Abstract
1. The rate of energy expended for the clearance of sarcoplasmic Ca2+ by sarcoreticular Ca2+ uptake process(es), plus the concomitant metabolic reactions, was evaluated from measurements of resting heat production by mouse soleus muscle before and after indirect inhibition of Ca2+ uptake by sarcoplasmic reticulum (SR). 2. Direct inhibition of the Ca2+, Mg(2+)-ATPase of SR membrane in intact muscle preparations exposed to the specific inhibitor 2,5-di(tert-butyl-1,4-benzohydroquinone (tBuBHQ) slowly increased the rate of heat production (E). Indirect inhibition of SR Ca2+ uptake was obtained by reducing sarcoplasmic Ca2+ concentration (Ca2+i) as a consequence of reducing Ca2+ release from the SR using dantrolene sodium. This promptly decreased E by 12%. Exposure of the preparations to an Mg(2+)-enriched environment (high Mg2+) or to the chemical phosphatase 2,3-butanedione monoxime (BDM), two other procedures aimed at decreasing SR Ca2+ release, also acutely decreased E, by 20 and 24%, respectively. 3. Subthreshold-for-contracture depolarization of the sarcolemma achieved by increasing extracellular K+ concentration to 11.8 mM induced a biphasic increase of E: an initial peak to 290% of basal E, followed by a plateau phase at 140% of basal E during which resting muscle tension was increased by less than 3%. Most, if not all, of the plateau-phase metabolic response was quickly suppressed by dantrolene or high Mg2+ or BDM. Another means of increasing SR Ca2+ cycling was to partially remove the calmodulin-dependent control of SR Ca2+ release using the calmodulin inhibitor W-7. The