Abstract
1. Electrical slow waves of gastrointestinal smooth muscles are not abolished by organic Ca2+ channel blocking drugs, such as nifedipine or D600. These compounds reduce the amplitude and duration of the plateau phase, but the upstroke phase of slow waves persists. 2. Voltage clamp experiments were performed on isolated circular muscle cells from the canine proximal colon to characterize the dihydropyridine-resistant component of inward current. Inward currents were measured at 25 and 35 degrees C. The higher temperature increased the amplitudes of the transient and sustained phases of the inward current. The voltage dependence of activation and inactivation of the inward current was not significantly changed at 35 vs. 25 degrees C. 3. At 35 degrees C the transient phase of the inward current was reduced but not blocked by nifedipine (10(-6) M). The sustained phase was blocked by nifedipine. 4. The block by nifedipine was voltage dependent, increasing with depolarization. At voltages reached during the upstroke depolarization about 35% of the inward current persisted in the presence of nifedipine (10(-6) M). This may be sufficient inward current to sustain the upstroke depolarization in intact muscles. 5. Nifedipine caused a 20 mV negative shift in the voltage dependence of inactivation suggesting that dihydropyridines may preferentially bind to Ca2+ channels in an inactivated state. 6. Ni2+ (< 100 microM) significantly decreased the transient phase of inward current. A combination of Ni2+ (40 microM) and nifedipine (10(-6) M) blocked all of the inward current at 35 degrees C. Combination of nifedipine (10(-6) M) and Ni2+ (40 microM) blocked slow waves in intact muscles. 7. Bay K 8644 (10(-6) M) increased the amplitude of the transient and sustained components of inward current. On a percentage basis the increase in the