A., Williams S. R., Radda G. K., Gadian D. G. Activity of phosphorylase in total global ischaemia in the rat heart. A phosphorus-31 nuclear-magnetic-resonance study. Biochem J. 1981 Apr 15;196(1):171–178. doi: 10.1042/bj1960171. [DOI] [PMC free article] [PubMed] [Google Scholar]
- Bountra C., Powell T., Vaughan-Jones R. D. Comparison of intracellular pH transients in single ventricular myocytes and isolated ventricular muscle of guinea-pig. J Physiol. 1990 May;424:343–365. doi: 10.1113/jphysiol.1990.sp018071. [DOI] [PMC free article] [PubMed] [Google Scholar]
- Brooks W. M., Willis R. J. 31P nuclear magnetic resonance study of the recovery characteristics of high energy phosphate compounds and intracellular pH after global ischaemia in the perfused guinea-pig heart. J Mol Cell Cardiol. 1983 Aug;15(8):495–502. doi: 10.1016/0022-2828(83)90325-5. [DOI] [PubMed] [Google Scholar]
- Carmeliet E. Cardiac transmembrane potentials and metabolism. Circ Res. 1978 May;42(5):577–587. doi: 10.1161/01.res.42.5.577. [DOI] [PubMed] [Google Scholar]
- Downar E., Janse M. J., Durrer D. The effect of acute coronary artery occlusion on subepicardial transmembrane potentials in the intact porcine heart. Circulation. 1977 Aug;56(2):217–224. doi: 10.1161/01.cir.56.2.217. [DOI] [PubMed] [Google Scholar]
- Eisner D. A., Elliott A. C., Smith G. L. The contribution of intracellular acidosis to the decline of developed pressure in ferret hearts exposed to cyanide. J Physiol. 1987 Oct;391:99–108. doi: 10.1113/jphysiol.1987.sp016728. [DOI] [PMC free article] [PubMed] [Google Scholar]
- Elliott A. C., Smith G. L., Allen D. G. Simultaneous measurements of action potential duration and intracellular ATP in isolated ferret hearts exposed to cyanide. Circ Res. 1989 Mar;64(3):583–591. doi: 10.1161/01.res.64.3.583. [DOI] [PubMed] [Google Scholar]
- Ellis D., Noireaud J. Intracellular pH in sheep Purkinje fibres and ferret papillary muscles during hypoxia and recovery. J Physiol. 1987 Feb;383:125–141. doi: 10.1113/jphysiol.1987.sp016400. [DOI] [PMC free article] [PubMed] [Google Scholar]
- Fabiato A., Fabiato F. Effects of magnesium on contractile activation of skinned cardiac cells. J Physiol. 1975 Aug;249(3):497–517. doi: 10.1113/jphysiol.1975.sp011027. [DOI] [PMC free article] [PubMed] [Google Scholar]
- Gasser R. N., Vaughan-Jones R. D. Mechanism of potassium efflux and action potential shortening during ischaemia in isolated mammalian cardiac muscle. J Physiol. 1990 Dec;431:713–741. doi: 10.1113/jphysiol.1990.sp018356. [DOI] [PMC free article] [PubMed] [Google Scholar]
- Jennings R. B., Steenbergen C., Jr Nucleotide metabolism and cellular damage in myocardial ischemia. Annu Rev Physiol. 1985;47:727–749. doi: 10.1146/annurev.ph.47.030185.003455. [DOI] [PubMed] [Google Scholar]
- Kentish J. C. The effects of inorganic phosphate and creatine phosphate on force production in skinned muscles from rat ventricle. J Physiol. 1986 Jan;370:585–604. doi: 10.1113/jphysiol.1986.sp015952. [DOI] [PMC free article] [PubMed] [Google Scholar]
- Kitakaze M., Marban E. Cellular mechanism of the modulation of contractile function by coronary perfusion pressure in ferret hearts. J Physiol. 1989 Jul;414:455–472. doi: 10.1113/jphysiol.1989.sp017698. [DOI] [PMC free article] [PubMed] [Google Scholar]
