Abstract
1. Dissociated neurones from embryonic rat hypothalamus were grown for several weeks in culture where they formed complex networks. These synaptically coupled networks were capable of generating synchronized bursting activity. Voltage-activated membrane currents were studied in these neurones using a patch clamp in the whole-cell configuration. 2. Outward currents were carried by K+ ions and consisted of an inactivating and a non-inactivating component. These components were similar to the transient K+ current (IA) and the delayed rectifier current (IK) reported in neurones from the postnatal rat hypothalamus. Application of Zn2+ (1 mM) blocked the transient component completely while reducing the non-inactivating component by only approximately 20%. 3. Inward currents were carried by Na+ and Ca2+ ions. Rapidly activating transient Na+ currents were activated at approximately -25 mV. TTX entirely blocked these currents at low concentration (300 nM). Voltage sensitivity of the Na+ conductance was 5.8 mV per e-fold change with half-maximal activation occurring at -8 mV. Na+ current kinetics could be well described by the Hodgkin-Huxley model (m3h). 4. With depolarizing pulses from a holding potential of -80 mV two Ca2+ current components with different ranges of activation were identified. Low voltage-activated (LVA, T-type) Ca2+ currents were activated at approximately -50 mV. High voltage-activated (HVA; also called L- or N-type) Ca2+ currents were observed at membrane potentials more positive to approximately -30 mV. LVA Ca2+ currents were observed in hypothalamic neurones that had developed a network of dendritic processes in the course of several weeks in culture. Activation and inactivation time constants of LVA Ca2+ currents were 15-25 ms and 30-100 ms (-30 to -45 mV). In contrast to HVA Ca2+ currents, no LVA Ca2+ currents were seen in neuronal somata obtained from the network cultures by mechanical dissociation. This suggests that most of the LVA Ca2+ channels are located on the dendritic tree rather than on the soma membrane. 5. HVA Ca2+ currents were maximal between 0 and +10 mV (external [Ca2+] = 5 mM). The time-to-peak was in the range of 1.7-5.4 ms (+30 to -10 mV). Tail currents following repolarization decayed monoexponentially with a time constant of approximately 210 microseconds. During 500 ms depolarizations, 90% of the current inactivated. The time course of inactivation showed two time constants of approximately 40 and approximately 700 ms.(ABSTRACT TRUNCATED AT 400 WORDS)
Full text
PDF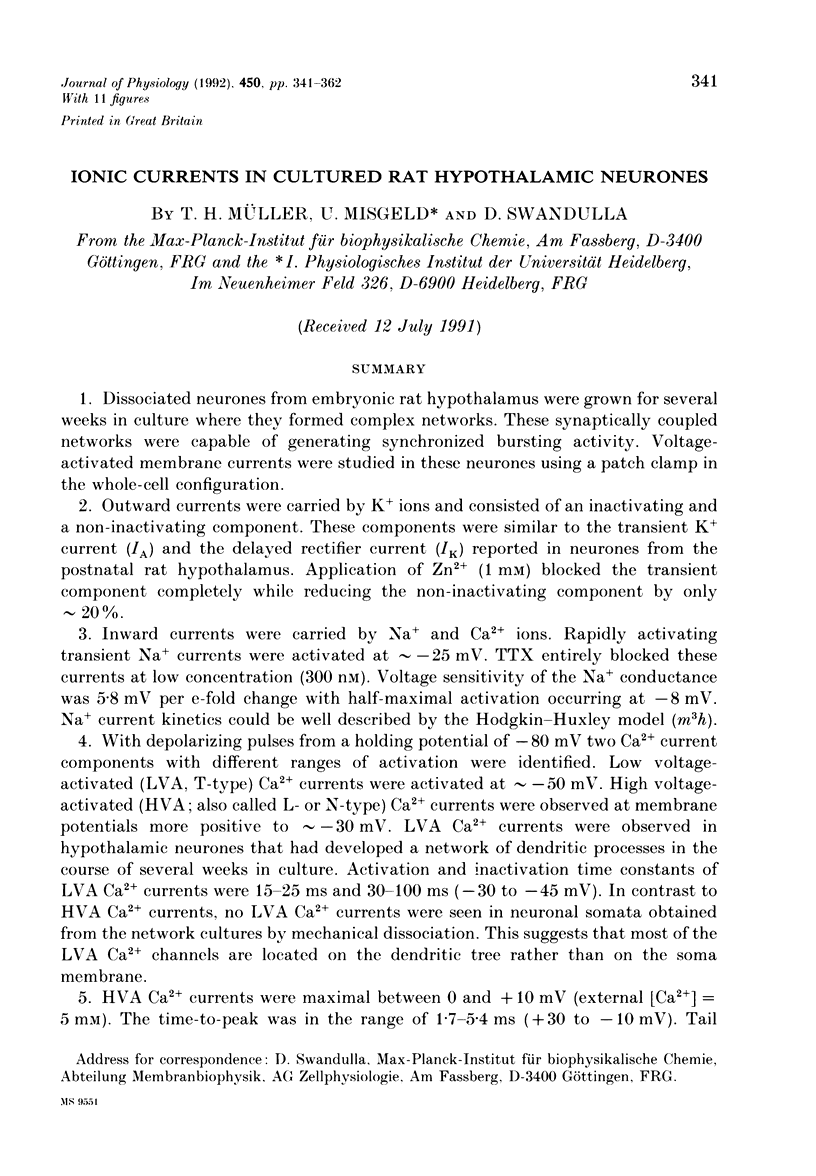
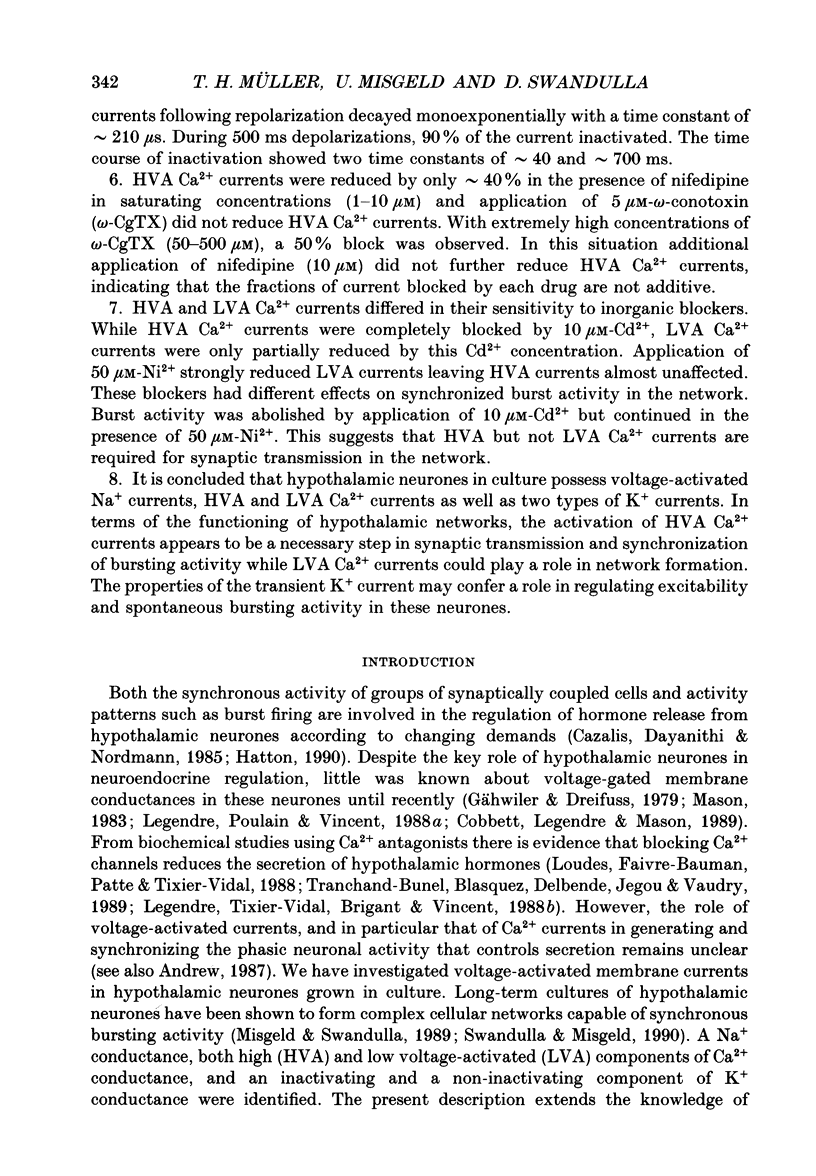
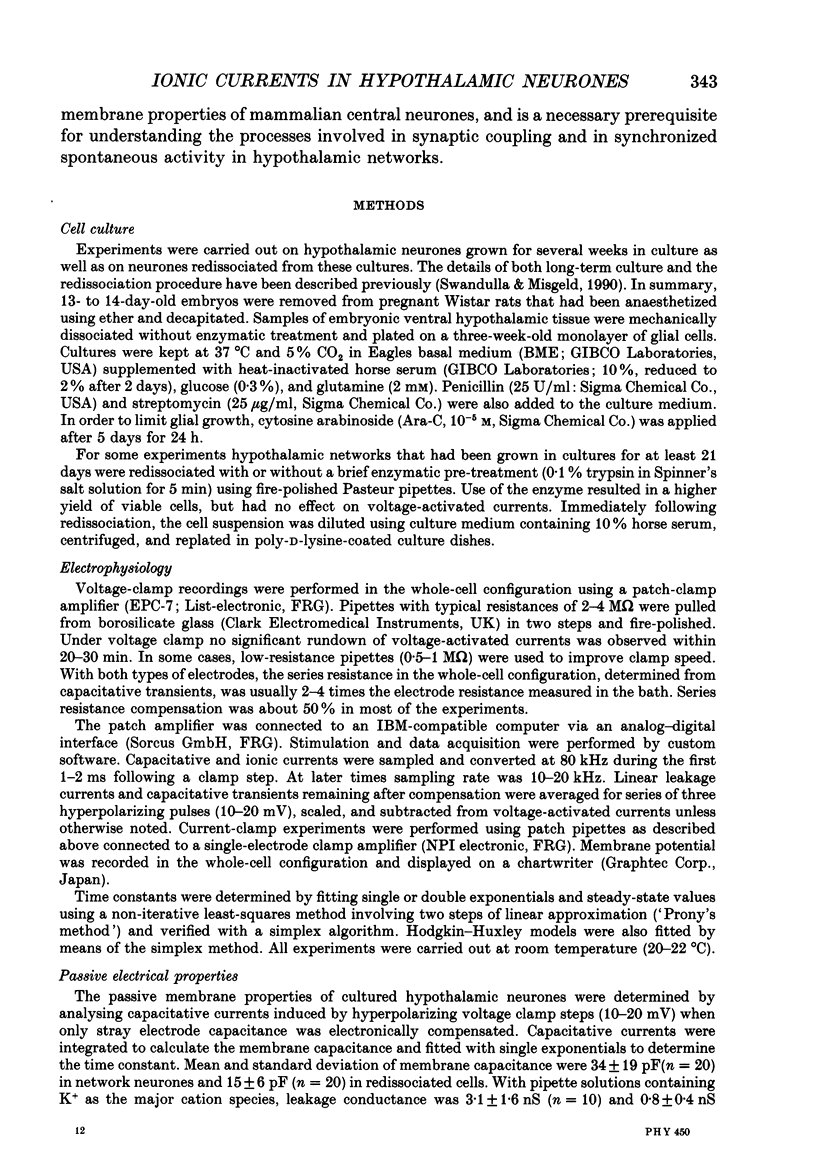
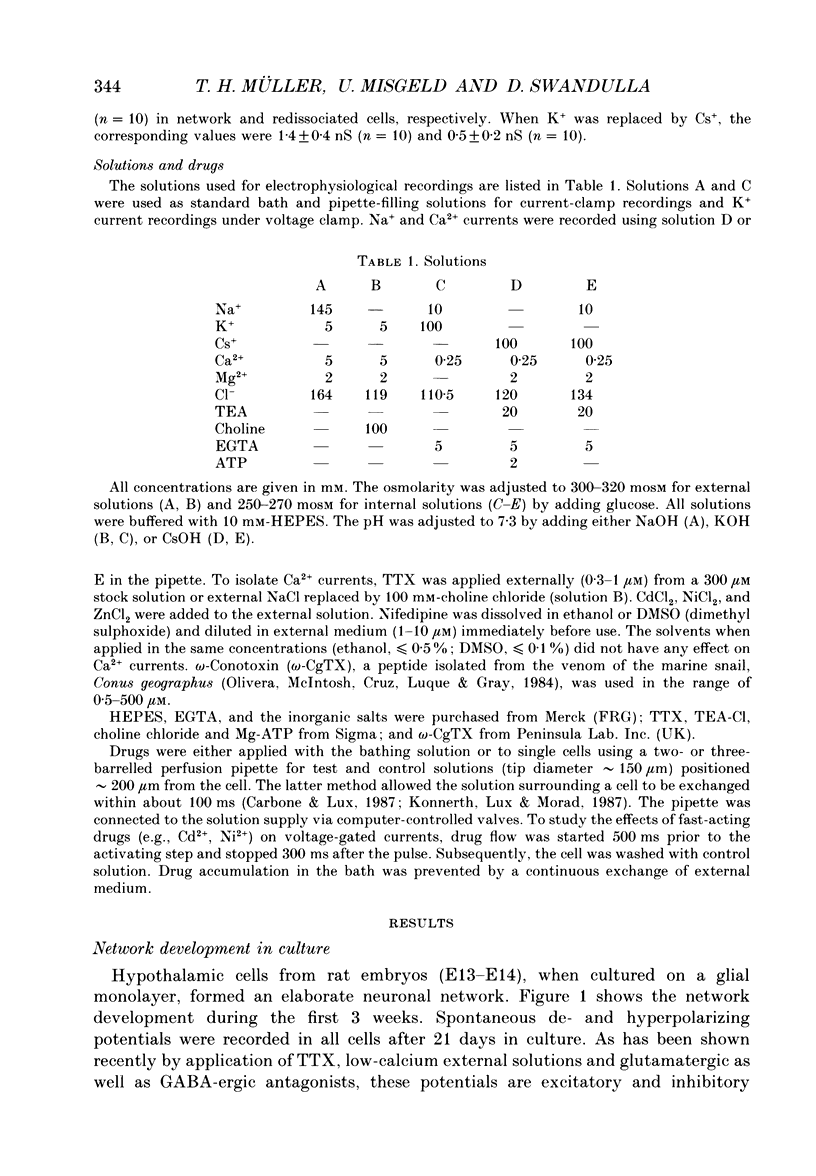
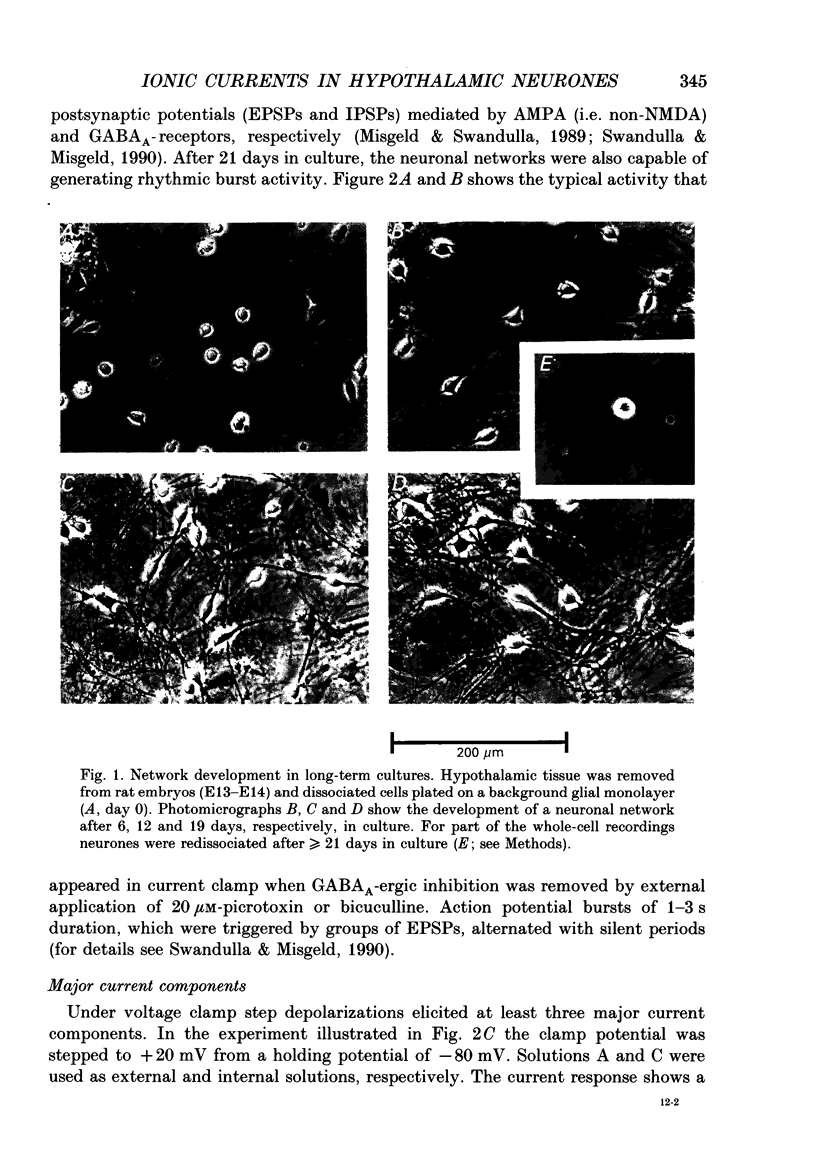
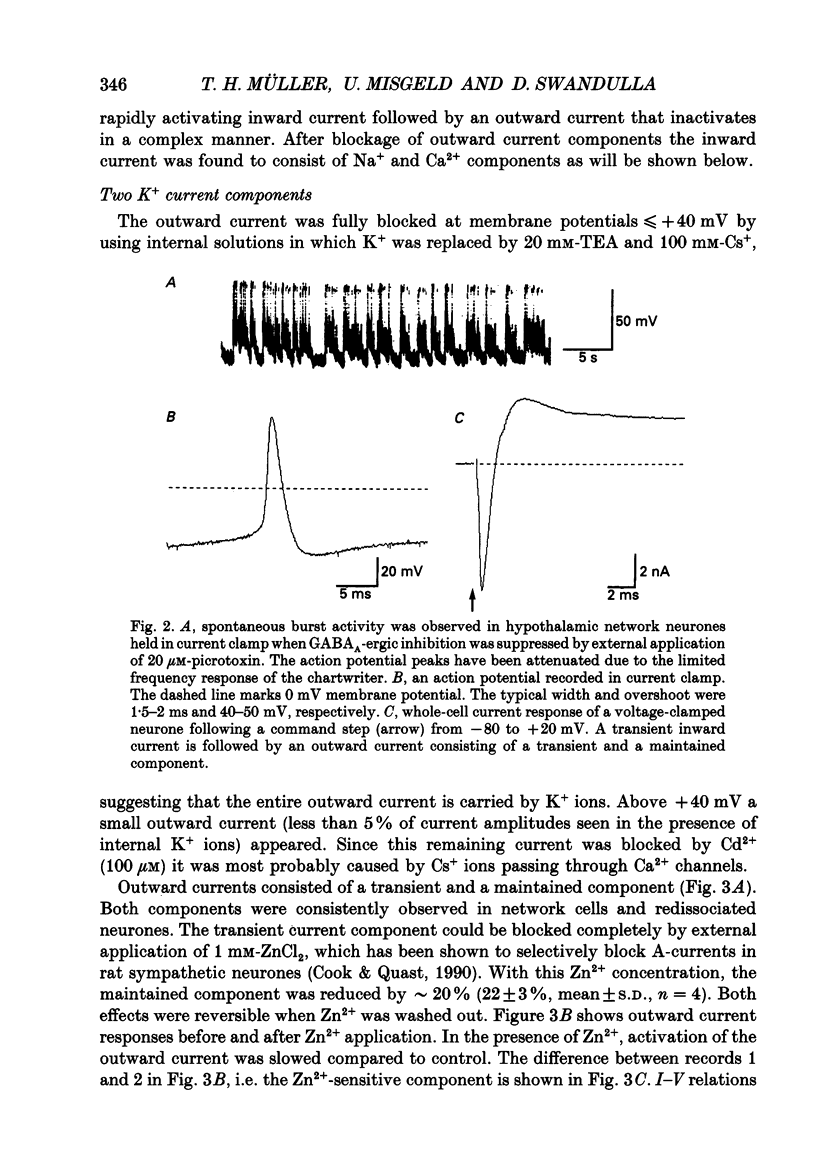
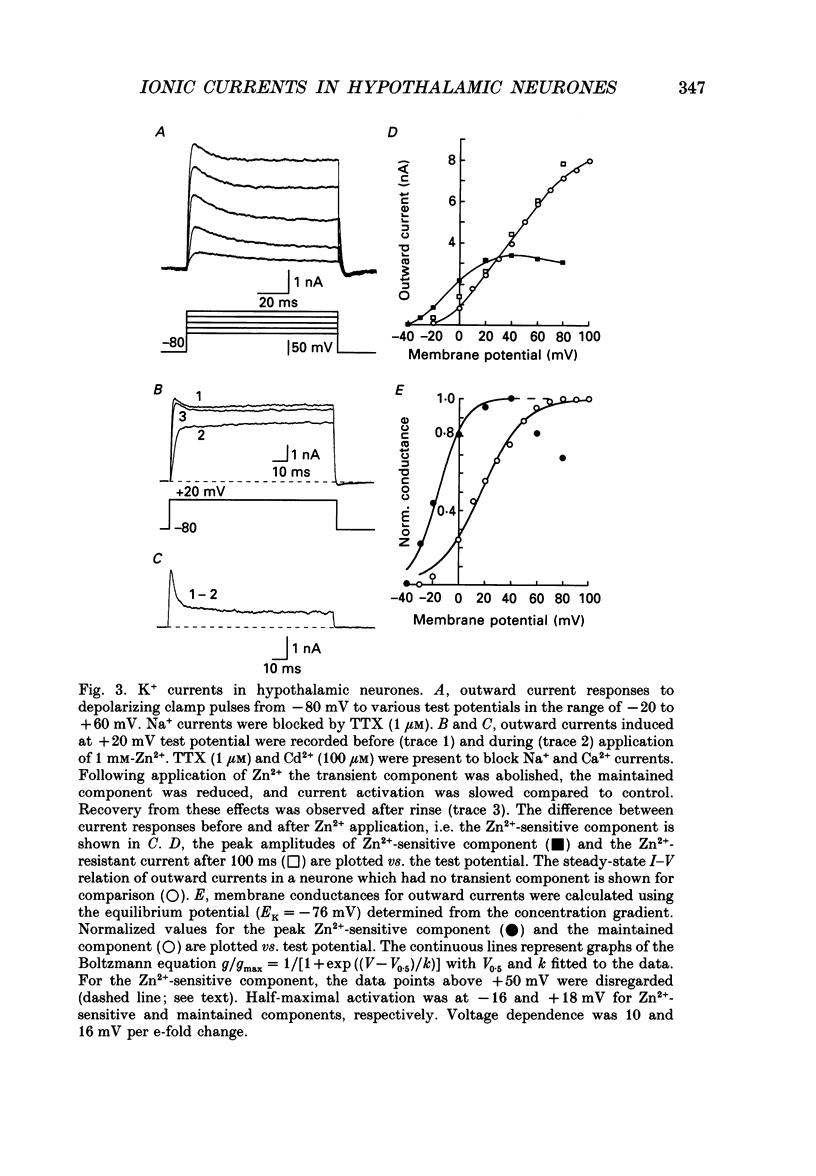
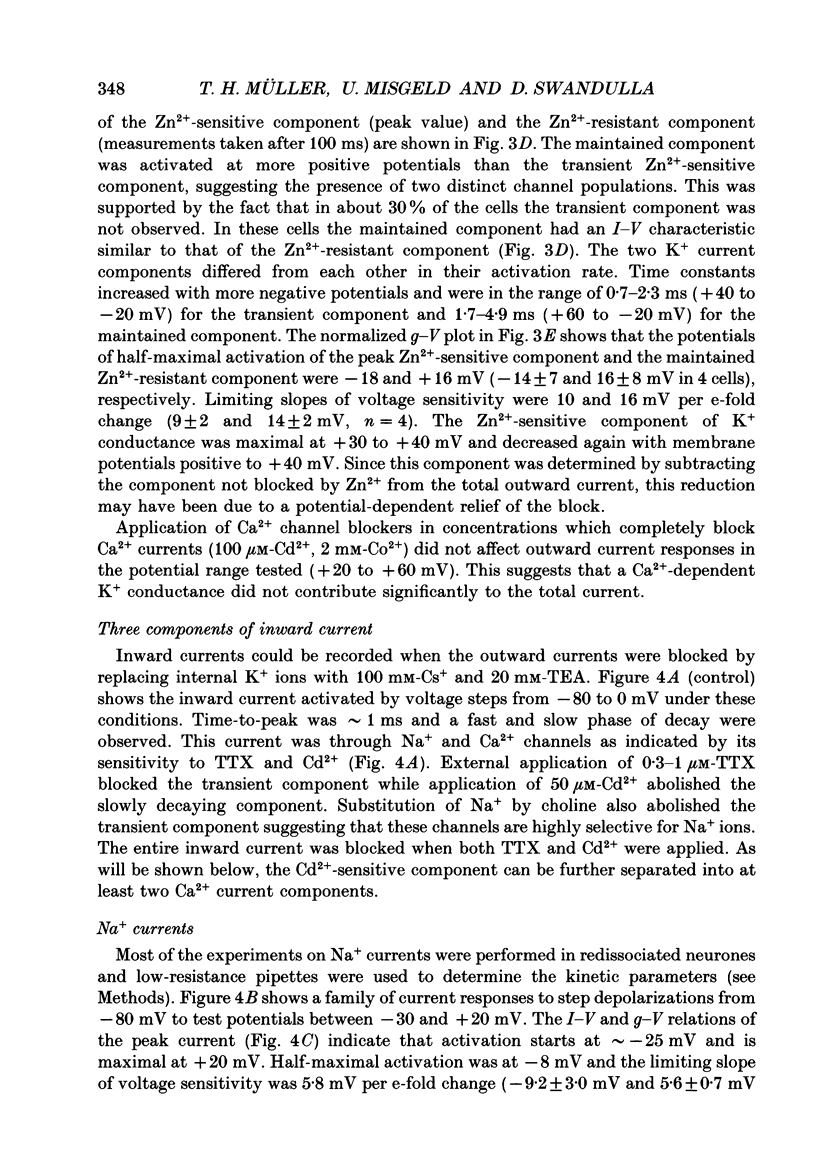
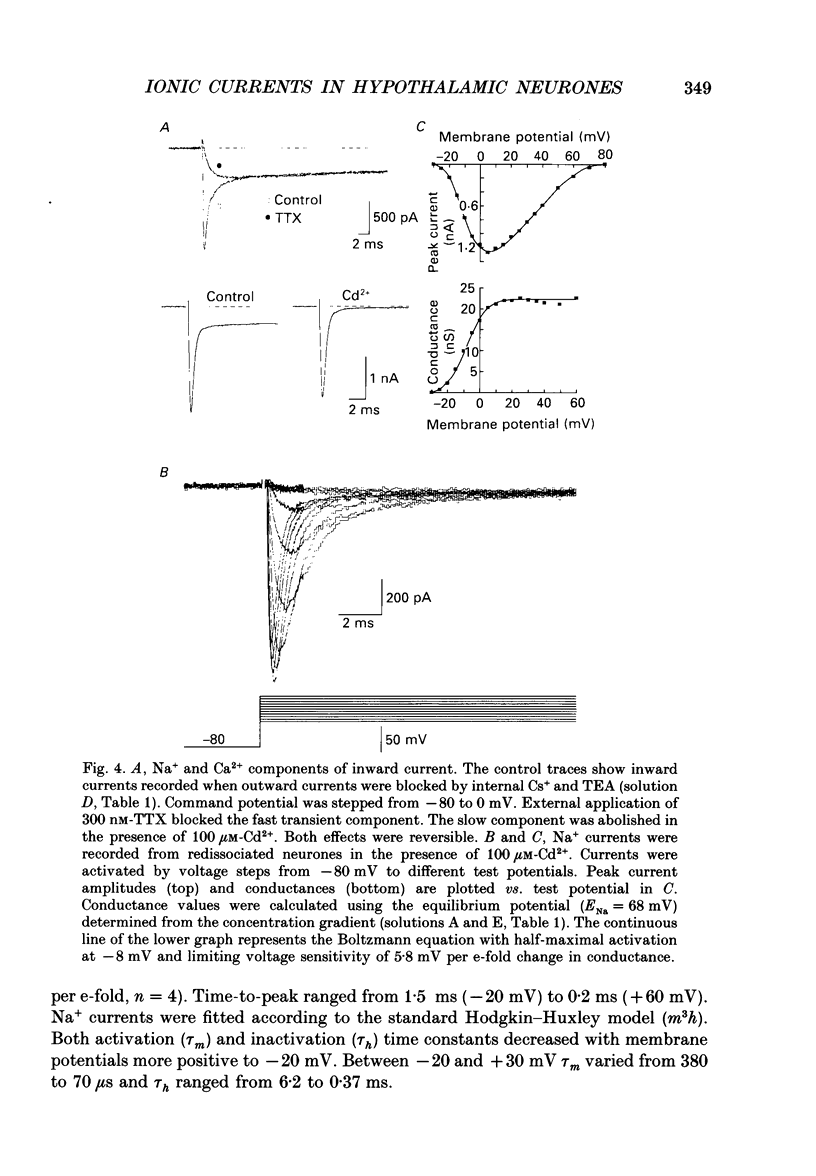
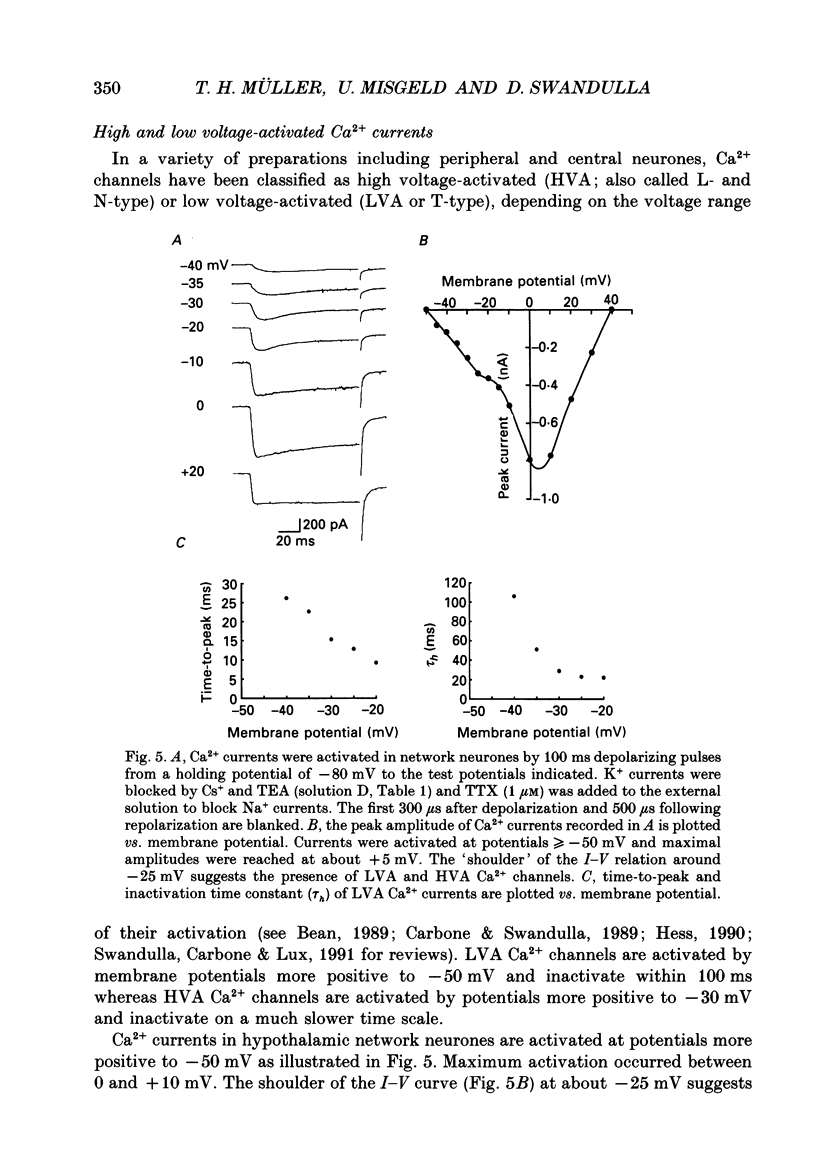
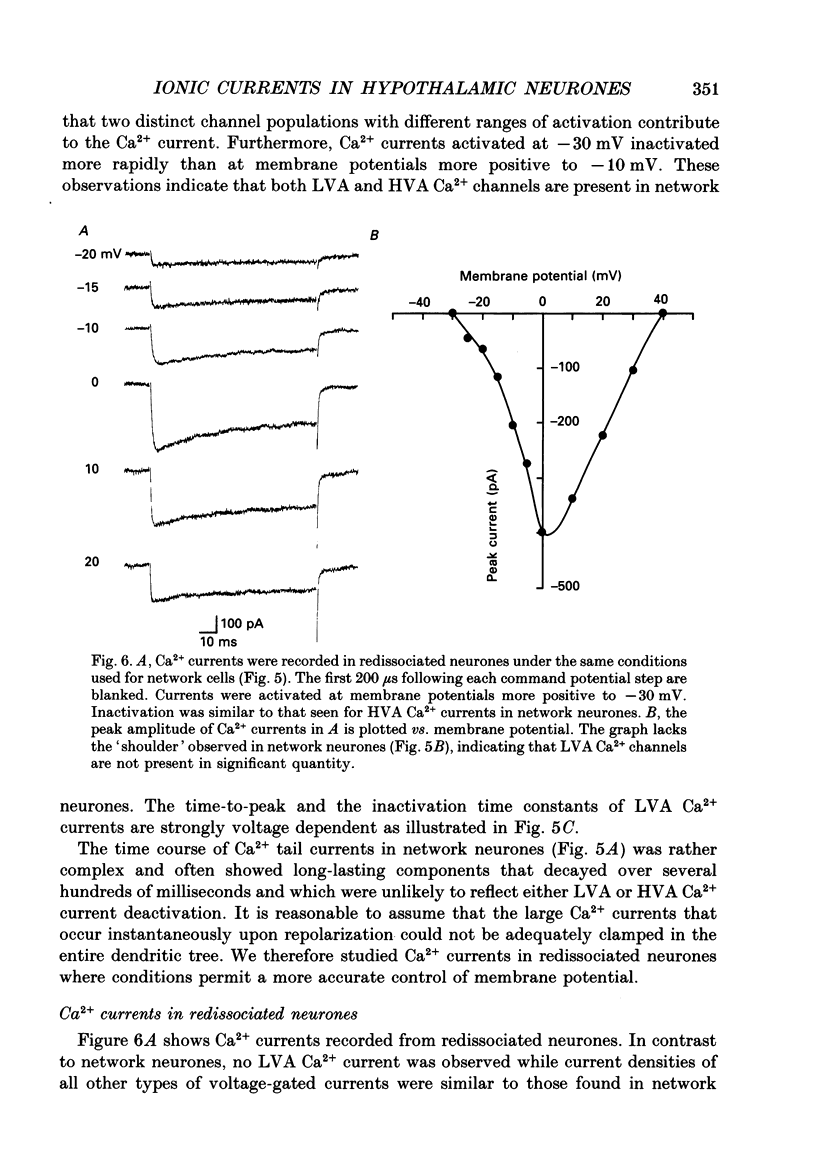
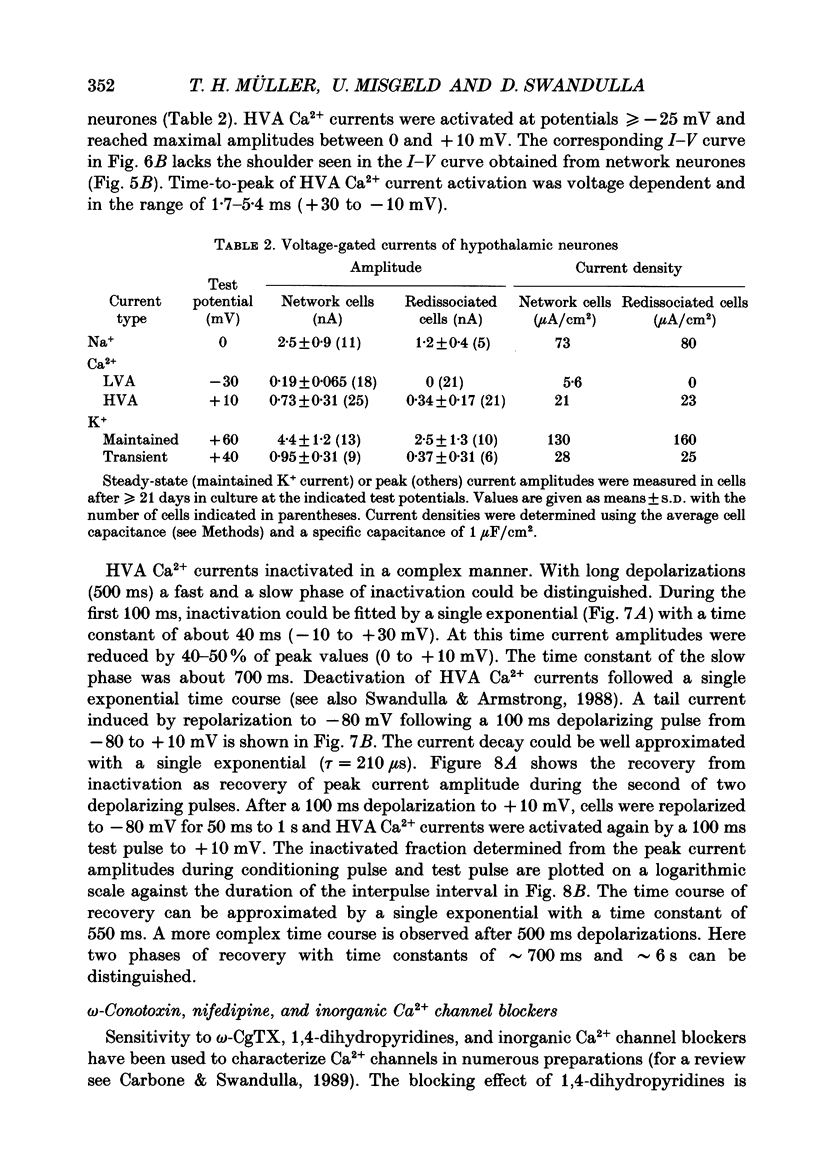
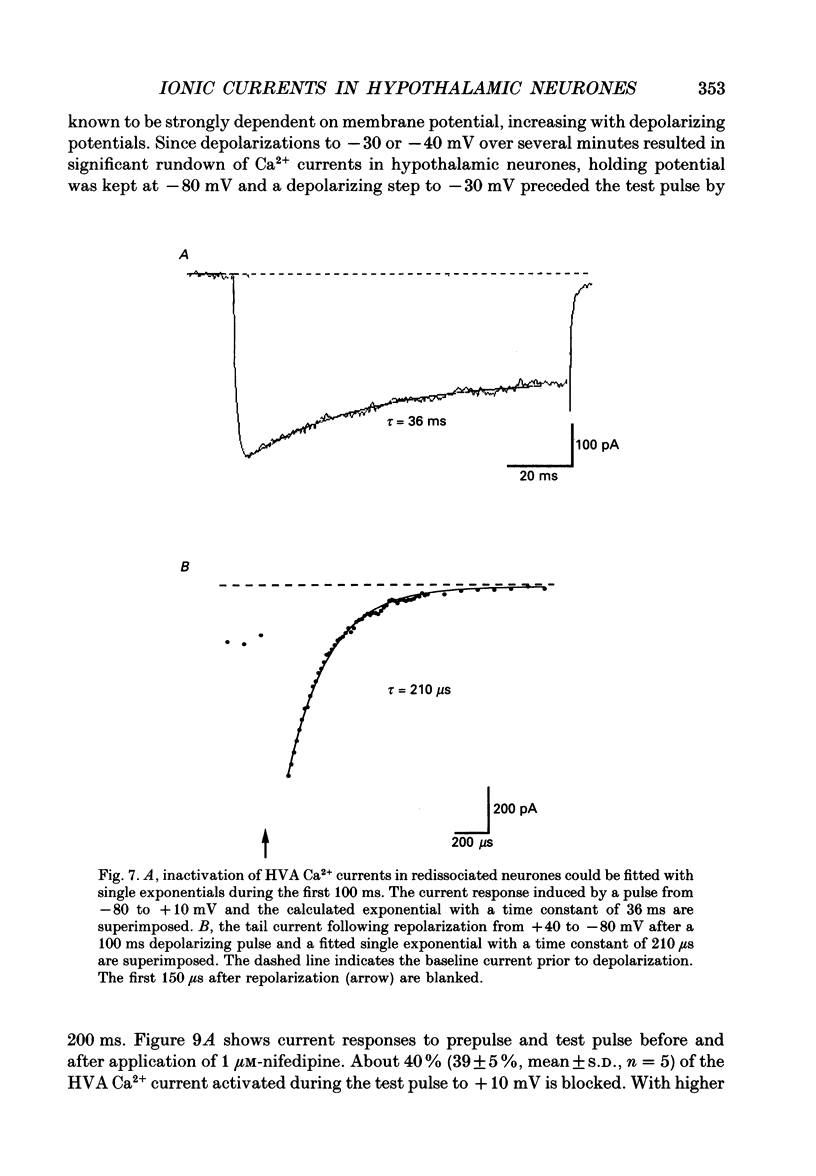