sustained component was greater than the increase in the transient component with test potentials in the range of -50 to -20 mV. This may explain why Bay K 8644 preferentially increases the plateau component of slow waves vs. the upstroke component. 8. The findings of this study suggest that the nifedipine resistance of the upstroke depolarization could be due to the voltage dependence of the block of Ca2+ channels by dihydropyridines. Thus a single class of voltage-dependent Ca2+ channels could be responsible for the upstroke and plateau phases of slow waves.
Full text
PDF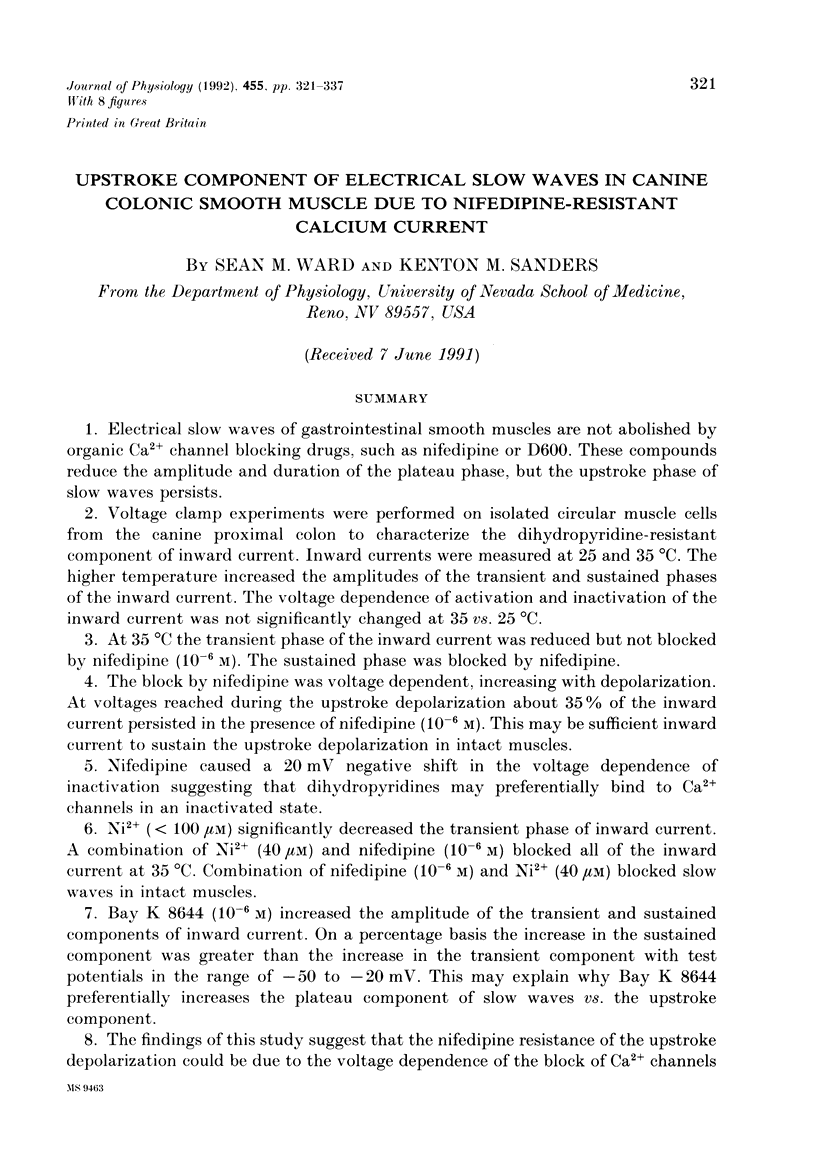
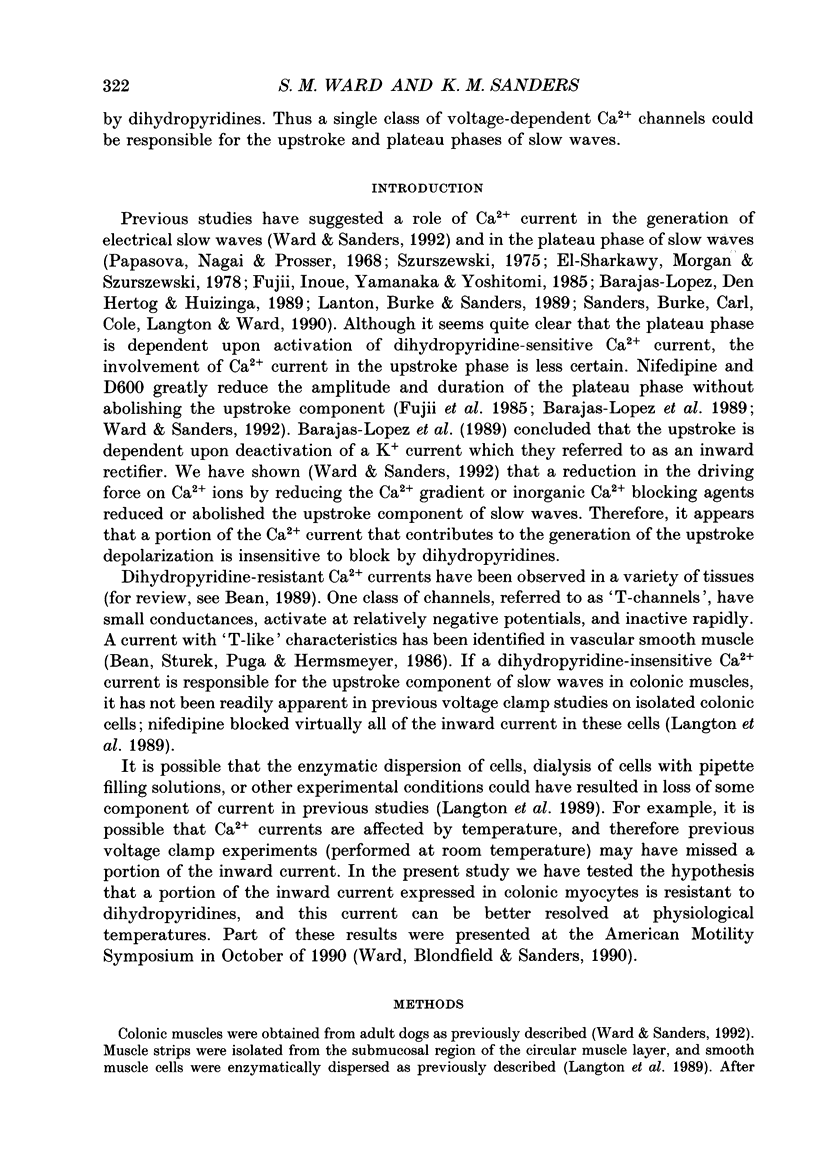
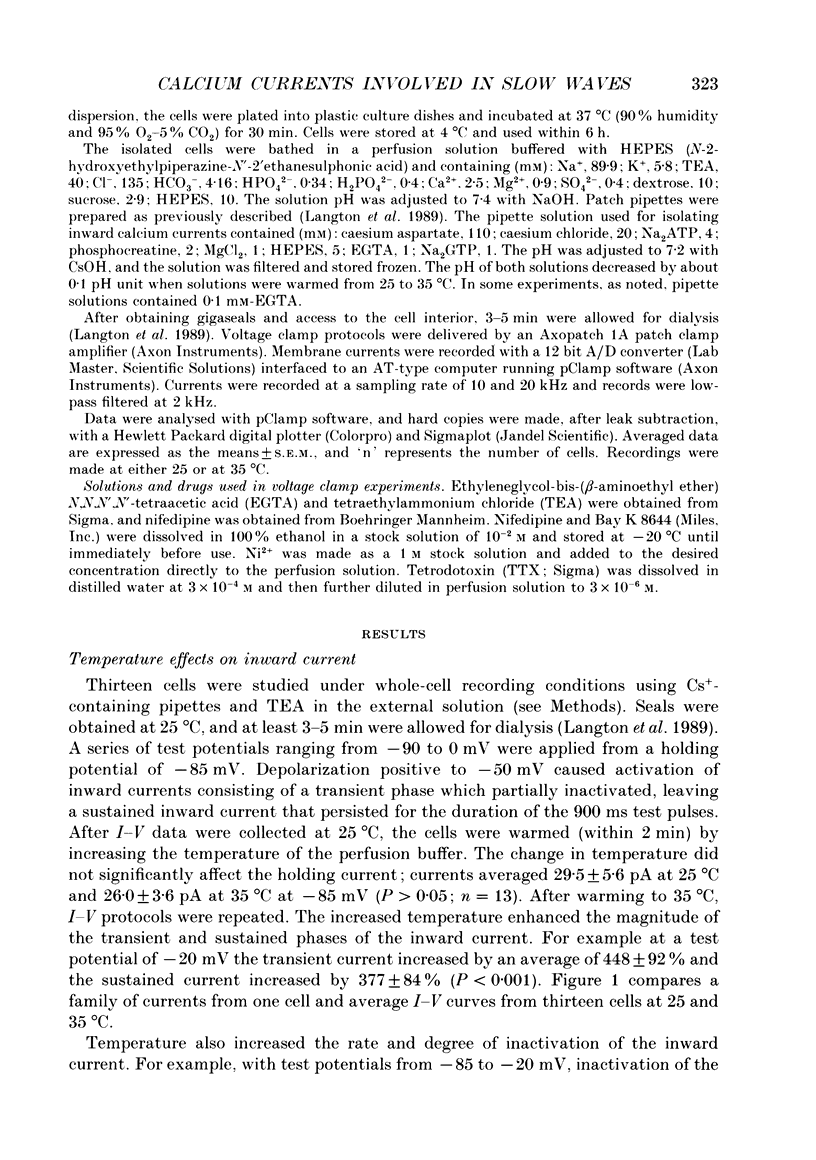
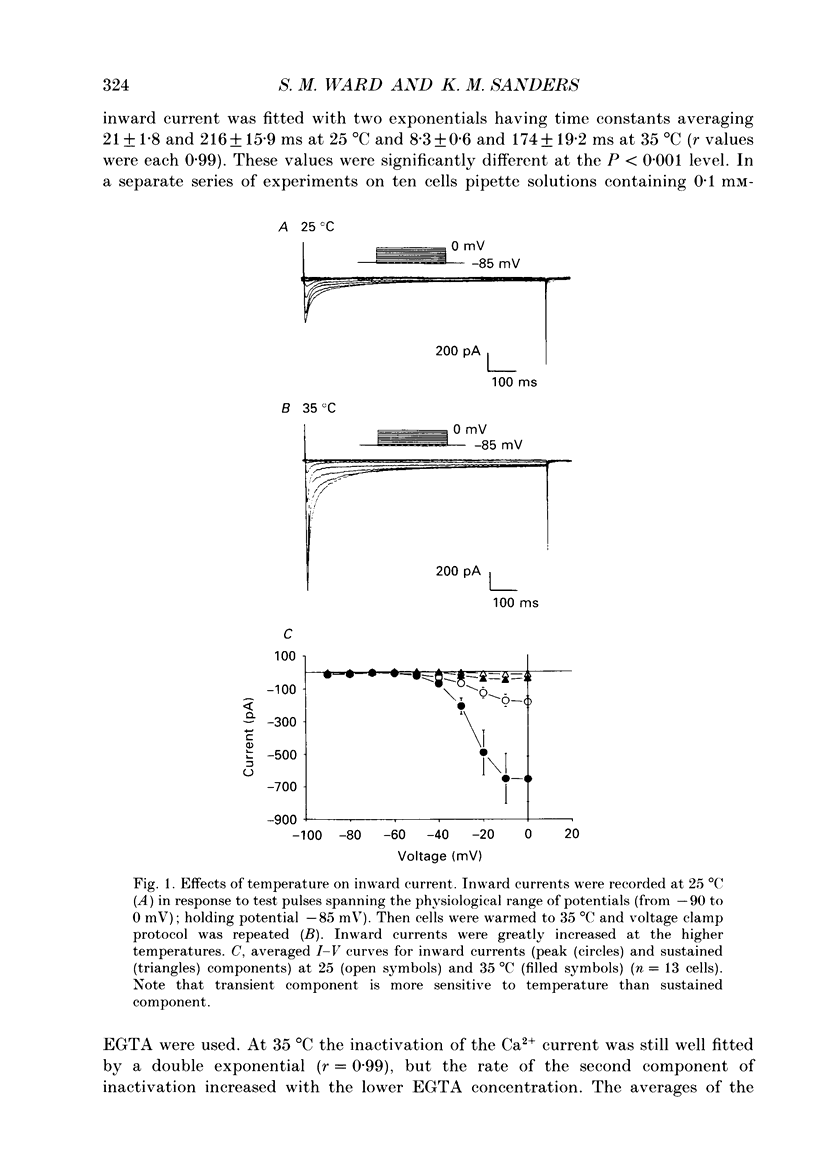
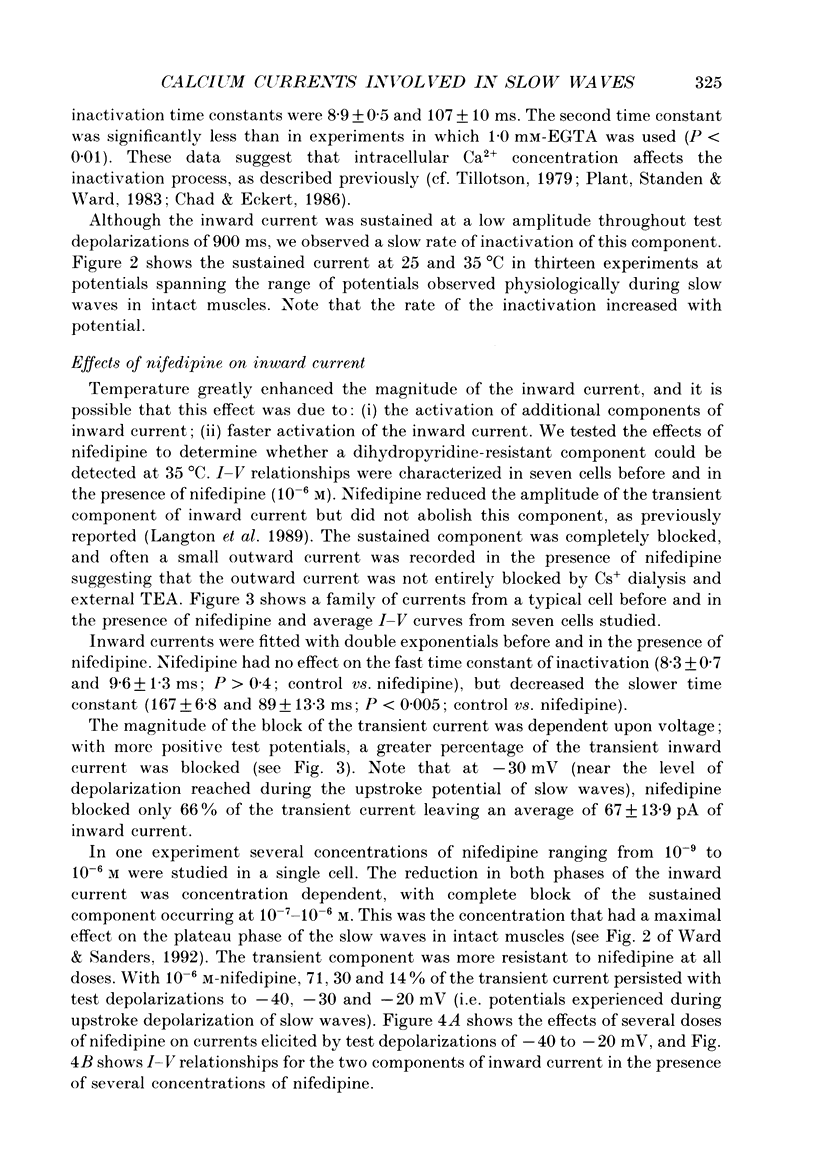
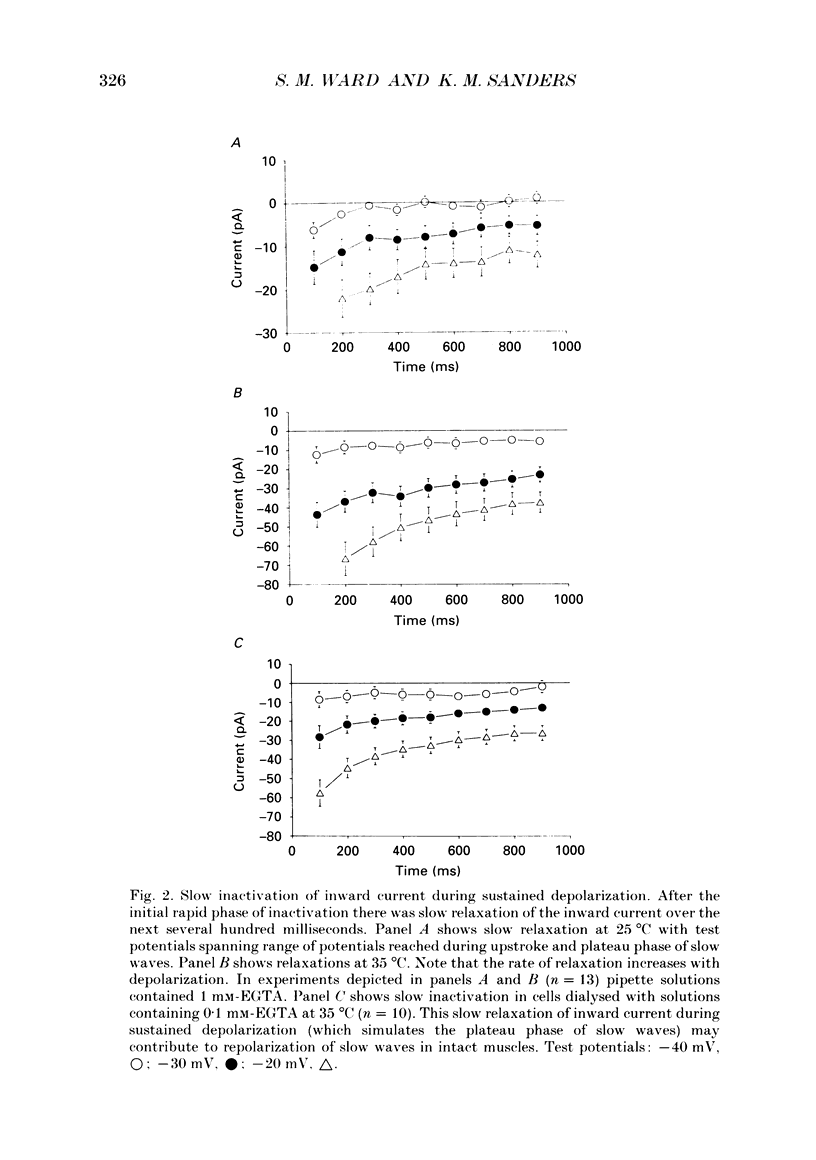
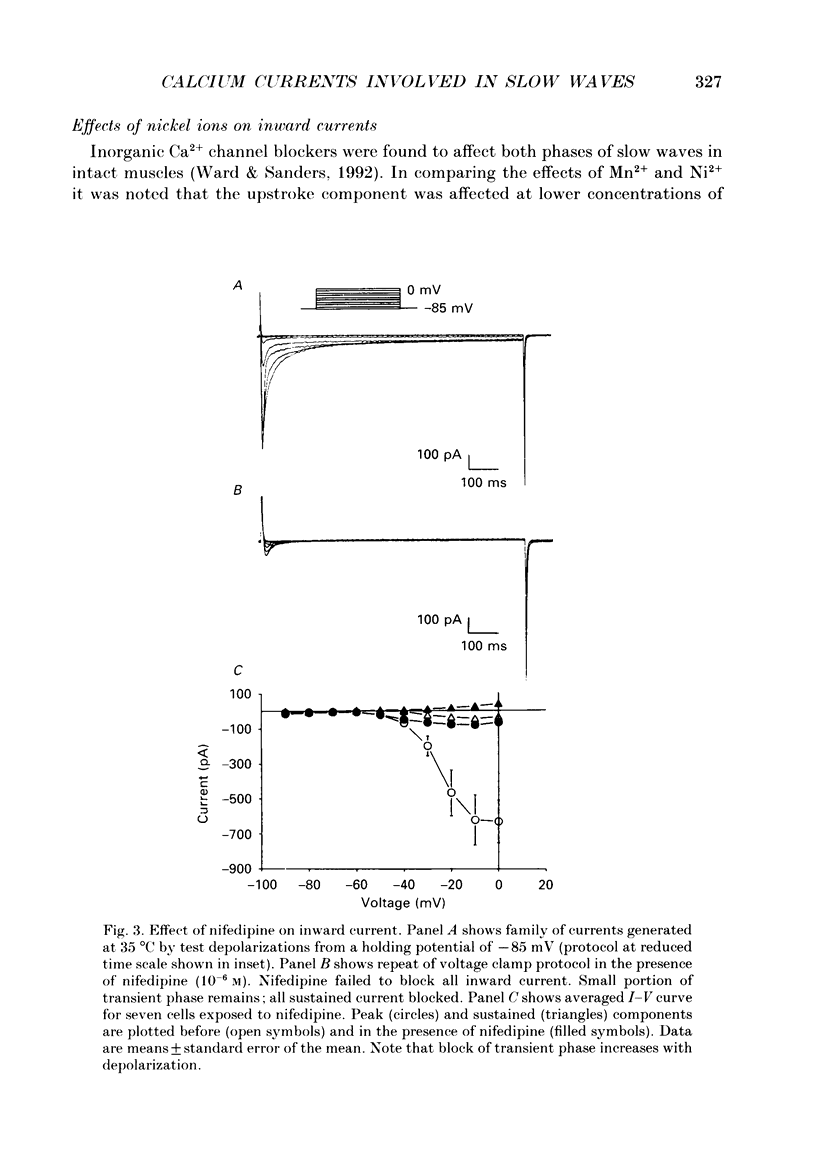
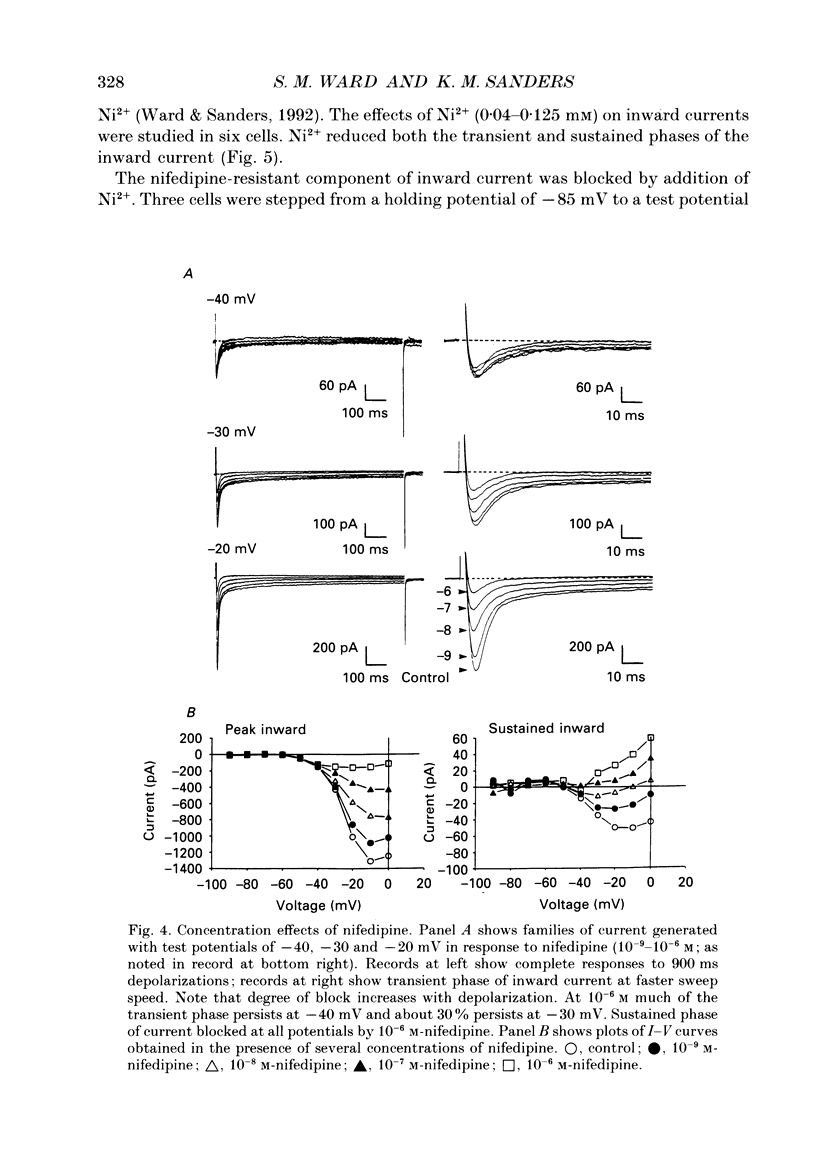
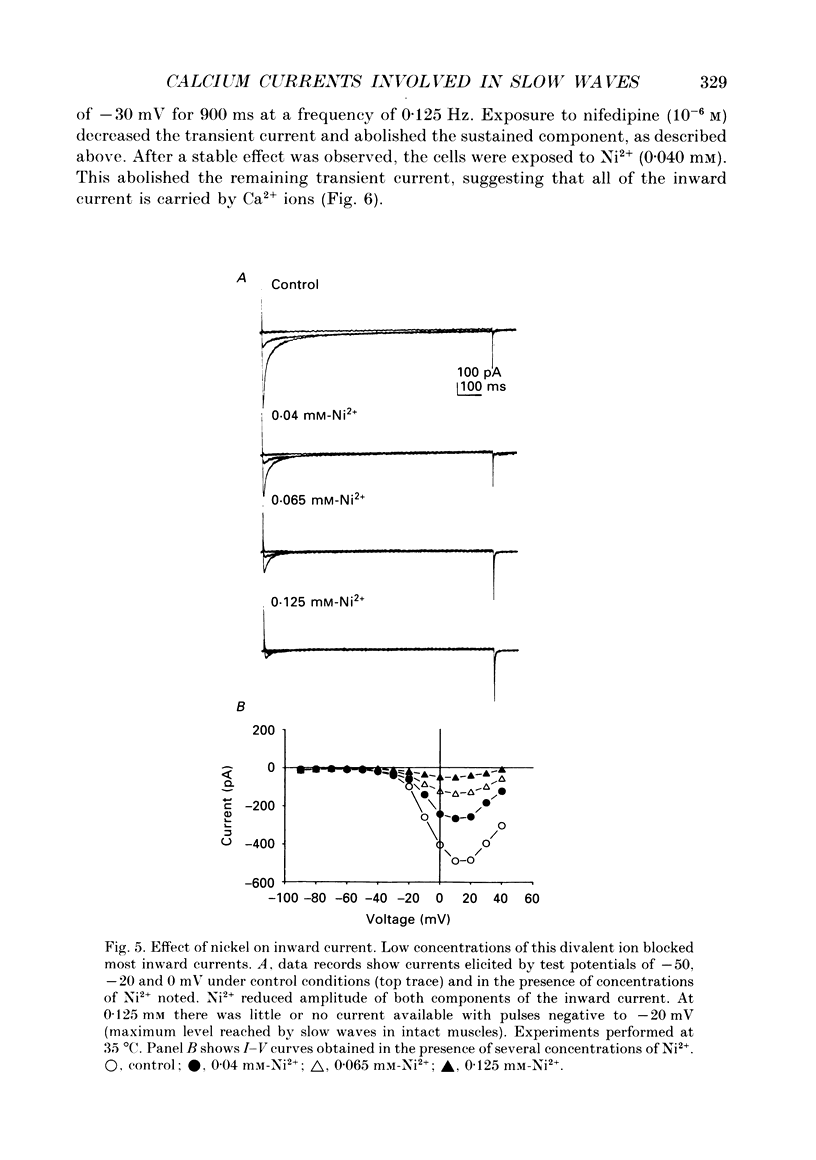