progressive increase in E with 30 microM-W-7 was largely reduced by dantrolene or high Mg2+ or BDM. 4. In the presence of either dantrolene or BDM to prevent the effect of W-7 on SR Ca2+ release, exposure of the muscle to W-7 acutely suppressed about 3% of E. This and the above results confirm that the plasmalemmal, calmodulin-dependent Ca(2+)-ATPase, although a qualitatively essential part of the Ca2+i homeostatic system of the cell, can only be responsible for a very minor part of the energy expenditure devoted to the homeostasis of Ca2+i. Active Ca2+ uptake by SR which, at least in the submicromolar range of Ca2+i, is expected to be responsible for most of this Ca(2+)-dependent energy expenditure, might dissipate up to 25-40% of total metabolic energy in the intact mouse soleus under basal and near-basal conditions.
Full text
PDF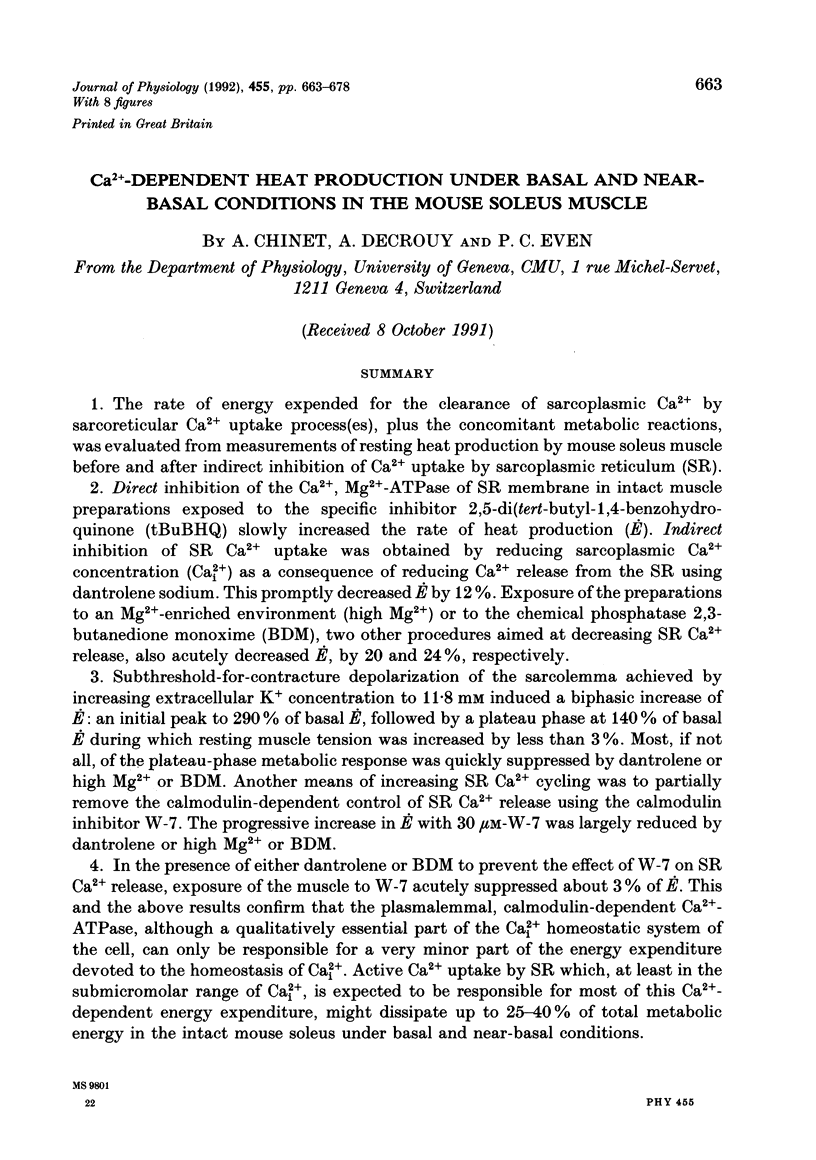
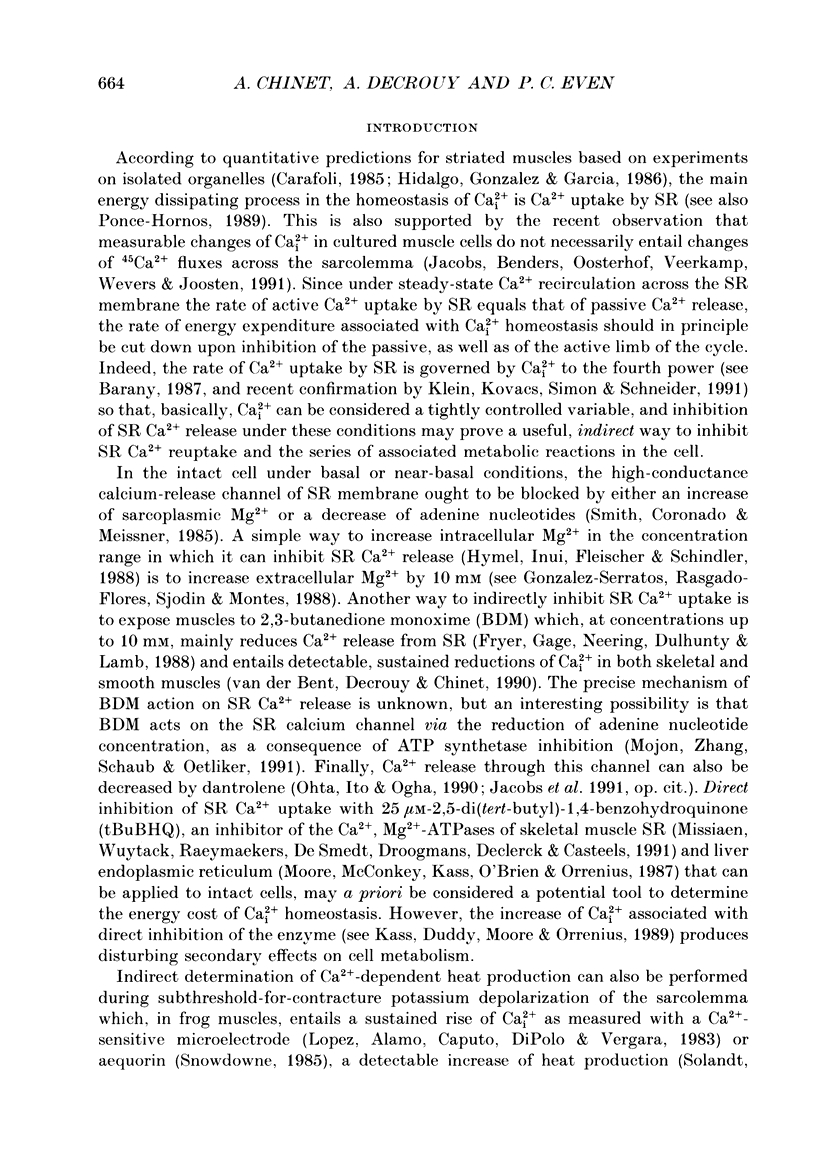
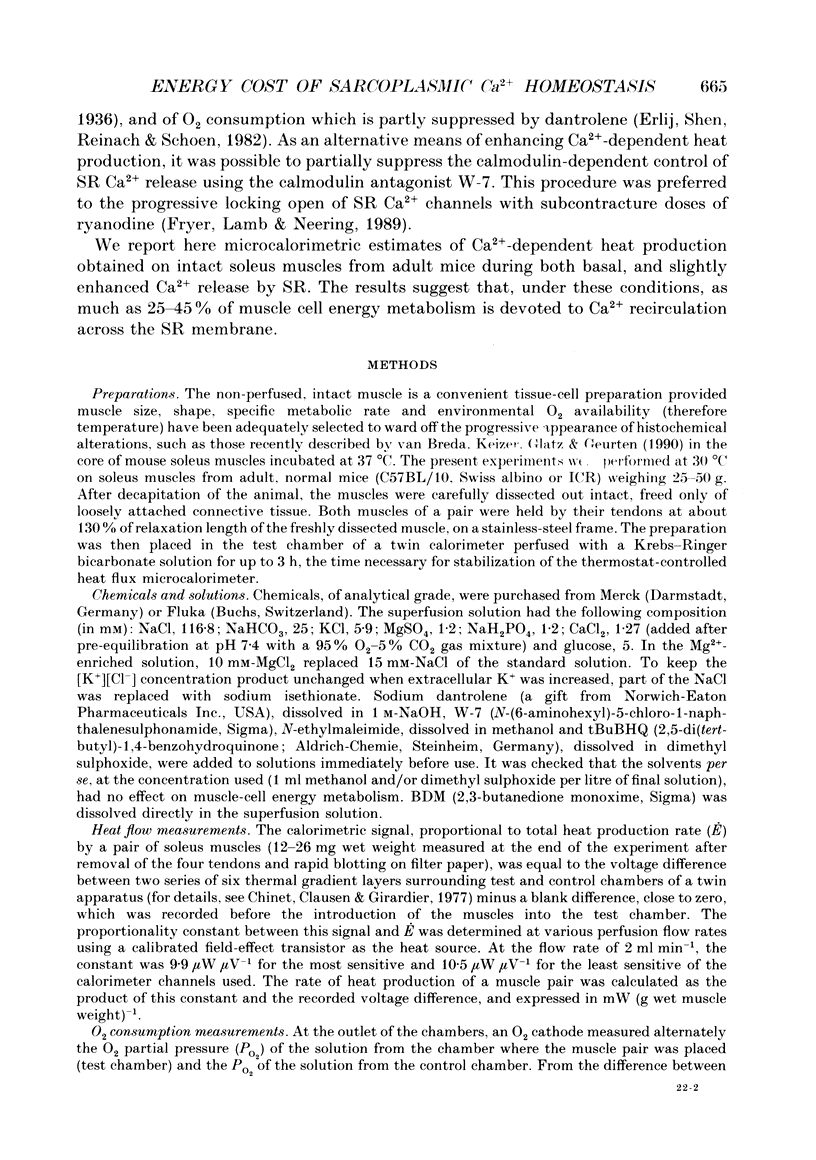
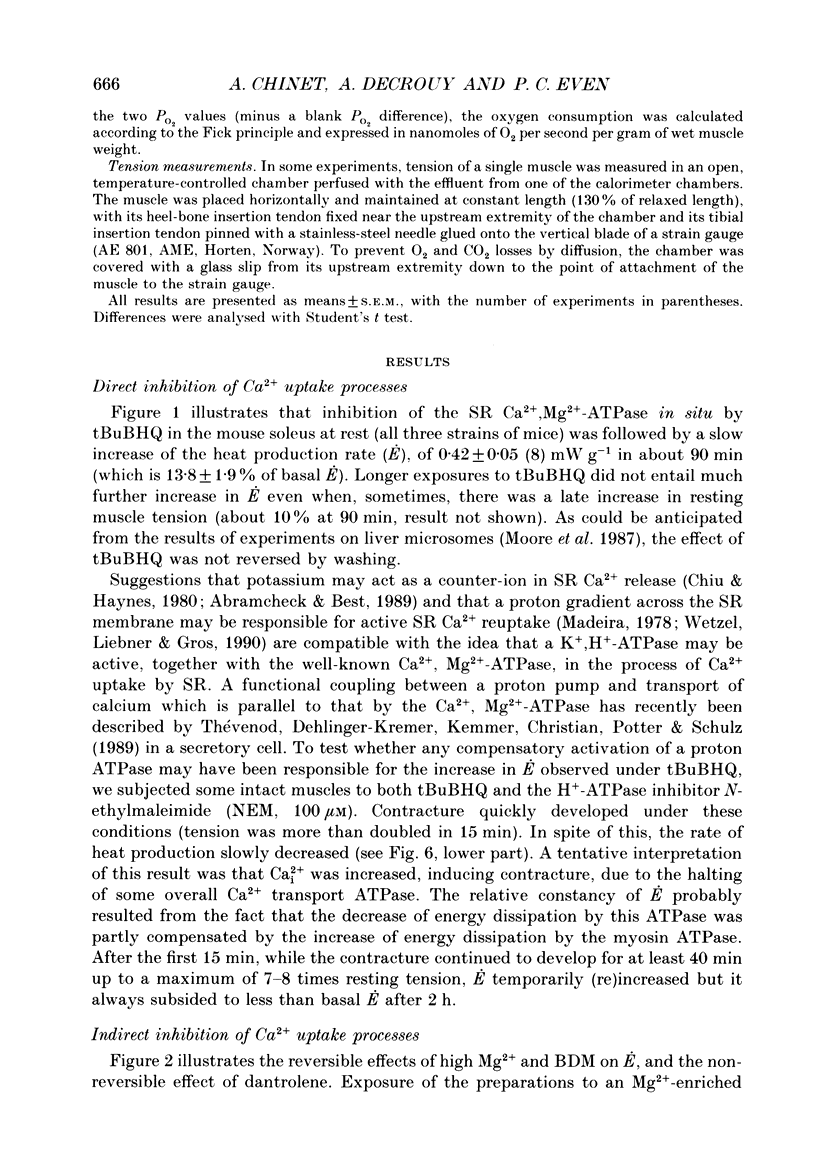
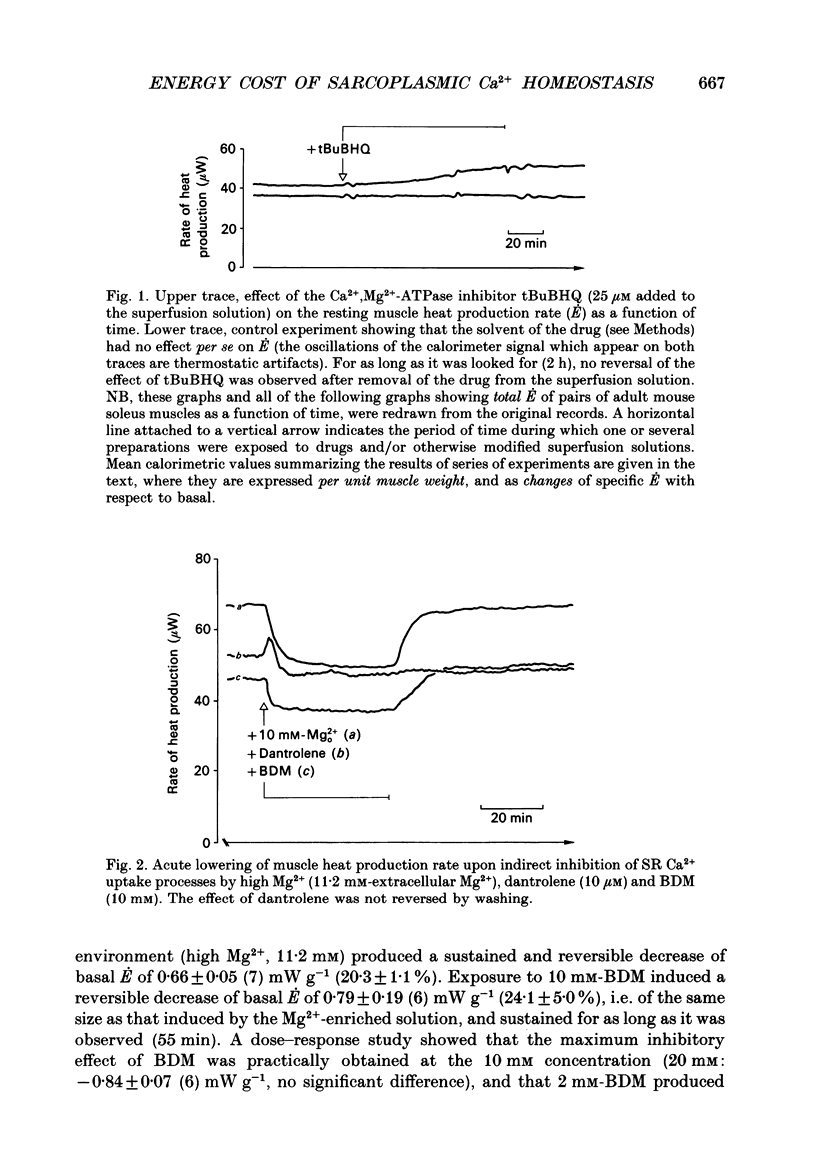
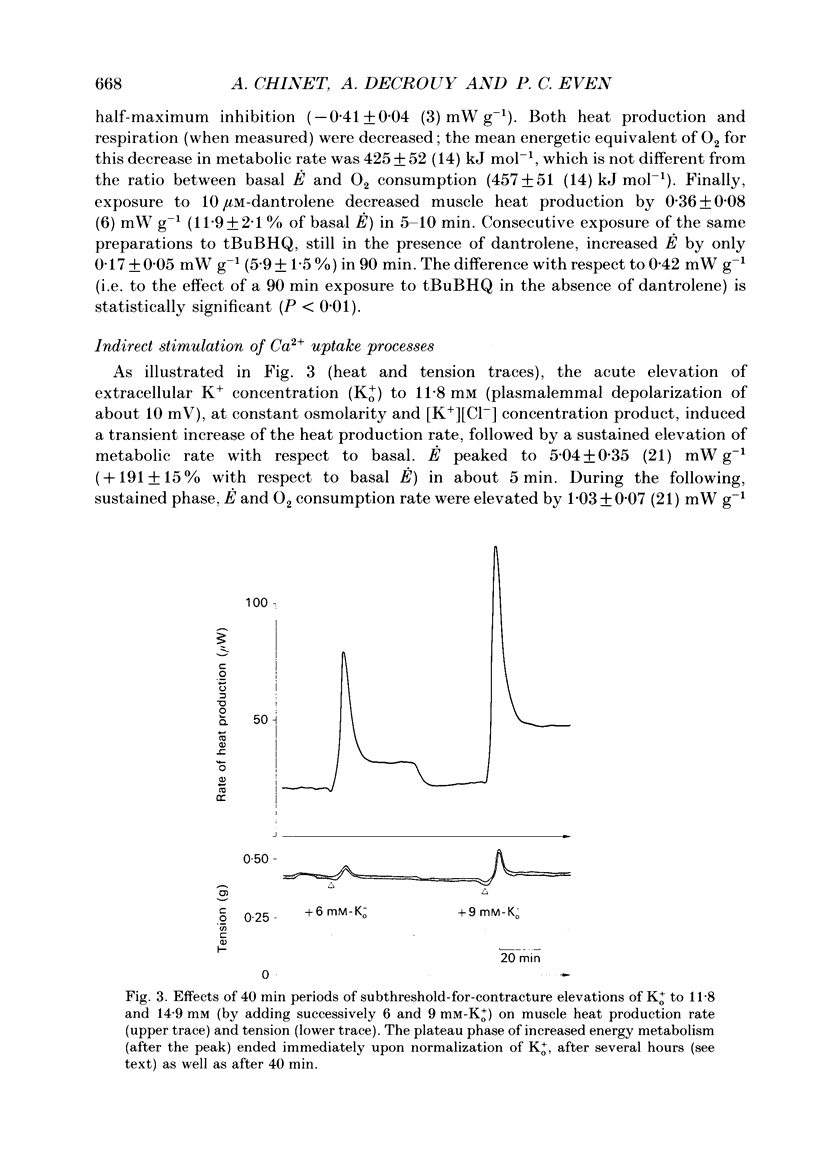
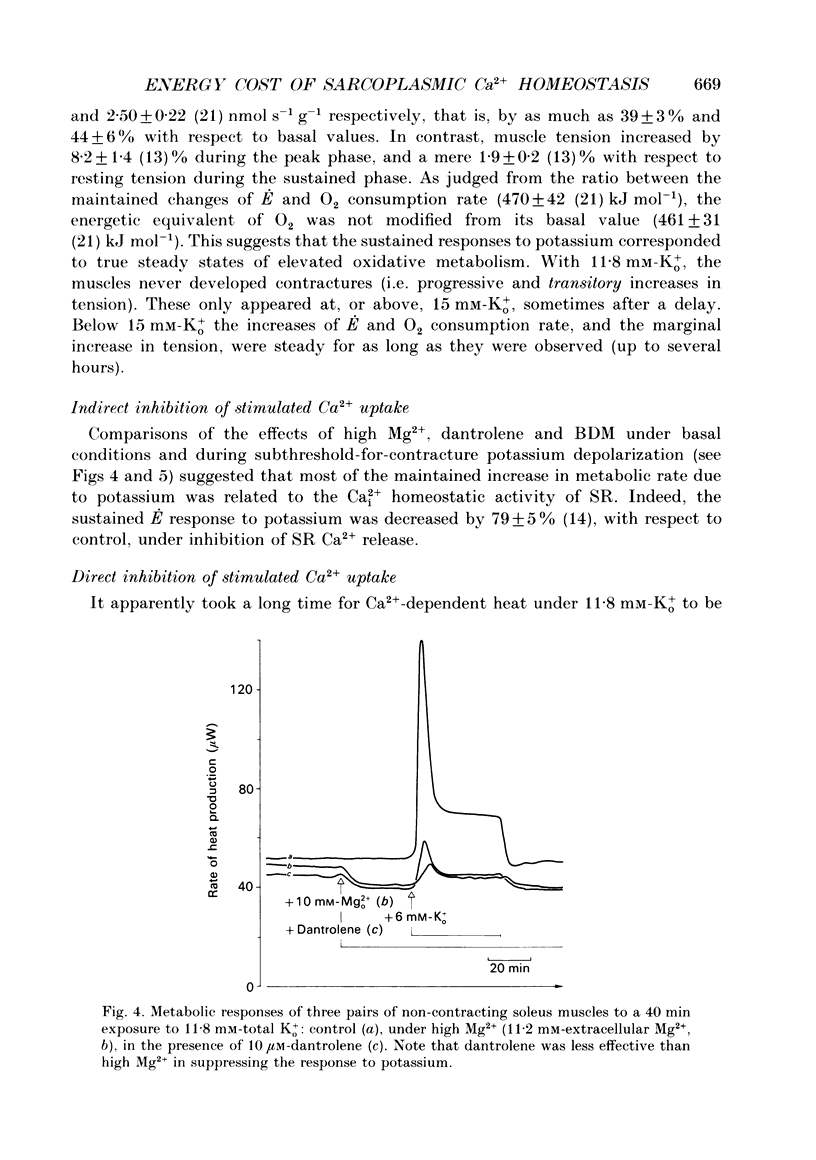
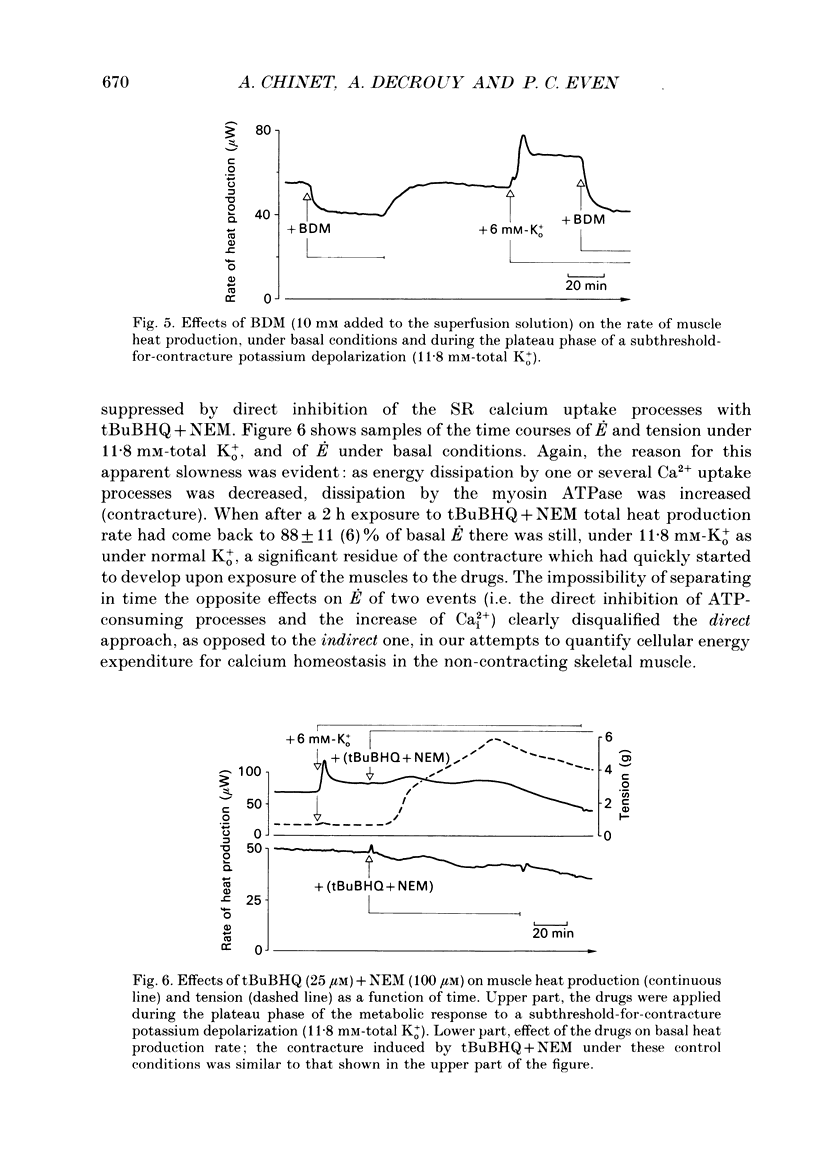
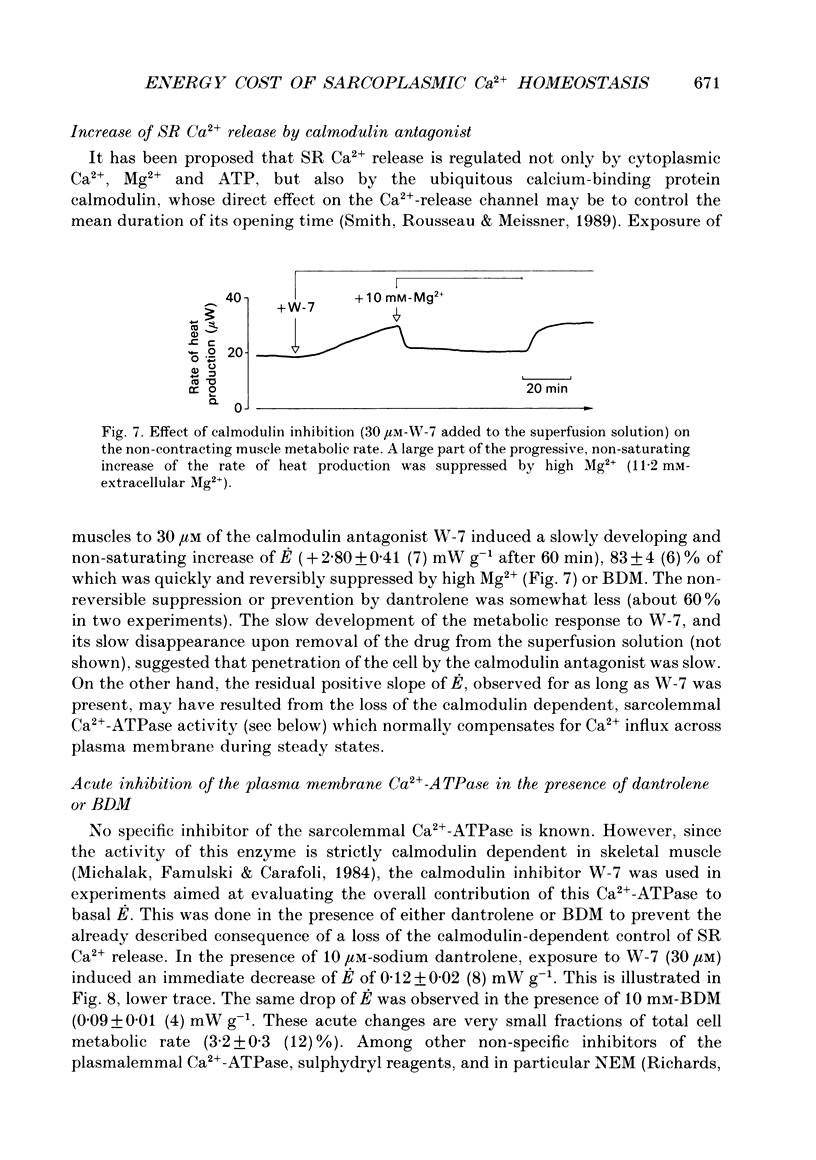
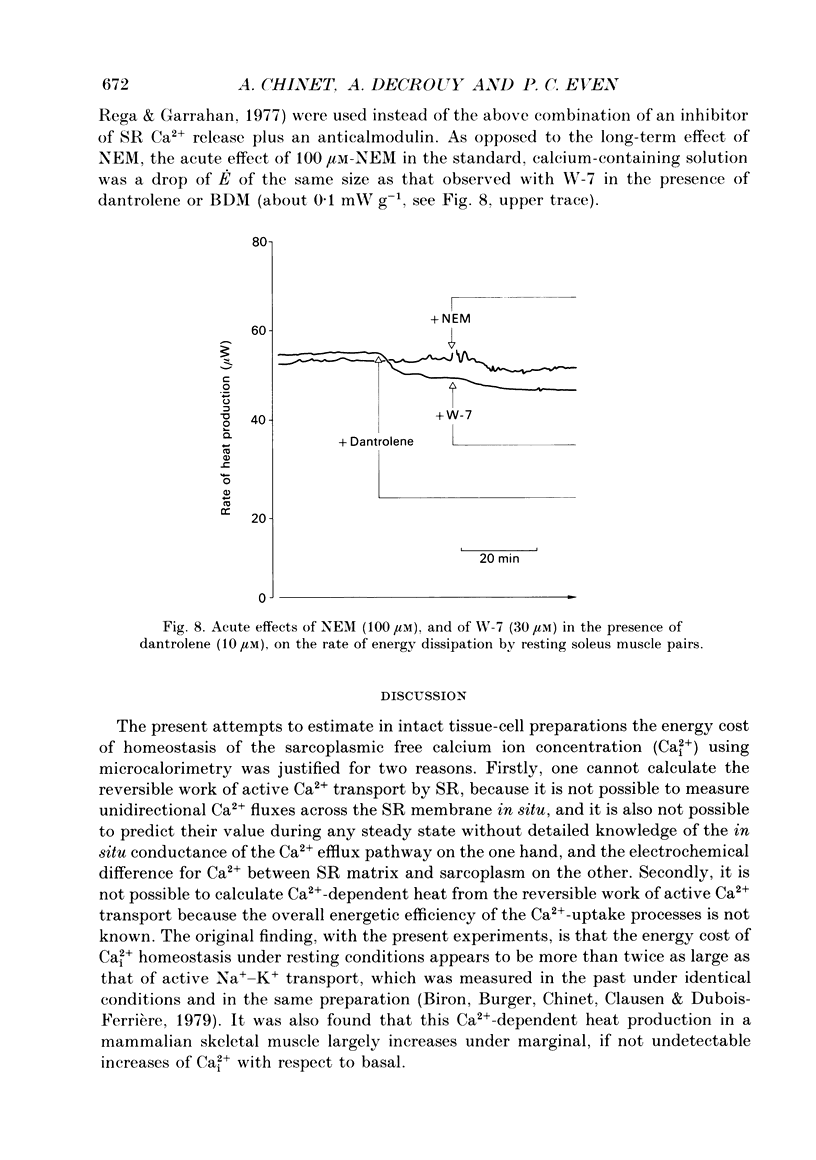
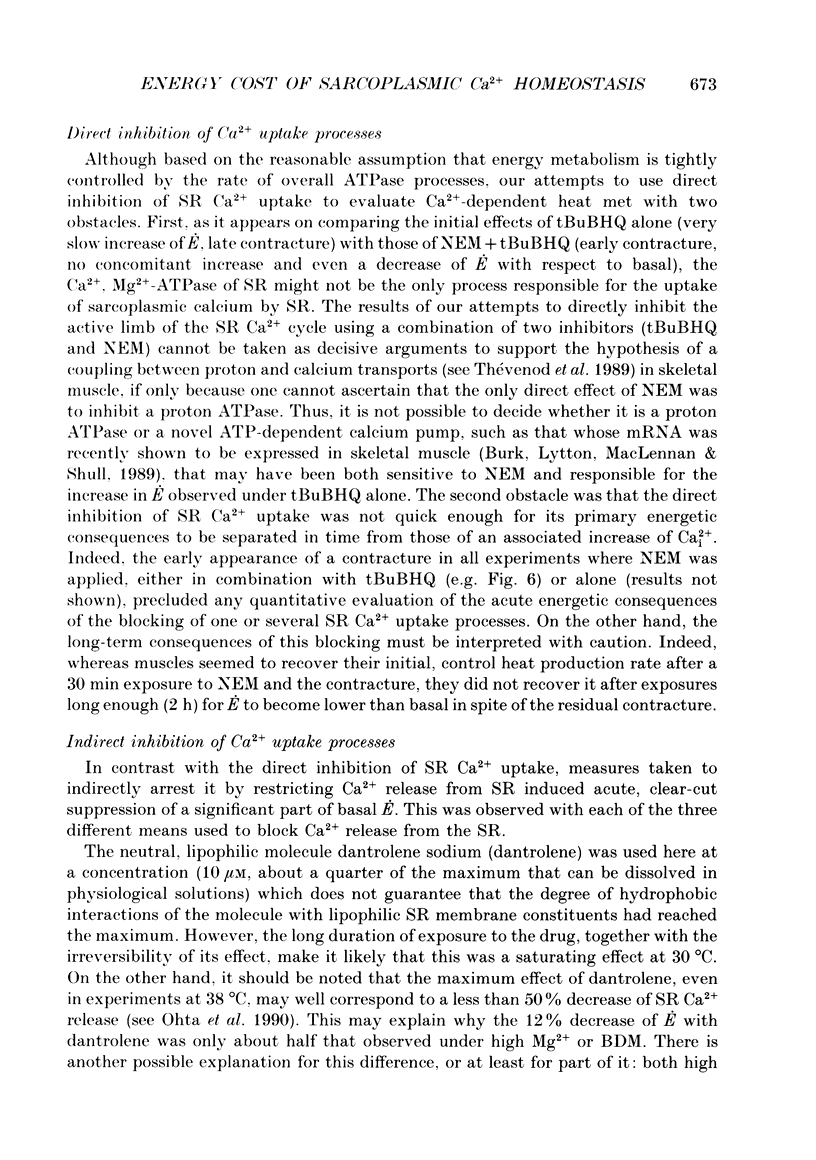
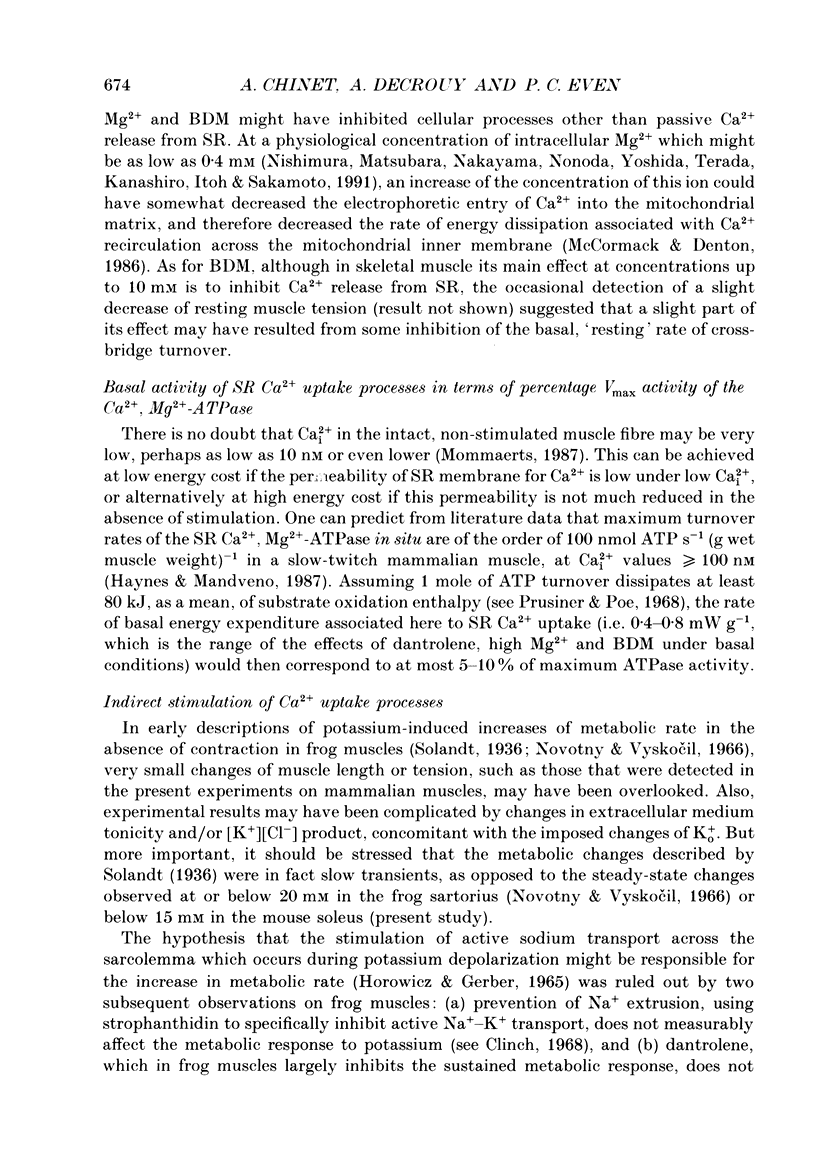
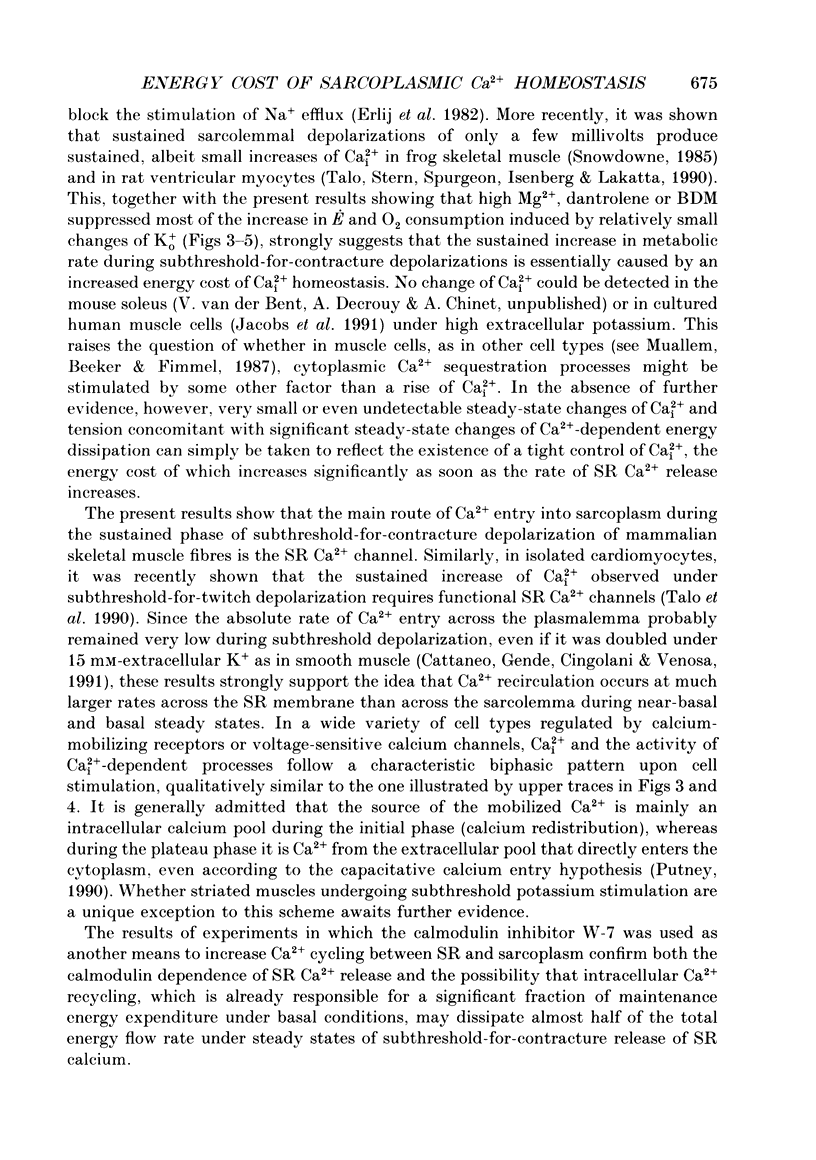
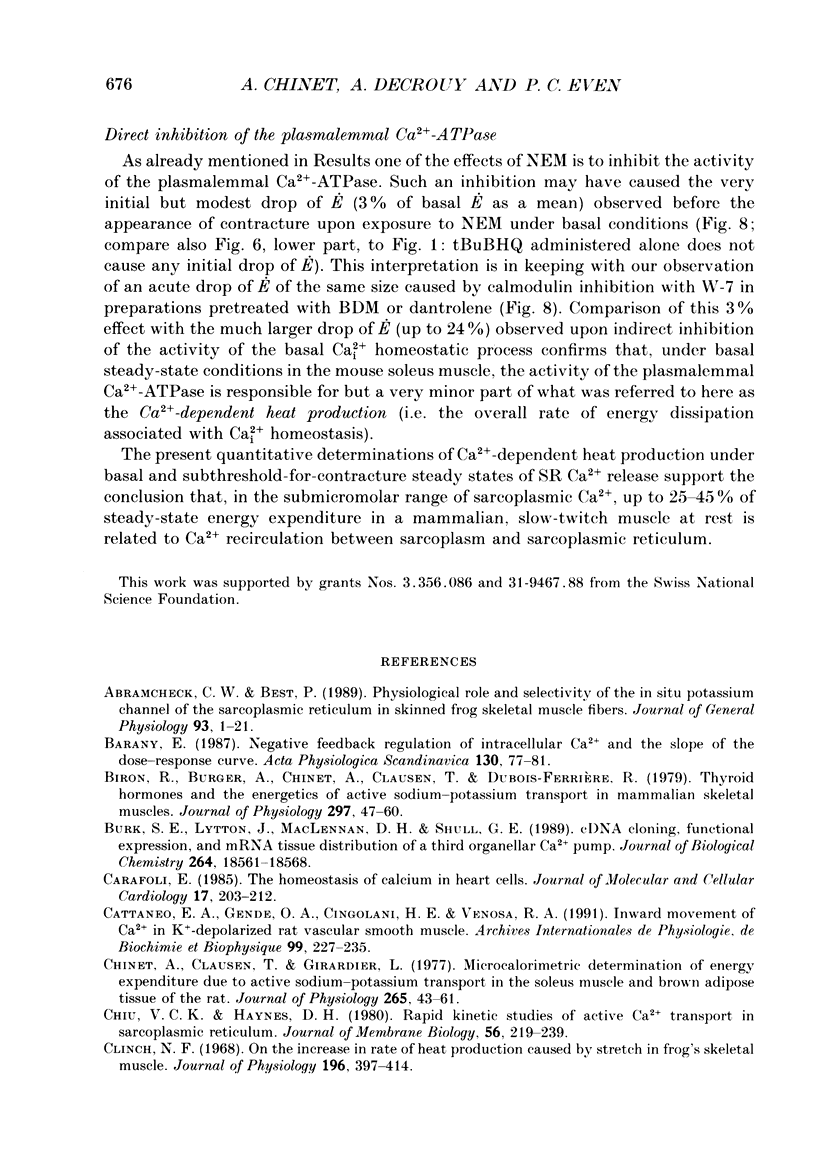
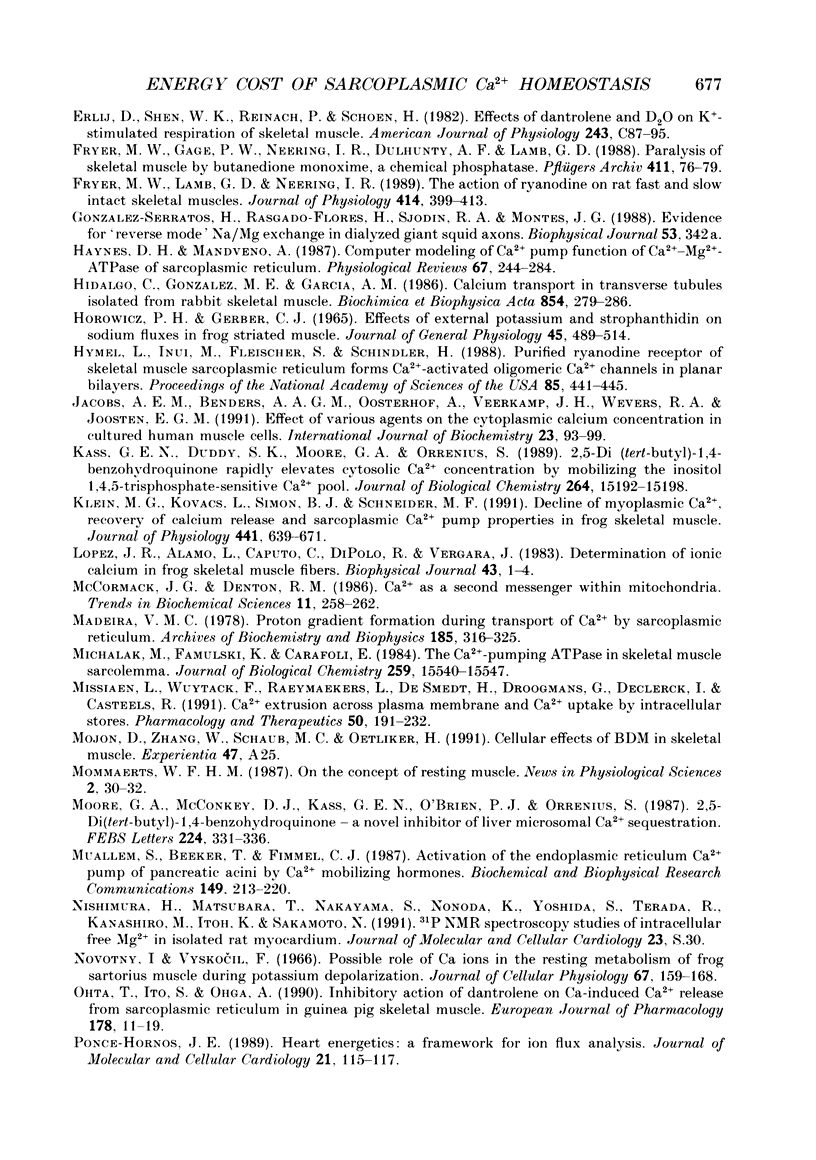
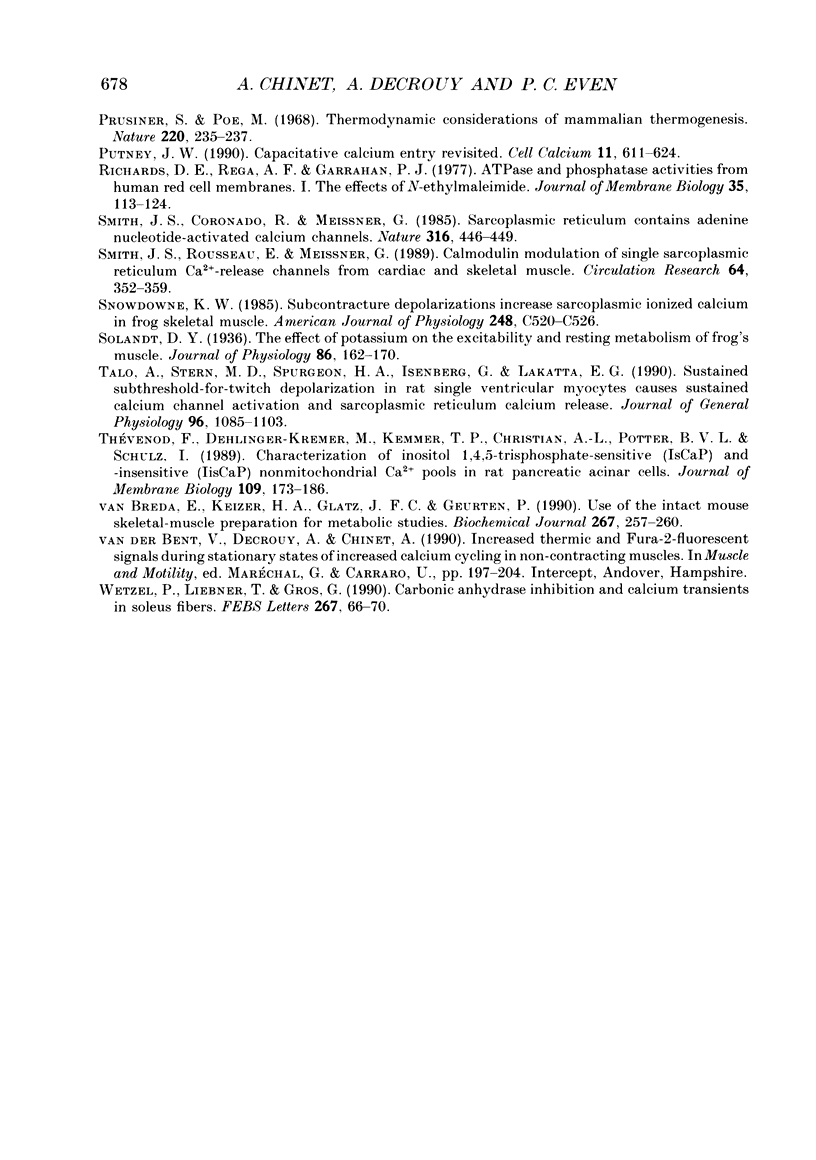
Images in this article
Selected References
These references are in PubMed. This may not be the complete list of references from this article.
