Abstract
1. Whole-cell K+ currents contributing to the resting membrane potential and repolarization of the action potential were studied in voltage-clamped parasympathetic neurones dissociated from neonatal rat intracardiac ganglia and maintained in tissue culture. 2. Rat intracardiac neurones had a mean resting membrane potential of -52 mV and mean input resistance of 850 M omega. The current-voltage relationship recorded during slow voltage ramps indicated the presence of both leakage and voltage-dependent currents. The contribution of Na+, K+ and Cl- to the resting membrane potential was examined and relative ionic permeabilities PNa/PK = 0.12 and PCl/PK < 0.001 were calculated using the Goldman-Hodgkin-Katz voltage equation. Bath application of the potassium channel blockers, tetraethylammonium ions (TEA; 1 mM) or Ba2+ (1 mM) depolarized the neurone by approximately 10 mV. Inhibition of the Na(+)-K+ pump by exposure to K(+)-free medium or by the addition of 0.1 mM ouabain to the bath solution depolarized the neurone by 3-5 mV. 3. In most neurones, depolarizing current pulses (0.5-1 s duration) elicited a single action potential of 85-100 mV, followed by an after-hyperpolarization of 200-500 ms. In 10-15% of the neurones, sustained current injection produced repetitive firing at maximal frequency of 5-8 Hz. 4. Tetrodotoxin (TTX; 300 nM) reduced, but failed to abolish, the action potential. The magnitude and duration of the TTX-insensitive action potential increased with the extracellular Ca2+ concentration, and was inhibited