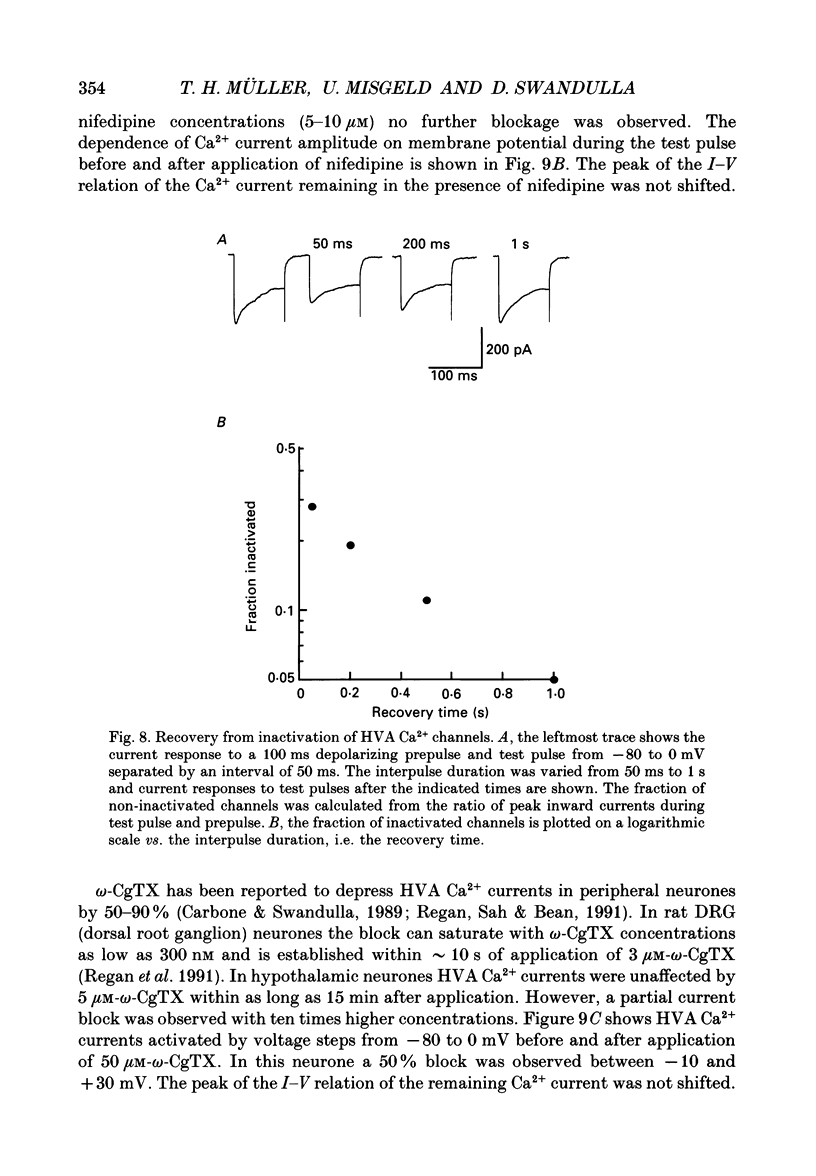
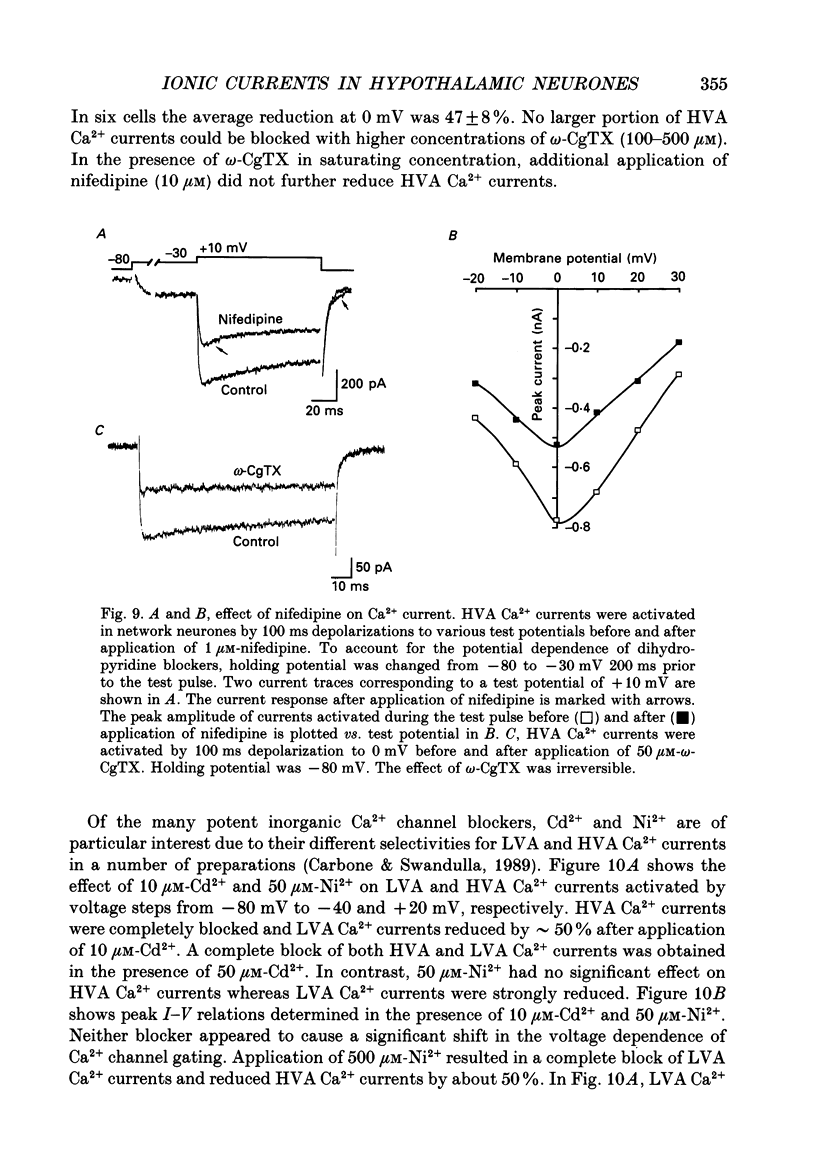
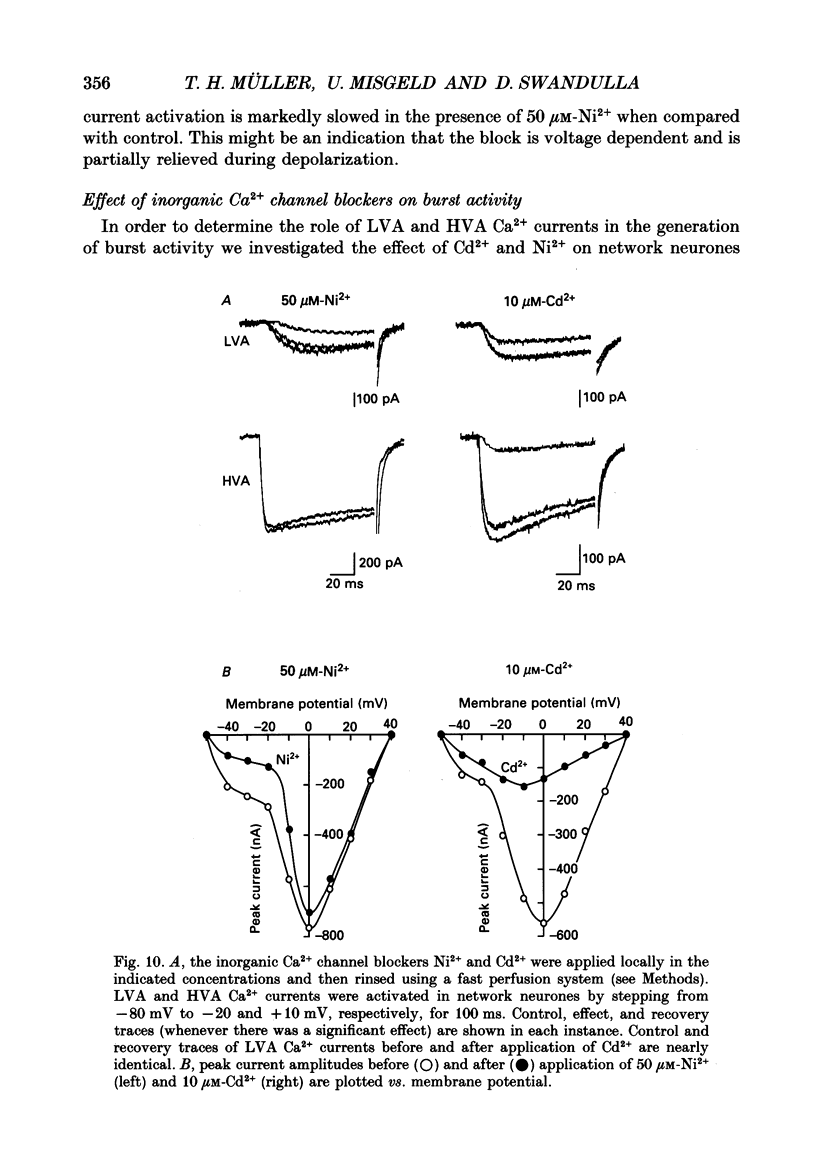
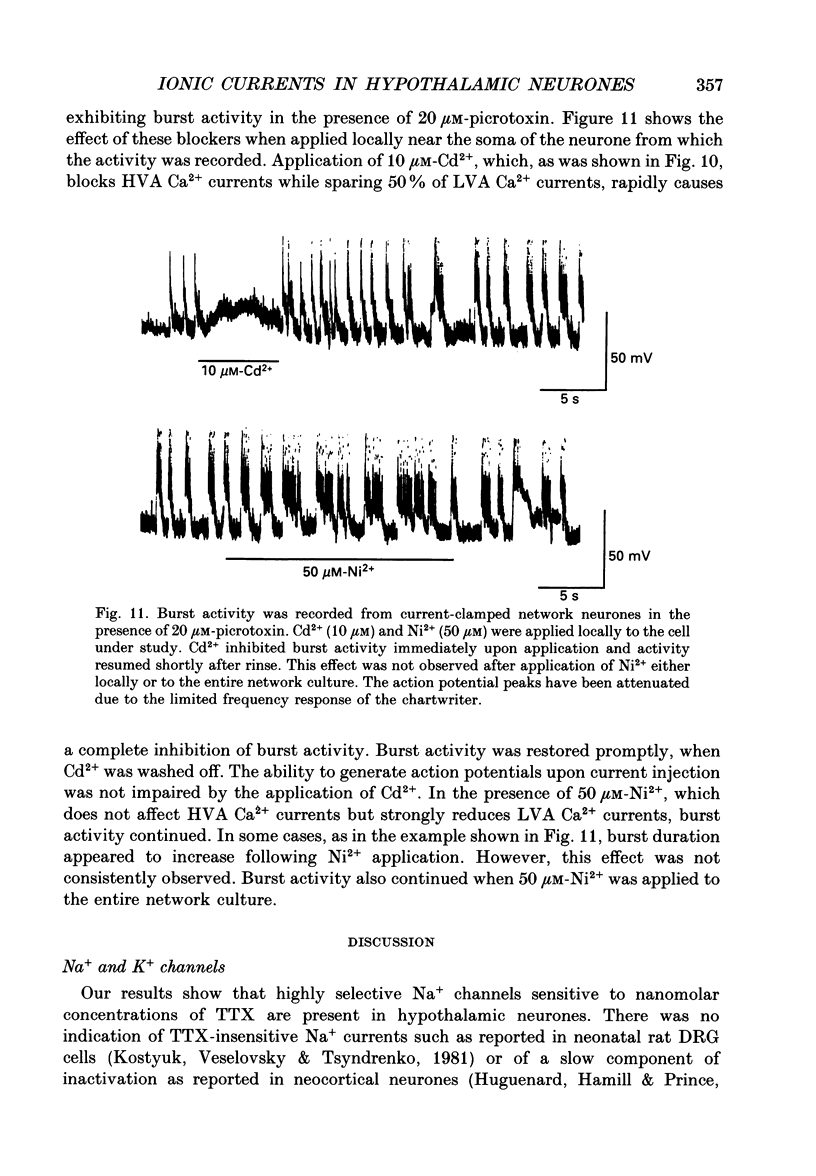
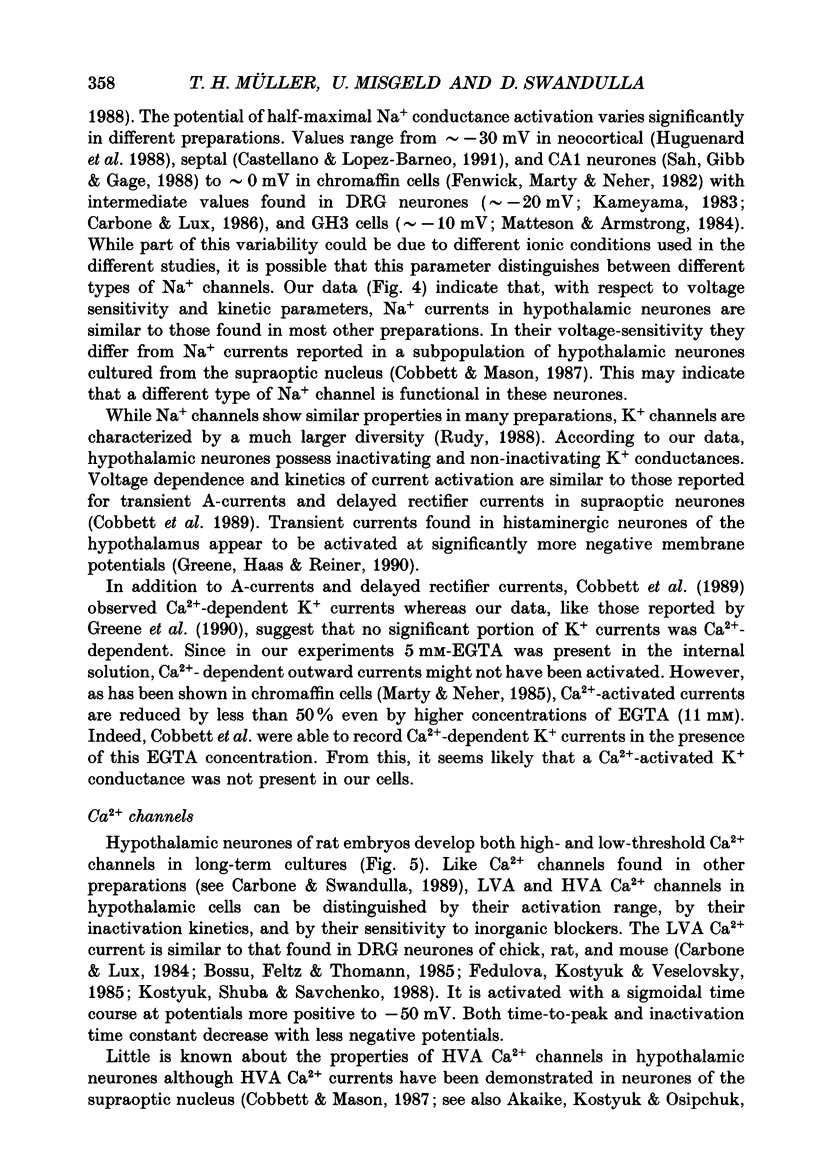
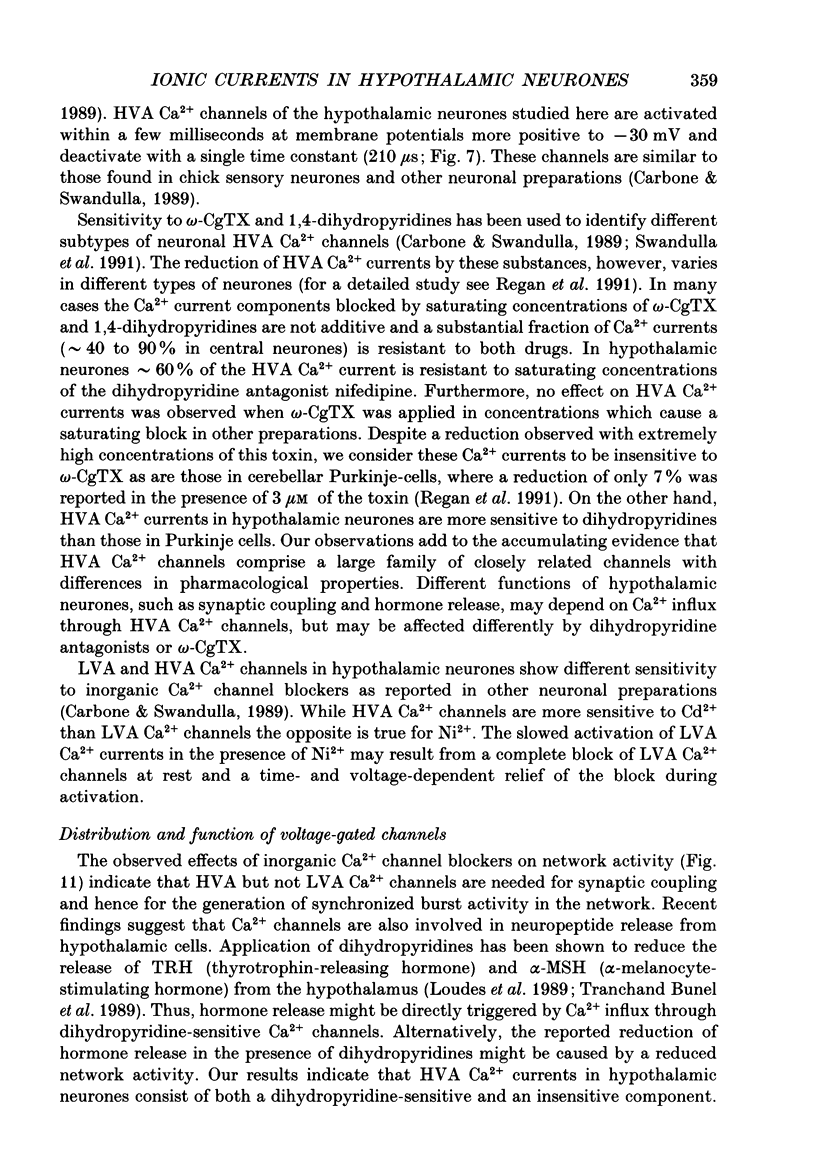
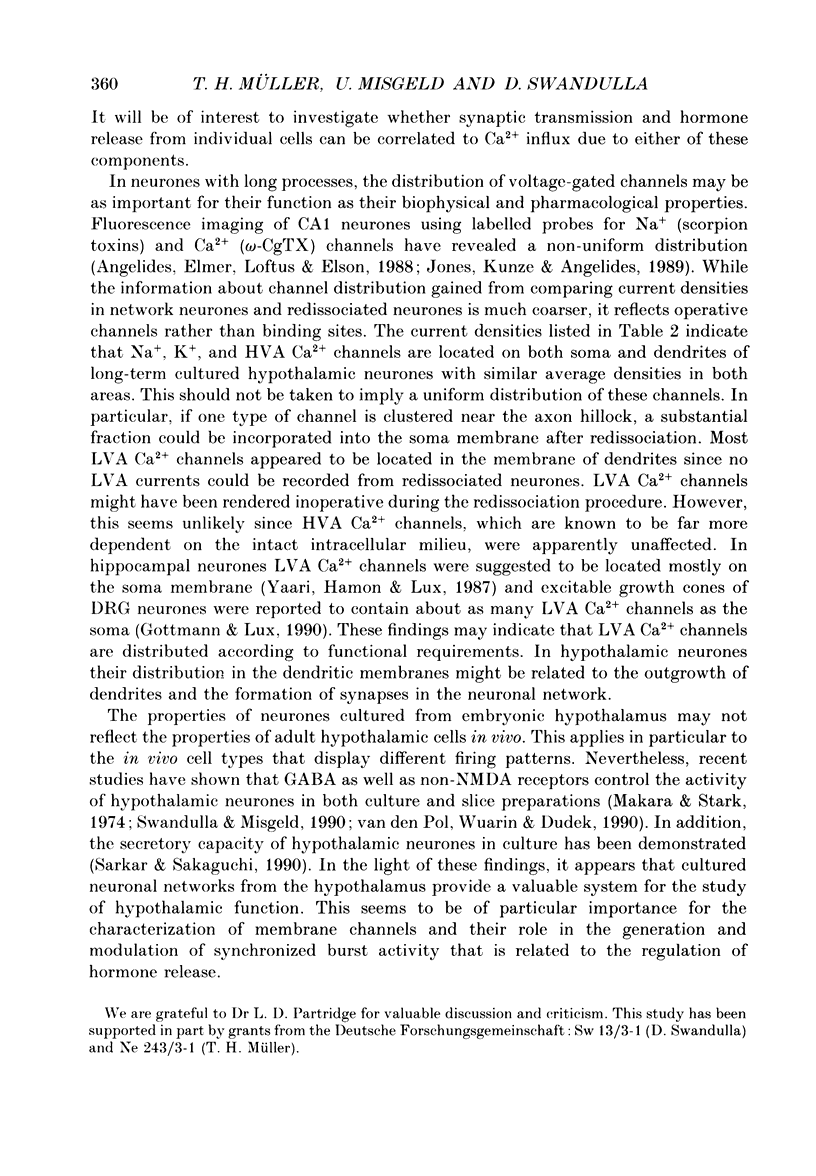
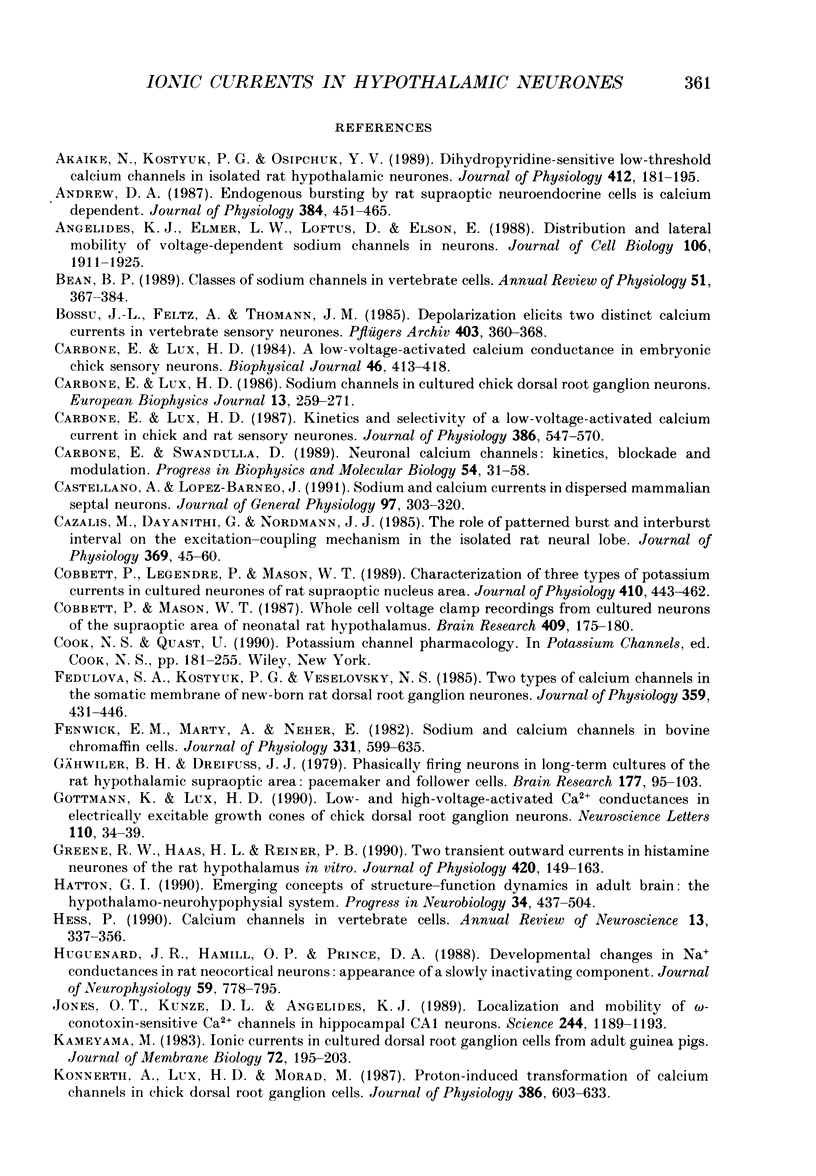
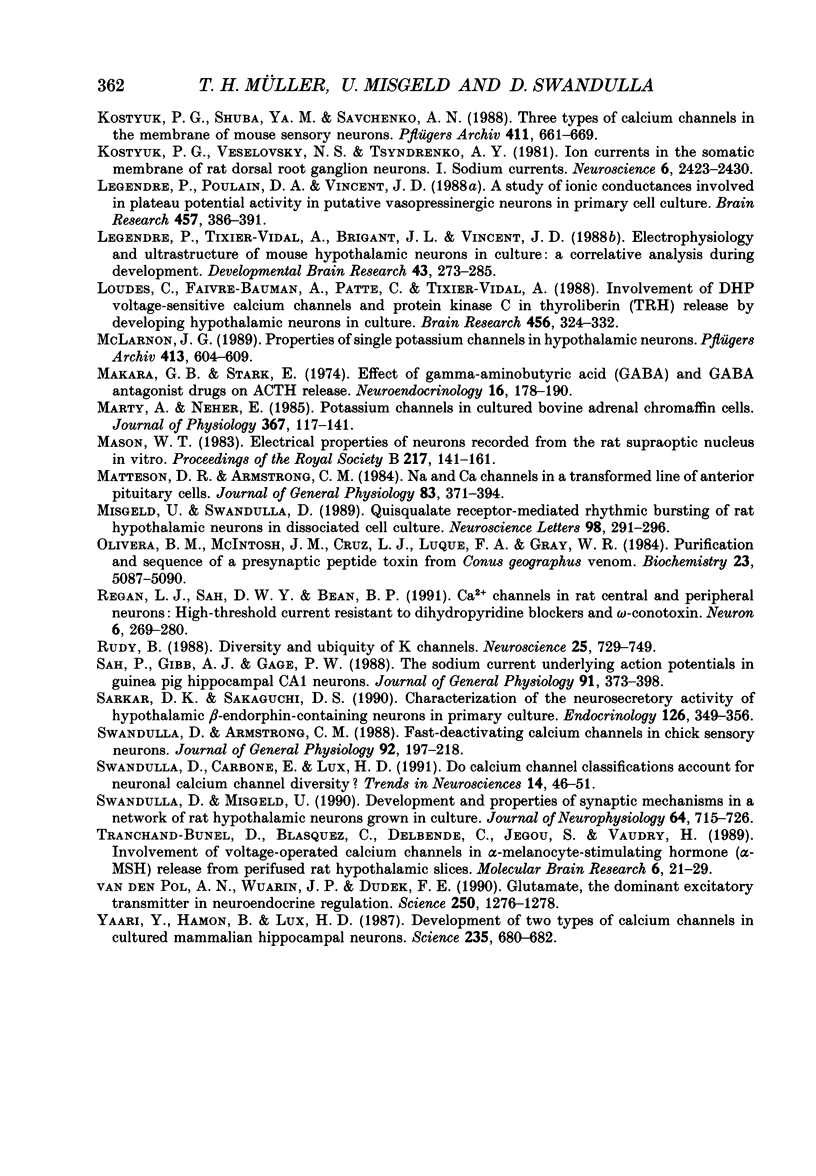
Images in this article
Selected References
These references are in PubMed. This may not be the complete list of references from this article.
