Abstract
1. Voltage signals of about 1 mV evoked in photoreceptors of the drone honey bee by shallow modulation of a background illumination of an intensity useful for behaviour are thought to be amplified by voltage-dependent Na+ channels. To elucidate the roles of the various membrane conductances in this amplification we have studied the effects of the Na+ channel blocker tetrodotoxin (TTX) and various putative K+ channel blockers on the membrane potential, Vm. 2. Superfusion of a slice of retina with 0.5-10 mM-4-aminopyridine (4-AP) depolarized the membrane and, in fifty of sixty-three cells induced repetitive action potentials. Ionophoretic injection of tetraethylammonium produced similar effects. 3. In order to measure the depolarization caused by 4-AP, action potentials were prevented by application of TTX: 4-AP was applied when the membrane was depolarized to different levels by light. 4-AP induced an additional depolarization at all membrane potentials tested (-64 to -27 mV). We conclude that there are 4-AP-sensitive K+ channels that are open at constant voltage over this range. 4. 4-AP slowed down the recovery phase of the action potential induced by a light flash by a factor that ranged from 0.51 to 0.16. This reduction could be accounted for by the reduction in a voltage-independent K+ conductance estimated from the steady-state depolarization. 5. After the voltage-gated Na+ channels had been blocked by TTX, exposure to 4-AP further changed