- Koretsune Y., Corretti M. C., Kusuoka H., Marban E. Mechanism of early ischemic contractile failure. Inexcitability, metabolite accumulation, or vascular collapse? Circ Res. 1991 Jan;68(1):255–262. doi: 10.1161/01.res.68.1.255. [DOI] [PubMed] [Google Scholar]
- Koretsune Y., Marban E. Mechanism of ischemic contracture in ferret hearts: relative roles of [Ca2+]i elevation and ATP depletion. Am J Physiol. 1990 Jan;258(1 Pt 2):H9–16. doi: 10.1152/ajpheart.1990.258.1.H9. [DOI] [PubMed] [Google Scholar]
- Kusuoka H., Weisfeldt M. L., Zweier J. L., Jacobus W. E., Marban E. Mechanism of early contractile failure during hypoxia in intact ferret heart: evidence for modulation of maximal Ca2+-activated force by inorganic phosphate. Circ Res. 1986 Sep;59(3):270–282. doi: 10.1161/01.res.59.3.270. [DOI] [PubMed] [Google Scholar]
- Kübler W., Spieckermann P. G. Regulation of glycolysis in the ischemic and the anoxic myocardium. J Mol Cell Cardiol. 1970 Dec;1(4):351–377. doi: 10.1016/0022-2828(70)90034-9. [DOI] [PubMed] [Google Scholar]
- Lazdunski M., Frelin C., Vigne P. The sodium/hydrogen exchange system in cardiac cells: its biochemical and pharmacological properties and its role in regulating internal concentrations of sodium and internal pH. J Mol Cell Cardiol. 1985 Nov;17(11):1029–1042. doi: 10.1016/s0022-2828(85)80119-x. [DOI] [PubMed] [Google Scholar]
- Lederer W. J., Nichols C. G., Smith G. L. The mechanism of early contractile failure of isolated rat ventricular myocytes subjected to complete metabolic inhibition. J Physiol. 1989 Jun;413:329–349. doi: 10.1113/jphysiol.1989.sp017657. [DOI] [PMC free article] [PubMed] [Google Scholar]
- Lee H. C., Smith N., Mohabir R., Clusin W. T. Cytosolic calcium transients from the beating mammalian heart. Proc Natl Acad Sci U S A. 1987 Nov;84(21):7793–7797. doi: 10.1073/pnas.84.21.7793. [DOI] [PMC free article] [PubMed] [Google Scholar]
- Lee J. A., Allen D. G. Mechanisms of acute ischemic contractile failure of the heart. Role of intracellular calcium. J Clin Invest. 1991 Aug;88(2):361–367. doi: 10.1172/JCI115311. [DOI] [PMC free article] [PubMed] [Google Scholar]
- Lee J. A., Allen D. G. The effects of repeated exposure to anoxia on intracellular calcium, glycogen and lactate in isolated ferret heart muscle. Pflugers Arch. 1988 Nov;413(1):83–89. doi: 10.1007/BF00581232. [DOI] [PubMed] [Google Scholar]
- Mann G. E., Zlokovic B. V., Yudilevich D. L. Evidence for a lactate transport system in the sarcolemmal membrane of the perfused rabbit heart: kinetics of unidirectional influx, carrier specificity and effects of glucagon. Biochim Biophys Acta. 1985 Oct 10;819(2):241–248. doi: 10.1016/0005-2736(85)90179-8. [DOI] [PubMed] [Google Scholar]
- Marban E., Kitakaze M., Koretsune Y., Yue D. T., Chacko V. P., Pike M. M. Quantification of [Ca2+]i in perfused hearts. Critical evaluation of the 5F-BAPTA and nuclear magnetic resonance method as applied to the study of ischemia and reperfusion. Circ Res. 1990 May;66(5):1255–1267. doi: 10.1161/01.res.66.5.1255. [DOI] [PubMed] [Google Scholar]
- Marban E., Kitakaze M., Kusuoka H., Porterfield J. K., Yue D. T., Chacko V. P. Intracellular free calcium concentration measured with 19F NMR spectroscopy in intact ferret hearts. Proc Natl Acad Sci U S A. 1987 Aug;84(16):6005–6009. doi: 10.1073/pnas.84.16.6005. [DOI] [PMC free article] [PubMed] [Google Scholar]
