Abstract
1. By using the whole-cell patch clamp method Ca2+ and Ba2+ currents were measured in the extremely thin twitch muscle cells of the protochordate Branchiostoma lanceolatum whose Ca2+ channels are likely to resemble the evolutionary ancestors of those found in vertebrate skeletal muscle. 2. When using 10 mM-Ca2+ in the artificial external solution and 1 mM-EGTA in the internal solution two kinetically different Ca2+ inward current components could be observed, showing very similar voltage dependence of activation and inactivation. 3. In solutions containing 10 mM-Ba2+ as an external charge carrier the biphasic inward current turned into a single rapidly activated and slowly inactivating current. 4. Inspecting peak currents, the voltage dependence of fractional activation and inactivation was nearly the same in Ca2+ and in Ba2+. 5. A transformation into a single component of the Ca2+ current could also be observed after perfusing the intracellular lumen with 10 mM of either EGTA or BAPTA. In the case of EGTA this transformation required considerably more time. Probably a higher internal concentration of EGTA is necessary since it binds Ca2+ more slowly than BAPTA. 6. Soon after establishing the whole-cell configuration a gradual increase of the second, slow inward current phase was observed relative to the fast component, indicating an enhancement of the slow component by intermediate intracellular buffer concentrations. 7. We conclude that the Branchiostoma myotome cells have only one Ca2+ channel system. The biphasic appearance of the inward current