- Abramcheck C. W., Best P. M. Physiological role and selectivity of the in situ potassium channel of the sarcoplasmic reticulum in skinned frog skeletal muscle fibers. J Gen Physiol. 1989 Jan;93(1):1–21. doi: 10.1085/jgp.93.1.1. [DOI] [PMC free article] [PubMed] [Google Scholar]
- Amado Cattaneo E., Gende O. A., Cingolani H. E., Venosa R. A. Inward movement of Ca2+ in K(+)-depolarized rat vascular smooth muscle. Arch Int Physiol Biochim Biophys. 1991 Jun;99(3):227–235. doi: 10.3109/13813459109146927. [DOI] [PubMed] [Google Scholar]
- Biron R., Burger A., Chinet A., Clausen T., Dubois-Ferrière R. Thyroid hormones and the energetics of active sodium-potassium transport in mammalian skeletal muscles. J Physiol. 1979 Dec;297(0):47–60. doi: 10.1113/jphysiol.1979.sp013026. [DOI] [PMC free article] [PubMed] [Google Scholar]
- Burk S. E., Lytton J., MacLennan D. H., Shull G. E. cDNA cloning, functional expression, and mRNA tissue distribution of a third organellar Ca2+ pump. J Biol Chem. 1989 Nov 5;264(31):18561–18568. [PubMed] [Google Scholar]
- Bárány E. Negative feedback regulation of intracellular Ca2+ and the slope of the dose-response curve. Acta Physiol Scand. 1987 May;130(1):77–81. doi: 10.1111/j.1748-1716.1987.tb08114.x. [DOI] [PubMed] [Google Scholar]
- Carafoli E. The homeostasis of calcium in heart cells. J Mol Cell Cardiol. 1985 Mar;17(3):203–212. doi: 10.1016/s0022-2828(85)80003-1. [DOI] [PubMed] [Google Scholar]