by bath application of 0.1 mM Cd2+. The repolarization rate of the TTX-insensitive action potential was reduced, and after-hyperpolarization was replaced by after-depolarization upon substitution of internal K+ by Cs+. The after-hyperpolarization of the action potential was reduced by bath application of Cd2+ (0.1 mM) and abolished by the addition of Cd2+ and TEA (10 mM). 5. Depolarization-activated outward K+ currents were isolated by adding 300 nM TTX and 0.1 mM Cd2+ to the external solution. The outward currents evoked by step depolarizations increased to a steady-state plateau which was maintained for > 5 s. The instantaneous current-voltage relationship, examined under varying external K+ concentrations, was linear, and the reversal (zero current) potential shifted in accordance with that predicted by the Nernst equation for a K(+)-selective electrode. The shift in reversal potential of the tail currents as a function of the extracellular K+ concentration gave a relative permeability, PNa/PK = 0.02 for the delayed outward K+ channel(s).(ABSTRACT TRUNCATED AT 400 WORDS)
Full text
PDF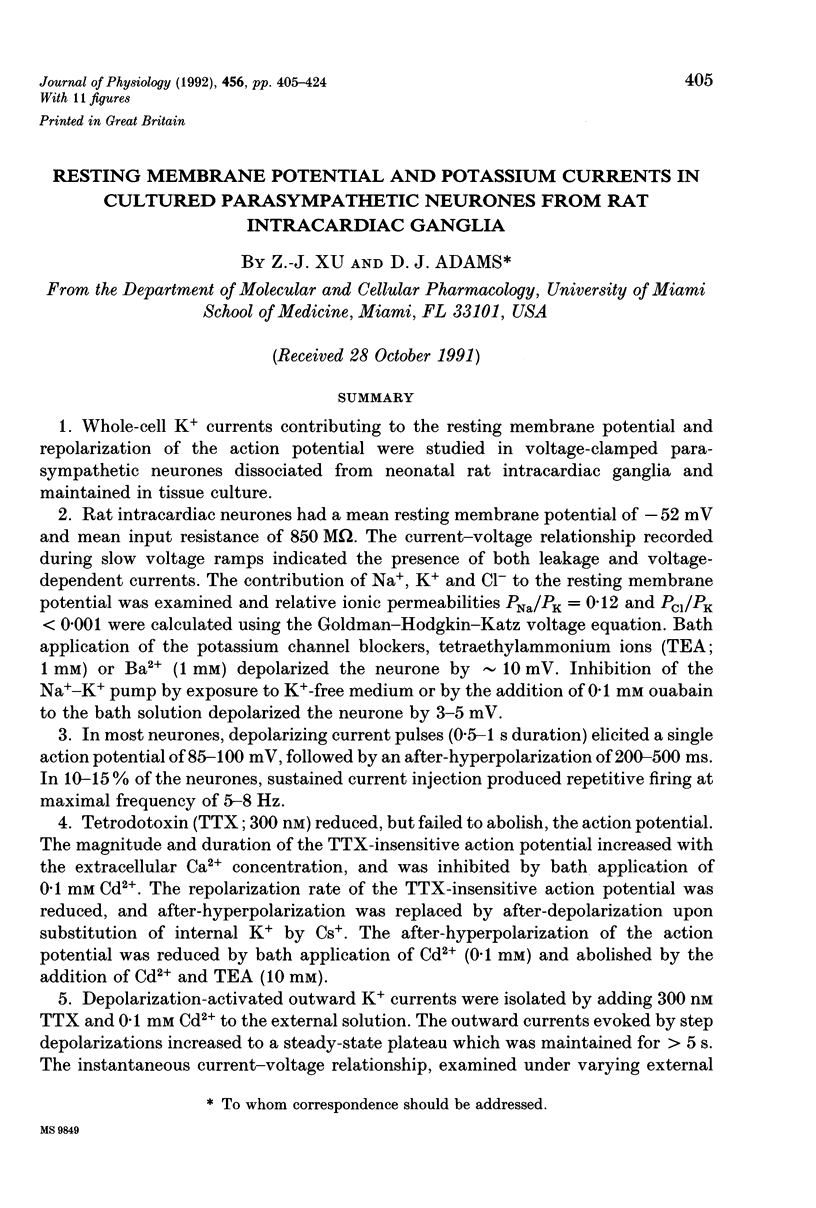
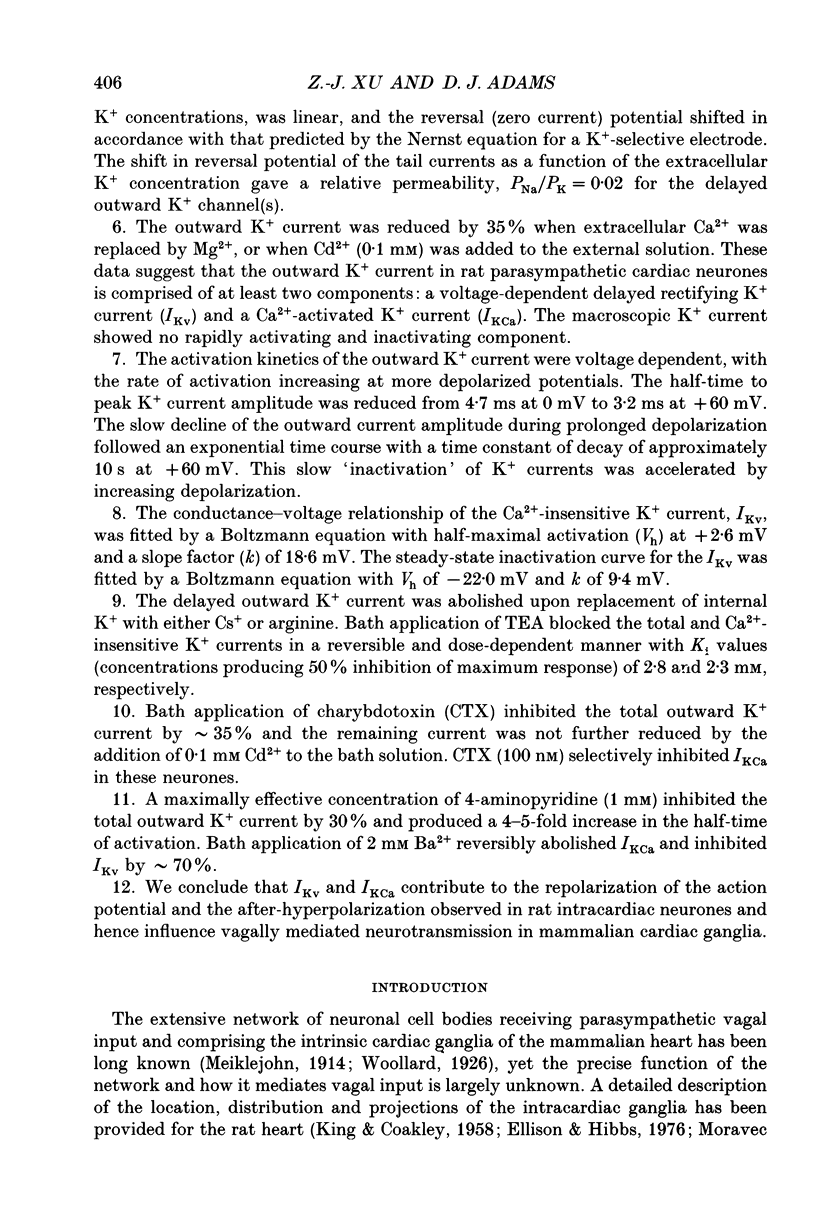
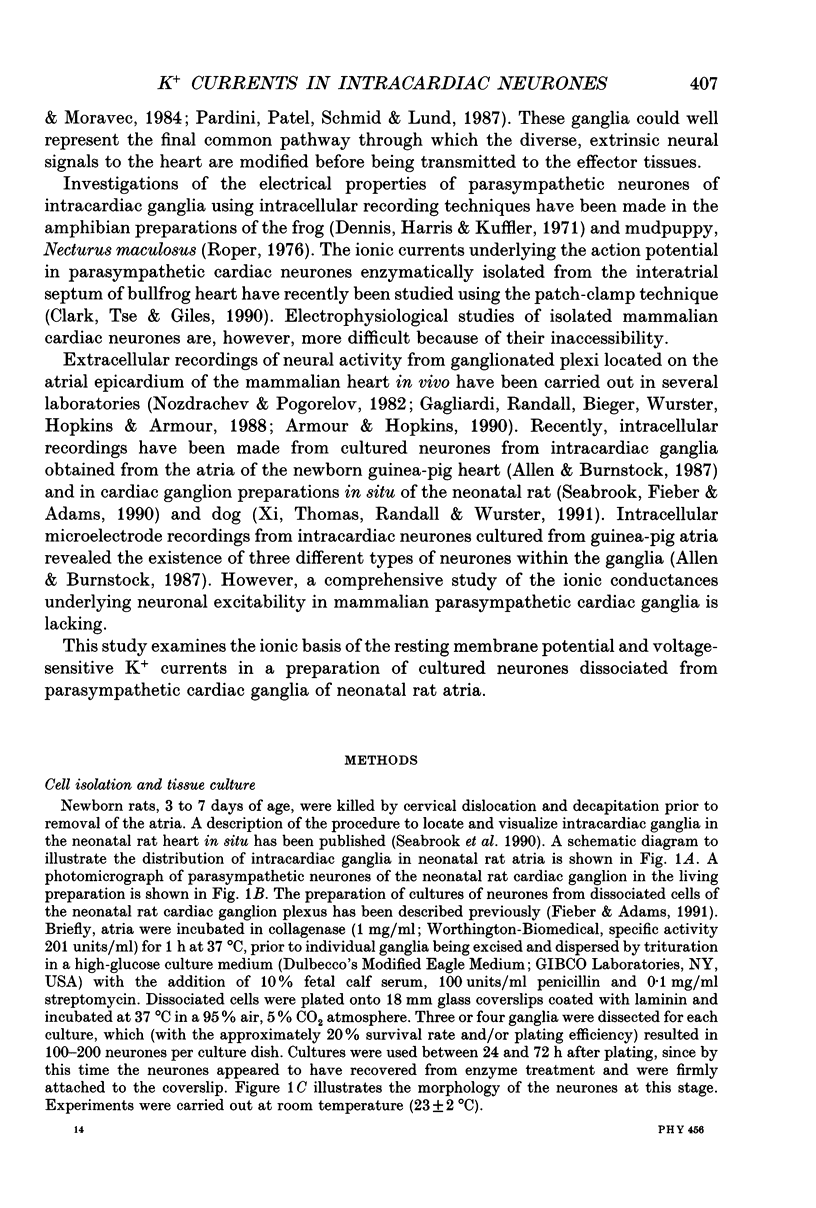
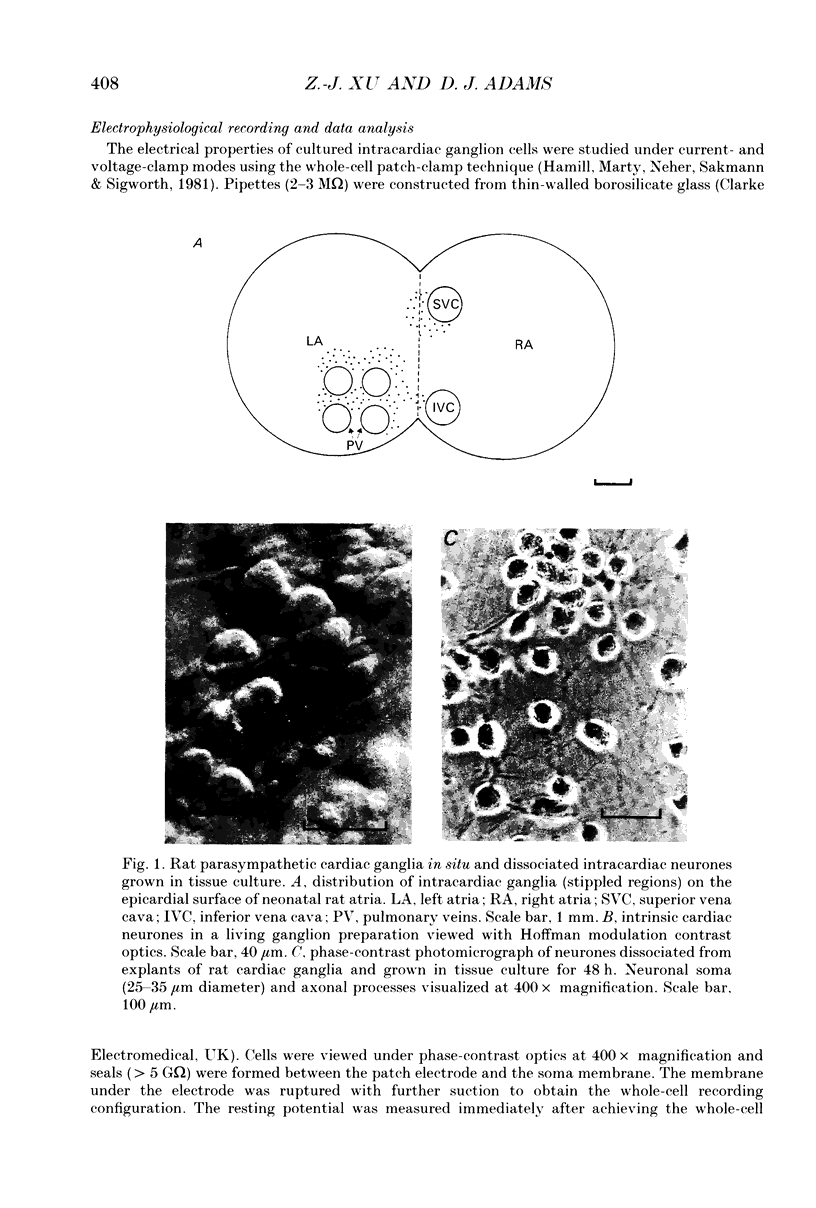
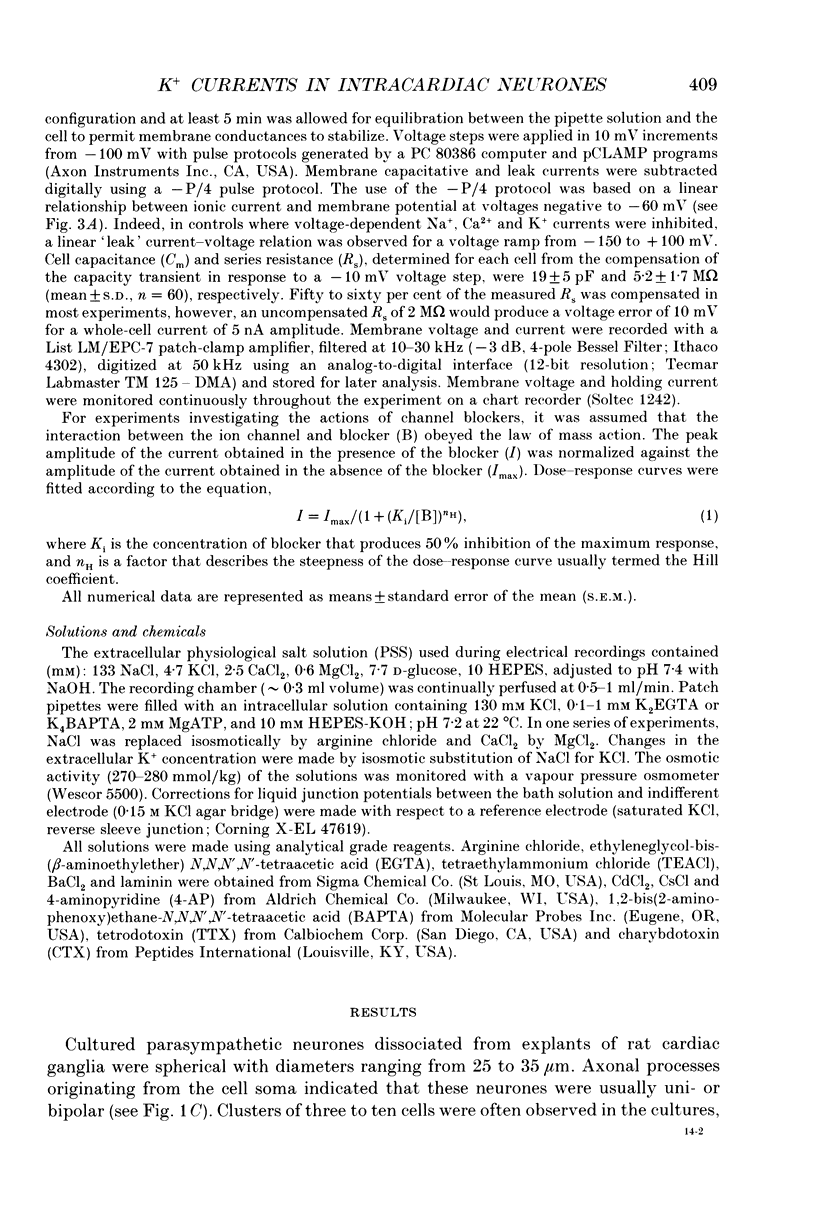
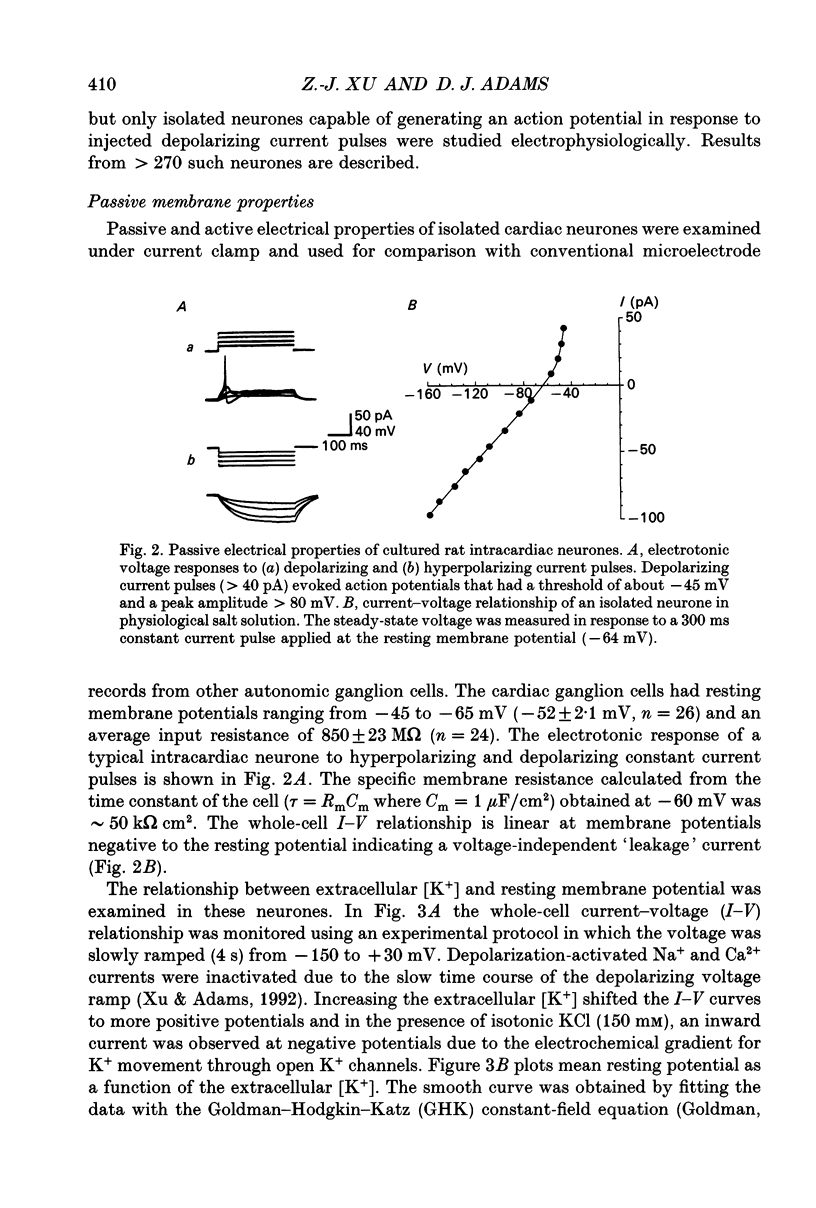
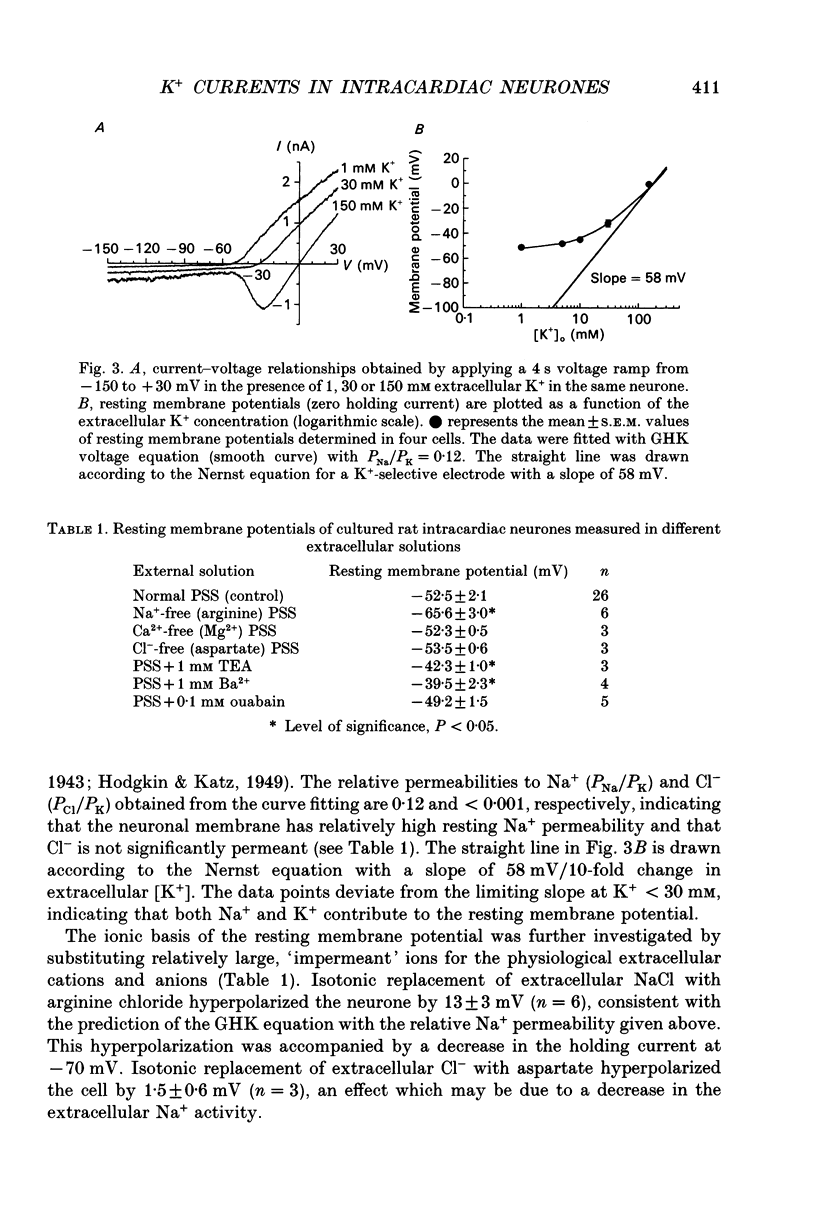
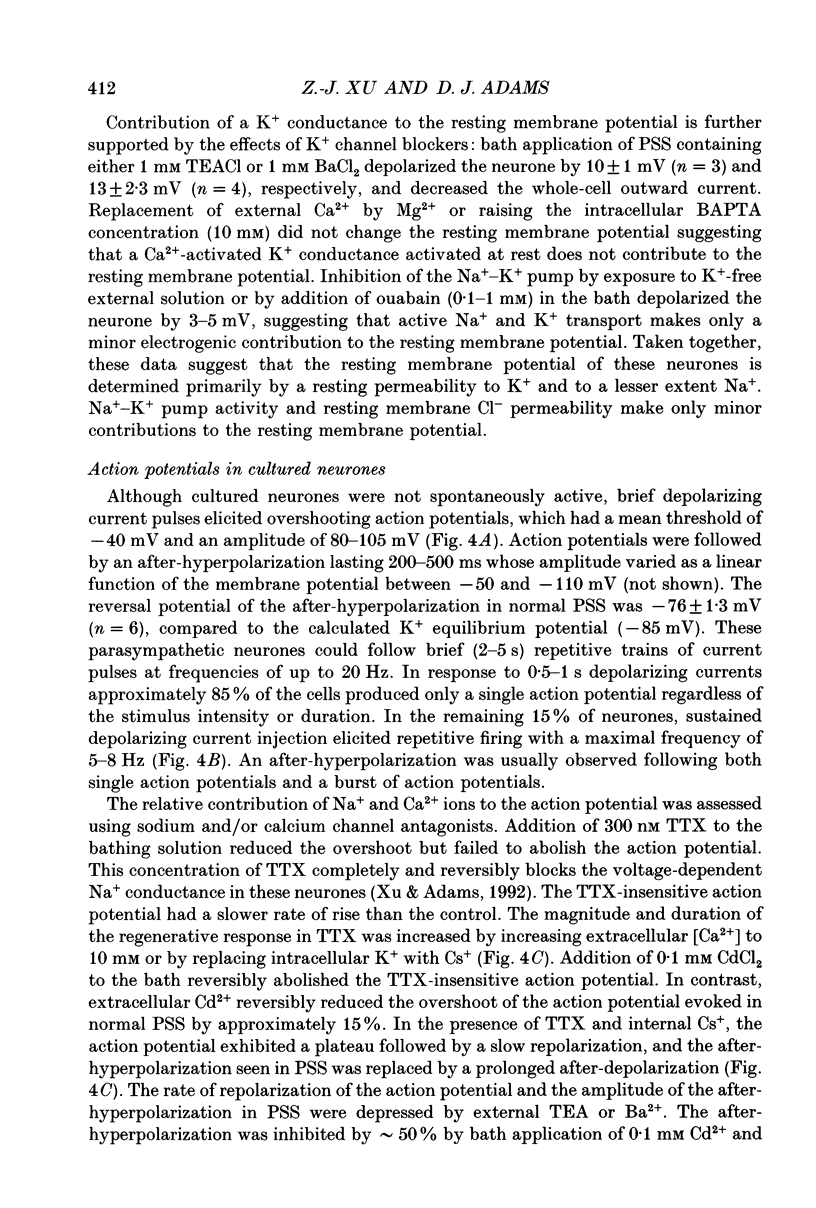
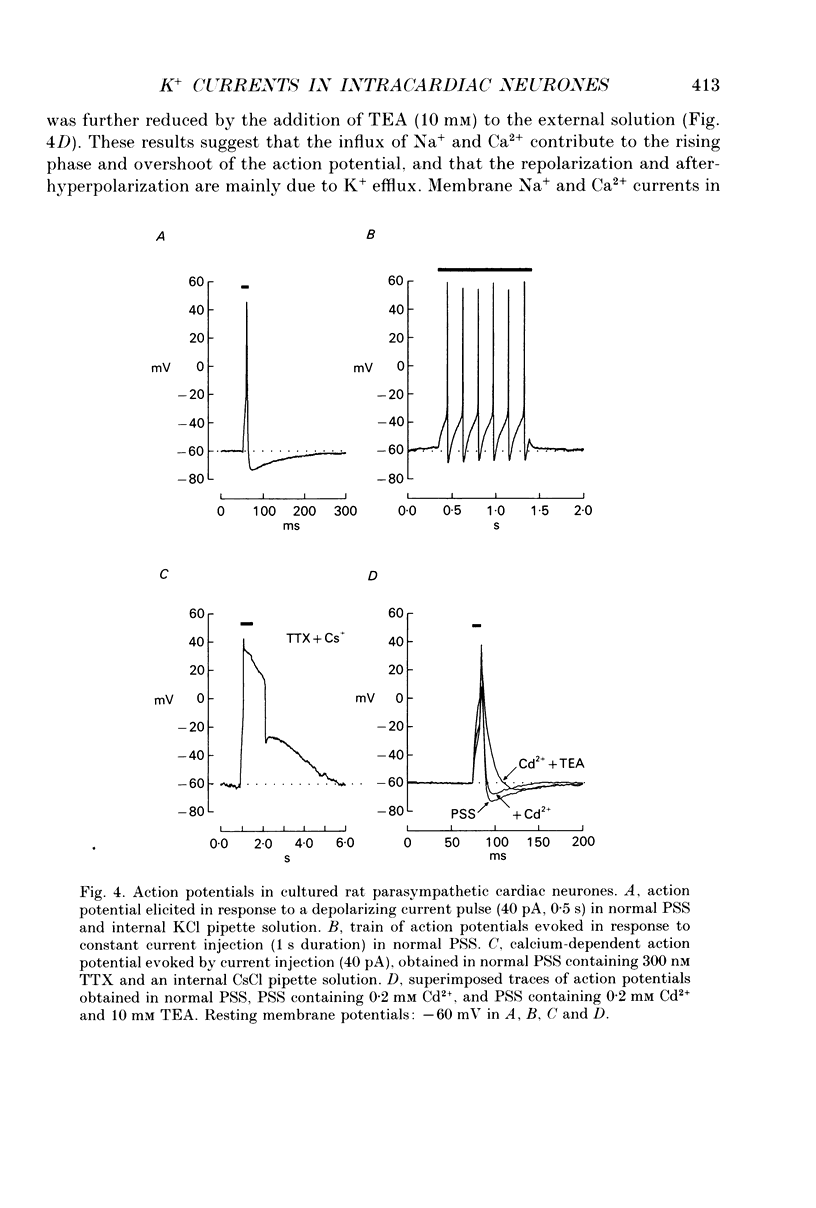
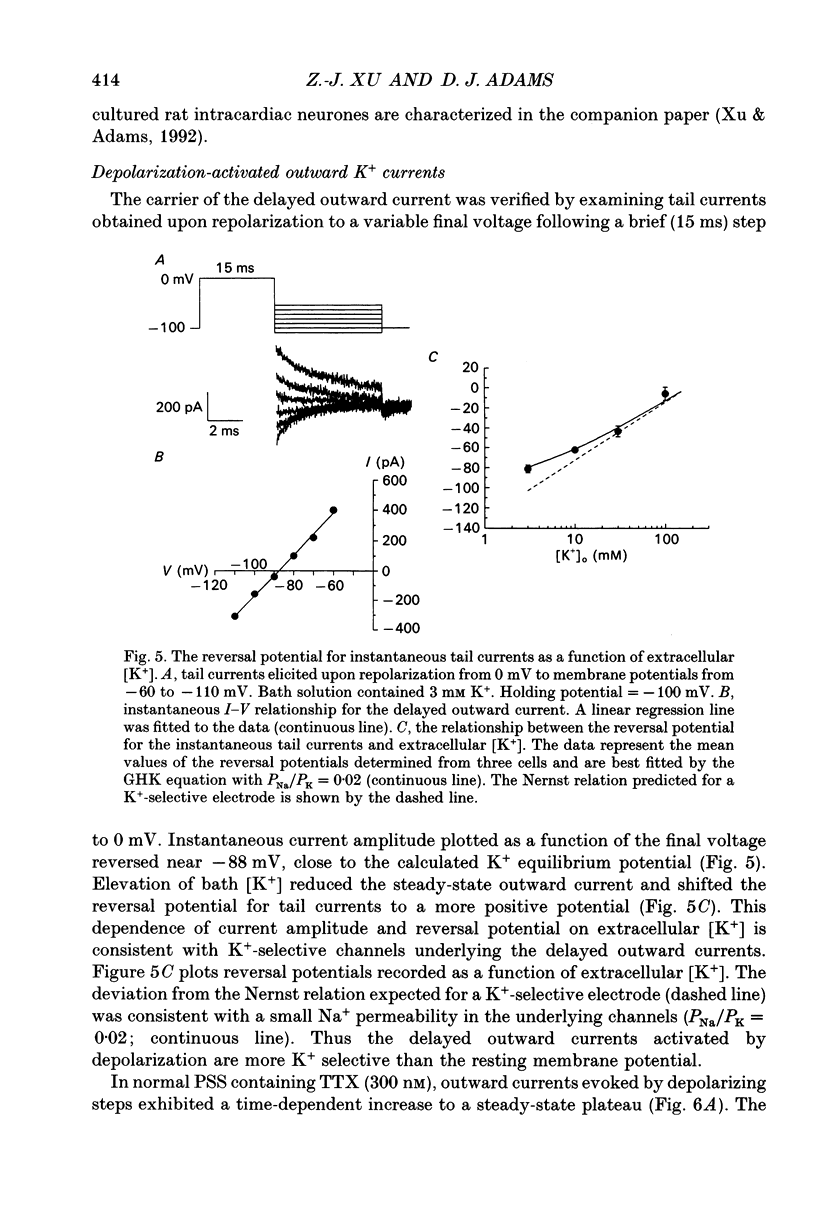
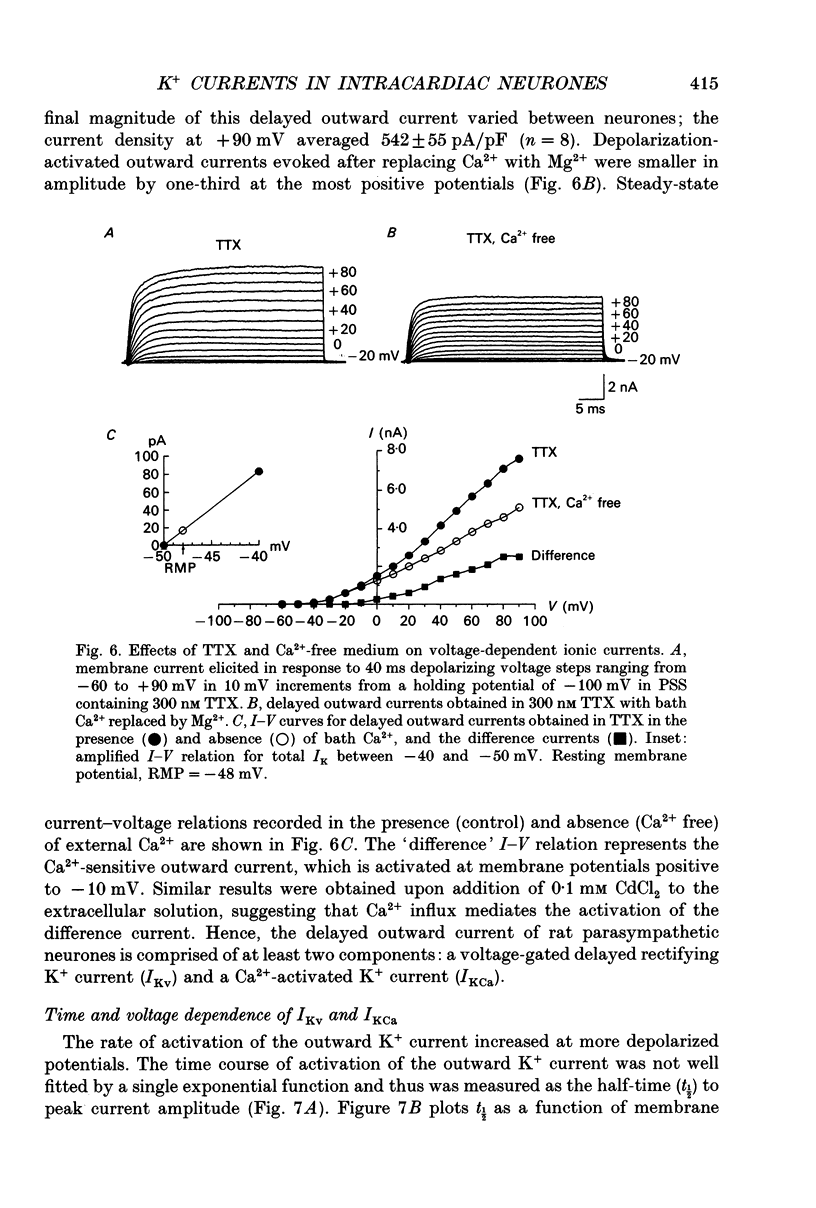