the amplitude of the response to a small (approximately 10%) decremental light stimulus. The change was an increase when the background illumination brought Vm to potentials more negative than about -40 mV; it was a decrease when Vm > -40 mV. The data could be fitted by a circuit representation of the membrane with a light-activated conductance and a K+ conductance (EK = -66 mV) that was partly blocked by 4-AP. The voltage range studied was from -52 to -27 mV; neither conductance in the model was voltage dependent. 6. The responses to small changes in light intensity in the absence of TTX were mimicked by a model. We conclude that a voltage-dependent Na+ conductance described by the Hodgkin-Huxley equations can amplify small voltage changes in a cell membrane that is also capable of generating action potentials; the magnitude of the K+ conductance is critical for optimization of signals while avoiding membrane instability.
Full text
PDF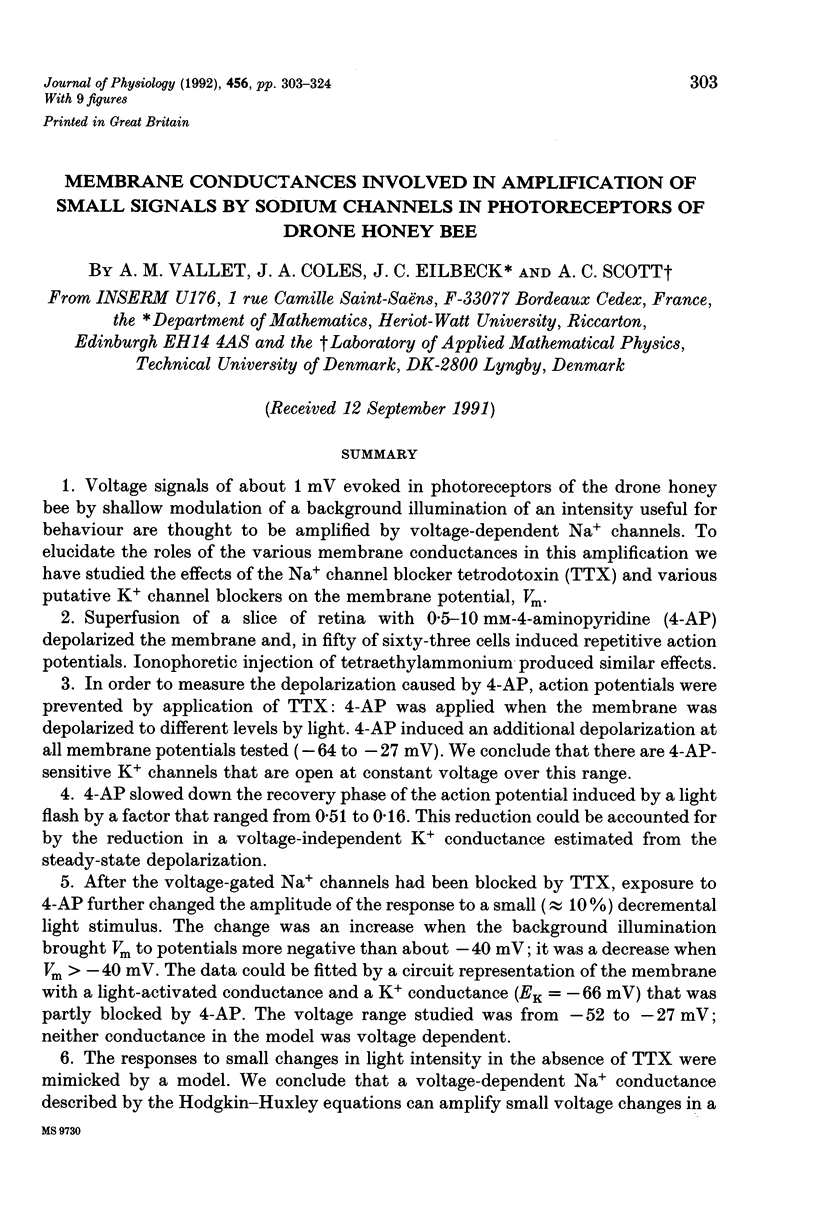