- Chinet A., Clausen T., Girardier L. Microcalorimetric determination of energy expenditure due to active sodium-potassium transport in the soleus muscle and brown adipose tissue of the rat. J Physiol. 1977 Feb;265(1):43–61. doi: 10.1113/jphysiol.1977.sp011704. [DOI] [PMC free article] [PubMed] [Google Scholar]
- Chiu V. C., Haynes D. H. Rapid kinetic studies of active Ca2+ transport in sarcoplasmic reticulum. J Membr Biol. 1980 Oct 31;56(3):219–239. doi: 10.1007/BF01869478. [DOI] [PubMed] [Google Scholar]
- Clinch N. F. On the increase in rate of heat production caused by stretch in frog's skeletal muscle. J Physiol. 1968 May;196(2):397–414. doi: 10.1113/jphysiol.1968.sp008514. [DOI] [PMC free article] [PubMed] [Google Scholar]
- Erlij D., Shen W. K., Reinach P., Schoen H. Effects of dantrolene and D2O on K+-stimulated respiration of skeletal muscle. Am J Physiol. 1982 Jul;243(1):C87–C95. doi: 10.1152/ajpcell.1982.243.1.C87. [DOI] [PubMed] [Google Scholar]
- Fryer M. W., Gage P. W., Neering I. R., Dulhunty A. F., Lamb G. D. Paralysis of skeletal muscle by butanedione monoxime, a chemical phosphatase. Pflugers Arch. 1988 Jan;411(1):76–79. doi: 10.1007/BF00581649. [DOI] [PubMed] [Google Scholar]
- Fryer M. W., Lamb G. D., Neering I. R. The action of ryanodine on rat fast and slow intact skeletal muscles. J Physiol. 1989 Jul;414:399–413. doi: 10.1113/jphysiol.1989.sp017695. [DOI] [PMC free article] [PubMed] [Google Scholar]
- HOROWICZ P., GERBER C. J. EFFECTS OF EXTERNAL POTASSIUM AND STROPHANTHIDIN ON SODIUM FLUXES IN FROG STRIATED MUSCLE. J Gen Physiol. 1965 Jan;48:489–514. doi: 10.1085/jgp.48.3.489. [DOI] [PMC free article] [PubMed] [Google Scholar]