- Akaike N., Kostyuk P. G., Osipchuk Y. V. Dihydropyridine-sensitive low-threshold calcium channels in isolated rat hypothalamic neurones. J Physiol. 1989 May;412:181–195. doi: 10.1113/jphysiol.1989.sp017610. [DOI] [PMC free article] [PubMed] [Google Scholar]
- Andrew R. D. Endogenous bursting by rat supraoptic neuroendocrine cells is calcium dependent. J Physiol. 1987 Mar;384:451–465. doi: 10.1113/jphysiol.1987.sp016463. [DOI] [PMC free article] [PubMed] [Google Scholar]
- Angelides K. J., Elmer L. W., Loftus D., Elson E. Distribution and lateral mobility of voltage-dependent sodium channels in neurons. J Cell Biol. 1988 Jun;106(6):1911–1925. doi: 10.1083/jcb.106.6.1911. [DOI] [PMC free article] [PubMed] [Google Scholar]
- Bean B. P. Classes of calcium channels in vertebrate cells. Annu Rev Physiol. 1989;51:367–384. doi: 10.1146/annurev.ph.51.030189.002055. [DOI] [PubMed] [Google Scholar]
- Bossu J. L., Feltz A., Thomann J. M. Depolarization elicits two distinct calcium currents in vertebrate sensory neurones. Pflugers Arch. 1985 Apr;403(4):360–368. doi: 10.1007/BF00589247. [DOI] [PubMed] [Google Scholar]
- Carbone E., Lux H. D. A low voltage-activated calcium conductance in embryonic chick sensory neurons. Biophys J. 1984 Sep;46(3):413–418. doi: 10.1016/S0006-3495(84)84037-0. [DOI] [PMC free article] [PubMed] [Google Scholar]
- Carbone E., Lux H. D. Kinetics and selectivity of a low-voltage-activated calcium current in chick and rat sensory neurones. J Physiol. 1987 May;386:547–570. doi: 10.1113/jphysiol.1987.sp016551. [DOI] [PMC free article] [PubMed] [Google Scholar]
- Carbone E., Swandulla D. Neuronal calcium channels: kinetics, blockade and modulation. Prog Biophys Mol Biol. 1989;54(1):31–58. doi: 10.1016/0079-6107(89)90008-4. [DOI] [PubMed] [Google Scholar]
- Castellano A., López-Barneo J. Sodium and calcium currents in dispersed mammalian septal neurons. J Gen Physiol. 1991 Feb;97(2):303–320. doi: 10.1085/jgp.97.2.303. [DOI] [PMC free article] [PubMed] [Google Scholar]
- Cazalis M., Dayanithi G., Nordmann J. J. The role of patterned burst and interburst interval on the excitation-coupling mechanism in the isolated rat neural lobe. J Physiol. 1985 Dec;369:45–60. doi: 10.1113/jphysiol.1985.sp015887. [DOI] [PMC free article] [PubMed] [Google Scholar]
- Cobbett P., Legendre P., Mason W. T. Characterization of three types of potassium current in cultured neurones of rat supraoptic nucleus area. J Physiol. 1989 Mar;410:443–462. doi: 10.1113/jphysiol.1989.sp017543. [DOI] [PMC free article] [PubMed] [Google Scholar]
- Cobbett P., Mason W. T. Whole cell voltage clamp recordings from cultured neurons of the supraoptic area of neonatal rat hypothalamus. Brain Res. 1987 Apr 14;409(1):175–180. doi: 10.1016/0006-8993(87)90756-6. [DOI] [PubMed] [Google Scholar]
- Fedulova S. A., Kostyuk P. G., Veselovsky N. S. Two types of calcium channels in the somatic membrane of new-born rat dorsal root ganglion neurones. J Physiol. 1985 Feb;359:431–446. doi: 10.1113/jphysiol.1985.sp015594. [DOI] [PMC free article] [PubMed] [Google Scholar]
- Fenwick E. M., Marty A., Neher E. Sodium and calcium channels in bovine chromaffin cells. J Physiol. 1982 Oct;331:599–635. doi: 10.1113/jphysiol.1982.sp014394. [DOI] [PMC free article] [PubMed] [Google Scholar]
- Gottmann K., Lux H. D. Low- and high-voltage-activated Ca2+ conductances in electrically excitable growth cones of chick dorsal root ganglion neurons. Neurosci Lett. 1990 Mar 2;110(1-2):34–39. doi: 10.1016/0304-3940(90)90783-6. [DOI] [PubMed] [Google Scholar]
- Greene R. W., Haas H. L., Reiner P. B. Two transient outward currents in histamine neurones of the rat hypothalamus in vitro. J Physiol. 1990 Jan;420:149–163. doi: 10.1113/jphysiol.1990.sp017905. [DOI] [PMC free article] [PubMed] [Google Scholar]
- Gähwiler B. H., Dreifuss J. J. Phasically firing neurons in long-term cultures of the rat hypothalamic supraoptic area: pacemaker and follower cells. Brain Res. 1979 Nov 9;177(1):95–103. doi: 10.1016/0006-8993(79)90920-x. [DOI] [PubMed] [Google Scholar]
- Hatton G. I. Emerging concepts of structure-function dynamics in adult brain: the hypothalamo-neurohypophysial system. Prog Neurobiol. 1990;34(6):437–504. doi: 10.1016/0301-0082(90)90017-b. [DOI] [PubMed] [Google Scholar]
- Hess P. Calcium channels in vertebrate cells. Annu Rev Neurosci. 1990;13:337–356. doi: 10.1146/annurev.ne.13.030190.002005. [DOI] [PubMed] [Google Scholar]
- Huguenard J. R., Hamill O. P., Prince D. A. Developmental changes in Na+ conductances in rat neocortical neurons: appearance of a slowly inactivating component. J Neurophysiol. 1988 Mar;59(3):778–795. doi: 10.1152/jn.1988.59.3.778. [DOI] [PubMed] [Google Scholar]
- Jones O. T., Kunze D. L., Angelides K. J. Localization and mobility of omega-conotoxin-sensitive Ca2+ channels in hippocampal CA1 neurons. Science. 1989 Jun 9;244(4909):1189–1193. doi: 10.1126/science.2543080. [DOI] [PubMed] [Google Scholar]
- Kameyama M. Ionic currents in cultured dorsal root ganglion cells from adult guinea pigs. J Membr Biol. 1983;72(3):195–203. doi: 10.1007/BF01870586. [DOI] [PubMed] [Google Scholar]
- Konnerth A., Lux H. D., Morad M. Proton-induced transformation of calcium channel in chick dorsal root ganglion cells. J Physiol. 1987 May;386:603–633. doi: 10.1113/jphysiol.1987.sp016553. [DOI] [PMC free article] [PubMed] [Google Scholar]
- Kostyuk P. G., Shuba YaM, Savchenko A. N. Three types of calcium channels in the membrane of mouse sensory neurons. Pflugers Arch. 1988 Jun;411(6):661–669. doi: 10.1007/BF00580863. [DOI] [PubMed] [Google Scholar]
- Kostyuk P. G., Veselovsky N. S., Tsyndrenko A. Y. Ionic currents in the somatic membrane of rat dorsal root ganglion neurons-I. Sodium currents. Neuroscience. 1981;6(12):2423–2430. doi: 10.1016/0306-4522(81)90088-9. [DOI] [PubMed] [Google Scholar]
- Legendre P., Poulain D. A., Vincent J. D. A study of ionic conductances involved in plateau potential activity in putative vasopressinergic neurons in primary cell culture. Brain Res. 1988 Aug 9;457(2):386–391. doi: 10.1016/0006-8993(88)90713-5. [DOI] [PubMed] [Google Scholar]
- Loudes C., Faivre-Bauman A., Patte C., Tixier-Vidal A. Involvement of DHP voltage-sensitive calcium channels and protein kinase C in thyroliberin (TRH) release by developing hypothalamic neurons in culture. Brain Res. 1988 Jul 26;456(2):324–332. doi: 10.1016/0006-8993(88)90235-1. [DOI] [PubMed] [Google Scholar]
- Makara G. B., Stark E. Effects of gamma-aminobutyric acid (GABA) and GABA antagonist drugs on ACTH release. Neuroendocrinology. 1974;16(3-4):178–190. doi: 10.1159/000122564. [DOI] [PubMed] [Google Scholar]
- Marty A., Neher E. Potassium channels in cultured bovine adrenal chromaffin cells. J Physiol. 1985 Oct;367:117–141. doi: 10.1113/jphysiol.1985.sp015817. [DOI] [PMC free article] [PubMed] [Google Scholar]
- Mason W. T. Electrical properties of neurons recorded from the rat supraoptic nucleus in vitro. Proc R Soc Lond B Biol Sci. 1983 Jan 22;217(1207):141–161. doi: 10.1098/rspb.1983.0003. [DOI] [PubMed] [Google Scholar]
- Matteson D. R., Armstrong C. M. Na and Ca channels in a transformed line of anterior pituitary cells. J Gen Physiol. 1984 Mar;83(3):371–394. doi: 10.1085/jgp.83.3.371. [DOI] [PMC free article] [PubMed] [Google Scholar]
- McLarnon J. G. Properties of single potassium channels in hypothalamic neurons. Pflugers Arch. 1989 Apr;413(6):604–609. doi: 10.1007/BF00581809. [DOI] [PubMed] [Google Scholar]
- Misgeld U., Swandulla D. Quisqualate receptor-mediated rhythmic bursting of rat hypothalamic neurons in dissociated cell culture. Neurosci Lett. 1989 Apr 10;98(3):291–296. doi: 10.1016/0304-3940(89)90416-3. [DOI] [PubMed] [Google Scholar]
- Olivera B. M., McIntosh J. M., Cruz L. J., Luque F. A., Gray W. R. Purification and sequence of a presynaptic peptide toxin from Conus geographus venom. Biochemistry. 1984 Oct 23;23(22):5087–5090. doi: 10.1021/bi00317a001. [DOI] [PubMed] [Google Scholar]
- Regan L. J., Sah D. W., Bean B. P. Ca2+ channels in rat central and peripheral neurons: high-threshold current resistant to dihydropyridine blockers and omega-conotoxin. Neuron. 1991 Feb;6(2):269–280. doi: 10.1016/0896-6273(91)90362-4. [DOI] [PubMed] [Google Scholar]
- Rudy B. Diversity and ubiquity of K channels. Neuroscience. 1988 Jun;25(3):729–749. doi: 10.1016/0306-4522(88)90033-4. [DOI] [PubMed] [Google Scholar]
- Sah P., Gibb A. J., Gage P. W. The sodium current underlying action potentials in guinea pig hippocampal CA1 neurons. J Gen Physiol. 1988 Mar;91(3):373–398. doi: 10.1085/jgp.91.3.373. [DOI] [PMC free article] [PubMed] [Google Scholar]
- Sarkar D. K., Sakaguchi D. S. Characterization of the neurosecretory activity of hypothalamic beta-endorphin-containing neurons in primary culture. Endocrinology. 1990 Jan;126(1):349–356. doi: 10.1210/endo-126-1-349. [DOI] [PubMed] [Google Scholar]
- Swandulla D., Armstrong C. M. Fast-deactivating calcium channels in chick sensory neurons. J Gen Physiol. 1988 Aug;92(2):197–218. doi: 10.1085/jgp.92.2.197. [DOI] [PMC free article] [PubMed] [Google Scholar]
- Swandulla D., Carbone E., Lux H. D. Do calcium channel classifications account for neuronal calcium channel diversity? Trends Neurosci. 1991 Feb;14(2):46–51. doi: 10.1016/0166-2236(91)90018-p. [DOI] [PubMed] [Google Scholar]
- Swandulla D., Misgeld U. Development and properties of synaptic mechanisms in a network of rat hypothalamic neurons grown in culture. J Neurophysiol. 1990 Sep;64(3):715–726. doi: 10.1152/jn.1990.64.3.715. [DOI] [PubMed] [Google Scholar]
- Tranchand Bunel D., Blasquez C., Delbende C., Jégou S., Vaudry H. Involvement of voltage-operated calcium channels in alpha-melanocyte-stimulating hormone (alpha-MSH) release from perifused rat hypothalamic slices. Brain Res Mol Brain Res. 1989 Jul;6(1):21–29. doi: 10.1016/0169-328x(89)90024-7. [DOI] [PubMed] [Google Scholar]
- Yaari Y., Hamon B., Lux H. D. Development of two types of calcium channels in cultured mammalian hippocampal neurons. Science. 1987 Feb 6;235(4789):680–682. doi: 10.1126/science.2433765. [DOI] [PubMed] [Google Scholar]
- van den Pol A. N., Wuarin J. P., Dudek F. E. Glutamate, the dominant excitatory transmitter in neuroendocrine regulation. Science. 1990 Nov 30;250(4985):1276–1278. doi: 10.1126/science.1978759. [DOI] [PubMed] [Google Scholar]