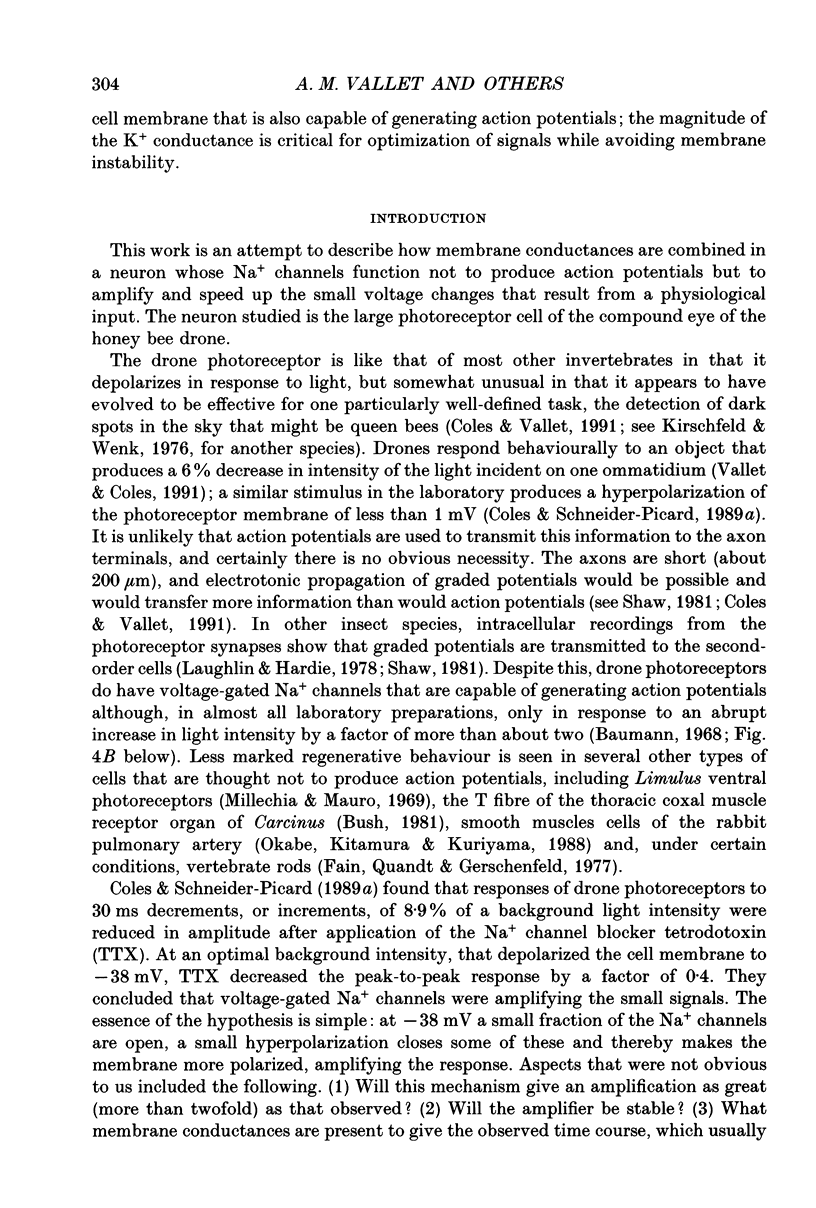
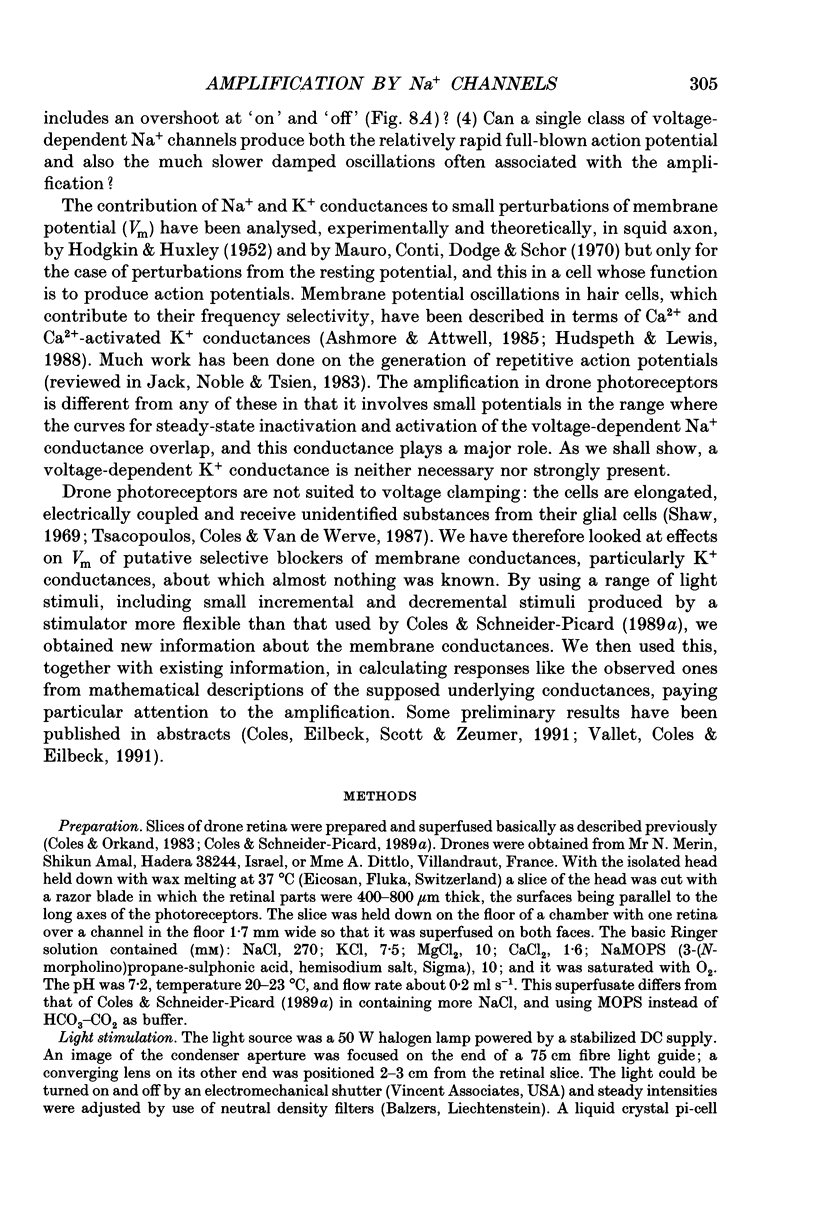
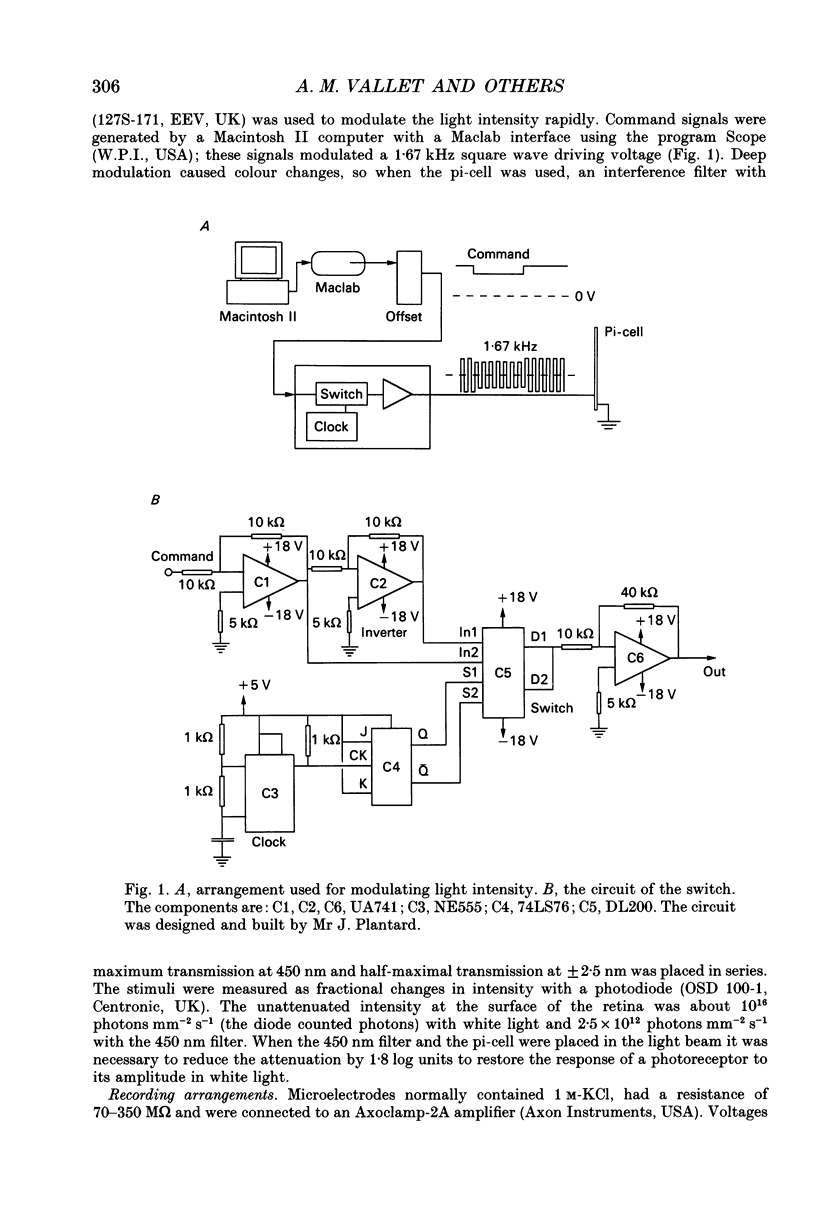
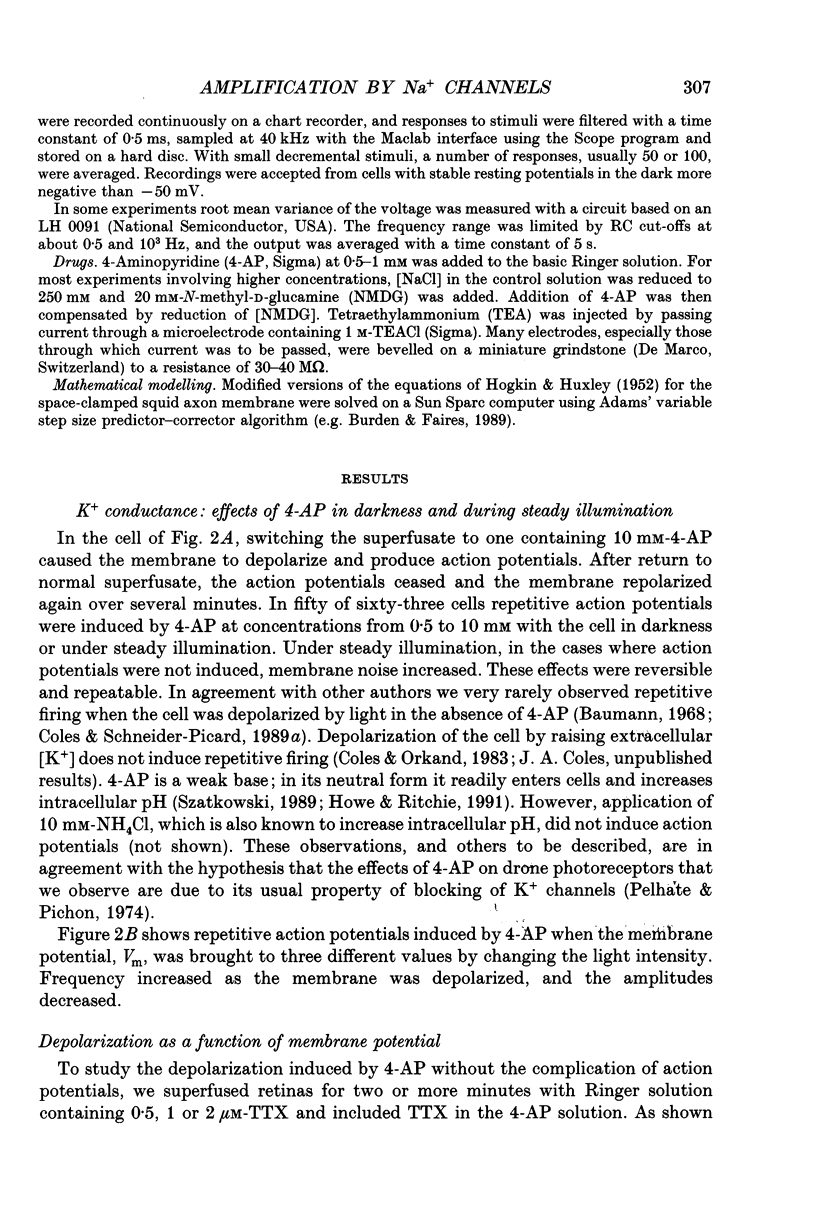
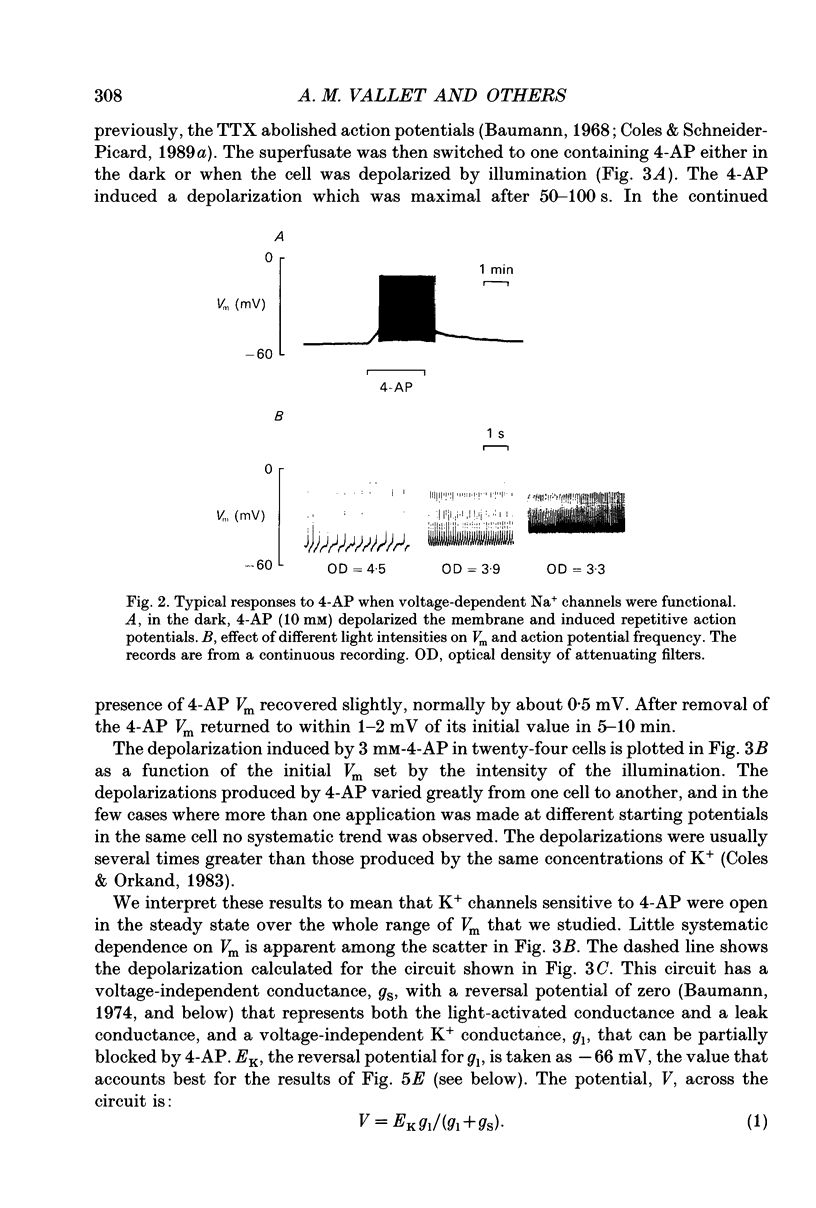
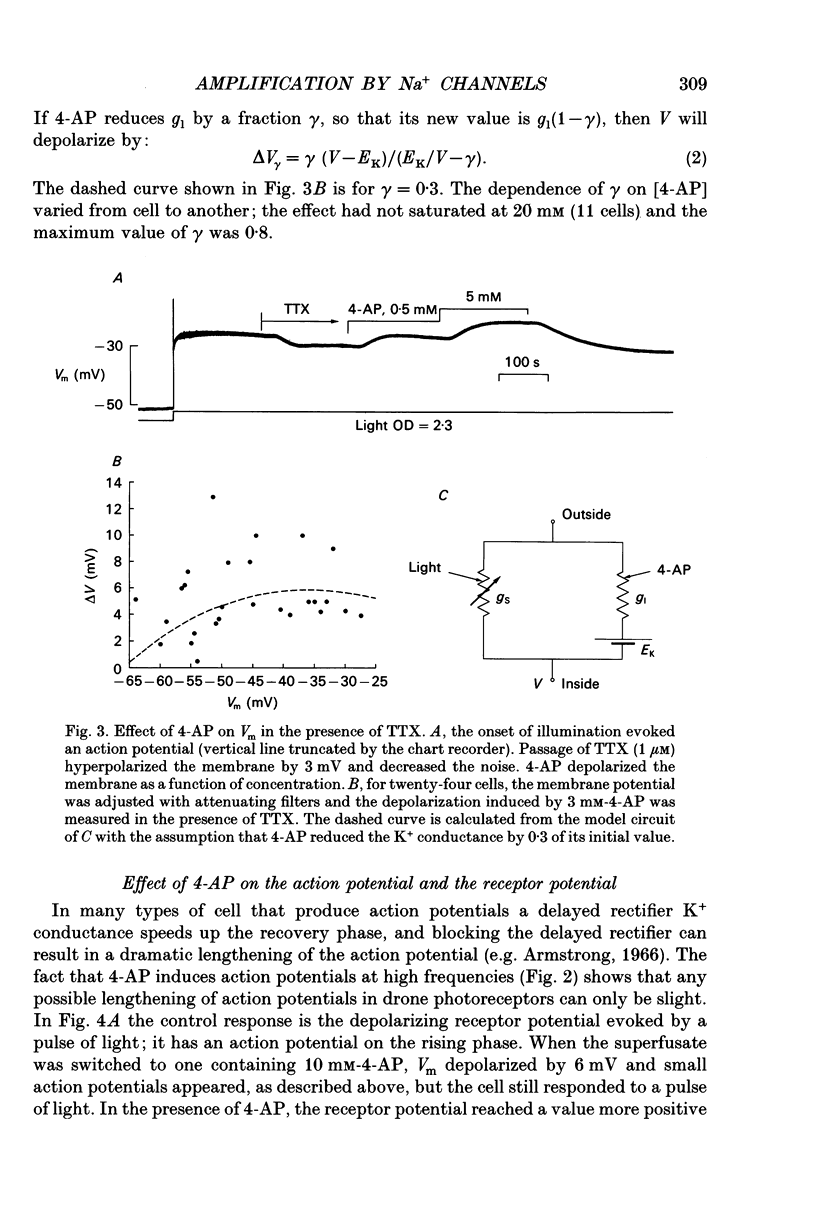
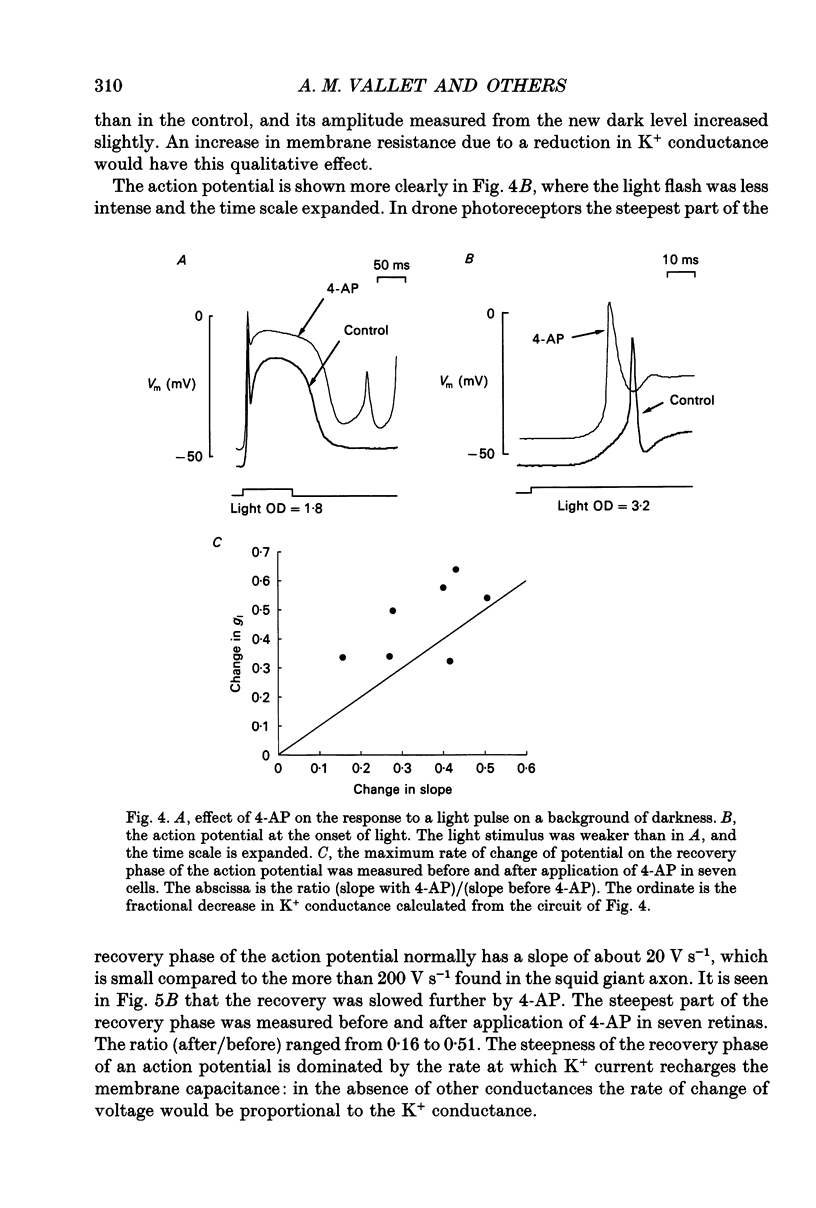
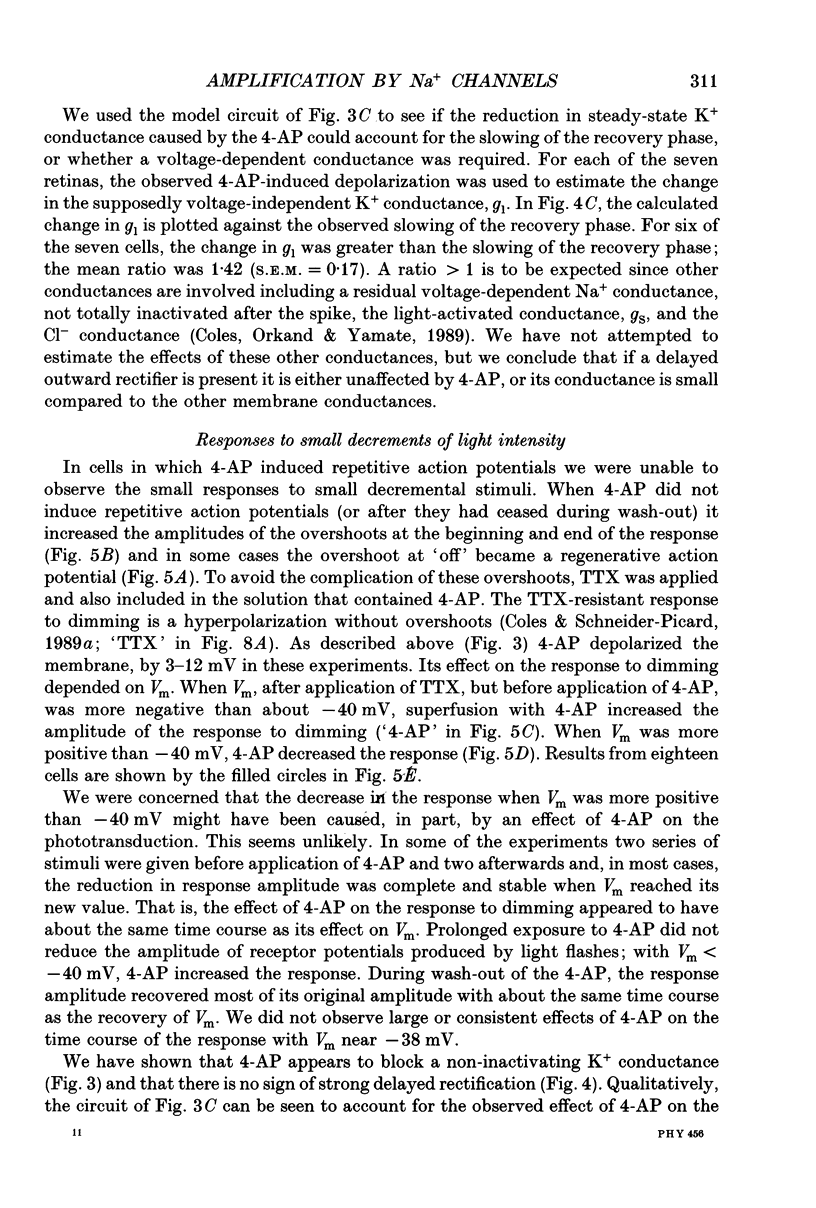
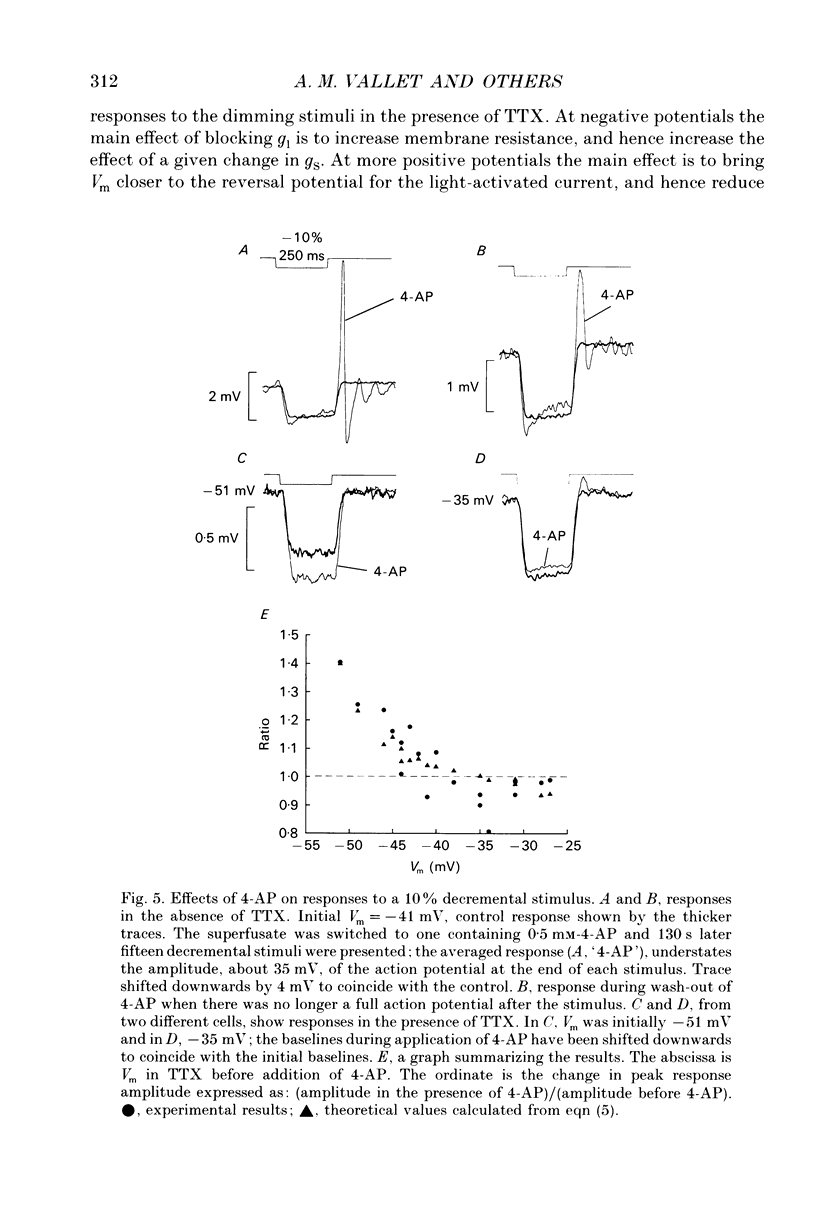
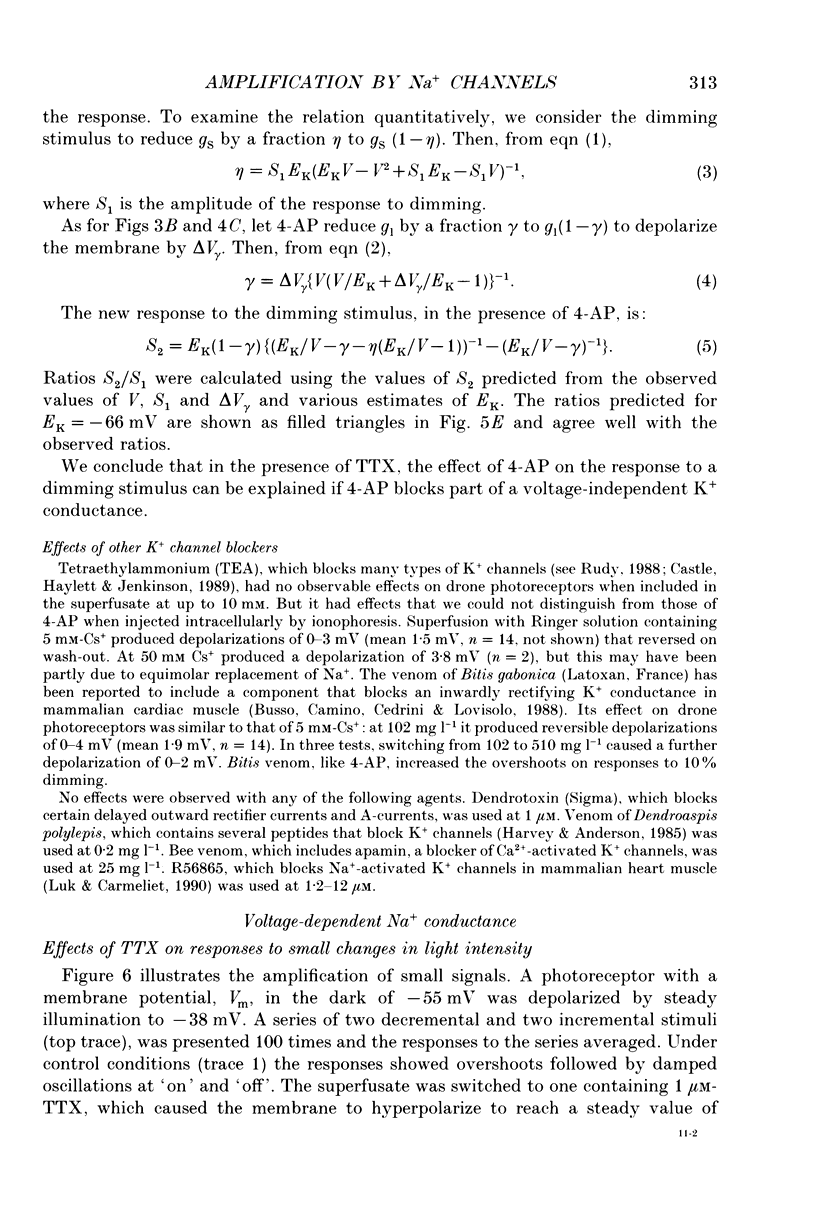
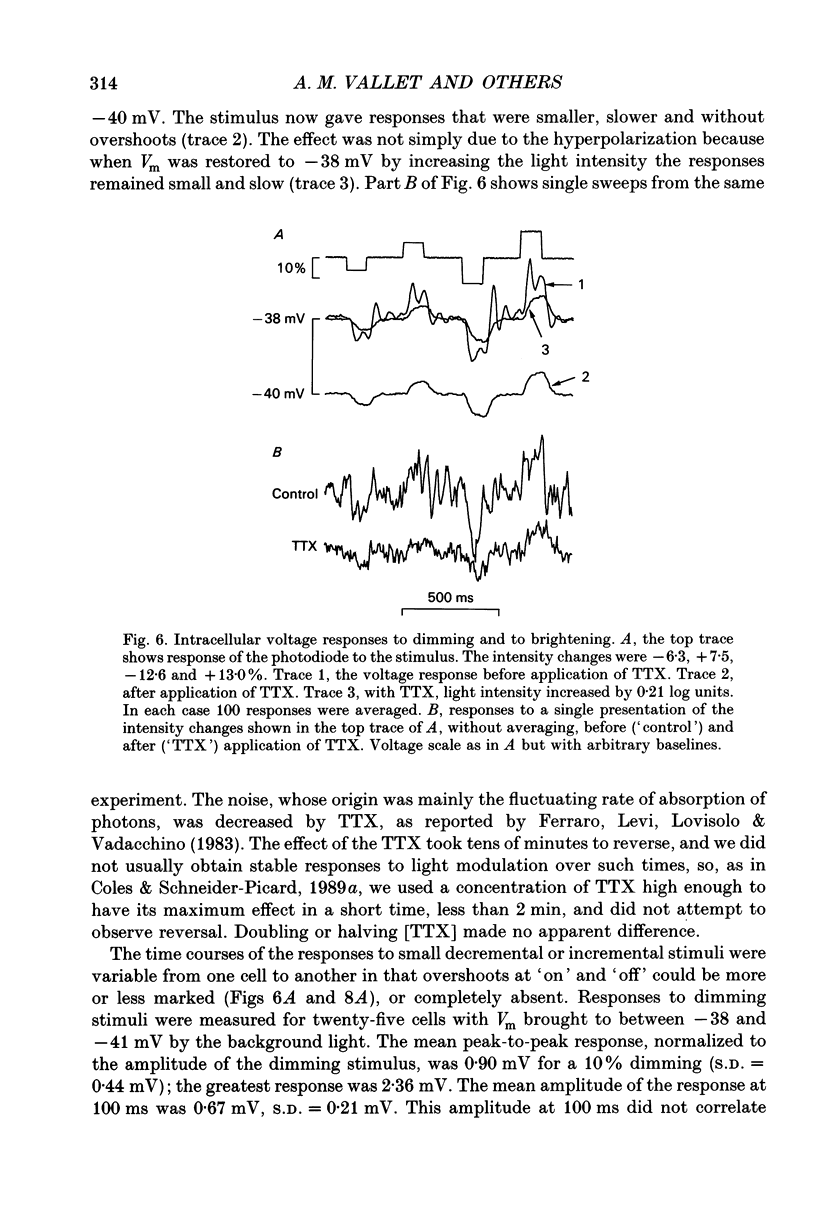
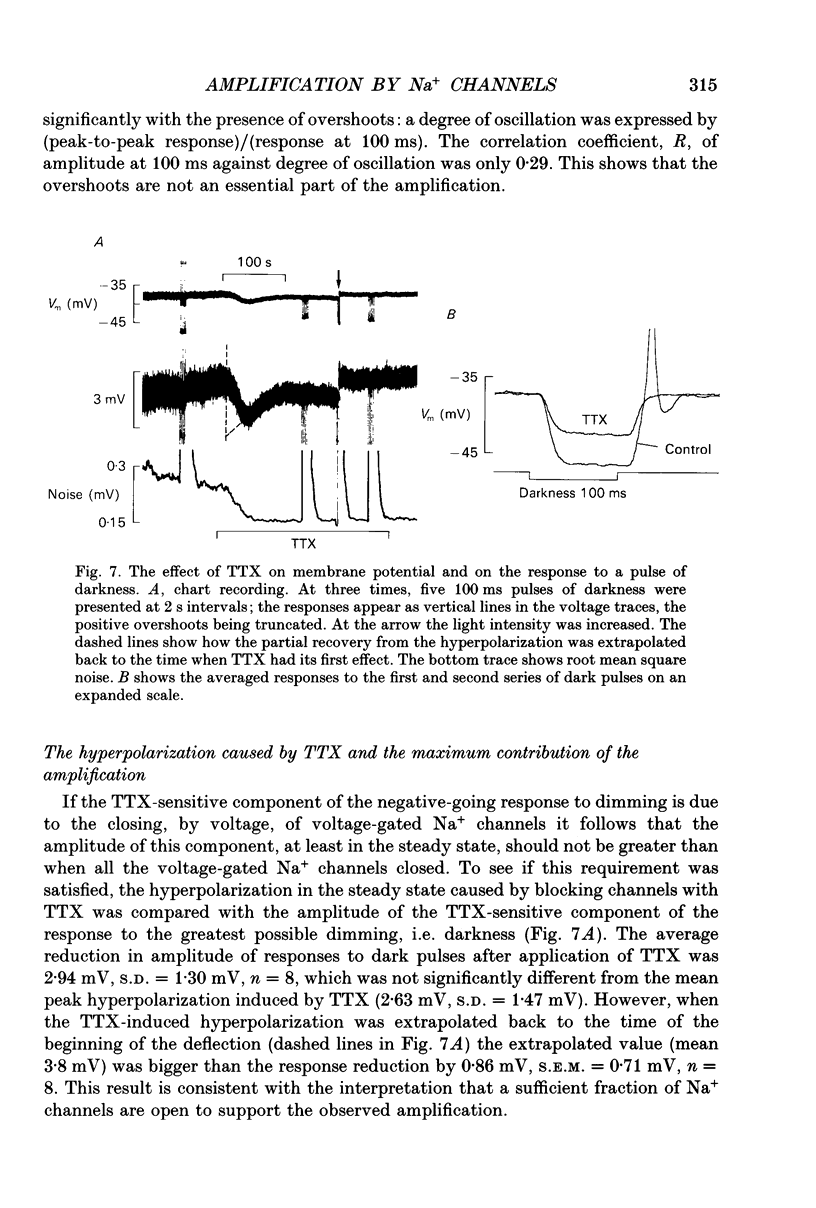