- Haynes D. H., Mandveno A. Computer modeling of Ca2+ pump function of Ca2+-Mg2+-ATPase of sarcoplasmic reticulum. Physiol Rev. 1987 Jan;67(1):244–284. doi: 10.1152/physrev.1987.67.1.244. [DOI] [PubMed] [Google Scholar]
- Hidalgo C., González M. E., García A. M. Calcium transport in transverse tubules isolated from rabbit skeletal muscle. Biochim Biophys Acta. 1986 Jan 29;854(2):279–286. doi: 10.1016/0005-2736(86)90121-5. [DOI] [PubMed] [Google Scholar]
- Hymel L., Inui M., Fleischer S., Schindler H. Purified ryanodine receptor of skeletal muscle sarcoplasmic reticulum forms Ca2+-activated oligomeric Ca2+ channels in planar bilayers. Proc Natl Acad Sci U S A. 1988 Jan;85(2):441–445. doi: 10.1073/pnas.85.2.441. [DOI] [PMC free article] [PubMed] [Google Scholar]
- Jacobs A. E., Benders A. A., Oosterhof A., Veerkamp J. H., Wevers R. A., Joosten E. G. Effect of various agents on the cytoplasmic calcium concentration in cultured human muscle cells. Int J Biochem. 1991;23(1):93–99. doi: 10.1016/0020-711x(91)90014-e. [DOI] [PubMed] [Google Scholar]
- Kass G. E., Duddy S. K., Moore G. A., Orrenius S. 2,5-Di-(tert-butyl)-1,4-benzohydroquinone rapidly elevates cytosolic Ca2+ concentration by mobilizing the inositol 1,4,5-trisphosphate-sensitive Ca2+ pool. J Biol Chem. 1989 Sep 15;264(26):15192–15198. [PubMed] [Google Scholar]
- Klein M. G., Kovacs L., Simon B. J., Schneider M. F. Decline of myoplasmic Ca2+, recovery of calcium release and sarcoplasmic Ca2+ pump properties in frog skeletal muscle. J Physiol. 1991 Sep;441:639–671. doi: 10.1113/jphysiol.1991.sp018771. [DOI] [PMC free article] [PubMed] [Google Scholar]