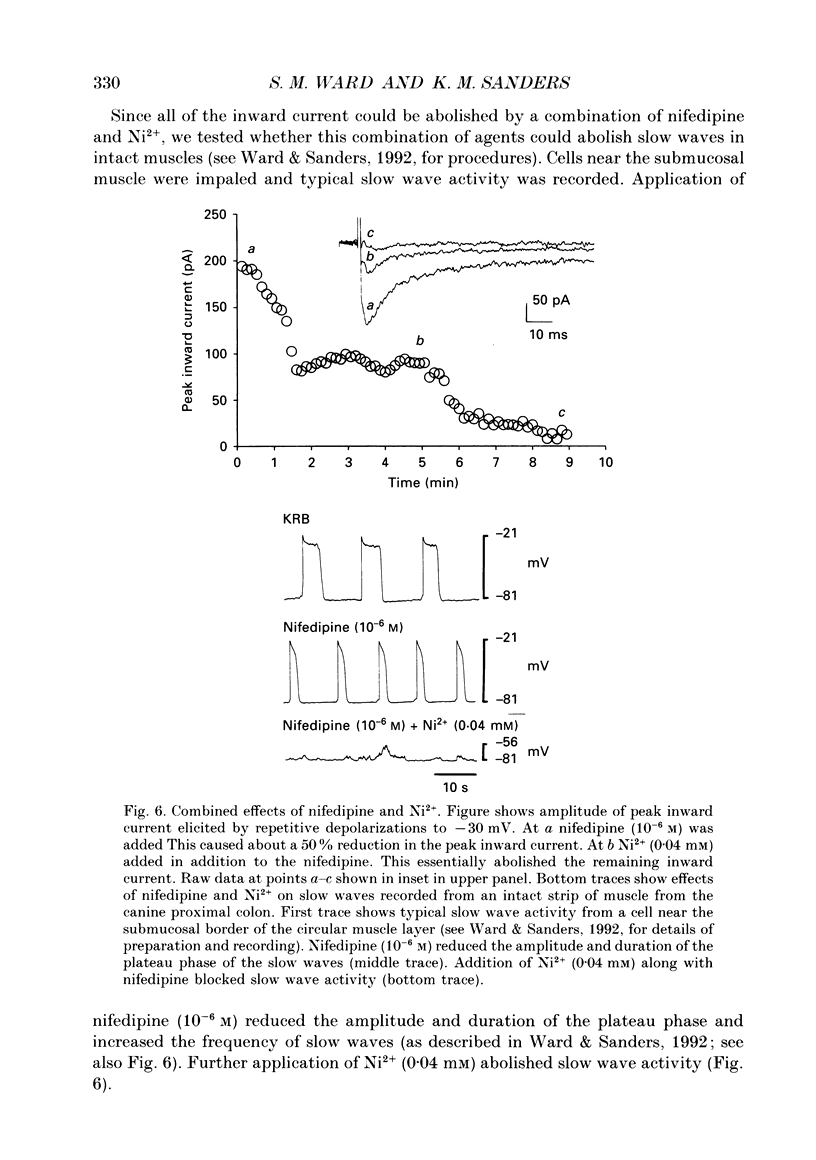
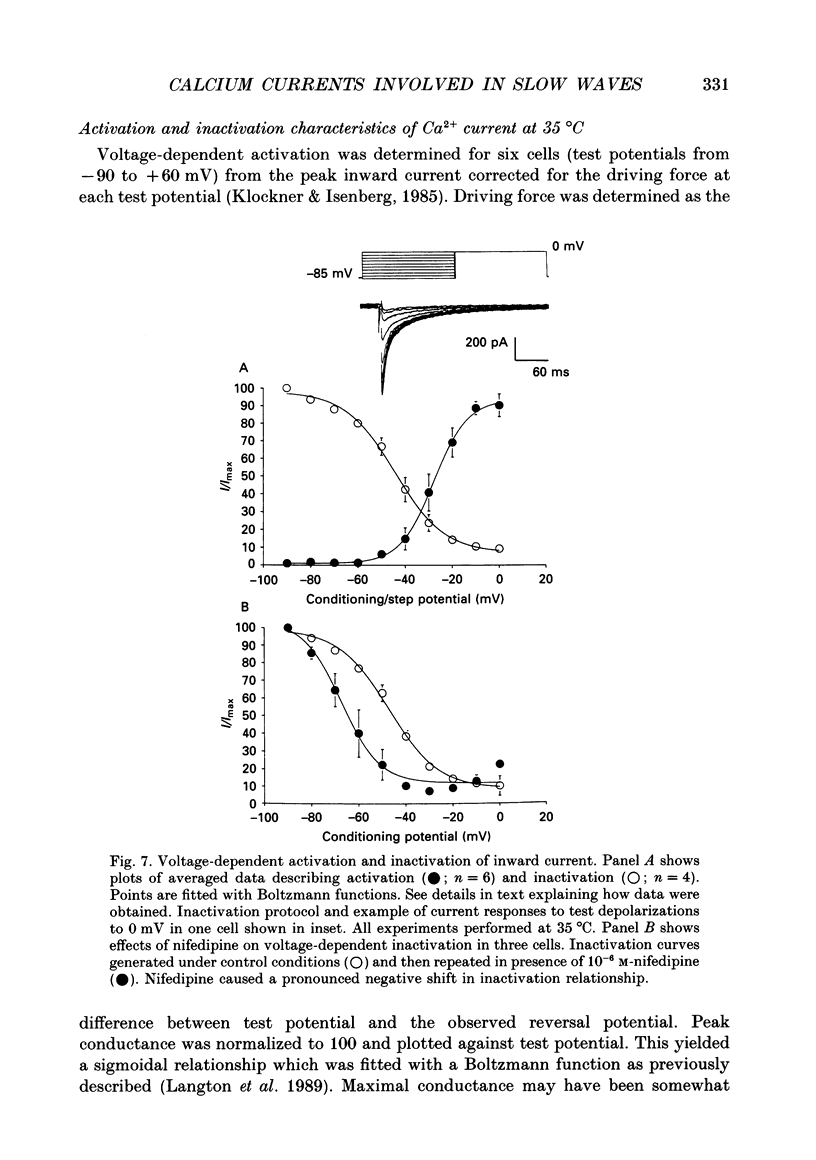
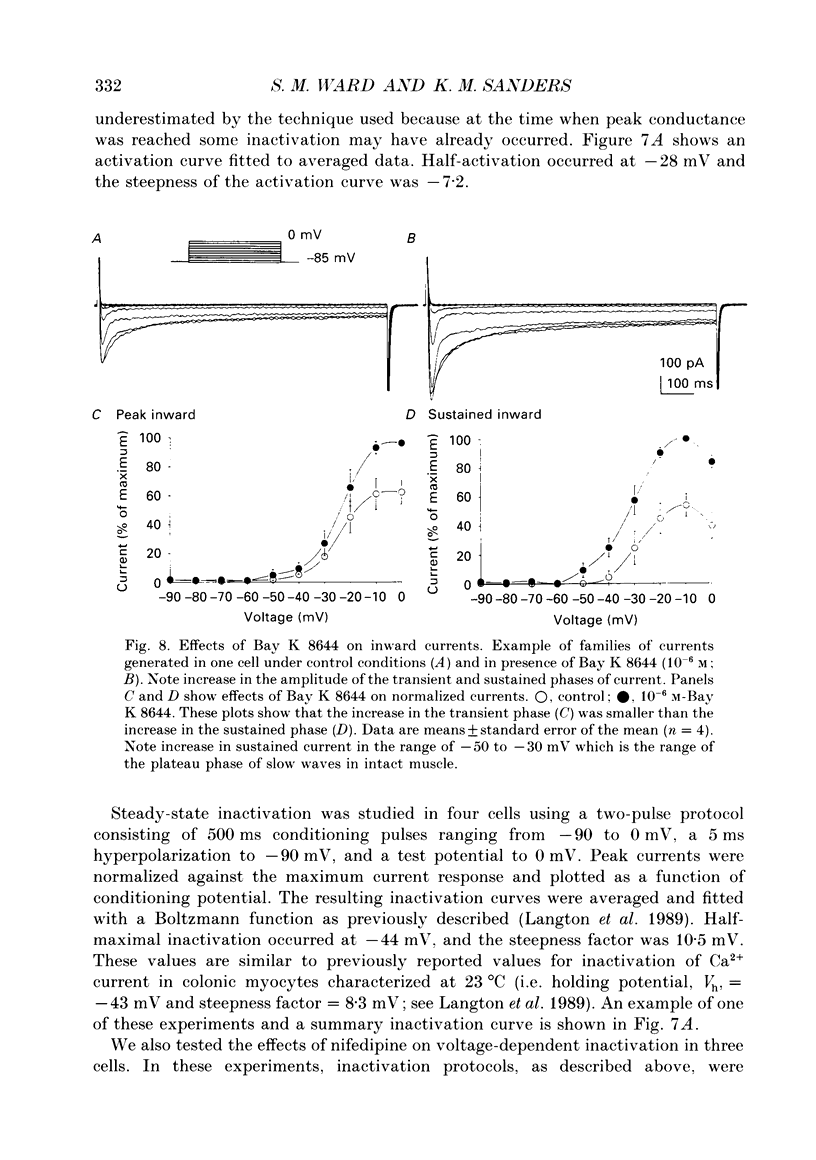
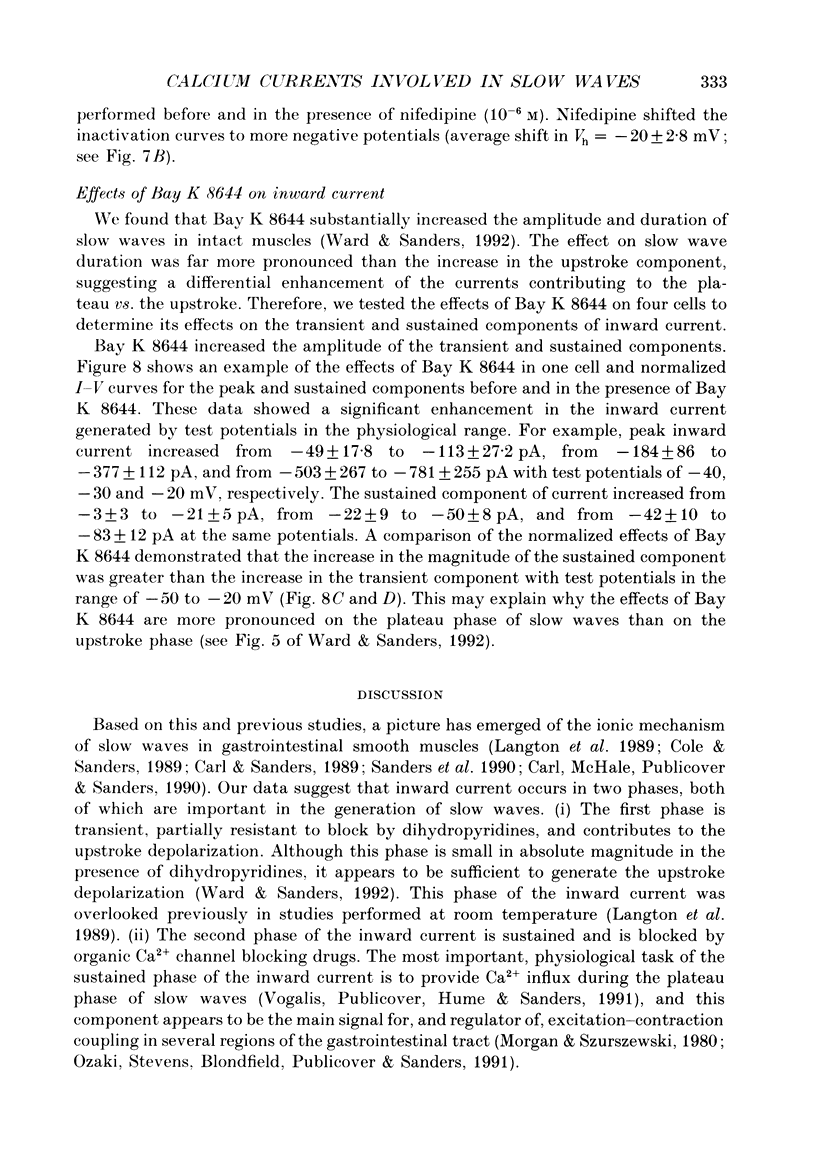
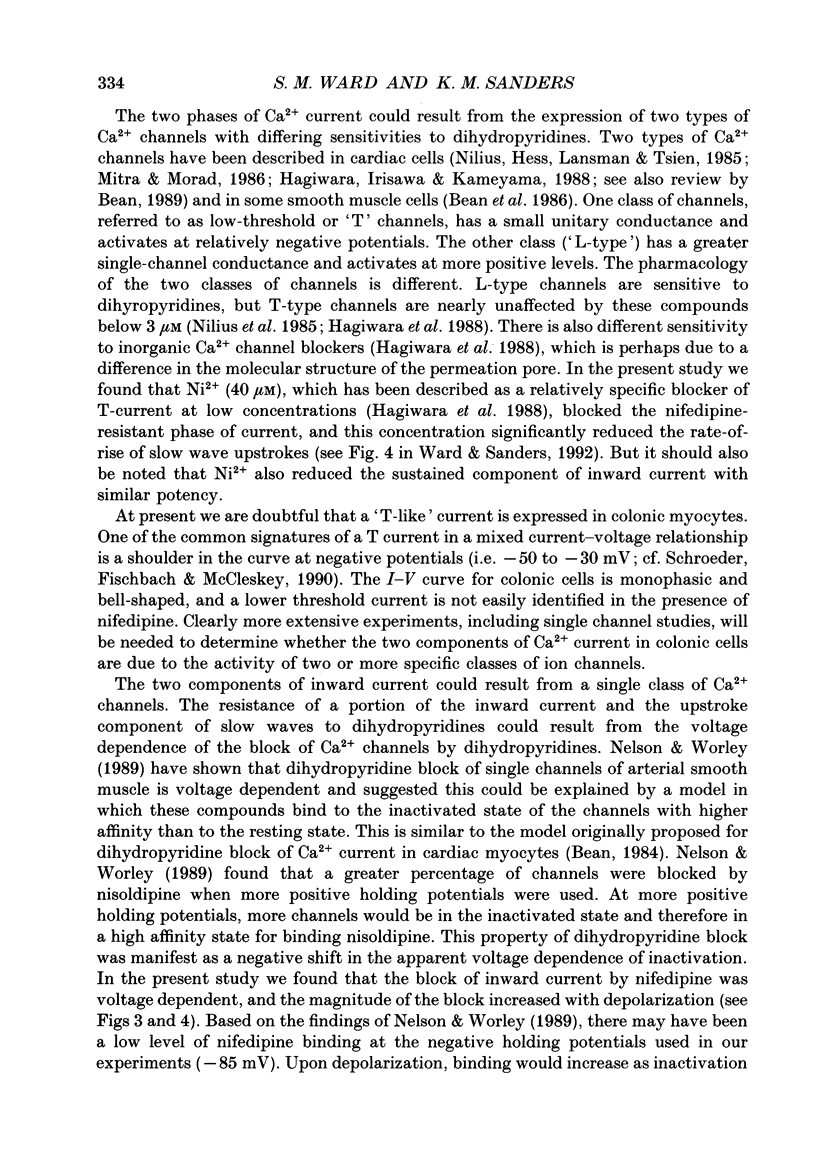
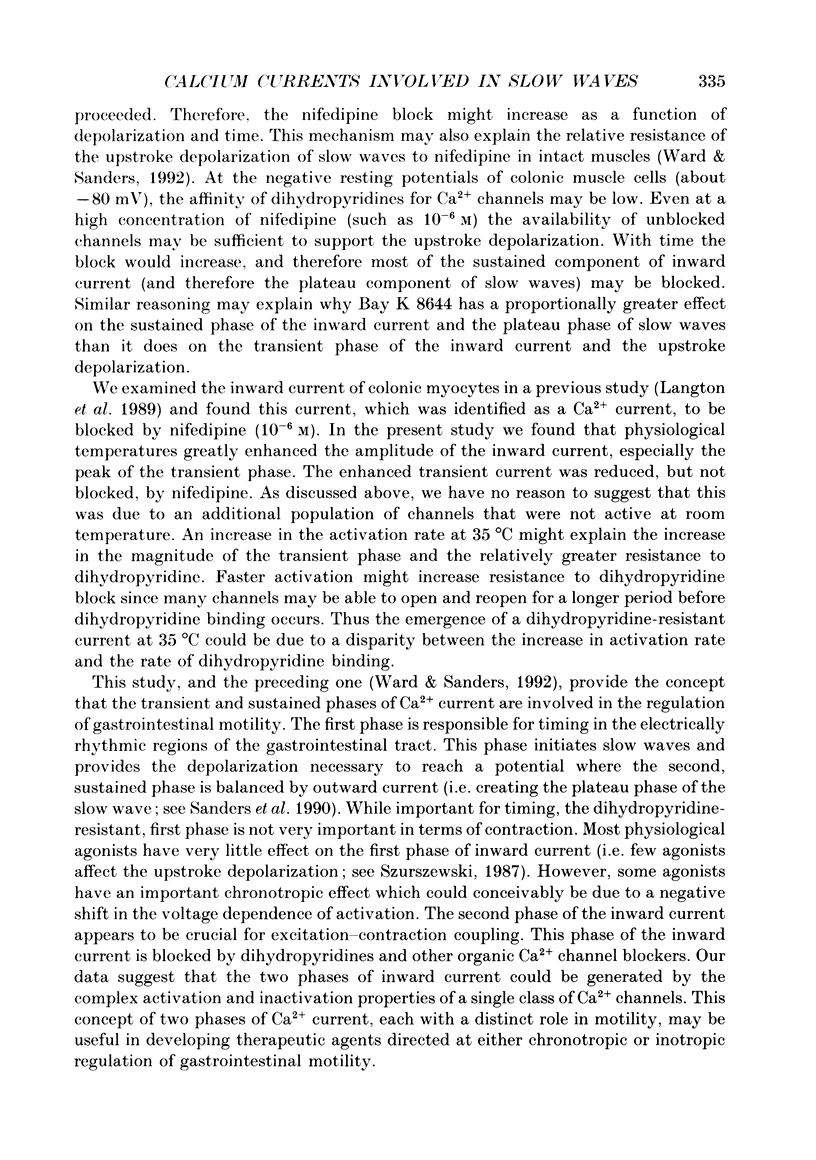
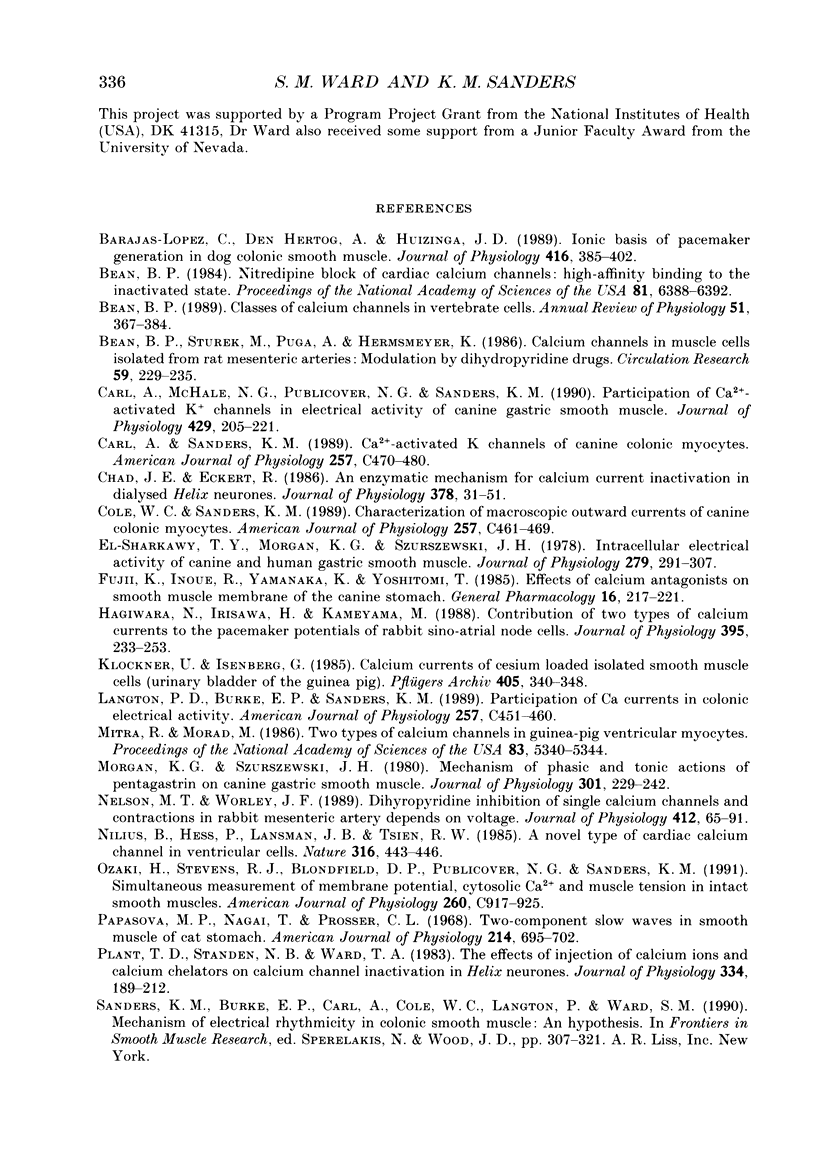
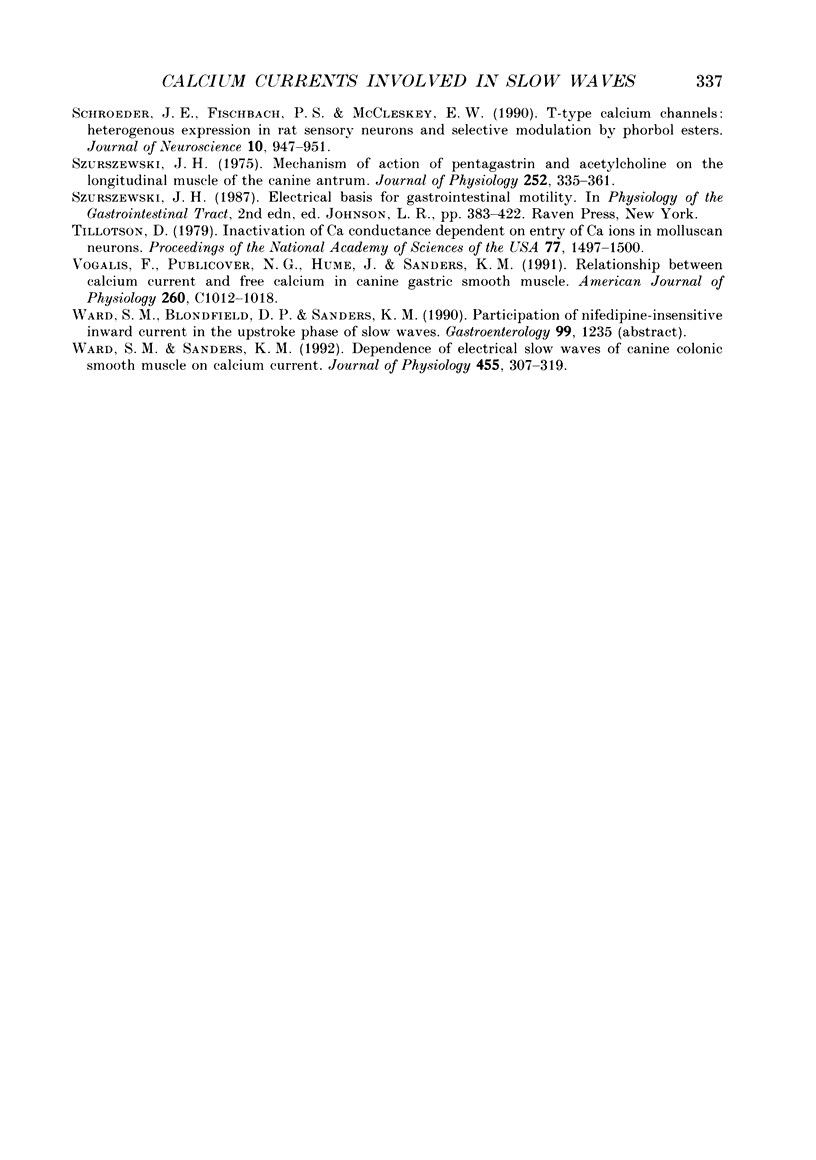
Selected References
These references are in PubMed. This may not be the complete list of references from this article.