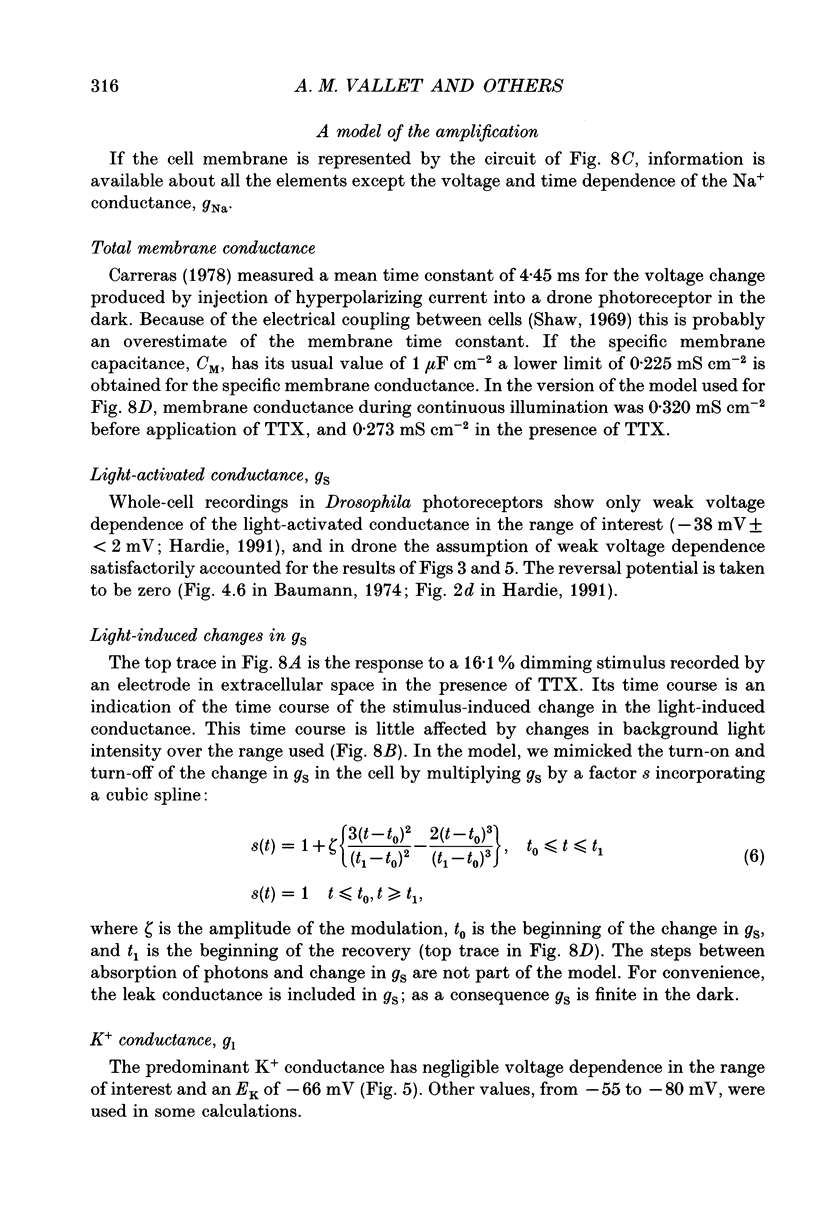
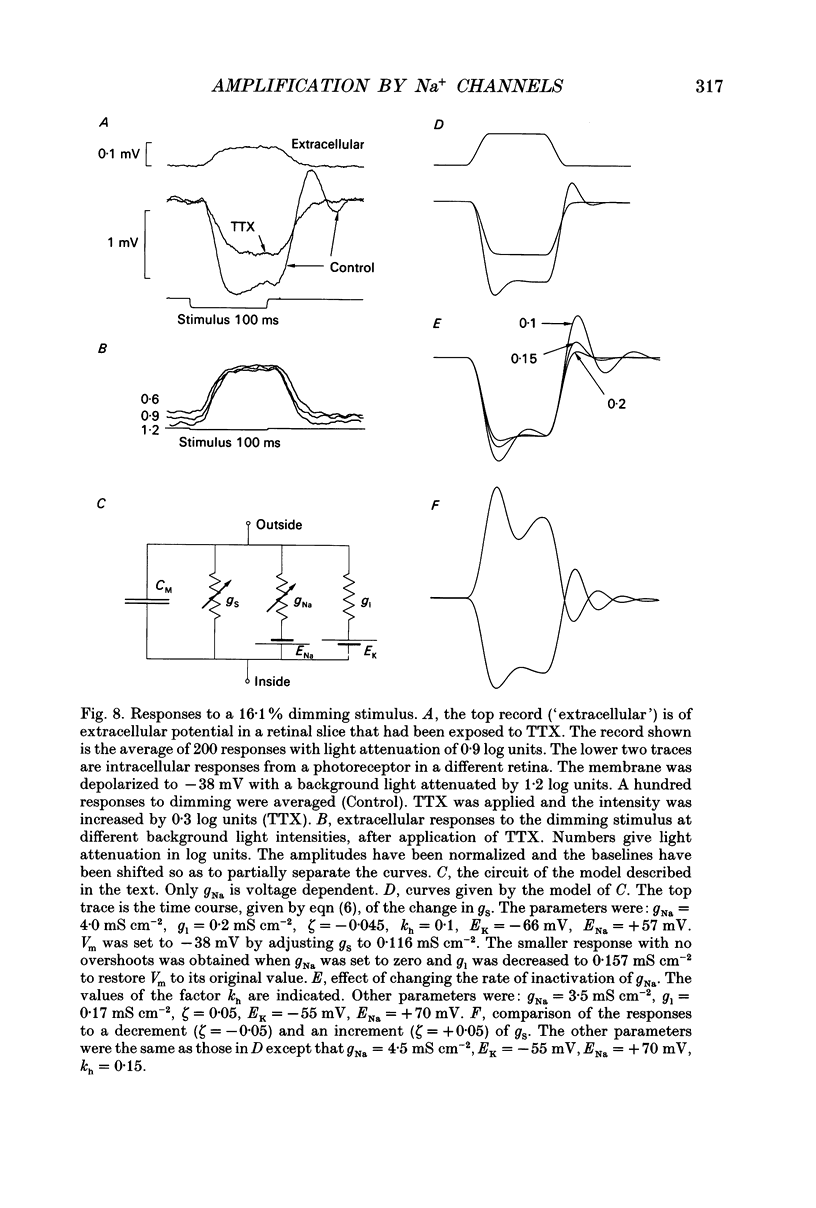
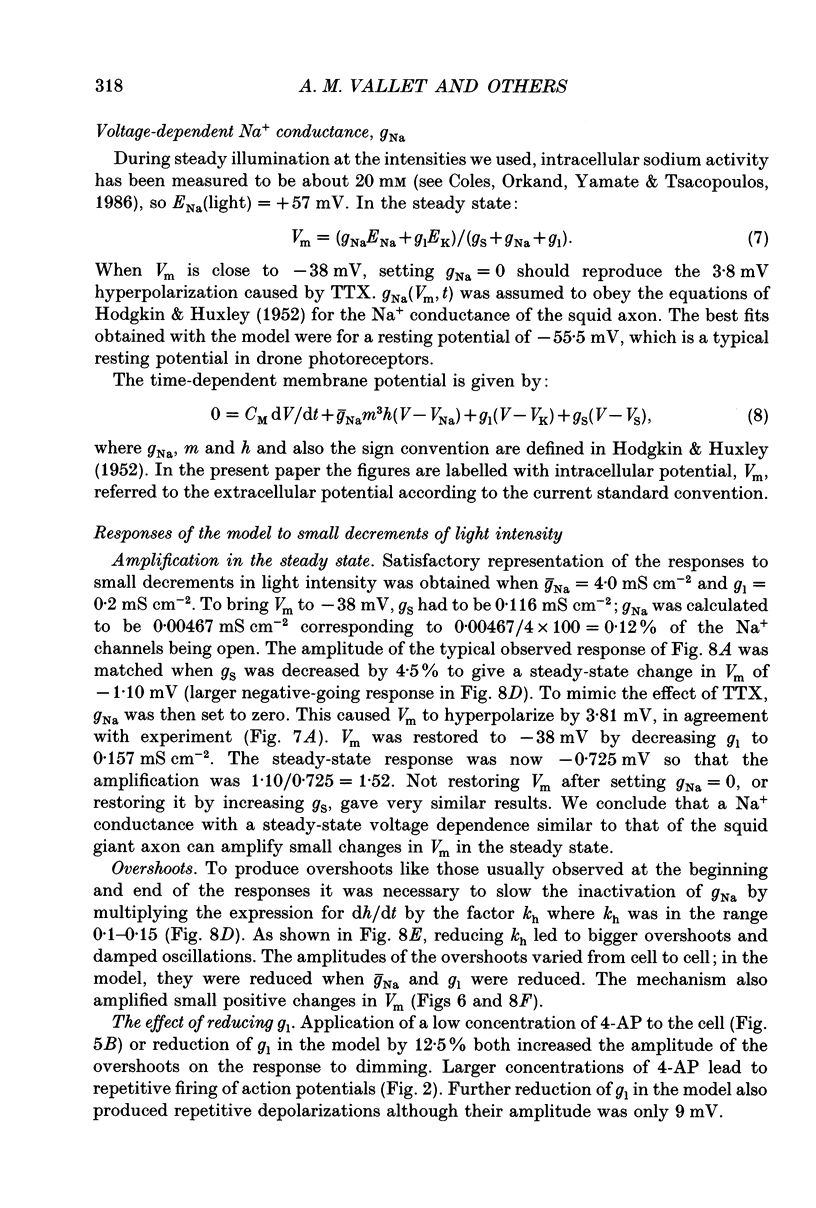
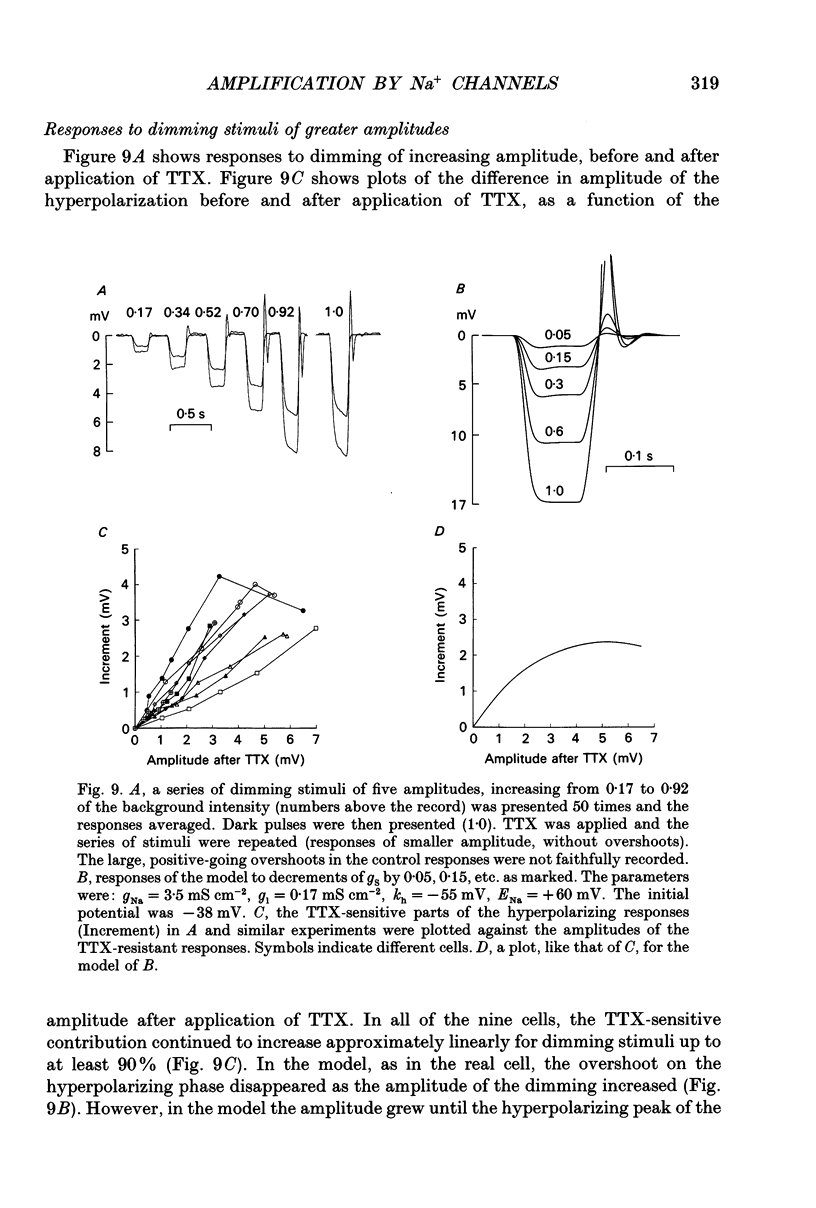
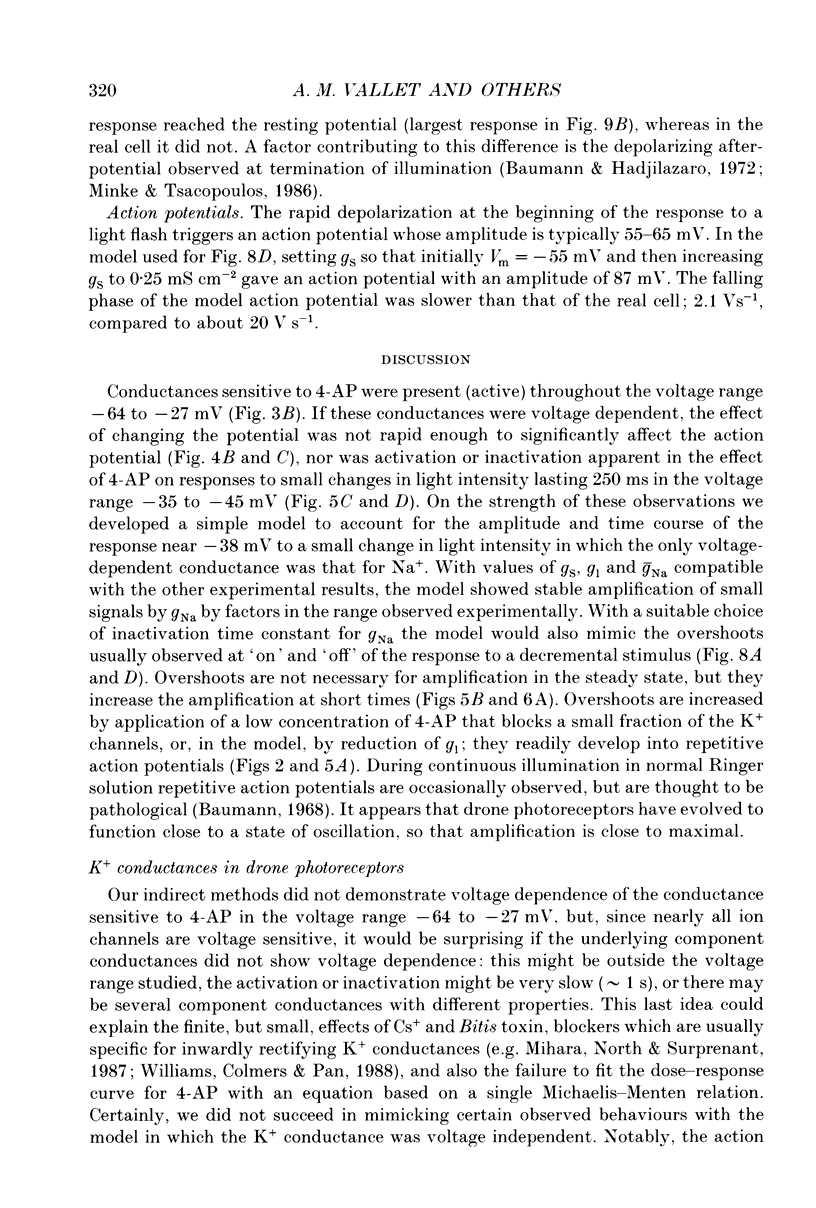
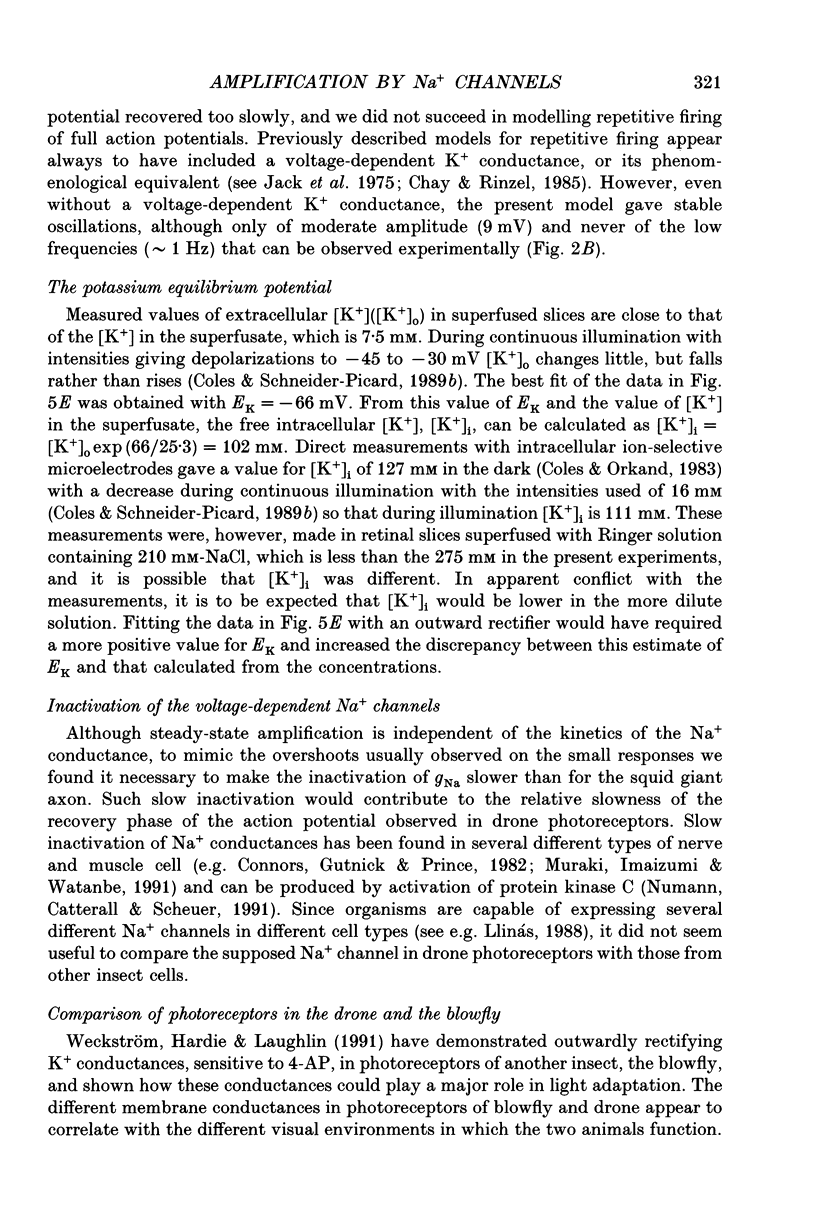
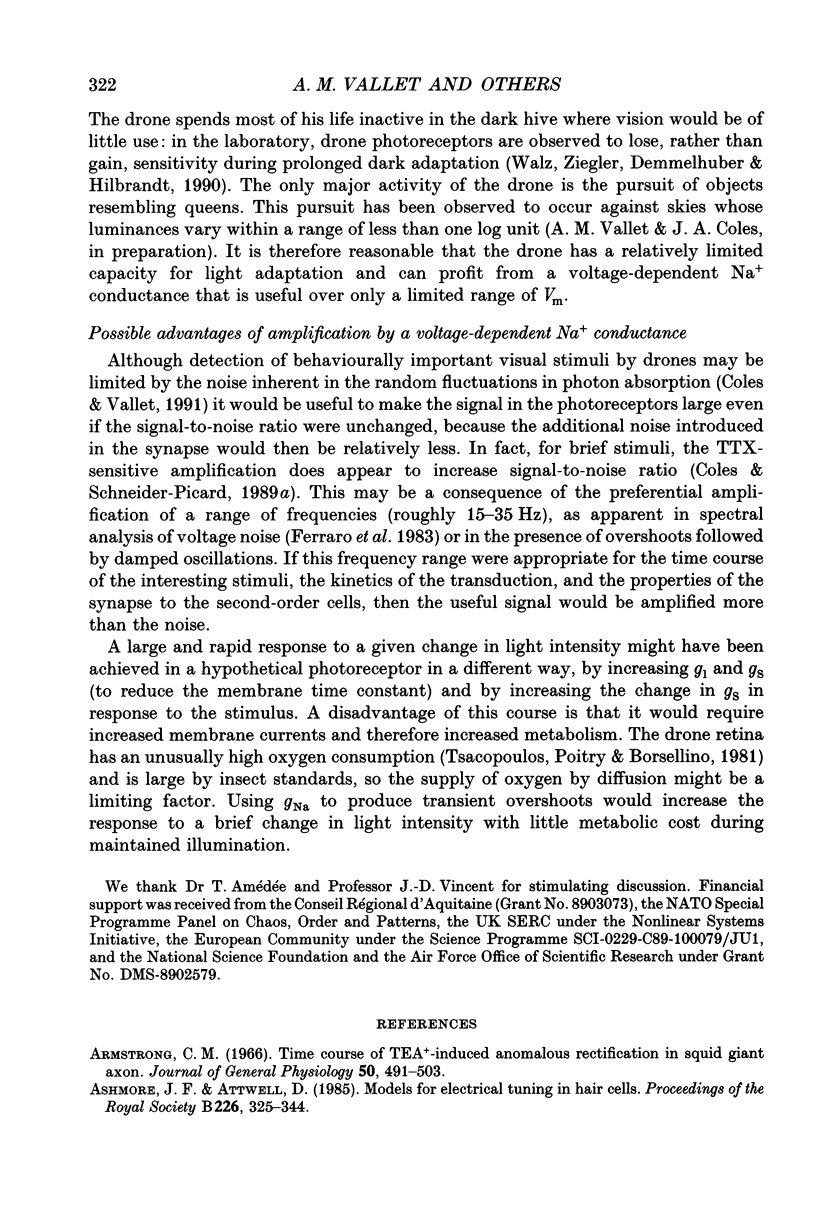
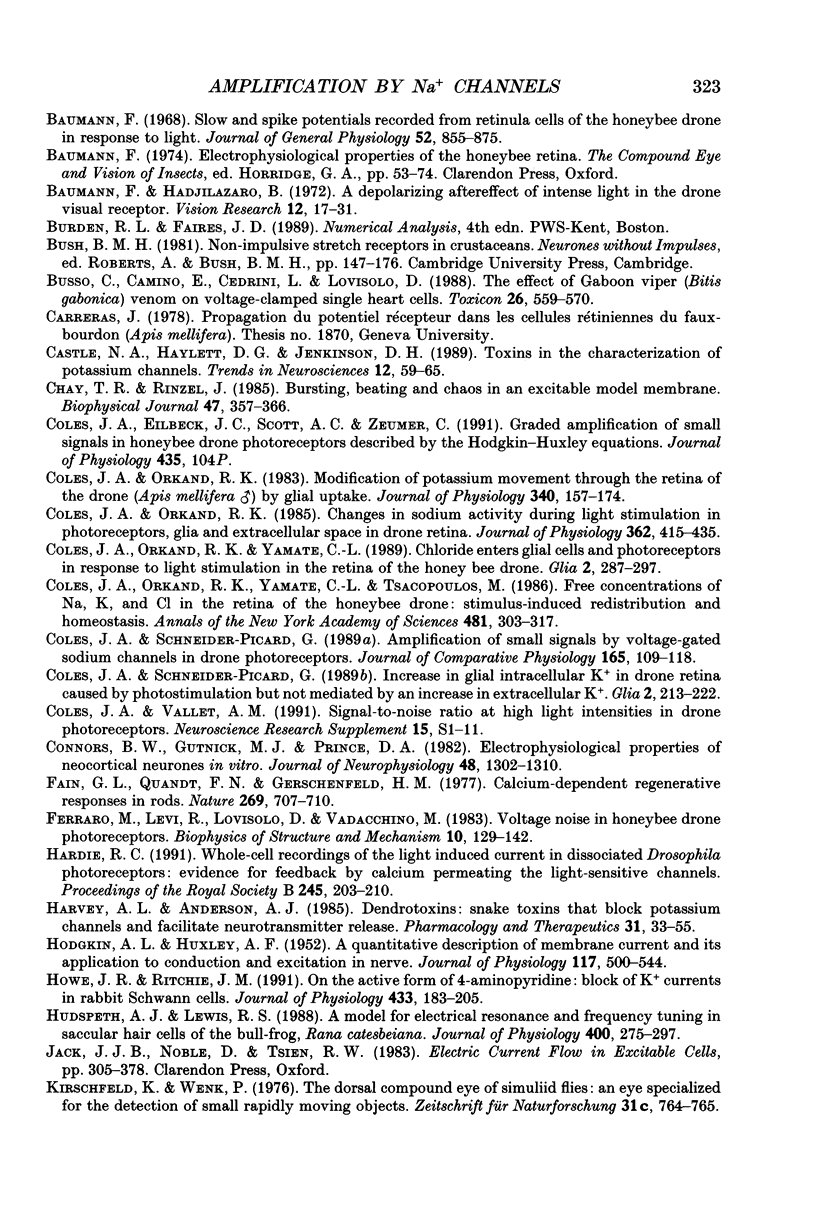
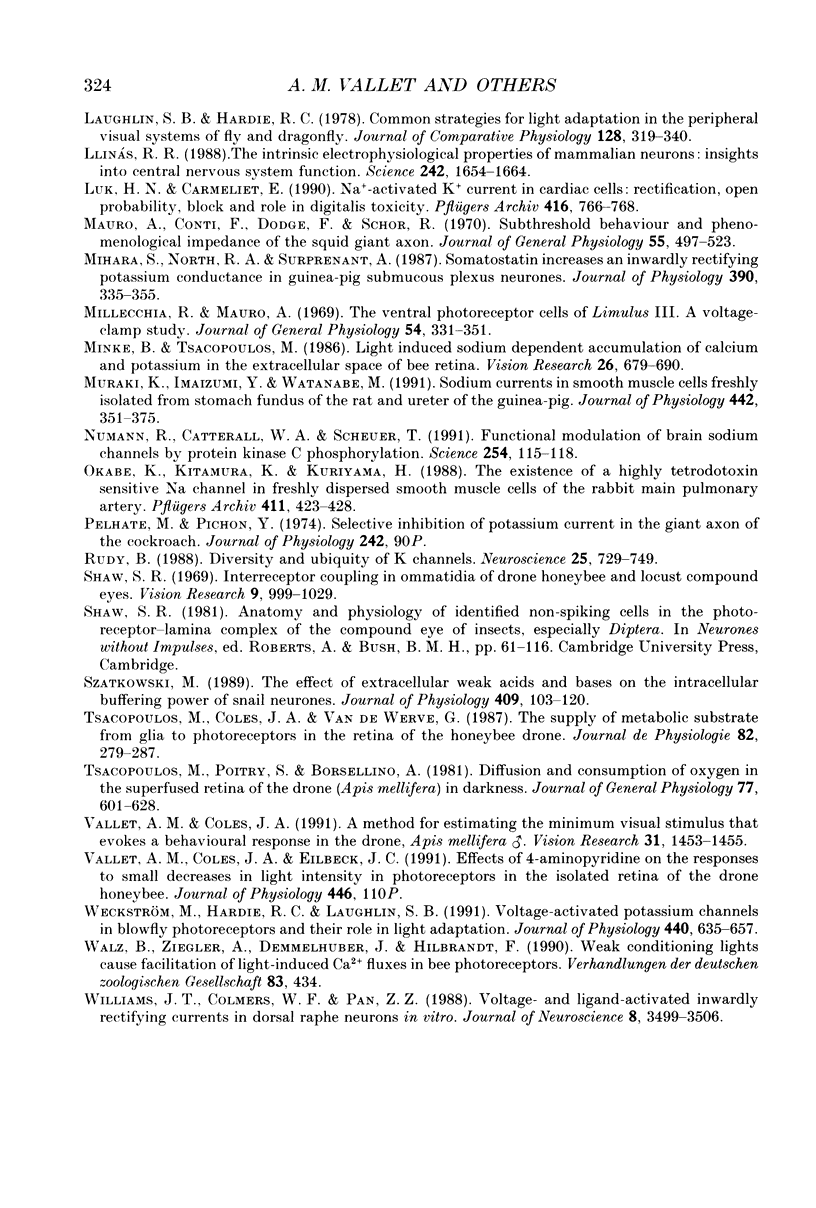
Selected References
These references are in PubMed. This may not be the complete list of references from this article.