- López J. R., Alamo L., Caputo C., DiPolo R., Vergara S. Determination of ionic calcium in frog skeletal muscle fibers. Biophys J. 1983 Jul;43(1):1–4. doi: 10.1016/S0006-3495(83)84316-1. [DOI] [PMC free article] [PubMed] [Google Scholar]
- Madeira V. M. Proton gradient formation during transport of Ca2+ by sarcoplasmic reticulum. Arch Biochem Biophys. 1978 Jan 30;185(2):316–325. doi: 10.1016/0003-9861(78)90173-x. [DOI] [PubMed] [Google Scholar]
- Michalak M., Famulski K., Carafoli E. The Ca2+-pumping ATPase in skeletal muscle sarcolemma. Calmodulin dependence, regulation by cAMP-dependent phosphorylation, and purification. J Biol Chem. 1984 Dec 25;259(24):15540–15547. [PubMed] [Google Scholar]
- Missiaen L., Wuytack F., Raeymaekers L., De Smedt H., Droogmans G., Declerck I., Casteels R. Ca2+ extrusion across plasma membrane and Ca2+ uptake by intracellular stores. Pharmacol Ther. 1991;50(2):191–232. doi: 10.1016/0163-7258(91)90014-d. [DOI] [PubMed] [Google Scholar]
- Moore G. A., McConkey D. J., Kass G. E., O'Brien P. J., Orrenius S. 2,5-Di(tert-butyl)-1,4-benzohydroquinone--a novel inhibitor of liver microsomal Ca2+ sequestration. FEBS Lett. 1987 Nov 30;224(2):331–336. doi: 10.1016/0014-5793(87)80479-9. [DOI] [PubMed] [Google Scholar]
- Muallem S., Beeker T. G., Fimmel C. J. Activation of the endoplasmic reticulum Ca2+ pump of pancreatic acini by Ca2+ mobilizing hormones. Biochem Biophys Res Commun. 1987 Nov 30;149(1):213–220. doi: 10.1016/0006-291x(87)91626-3. [DOI] [PubMed] [Google Scholar]
- Novotný I., Vyskocil F. Possible role of Ca ions in the resting metabolism of frog sartorius muscle during potassium depolarization. J Cell Physiol. 1966 Feb;67(1):159–168. doi: 10.1002/jcp.1040670118. [DOI] [PubMed] [Google Scholar]