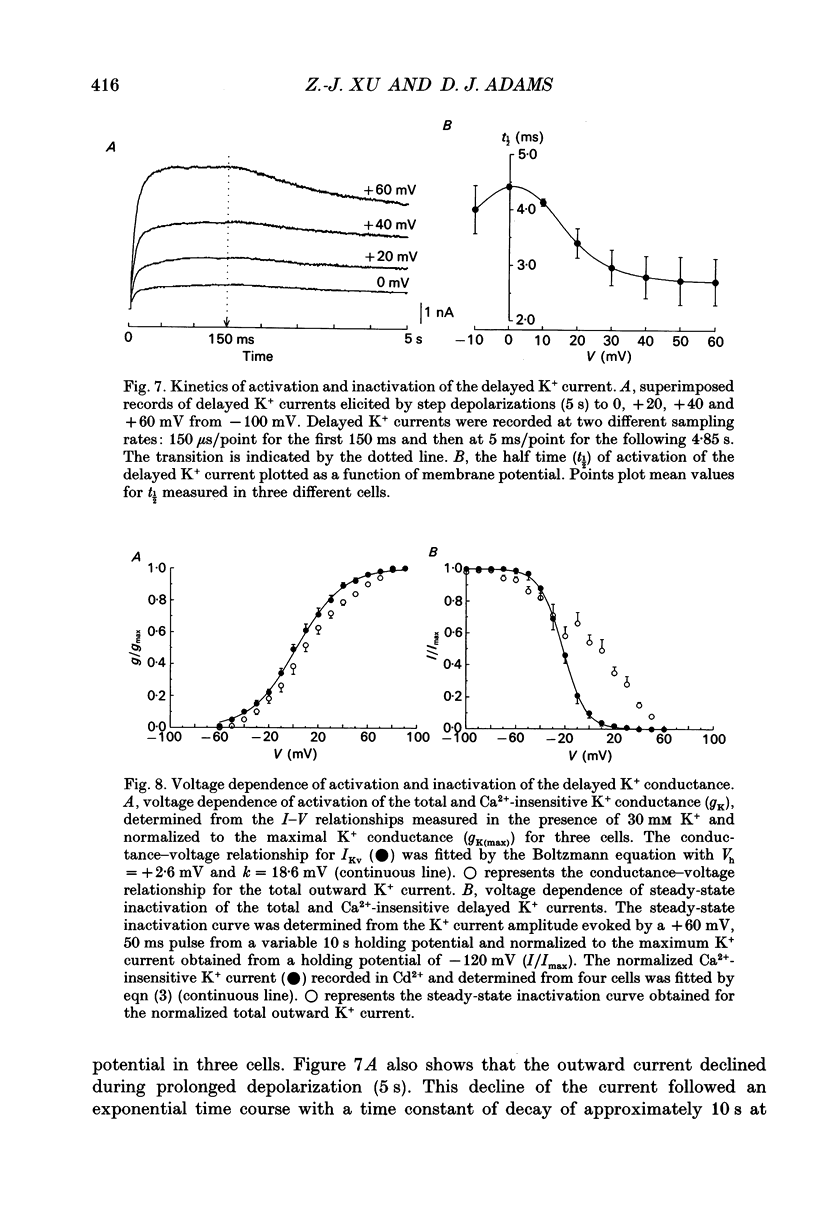
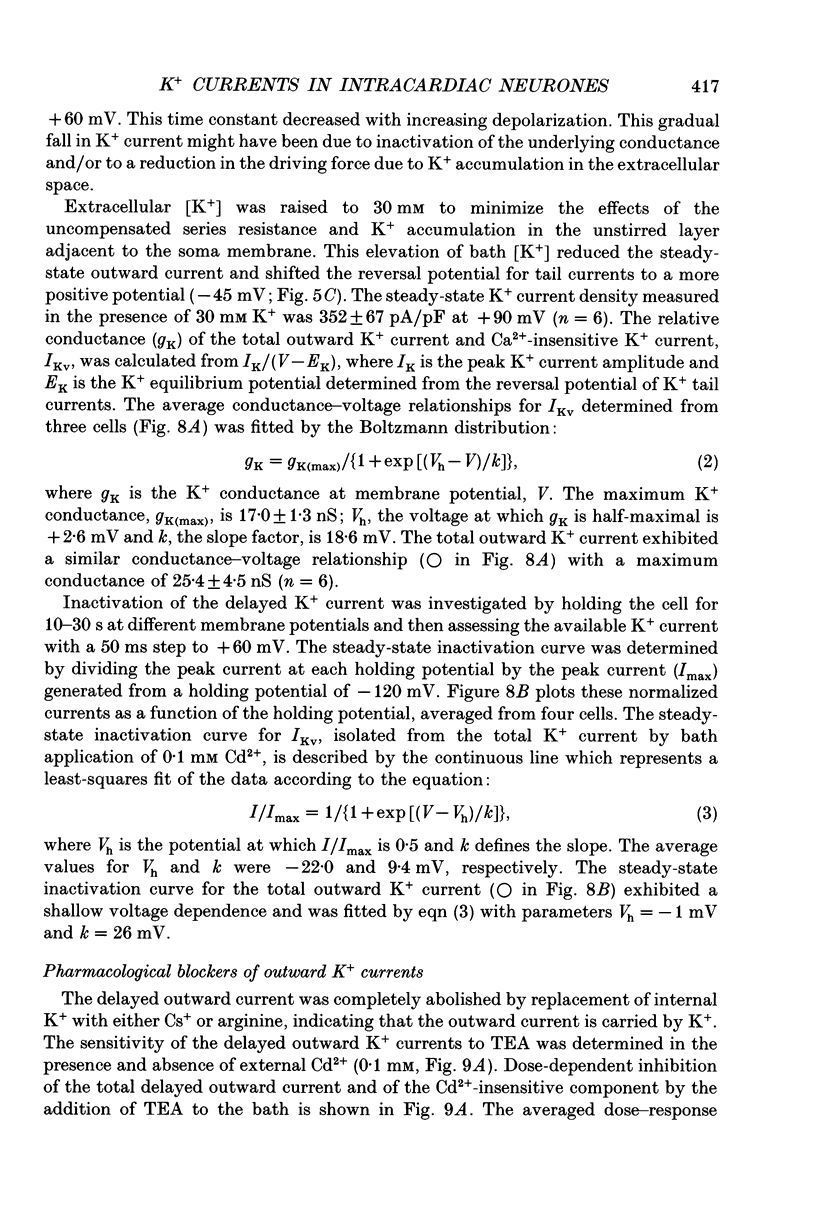
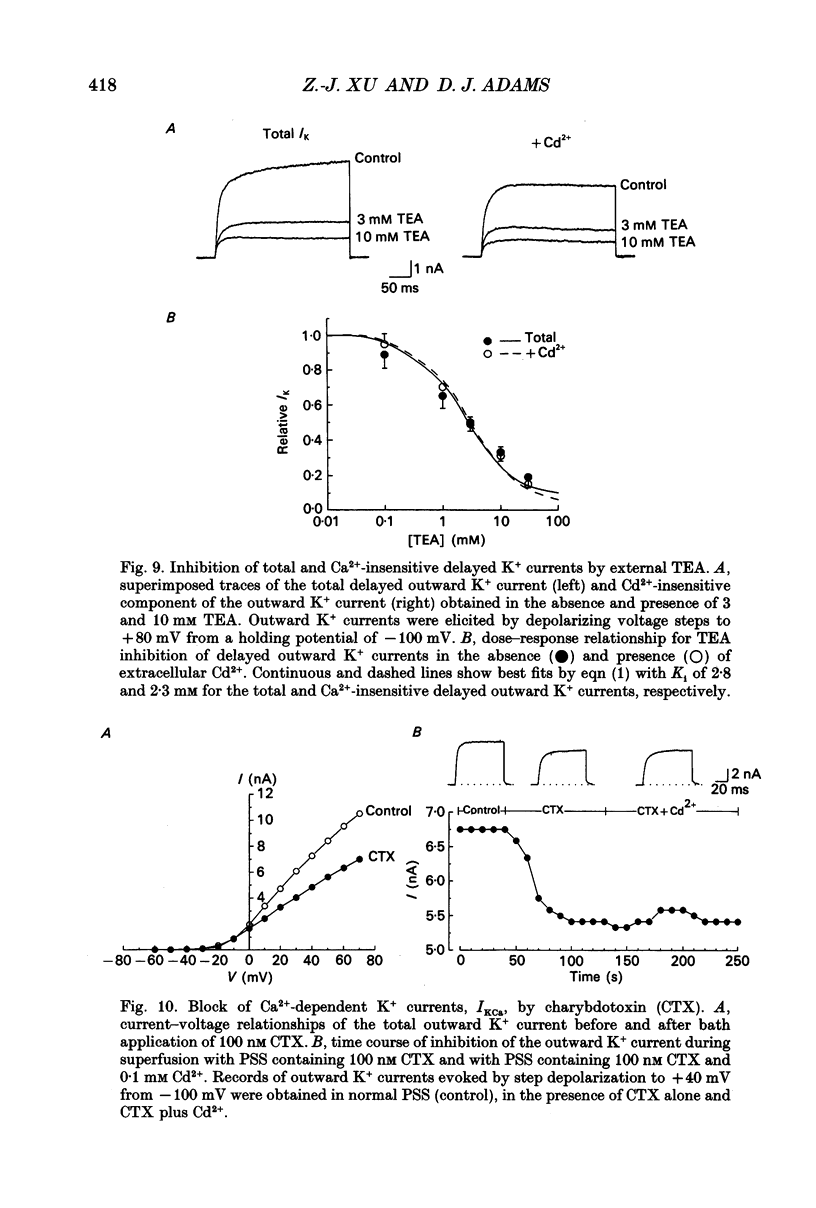
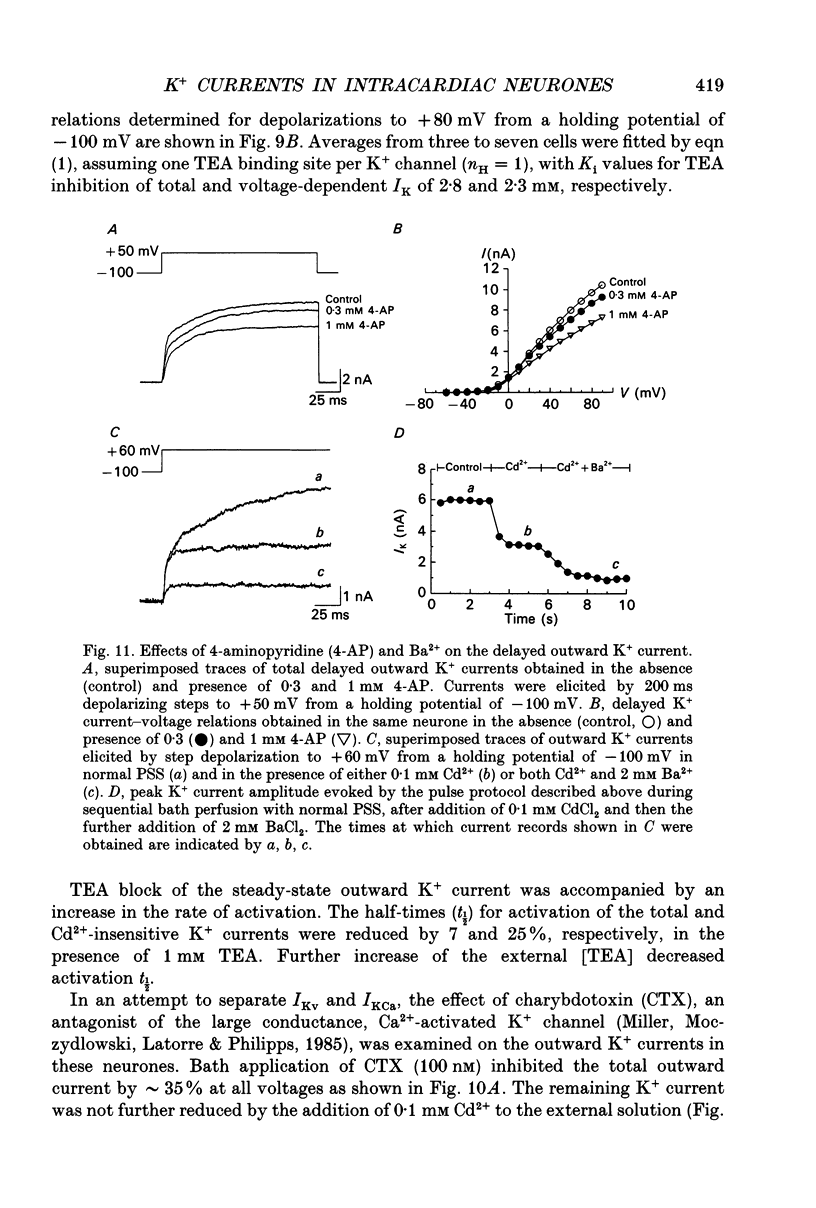
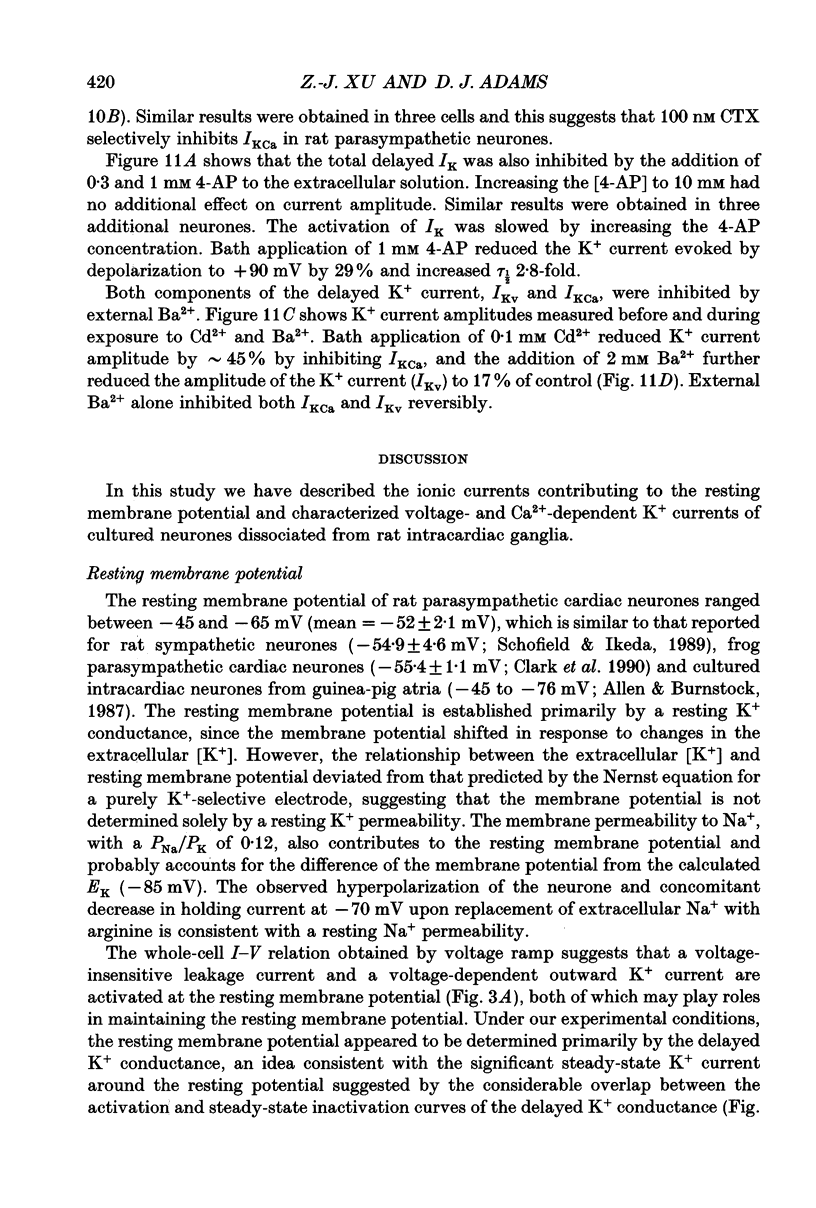
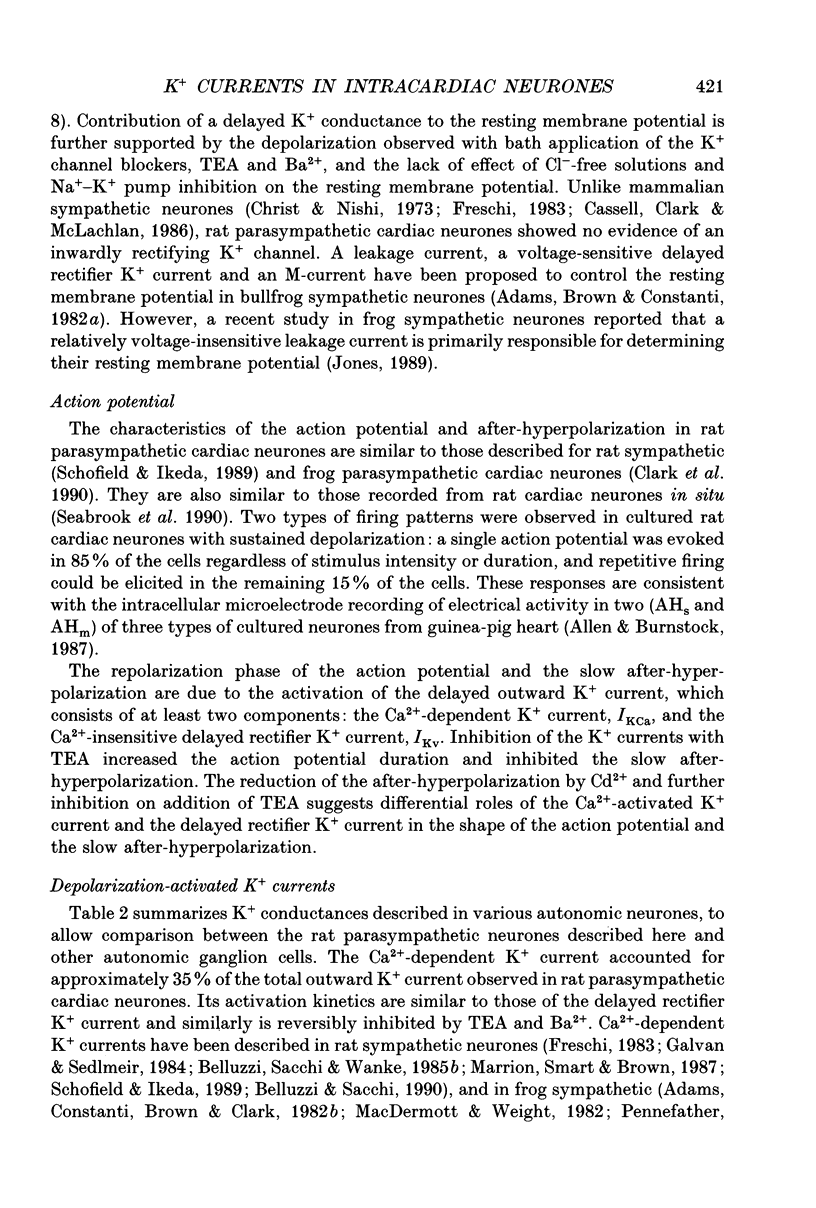
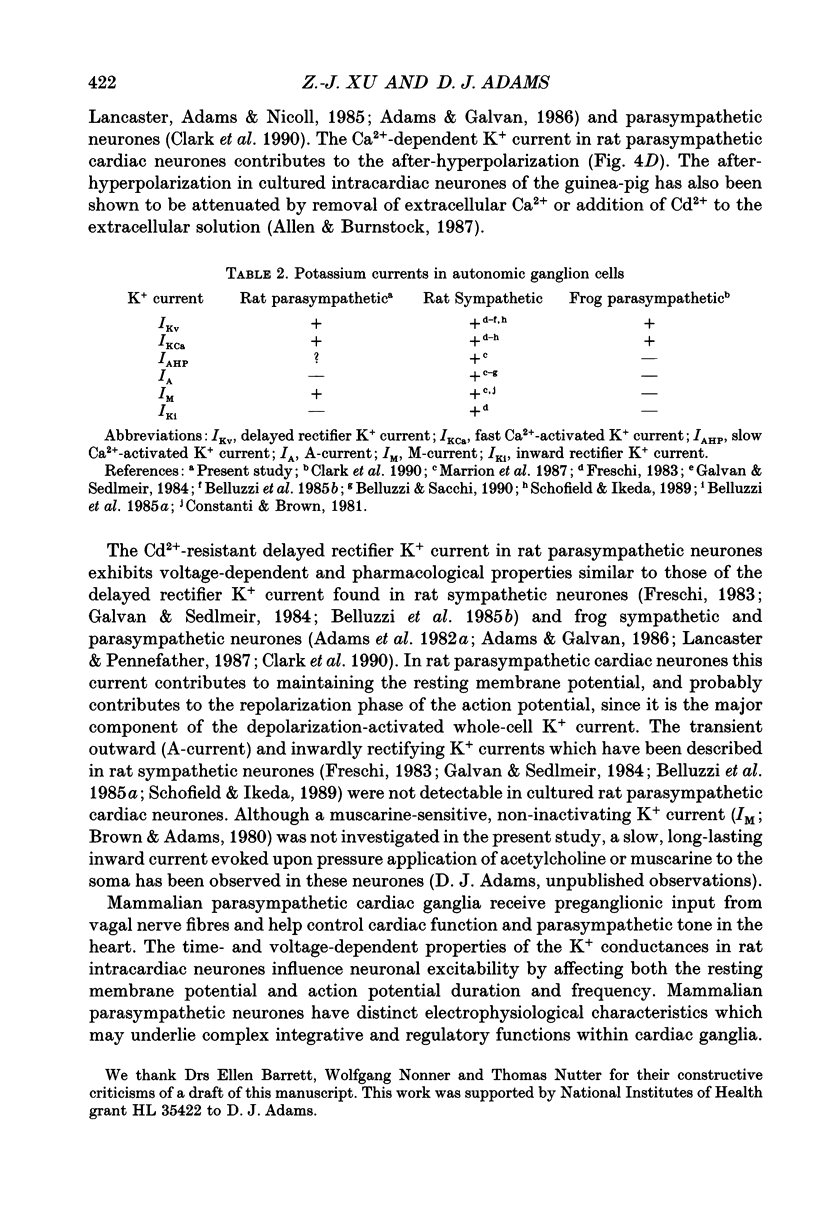
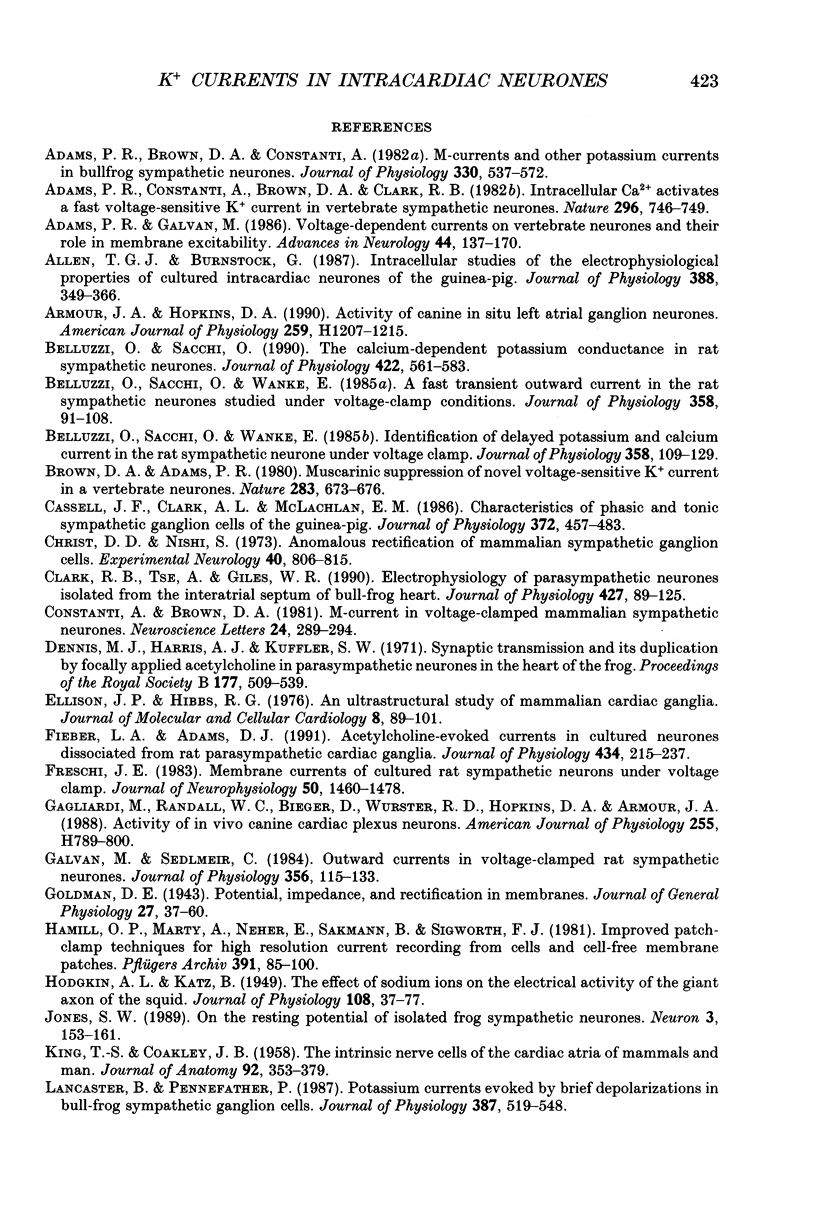
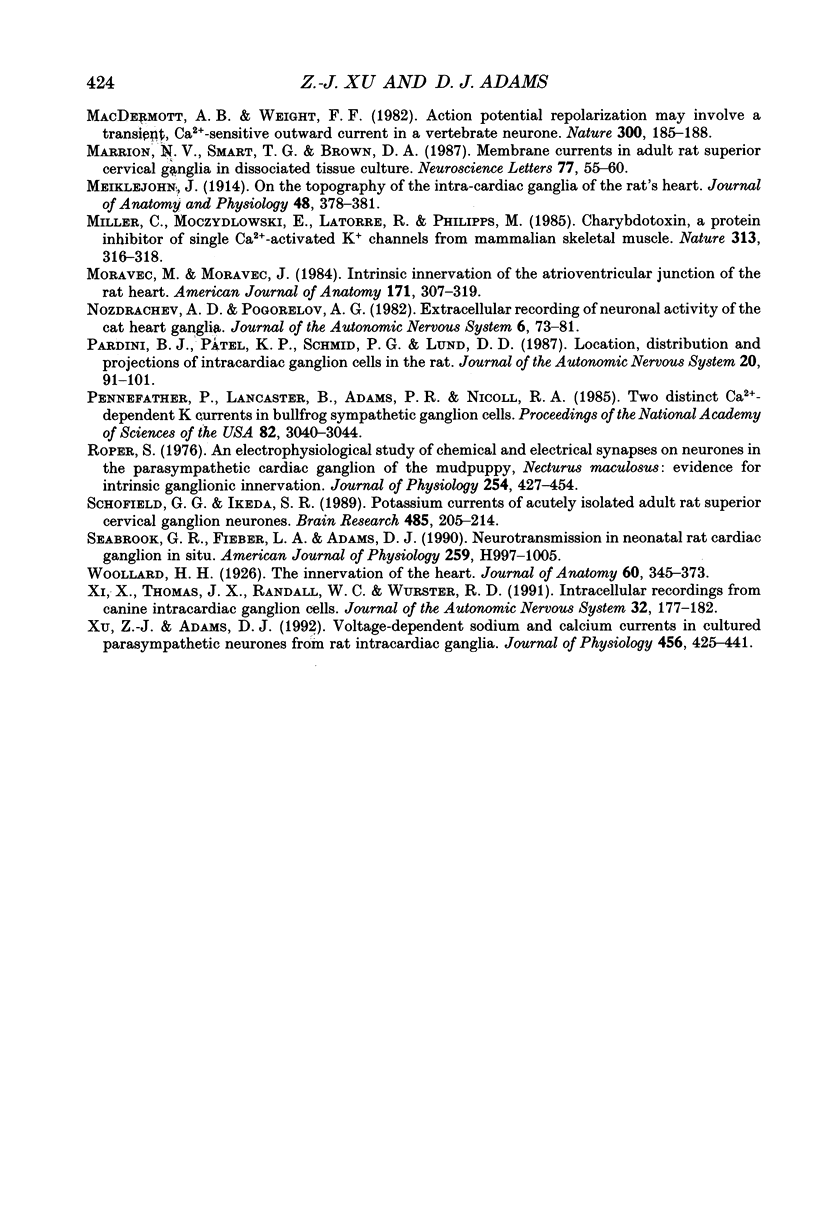
Images in this article
Selected References
These references are in PubMed. This may not be the complete list of references from this article.