- Armstrong C. M. Time course of TEA(+)-induced anomalous rectification in squid giant axons. J Gen Physiol. 1966 Nov;50(2):491–503. doi: 10.1085/jgp.50.2.491. [DOI] [PMC free article] [PubMed] [Google Scholar]
- Baumann F., Hadjilazaro B. A depolarizing aftereffect of intense light in the drone visual receptor. Vision Res. 1972 Jan;12(1):17–31. doi: 10.1016/0042-6989(72)90134-4. [DOI] [PubMed] [Google Scholar]
- Baumann F. Slow and spike potentials recorded from retinula cells of the honeybee drone in response to light. J Gen Physiol. 1968 Dec;52(6):855–875. doi: 10.1085/jgp.52.6.855. [DOI] [PMC free article] [PubMed] [Google Scholar]
- Busso C., Camino E., Cedrini L., Lovisolo D. The effects of gaboon viper (Bitis gabonica) venom on voltage-clamped single heart cells. Toxicon. 1988;26(6):559–570. doi: 10.1016/0041-0101(88)90236-x. [DOI] [PubMed] [Google Scholar]
- Castle N. A., Haylett D. G., Jenkinson D. H. Toxins in the characterization of potassium channels. Trends Neurosci. 1989 Feb;12(2):59–65. doi: 10.1016/0166-2236(89)90137-9. [DOI] [PubMed] [Google Scholar]
- Chay T. R., Rinzel J. Bursting, beating, and chaos in an excitable membrane model. Biophys J. 1985 Mar;47(3):357–366. doi: 10.1016/S0006-3495(85)83926-6. [DOI] [PMC free article] [PubMed] [Google Scholar]
- Coles J. A., Orkand R. K. Changes in sodium activity during light stimulation in photoreceptors, glia and extracellular space in drone retina. J Physiol. 1985 May;362:415–435. doi: 10.1113/jphysiol.1985.sp015686. [DOI] [PMC free article] [PubMed] [Google Scholar]
- Coles J. A., Orkand R. K. Modification of potassium movement through the retina of the drone (Apis mellifera male) by glial uptake. J Physiol. 1983 Jul;340:157–174. doi: 10.1113/jphysiol.1983.sp014756. [DOI] [PMC free article] [PubMed] [Google Scholar]
- Coles J. A., Orkand R. K., Yamate C. L. Chloride enters glial cells and photoreceptors in response to light stimulation in the retina of the honey bee drone. Glia. 1989;2(5):287–297. doi: 10.1002/glia.440020502. [DOI] [PubMed] [Google Scholar]
- Coles J. A., Orkand R. K., Yamate C. L., Tsacopoulos M. Free concentrations of Na, K, and Cl in the retina of the honeybee drone: stimulus-induced redistribution and homeostasis. Ann N Y Acad Sci. 1986;481:303–317. doi: 10.1111/j.1749-6632.1986.tb27160.x. [DOI] [PubMed] [Google Scholar]
- Coles J. A., Schneider-Picard G. Amplification of small signals by voltage-gated sodium channels in drone photoreceptors. J Comp Physiol A. 1989 Apr;165(1):109–118. doi: 10.1007/BF00613804. [DOI] [PubMed] [Google Scholar]
- Coles J. A., Schneider-Picard G. Increase in glial intracellular K+ in drone retina caused by photostimulation but not mediated by an increase in extracellular K+. Glia. 1989;2(4):213–222. doi: 10.1002/glia.440020402. [DOI] [PubMed] [Google Scholar]
- Coles J. A., Vallet A. M. Signal-to-noise ratio at high light intensities in drone photoreceptors. Neurosci Res Suppl. 1991;15:S1–11. [PubMed] [Google Scholar]
- Connors B. W., Gutnick M. J., Prince D. A. Electrophysiological properties of neocortical neurons in vitro. J Neurophysiol. 1982 Dec;48(6):1302–1320. doi: 10.1152/jn.1982.48.6.1302. [DOI] [PubMed] [Google Scholar]
- Fain G. L., Quandt F. N., Gerschenfeld H. M. Calcium-dependent regenerative responses in rods. Nature. 1977 Oct 20;269(5630):707–710. doi: 10.1038/269707a0. [DOI] [PubMed] [Google Scholar]
- HODGKIN A. L., HUXLEY A. F. A quantitative description of membrane current and its application to conduction and excitation in nerve. J Physiol. 1952 Aug;117(4):500–544. doi: 10.1113/jphysiol.1952.sp004764. [DOI] [PMC free article] [PubMed] [Google Scholar]
- Harvey A. L., Anderson A. J. Dendrotoxins: snake toxins that block potassium channels and facilitate neurotransmitter release. Pharmacol Ther. 1985;31(1-2):33–55. doi: 10.1016/0163-7258(85)90036-1. [DOI] [PubMed] [Google Scholar]
- Howe J. R., Ritchie J. M. On the active form of 4-aminopyridine: block of K+ currents in rabbit Schwann cells. J Physiol. 1991 Feb;433:183–205. doi: 10.1113/jphysiol.1991.sp018421. [DOI] [PMC free article] [PubMed] [Google Scholar]
- Hudspeth A. J., Lewis R. S. A model for electrical resonance and frequency tuning in saccular hair cells of the bull-frog, Rana catesbeiana. J Physiol. 1988 Jun;400:275–297. doi: 10.1113/jphysiol.1988.sp017120. [DOI] [PMC free article] [PubMed] [Google Scholar]
- Kirschfeld K., Wenk P. The dorsal compound eye of simuliid flies: an eye specialized for the detection of small, rapidly moving objects. Z Naturforsch C. 1976 Nov-Dec;31(11-12):764–765. [PubMed] [Google Scholar]
- Llinás R. R. The intrinsic electrophysiological properties of mammalian neurons: insights into central nervous system function. Science. 1988 Dec 23;242(4886):1654–1664. doi: 10.1126/science.3059497. [DOI] [PubMed] [Google Scholar]
- Luk H. N., Carmeliet E. Na(+)-activated K+ current in cardiac cells: rectification, open probability, block and role in digitalis toxicity. Pflugers Arch. 1990 Aug;416(6):766–768. doi: 10.1007/BF00370627. [DOI] [PubMed] [Google Scholar]
- Mauro A., Conti F., Dodge F., Schor R. Subthreshold behavior and phenomenological impedance of the squid giant axon. J Gen Physiol. 1970 Apr;55(4):497–523. doi: 10.1085/jgp.55.4.497. [DOI] [PMC free article] [PubMed] [Google Scholar]
- Mihara S., North R. A., Surprenant A. Somatostatin increases an inwardly rectifying potassium conductance in guinea-pig submucous plexus neurones. J Physiol. 1987 Sep;390:335–355. doi: 10.1113/jphysiol.1987.sp016704. [DOI] [PMC free article] [PubMed] [Google Scholar]
- Millecchia R., Mauro A. The ventral photoreceptor cells of Limulus. 3. A voltage-clamp study. J Gen Physiol. 1969 Sep;54(3):331–351. doi: 10.1085/jgp.54.3.331. [DOI] [PMC free article] [PubMed] [Google Scholar]
- Minke B., Tsacopoulos M. Light induced sodium dependent accumulation of calcium and potassium in the extracellular space of bee retina. Vision Res. 1986;26(5):679–690. doi: 10.1016/0042-6989(86)90082-9. [DOI] [PubMed] [Google Scholar]
- Muraki K., Imaizumi Y., Watanabe M. Sodium currents in smooth muscle cells freshly isolated from stomach fundus of the rat and ureter of the guinea-pig. J Physiol. 1991 Oct;442:351–375. doi: 10.1113/jphysiol.1991.sp018797. [DOI] [PMC free article] [PubMed] [Google Scholar]
- Numann R., Catterall W. A., Scheuer T. Functional modulation of brain sodium channels by protein kinase C phosphorylation. Science. 1991 Oct 4;254(5028):115–118. doi: 10.1126/science.1656525. [DOI] [PubMed] [Google Scholar]
- Okabe K., Kitamura K., Kuriyama H. The existence of a highly tetrodotoxin sensitive Na channel in freshly dispersed smooth muscle cells of the rabbit main pulmonary artery. Pflugers Arch. 1988 Apr;411(4):423–428. doi: 10.1007/BF00587722. [DOI] [PubMed] [Google Scholar]
- Pelhate M., Pichon Y. Proceedings: Selective inhibition of potassium current in the giant axon of the cockroach. J Physiol. 1974 Oct;242(2):90P–91P. [PubMed] [Google Scholar]
- Rudy B. Diversity and ubiquity of K channels. Neuroscience. 1988 Jun;25(3):729–749. doi: 10.1016/0306-4522(88)90033-4. [DOI] [PubMed] [Google Scholar]
- Shaw S. R. Interreceptor coupling in ommatidia of drone honeybee and locust compound eyes. Vision Res. 1969 Sep;9(9):999–1029. doi: 10.1016/0042-6989(69)90044-3. [DOI] [PubMed] [Google Scholar]
- Szatkowski M. S. The effect of extracellular weak acids and bases on the intracellular buffering power of snail neurones. J Physiol. 1989 Feb;409:103–120. doi: 10.1113/jphysiol.1989.sp017487. [DOI] [PMC free article] [PubMed] [Google Scholar]
- Tsacopoulos M., Coles J. A., Van de Werve G. The supply of metabolic substrate from glia to photoreceptors in the retina of the honeybee drone. J Physiol (Paris) 1987;82(4):279–287. [PubMed] [Google Scholar]
- Tsacopoulos M., Poitry S., Borsellino A. Diffusion and consumption of oxygen in the superfused retina of the drone (Apis mellifera) in darkness. J Gen Physiol. 1981 Jun;77(6):601–628. doi: 10.1085/jgp.77.6.601. [DOI] [PMC free article] [PubMed] [Google Scholar]
- Vallet A. M., Coles J. A. A method for estimating the minimum visual stimulus that evokes a behavioural response in the drone, Apis mellifera male. Vision Res. 1991;31(7-8):1453–1455. doi: 10.1016/0042-6989(91)90065-d. [DOI] [PubMed] [Google Scholar]
- Weckström M., Hardie R. C., Laughlin S. B. Voltage-activated potassium channels in blowfly photoreceptors and their role in light adaptation. J Physiol. 1991;440:635–657. doi: 10.1113/jphysiol.1991.sp018729. [DOI] [PMC free article] [PubMed] [Google Scholar]
- Williams J. T., Colmers W. F., Pan Z. Z. Voltage- and ligand-activated inwardly rectifying currents in dorsal raphe neurons in vitro. J Neurosci. 1988 Sep;8(9):3499–3506. doi: 10.1523/JNEUROSCI.08-09-03499.1988. [DOI] [PMC free article] [PubMed] [Google Scholar]