- Ohta T., Ito S., Ohga A. Inhibitory action of dantrolene on Ca-induced Ca2+ release from sarcoplasmic reticulum in guinea pig skeletal muscle. Eur J Pharmacol. 1990 Mar 13;178(1):11–19. doi: 10.1016/0014-2999(90)94788-y. [DOI] [PubMed] [Google Scholar]
- Ponce-Hornos J. E. Heart energetics: a framework for ion flux analysis. J Mol Cell Cardiol. 1989 Jan;21(1):115–117. doi: 10.1016/0022-2828(89)91499-5. [DOI] [PubMed] [Google Scholar]
- Prusiner S., Poe M. Thermodynamic cosiderations of mammalian thermogenesis. Nature. 1968 Oct 19;220(5164):235–237. doi: 10.1038/220235a0. [DOI] [PubMed] [Google Scholar]
- Putney J. W., Jr Capacitative calcium entry revisited. Cell Calcium. 1990 Nov-Dec;11(10):611–624. doi: 10.1016/0143-4160(90)90016-n. [DOI] [PubMed] [Google Scholar]
- Richards D. E., Rega A. F., Garrahan P. J. ATPase and phosphatase activities from human red cell membranes: I. The effects of N-ethylmaleimide. J Membr Biol. 1977 Jun 30;35(2):113–124. doi: 10.1007/BF01869943. [DOI] [PubMed] [Google Scholar]
- Smith J. S., Coronado R., Meissner G. Sarcoplasmic reticulum contains adenine nucleotide-activated calcium channels. Nature. 1985 Aug 1;316(6027):446–449. doi: 10.1038/316446a0. [DOI] [PubMed] [Google Scholar]
- Smith J. S., Rousseau E., Meissner G. Calmodulin modulation of single sarcoplasmic reticulum Ca2+-release channels from cardiac and skeletal muscle. Circ Res. 1989 Feb;64(2):352–359. doi: 10.1161/01.res.64.2.352. [DOI] [PubMed] [Google Scholar]