- Barajas-López C., Den Hertog A., Huizinga J. D. Ionic basis of pacemaker generation in dog colonic smooth muscle. J Physiol. 1989 Sep;416:385–402. doi: 10.1113/jphysiol.1989.sp017767. [DOI] [PMC free article] [PubMed] [Google Scholar]
- Bean B. P. Classes of calcium channels in vertebrate cells. Annu Rev Physiol. 1989;51:367–384. doi: 10.1146/annurev.ph.51.030189.002055. [DOI] [PubMed] [Google Scholar]
- Bean B. P. Nitrendipine block of cardiac calcium channels: high-affinity binding to the inactivated state. Proc Natl Acad Sci U S A. 1984 Oct;81(20):6388–6392. doi: 10.1073/pnas.81.20.6388. [DOI] [PMC free article] [PubMed] [Google Scholar]
- Bean B. P., Sturek M., Puga A., Hermsmeyer K. Calcium channels in muscle cells isolated from rat mesenteric arteries: modulation by dihydropyridine drugs. Circ Res. 1986 Aug;59(2):229–235. doi: 10.1161/01.res.59.2.229. [DOI] [PubMed] [Google Scholar]
- Carl A., McHale N. G., Publicover N. G., Sanders K. M. Participation of Ca2(+)-activated K+ channels in electrical activity of canine gastric smooth muscle. J Physiol. 1990 Oct;429:205–221. doi: 10.1113/jphysiol.1990.sp018252. [DOI] [PMC free article] [PubMed] [Google Scholar]
- Carl A., Sanders K. M. Ca2+-activated K channels of canine colonic myocytes. Am J Physiol. 1989 Sep;257(3 Pt 1):C470–C480. doi: 10.1152/ajpcell.1989.257.3.C470. [DOI] [PubMed] [Google Scholar]
- Chad J. E., Eckert R. An enzymatic mechanism for calcium current inactivation in dialysed Helix neurones. J Physiol. 1986 Sep;378:31–51. doi: 10.1113/jphysiol.1986.sp016206. [DOI] [PMC free article] [PubMed] [Google Scholar]
- Cole W. C., Sanders K. M. Characterization of macroscopic outward currents of canine colonic myocytes. Am J Physiol. 1989 Sep;257(3 Pt 1):C461–C469. doi: 10.1152/ajpcell.1989.257.3.C461. [DOI] [PubMed] [Google Scholar]
- Fujii K., Inoue R., Yamanaka K., Yoshitomi T. Effects of calcium antagonists on smooth muscle membranes of the canine stomach. Gen Pharmacol. 1985;16(3):217–221. doi: 10.1016/0306-3623(85)90072-2. [DOI] [PubMed] [Google Scholar]
- Hagiwara N., Irisawa H., Kameyama M. Contribution of two types of calcium currents to the pacemaker potentials of rabbit sino-atrial node cells. J Physiol. 1988 Jan;395:233–253. doi: 10.1113/jphysiol.1988.sp016916. [DOI] [PMC free article] [PubMed] [Google Scholar]
- Klöckner U., Isenberg G. Calcium currents of cesium loaded isolated smooth muscle cells (urinary bladder of the guinea pig). Pflugers Arch. 1985 Dec;405(4):340–348. doi: 10.1007/BF00595686. [DOI] [PubMed] [Google Scholar]
- Langton P. D., Burke E. P., Sanders K. M. Participation of Ca currents in colonic electrical activity. Am J Physiol. 1989 Sep;257(3 Pt 1):C451–C460. doi: 10.1152/ajpcell.1989.257.3.C451. [DOI] [PubMed] [Google Scholar]
- Mitra R., Morad M. Two types of calcium channels in guinea pig ventricular myocytes. Proc Natl Acad Sci U S A. 1986 Jul;83(14):5340–5344. doi: 10.1073/pnas.83.14.5340. [DOI] [PMC free article] [PubMed] [Google Scholar]
- Morgan K. G., Szurszewski J. H. Mechanisms of phasic and tonic actions of pentagastrin on canine gastric smooth muscle. J Physiol. 1980 Apr;301:229–242. doi: 10.1113/jphysiol.1980.sp013201. [DOI] [PMC free article] [PubMed] [Google Scholar]
- Nelson M. T., Worley J. F. Dihydropyridine inhibition of single calcium channels and contraction in rabbit mesenteric artery depends on voltage. J Physiol. 1989 May;412:65–91. doi: 10.1113/jphysiol.1989.sp017604. [DOI] [PMC free article] [PubMed] [Google Scholar]
- Nilius B., Hess P., Lansman J. B., Tsien R. W. A novel type of cardiac calcium channel in ventricular cells. Nature. 1985 Aug 1;316(6027):443–446. doi: 10.1038/316443a0. [DOI] [PubMed] [Google Scholar]
- Ozaki H., Stevens R. J., Blondfield D. P., Publicover N. G., Sanders K. M. Simultaneous measurement of membrane potential, cytosolic Ca2+, and tension in intact smooth muscles. Am J Physiol. 1991 May;260(5 Pt 1):C917–C925. doi: 10.1152/ajpcell.1991.260.5.C917. [DOI] [PubMed] [Google Scholar]
- Papasova M. P., Nagai T., Prosser C. L. Two-component slow waves in smooth muscle of cat stomach. Am J Physiol. 1968 Apr;214(4):695–702. doi: 10.1152/ajplegacy.1968.214.4.695. [DOI] [PubMed] [Google Scholar]
- Plant T. D., Standen N. B., Ward T. A. The effects of injection of calcium ions and calcium chelators on calcium channel inactivation in Helix neurones. J Physiol. 1983 Jan;334:189–212. doi: 10.1113/jphysiol.1983.sp014489. [DOI] [PMC free article] [PubMed] [Google Scholar]
- Sanders K. M., Burke E. P., Carl A., Cole W. C., Langton P., Ward S. Mechanism of electrical rhythmicity in colonic smooth muscle: an hypothesis. Prog Clin Biol Res. 1990;327:307–321. [PubMed] [Google Scholar]
- Schroeder J. E., Fischbach P. S., McCleskey E. W. T-type calcium channels: heterogeneous expression in rat sensory neurons and selective modulation by phorbol esters. J Neurosci. 1990 Mar;10(3):947–951. doi: 10.1523/JNEUROSCI.10-03-00947.1990. [DOI] [PMC free article] [PubMed] [Google Scholar]
- Szurszewski J. H. Mechanism of action of pentagastrin and acetylcholine on the longitudinal muscle of the canine antrum. J Physiol. 1975 Nov;252(2):335–361. doi: 10.1113/jphysiol.1975.sp011147. [DOI] [PMC free article] [PubMed] [Google Scholar]
- Tillotson D. Inactivation of Ca conductance dependent on entry of Ca ions in molluscan neurons. Proc Natl Acad Sci U S A. 1979 Mar;76(3):1497–1500. doi: 10.1073/pnas.76.3.1497. [DOI] [PMC free article] [PubMed] [Google Scholar]
- Vogalis F., Publicover N. G., Hume J. R., Sanders K. M. Relationship between calcium current and cytosolic calcium in canine gastric smooth muscle cells. Am J Physiol. 1991 May;260(5 Pt 1):C1012–C1018. doi: 10.1152/ajpcell.1991.260.5.C1012. [DOI] [PubMed] [Google Scholar]
- Ward S. M., Sanders K. M. Dependence of electrical slow waves of canine colonic smooth muscle on calcium gradient. J Physiol. 1992 Sep;455:307–319. doi: 10.1113/jphysiol.1992.sp019303. [DOI] [PMC free article] [PubMed] [Google Scholar]
- el-Sharkawy T. Y., Morgan K. G., Szurszewski J. H. Intracellular electrical activity of canine and human gastric smooth muscle. J Physiol. 1978 Jun;279:291–307. doi: 10.1113/jphysiol.1978.sp012345. [DOI] [PMC free article] [PubMed] [Google Scholar]