- McDonald T. F., MacLeod D. P. Metabolism and the electrical activity of anoxic ventricular muscle. J Physiol. 1973 Mar;229(3):559–582. doi: 10.1113/jphysiol.1973.sp010154. [DOI] [PMC free article] [PubMed] [Google Scholar]
- Nichols C. G., Lederer W. J. The regulation of ATP-sensitive K+ channel activity in intact and permeabilized rat ventricular myocytes. J Physiol. 1990 Apr;423:91–110. doi: 10.1113/jphysiol.1990.sp018013. [DOI] [PMC free article] [PubMed] [Google Scholar]
- Nichols C. G., Ripoll C., Lederer W. J. ATP-sensitive potassium channel modulation of the guinea pig ventricular action potential and contraction. Circ Res. 1991 Jan;68(1):280–287. doi: 10.1161/01.res.68.1.280. [DOI] [PubMed] [Google Scholar]
- Pirolo J. S., Allen D. G. Assessment of techniques for preventing glycolysis in cardiac muscle. Cardiovasc Res. 1986 Nov;20(11):837–844. doi: 10.1093/cvr/20.11.837. [DOI] [PubMed] [Google Scholar]
- Poole R. C., Halestrap A. P., Price S. J., Levi A. J. The kinetics of transport of lactate and pyruvate into isolated cardiac myocytes from guinea pig. Kinetic evidence for the presence of a carrier distinct from that in erythrocytes and hepatocytes. Biochem J. 1989 Dec 1;264(2):409–418. doi: 10.1042/bj2640409. [DOI] [PMC free article] [PubMed] [Google Scholar]
- Renlund D. G., Gerstenblith G., Lakatta E. G., Jacobus W. E., Kallman C. H., Weisfeldt M. L. Perfusate sodium during ischemia modifies post-ischemic functional and metabolic recovery in the rabbit heart. J Mol Cell Cardiol. 1984 Sep;16(9):795–801. doi: 10.1016/s0022-2828(84)80003-6. [DOI] [PubMed] [Google Scholar]
- Rovetto M. J., Whitmer J. T., Neely J. R. Comparison of the effects of anoxia and whole heart ischemia on carbohydrate utilization in isolated working rat hearts. Circ Res. 1973 Jun;32(6):699–711. doi: 10.1161/01.res.32.6.699. [DOI] [PubMed] [Google Scholar]
- Russell D. C., Smith J. H., Oliver M. F. Transmembrane potential changes and ventricular fibrillation during repetitive myocardial ischaemia in the dog. Br Heart J. 1979 Jul;42(1):88–96. doi: 10.1136/hrt.42.1.88. [DOI] [PMC free article] [PubMed] [Google Scholar]
- SAMSON W. E., SCHER A. M. Mechanism of S-T segment alteration during acute myocardial injury. Circ Res. 1960 Jul;8:780–787. doi: 10.1161/01.res.8.4.780. [DOI] [PubMed] [Google Scholar]
- Steenbergen C., Murphy E., Levy L., London R. E. Elevation in cytosolic free calcium concentration early in myocardial ischemia in perfused rat heart. Circ Res. 1987 May;60(5):700–707. doi: 10.1161/01.res.60.5.700. [DOI] [PubMed] [Google Scholar]
- Stern M. D., Silverman H. S., Houser S. R., Josephson R. A., Capogrossi M. C., Nichols C. G., Lederer W. J., Lakatta E. G. Anoxic contractile failure in rat heart myocytes is caused by failure of intracellular calcium release due to alteration of the action potential. Proc Natl Acad Sci U S A. 1988 Sep;85(18):6954–6958. doi: 10.1073/pnas.85.18.6954. [DOI] [PMC free article] [PubMed] [Google Scholar]
- Trosper T. L., Philipson K. D. Lactate transport by cardiac sarcolemmal vesicles. Am J Physiol. 1987 May;252(5 Pt 1):C483–C489. doi: 10.1152/ajpcell.1987.252.5.C483. [DOI] [PubMed] [Google Scholar]
- Wilde A. A., Escande D., Schumacher C. A., Thuringer D., Mestre M., Fiolet J. W., Janse M. J. Potassium accumulation in the globally ischemic mammalian heart. A role for the ATP-sensitive potassium channel. Circ Res. 1990 Oct;67(4):835–843. doi: 10.1161/01.res.67.4.835. [DOI] [PubMed] [Google Scholar]