- Adams P. R., Brown D. A., Constanti A. M-currents and other potassium currents in bullfrog sympathetic neurones. J Physiol. 1982 Sep;330:537–572. doi: 10.1113/jphysiol.1982.sp014357. [DOI] [PMC free article] [PubMed] [Google Scholar]
- Adams P. R., Constanti A., Brown D. A., Clark R. B. Intracellular Ca2+ activates a fast voltage-sensitive K+ current in vertebrate sympathetic neurones. Nature. 1982 Apr 22;296(5859):746–749. doi: 10.1038/296746a0. [DOI] [PubMed] [Google Scholar]
- Adams P. R., Galvan M. Voltage-dependent currents of vertebrate neurons and their role in membrane excitability. Adv Neurol. 1986;44:137–170. [PubMed] [Google Scholar]
- Allen T. G., Burnstock G. Intracellular studies of the electrophysiological properties of cultured intracardiac neurones of the guinea-pig. J Physiol. 1987 Jul;388:349–366. doi: 10.1113/jphysiol.1987.sp016618. [DOI] [PMC free article] [PubMed] [Google Scholar]
- Armour J. A., Hopkins D. A. Activity of canine in situ left atrial ganglion neurons. Am J Physiol. 1990 Oct;259(4 Pt 2):H1207–H1215. doi: 10.1152/ajpheart.1990.259.4.H1207. [DOI] [PubMed] [Google Scholar]
- Belluzzi O., Sacchi O. The calcium-dependent potassium conductance in rat sympathetic neurones. J Physiol. 1990 Mar;422:561–583. doi: 10.1113/jphysiol.1990.sp018001. [DOI] [PMC free article] [PubMed] [Google Scholar]
- Belluzzi O., Sacchi O., Wanke E. A fast transient outward current in the rat sympathetic neurone studied under voltage-clamp conditions. J Physiol. 1985 Jan;358:91–108. doi: 10.1113/jphysiol.1985.sp015542. [DOI] [PMC free article] [PubMed] [Google Scholar]
- Belluzzi O., Sacchi O., Wanke E. Identification of delayed potassium and calcium currents in the rat sympathetic neurone under voltage clamp. J Physiol. 1985 Jan;358:109–129. doi: 10.1113/jphysiol.1985.sp015543. [DOI] [PMC free article] [PubMed] [Google Scholar]
- Brown D. A., Adams P. R. Muscarinic suppression of a novel voltage-sensitive K+ current in a vertebrate neurone. Nature. 1980 Feb 14;283(5748):673–676. doi: 10.1038/283673a0. [DOI] [PubMed] [Google Scholar]
- Cassell J. F., Clark A. L., McLachlan E. M. Characteristics of phasic and tonic sympathetic ganglion cells of the guinea-pig. J Physiol. 1986 Mar;372:457–483. doi: 10.1113/jphysiol.1986.sp016020. [DOI] [PMC free article] [PubMed] [Google Scholar]
- Christ D. D., Nishi S. Anomalous rectification of mammalian sympathetic ganglion cells. Exp Neurol. 1973 Sep;40(3):806–815. doi: 10.1016/0014-4886(73)90114-3. [DOI] [PubMed] [Google Scholar]
- Clark R. B., Tse A., Giles W. R. Electrophysiology of parasympathetic neurones isolated from the interatrial septum of bull-frog heart. J Physiol. 1990 Aug;427:89–125. doi: 10.1113/jphysiol.1990.sp018163. [DOI] [PMC free article] [PubMed] [Google Scholar]
- Constanti A., Brown D. A. M-Currents in voltage-clamped mammalian sympathetic neurones. Neurosci Lett. 1981 Jul 17;24(3):289–294. doi: 10.1016/0304-3940(81)90173-7. [DOI] [PubMed] [Google Scholar]
- Dennis M. J., Harris A. J., Kuffler S. W. Synaptic transmission and its duplication by focally applied acetylcholine in parasympathetic neurons in the heart of the frog. Proc R Soc Lond B Biol Sci. 1971 Apr 27;177(1049):509–539. doi: 10.1098/rspb.1971.0045. [DOI] [PubMed] [Google Scholar]
- Ellison J. P., Hibbs R. G. An ultrastructural study of mammalian cardiac ganglia. J Mol Cell Cardiol. 1976 Feb;8(2):89–101. doi: 10.1016/0022-2828(76)90023-7. [DOI] [PubMed] [Google Scholar]
- Fieber L. A., Adams D. J. Acetylcholine-evoked currents in cultured neurones dissociated from rat parasympathetic cardiac ganglia. J Physiol. 1991 Mar;434:215–237. doi: 10.1113/jphysiol.1991.sp018466. [DOI] [PMC free article] [PubMed] [Google Scholar]
- Freschi J. E. Membrane currents of cultured rat sympathetic neurons under voltage clamp. J Neurophysiol. 1983 Dec;50(6):1460–1478. doi: 10.1152/jn.1983.50.6.1460. [DOI] [PubMed] [Google Scholar]
- Gagliardi M., Randall W. C., Bieger D., Wurster R. D., Hopkins D. A., Armour J. A. Activity of in vivo canine cardiac plexus neurons. Am J Physiol. 1988 Oct;255(4 Pt 2):H789–H800. doi: 10.1152/ajpheart.1988.255.4.H789. [DOI] [PubMed] [Google Scholar]
- Galvan M., Sedlmeir C. Outward currents in voltage-clamped rat sympathetic neurones. J Physiol. 1984 Nov;356:115–133. doi: 10.1113/jphysiol.1984.sp015456. [DOI] [PMC free article] [PubMed] [Google Scholar]
- HODGKIN A. L., KATZ B. The effect of sodium ions on the electrical activity of giant axon of the squid. J Physiol. 1949 Mar 1;108(1):37–77. doi: 10.1113/jphysiol.1949.sp004310. [DOI] [PMC free article] [PubMed] [Google Scholar]
- Hamill O. P., Marty A., Neher E., Sakmann B., Sigworth F. J. Improved patch-clamp techniques for high-resolution current recording from cells and cell-free membrane patches. Pflugers Arch. 1981 Aug;391(2):85–100. doi: 10.1007/BF00656997. [DOI] [PubMed] [Google Scholar]
- Jones S. W. On the resting potential of isolated frog sympathetic neurons. Neuron. 1989 Aug;3(2):153–161. doi: 10.1016/0896-6273(89)90028-7. [DOI] [PubMed] [Google Scholar]
- KING T. S., COAKLEY J. B. The intrinsic nerve cells of the cardiac atria of mammals and man. J Anat. 1958 Jul;92(3):353–376. [PMC free article] [PubMed] [Google Scholar]
- Lancaster B., Pennefather P. Potassium currents evoked by brief depolarizations in bull-frog sympathetic ganglion cells. J Physiol. 1987 Jun;387:519–548. doi: 10.1113/jphysiol.1987.sp016587. [DOI] [PMC free article] [PubMed] [Google Scholar]
- MacDermott A. B., Weight F. F. Action potential repolarization may involve a transient, Ca2+-sensitive outward current in a vertebrate neurone. Nature. 1982 Nov 11;300(5888):185–188. doi: 10.1038/300185a0. [DOI] [PubMed] [Google Scholar]
- Marrion N. V., Smart T. G., Brown D. A. Membrane currents in adult rat superior cervical ganglia in dissociated tissue culture. Neurosci Lett. 1987 Jun 1;77(1):55–60. doi: 10.1016/0304-3940(87)90606-9. [DOI] [PubMed] [Google Scholar]
- Meiklejohn J. On the Topography of the Intracardiac Ganglia of the Rat's Heart. J Anat Physiol. 1914 Jul;48(Pt 4):378–390. [PMC free article] [PubMed] [Google Scholar]
- Miller C., Moczydlowski E., Latorre R., Phillips M. Charybdotoxin, a protein inhibitor of single Ca2+-activated K+ channels from mammalian skeletal muscle. Nature. 1985 Jan 24;313(6000):316–318. doi: 10.1038/313316a0. [DOI] [PubMed] [Google Scholar]
- Moravec M., Moravec J. Intrinsic innervation of the atrioventricular junction of the rat heart. Am J Anat. 1984 Nov;171(3):307–319. doi: 10.1002/aja.1001710307. [DOI] [PubMed] [Google Scholar]
- Nozdrachev A. D., Pogorelov A. G. Extracellular recording of neuronal activity of the cat heart ganglia. J Auton Nerv Syst. 1982 Jul;6(1):73–81. doi: 10.1016/0165-1838(82)90024-8. [DOI] [PubMed] [Google Scholar]
- Pardini B. J., Patel K. P., Schmid P. G., Lund D. D. Location, distribution and projections of intracardiac ganglion cells in the rat. J Auton Nerv Syst. 1987 Aug;20(2):91–101. doi: 10.1016/0165-1838(87)90106-8. [DOI] [PubMed] [Google Scholar]
- Pennefather P., Lancaster B., Adams P. R., Nicoll R. A. Two distinct Ca-dependent K currents in bullfrog sympathetic ganglion cells. Proc Natl Acad Sci U S A. 1985 May;82(9):3040–3044. doi: 10.1073/pnas.82.9.3040. [DOI] [PMC free article] [PubMed] [Google Scholar]
- Roper S. An electrophysiological study of chemical and electrical synapses on neurones in the parasympathetic cardiac ganglion of the mudpuppy, Necturus maculosus: evidence for intrinsic ganglionic innervation. J Physiol. 1976 Jan;254(2):427–454. doi: 10.1113/jphysiol.1976.sp011239. [DOI] [PMC free article] [PubMed] [Google Scholar]
- Schofield G. G., Ikeda S. R. Potassium currents of acutely isolated adult rat superior cervical ganglion neurons. Brain Res. 1989 Apr 24;485(2):205–214. doi: 10.1016/0006-8993(89)90563-5. [DOI] [PubMed] [Google Scholar]
- Seabrook G. R., Fieber L. A., Adams D. J. Neurotransmission in neonatal rat cardiac ganglion in situ. Am J Physiol. 1990 Oct;259(4 Pt 2):H997–1005. doi: 10.1152/ajpheart.1990.259.4.H997. [DOI] [PubMed] [Google Scholar]
- Woollard H. H. The Innervation of the Heart. J Anat. 1926 Jul;60(Pt 4):345–373. [PMC free article] [PubMed] [Google Scholar]
- Xi X. H., Thomas J. X., Jr, Randall W. C., Wurster R. D. Intracellular recordings from canine intracardiac ganglion cells. J Auton Nerv Syst. 1991 Feb;32(2):177–182. doi: 10.1016/0165-1838(91)90068-e. [DOI] [PubMed] [Google Scholar]
- Xu Z. J., Adams D. J. Voltage-dependent sodium and calcium currents in cultured parasympathetic neurones from rat intracardiac ganglia. J Physiol. 1992 Oct;456:425–441. doi: 10.1113/jphysiol.1992.sp019344. [DOI] [PMC free article] [PubMed] [Google Scholar]