- Snowdowne K. W. Subcontracture depolarizations increase sarcoplasmic ionized calcium in frog skeletal muscle. Am J Physiol. 1985 May;248(5 Pt 1):C520–C526. doi: 10.1152/ajpcell.1985.248.5.C520. [DOI] [PubMed] [Google Scholar]
- Solandt D. Y. The effect of potassium on the excitability and resting metabolism of frog's muscle. J Physiol. 1936 Feb 8;86(2):162–170. doi: 10.1113/jphysiol.1936.sp003351. [DOI] [PMC free article] [PubMed] [Google Scholar]
- Talo A., Stern M. D., Spurgeon H. A., Isenberg G., Lakatta E. G. Sustained subthreshold-for-twitch depolarization in rat single ventricular myocytes causes sustained calcium channel activation and sarcoplasmic reticulum calcium release. J Gen Physiol. 1990 Nov;96(5):1085–1103. doi: 10.1085/jgp.96.5.1085. [DOI] [PMC free article] [PubMed] [Google Scholar]
- Thévenod F., Dehlinger-Kremer M., Kemmer T. P., Christian A. L., Potter B. V., Schulz I. Characterization of inositol 1,4,5-trisphosphate-sensitive (IsCaP) and -insensitive (IisCaP) nonmitochondrial Ca2+ pools in rat pancreatic acinar cells. J Membr Biol. 1989 Jul;109(2):173–186. doi: 10.1007/BF01870856. [DOI] [PubMed] [Google Scholar]
- Wetzel P., Liebner T., Gros G. Carbonic anhydrase inhibition and calcium transients in soleus fibers. FEBS Lett. 1990 Jul 2;267(1):66–70. doi: 10.1016/0014-5793(90)80289-u. [DOI] [PubMed] [Google Scholar]
- van Breda E., Keizer H. A., Glatz J. F., Geurten P. Use of the intact mouse skeletal-muscle preparation for metabolic studies. Evaluation of the model. Biochem J. 1990 Apr 1;267(1):257–260. doi: 10.1042/bj2670257. [DOI] [PMC free article] [PubMed] [Google Scholar]