is caused by an unusually rapid inactivation due to Ca2+ ions, which enter the myoplasm during the current and temporarily bind to an inactivation site at the channel. The second phase probably reflects reactivation from the inactivated state upon dissociation of Ca2+ from the binding site.
Full text
PDF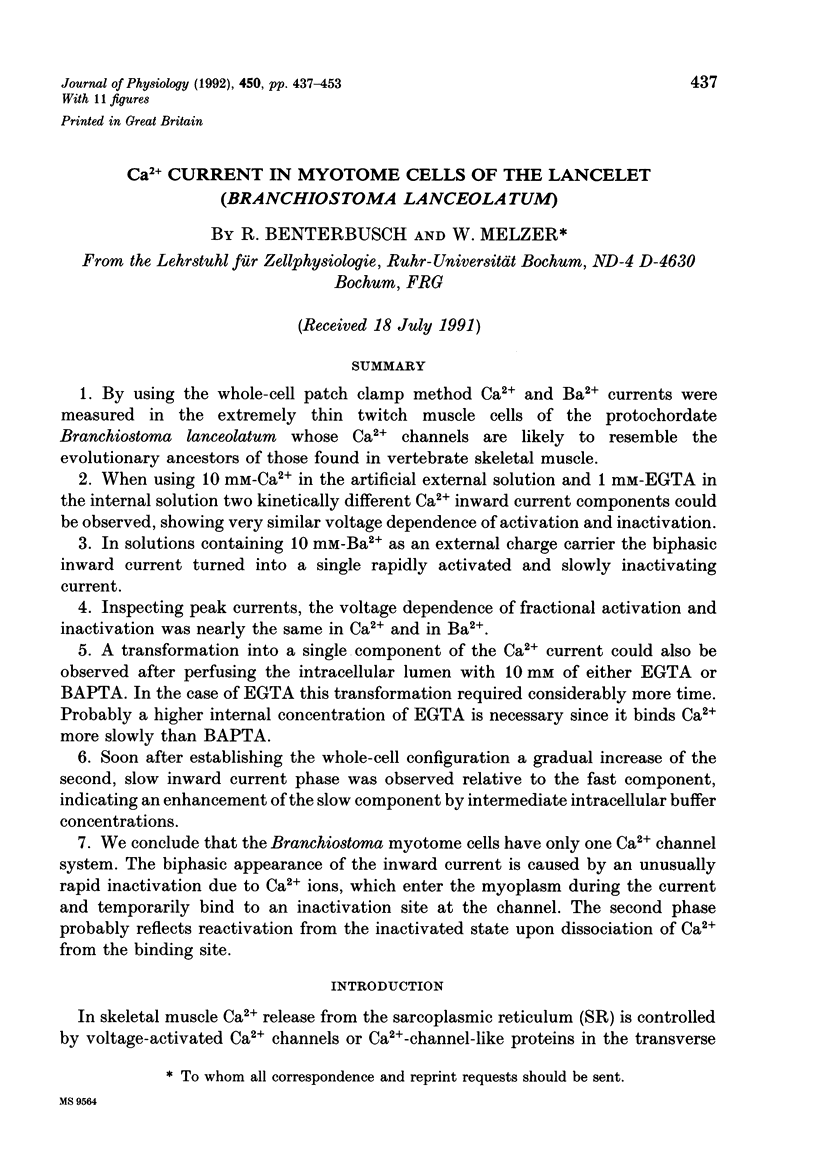
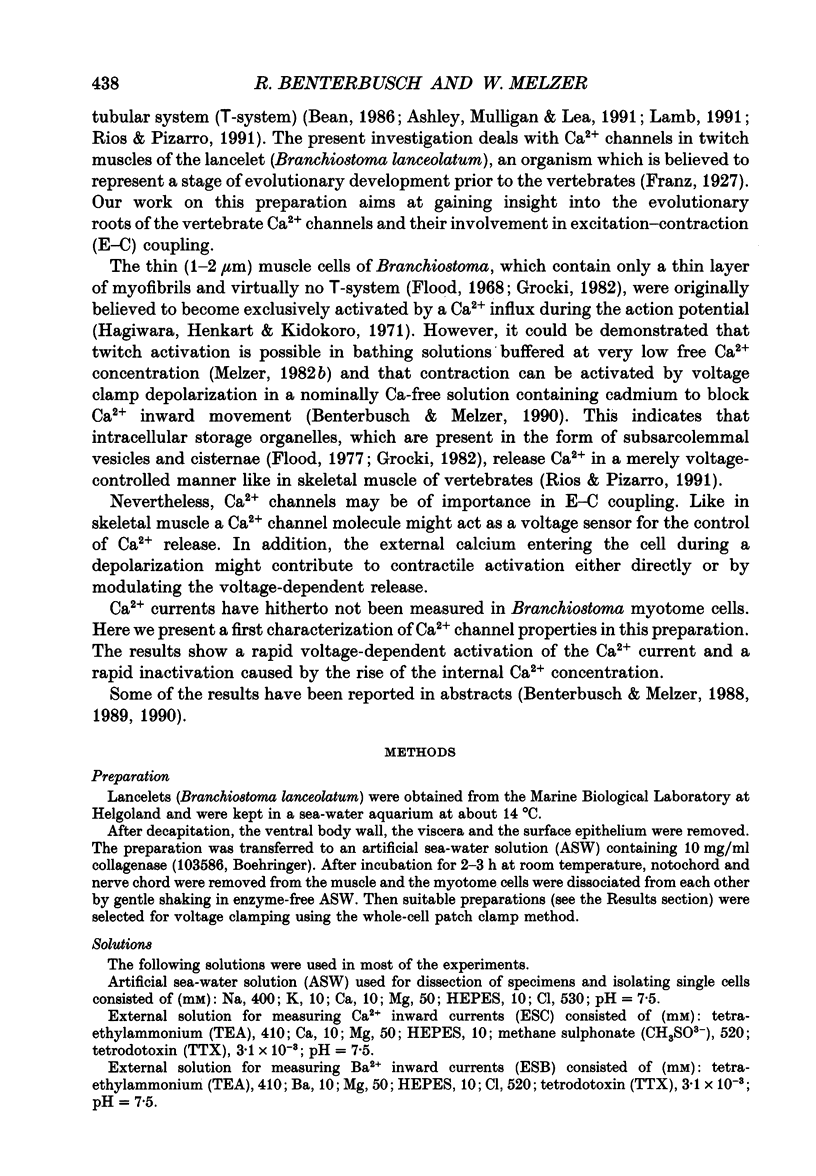
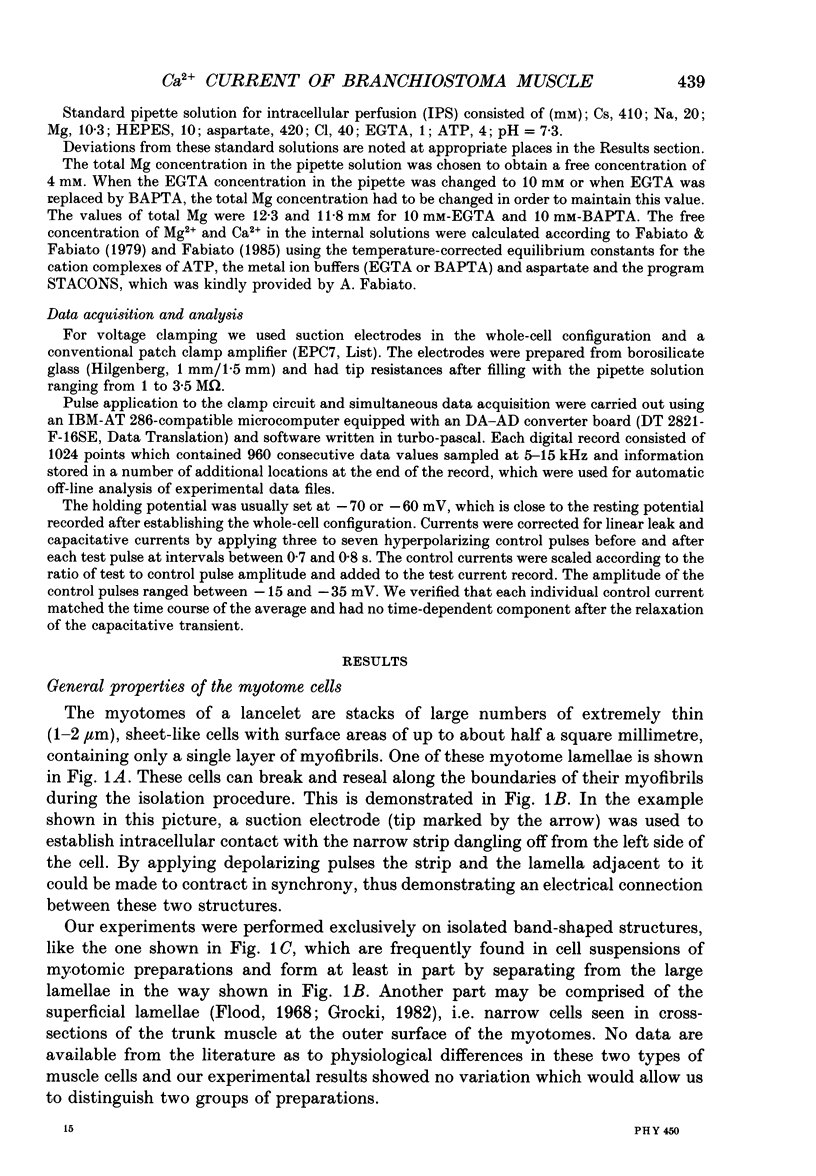
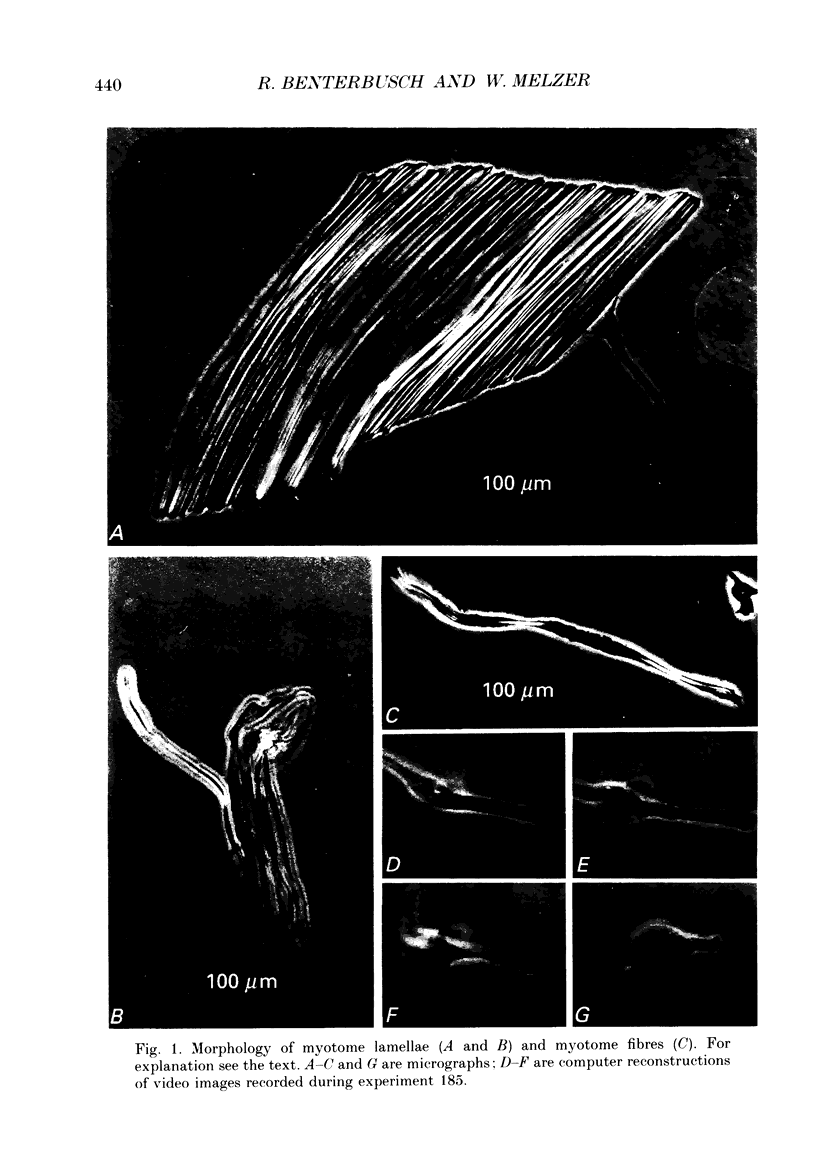
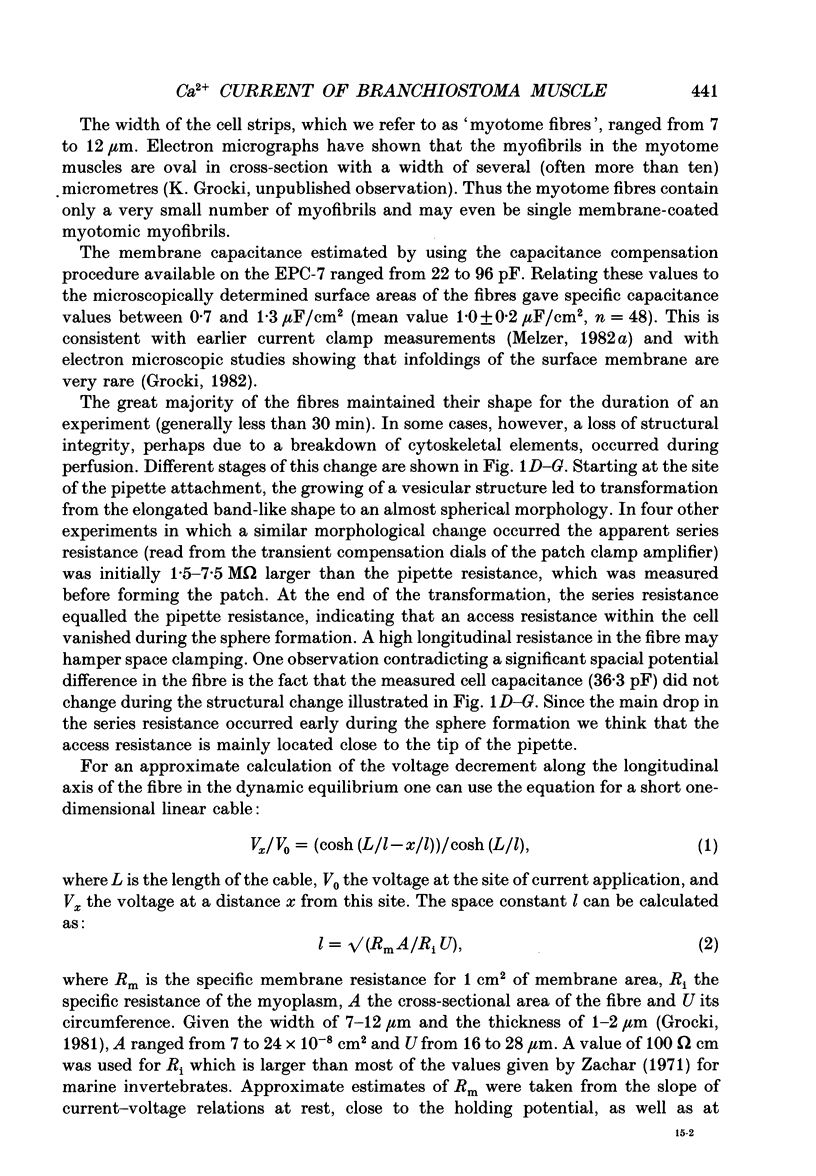
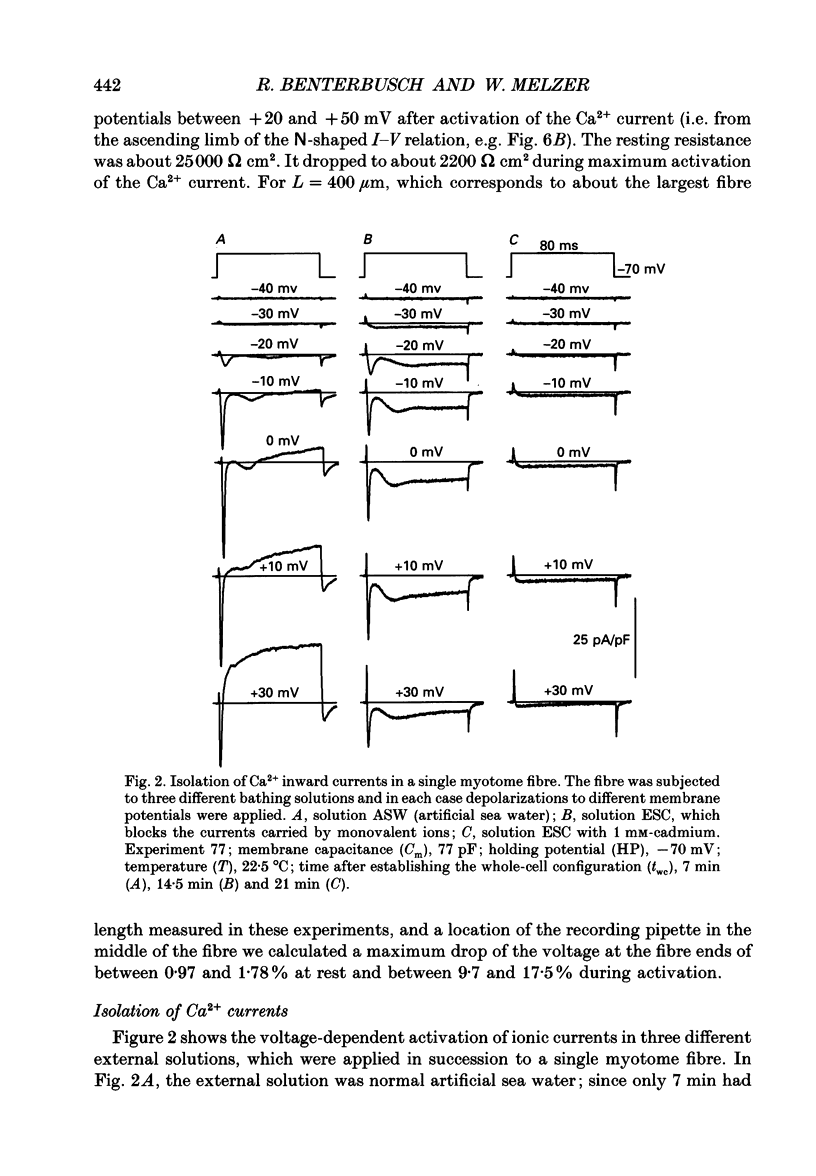
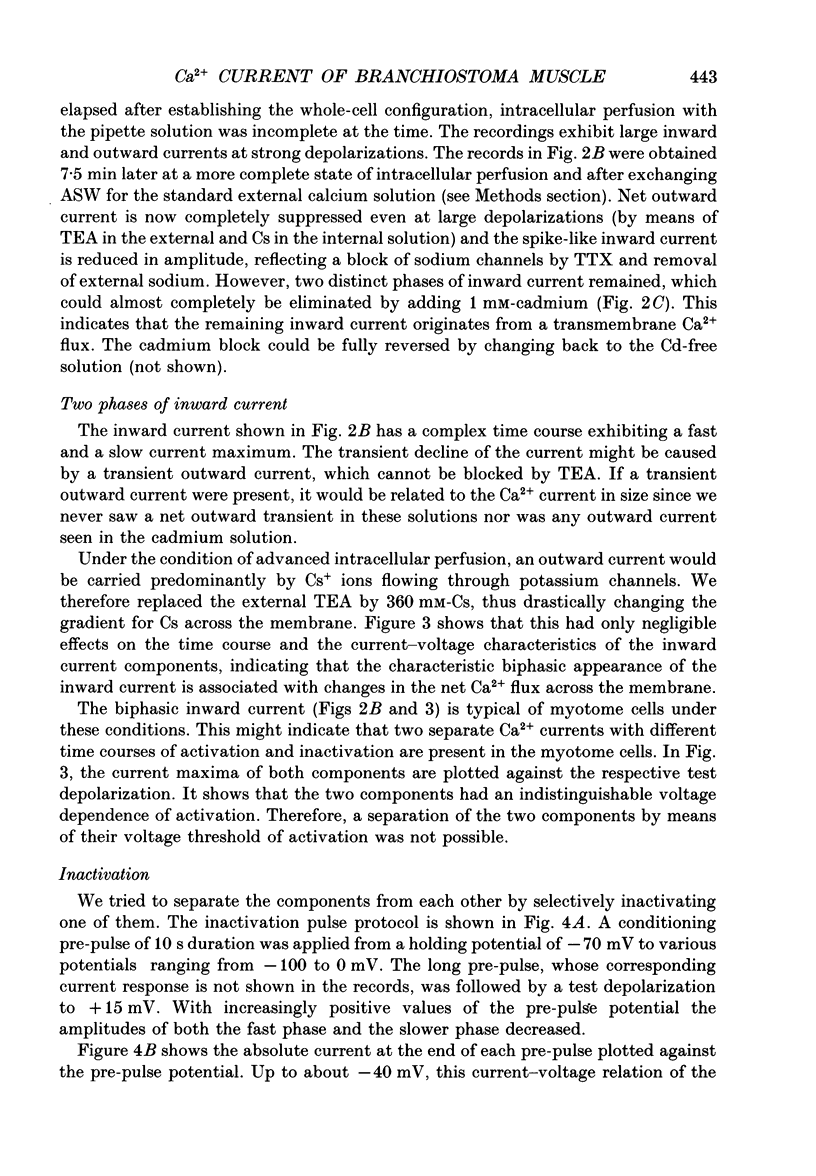
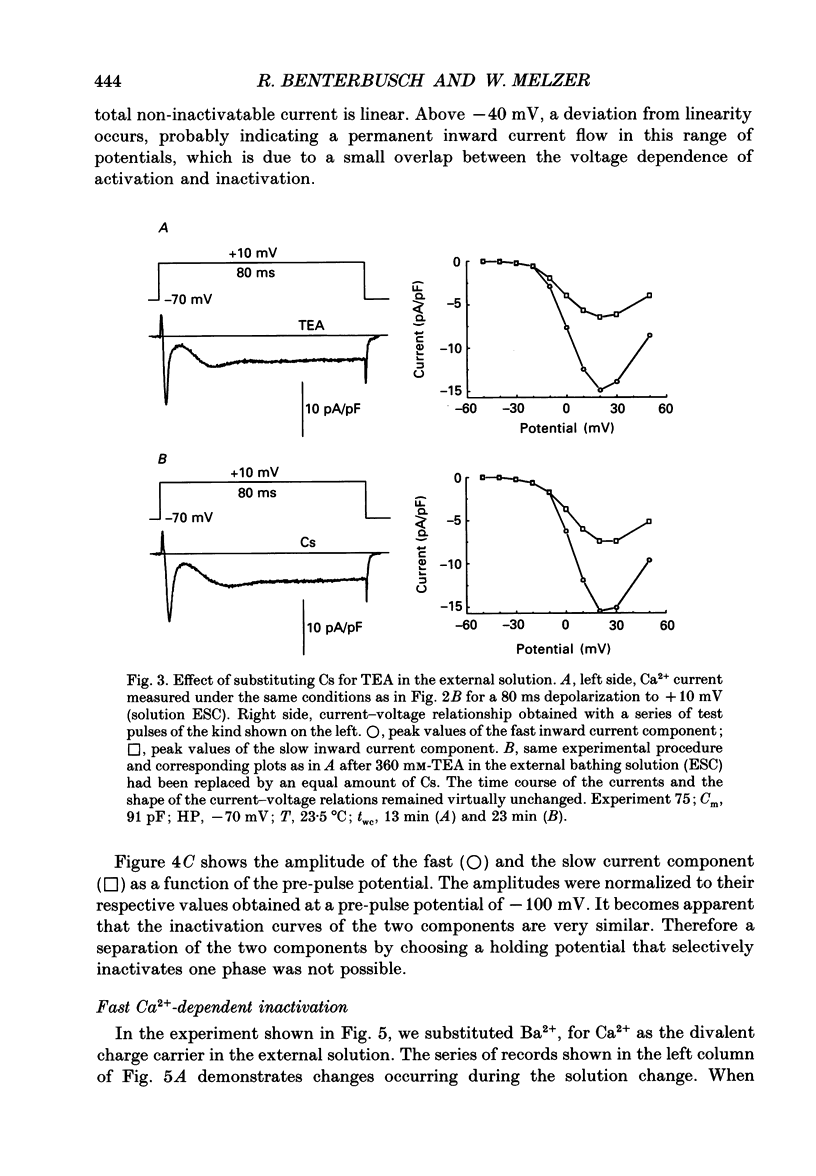
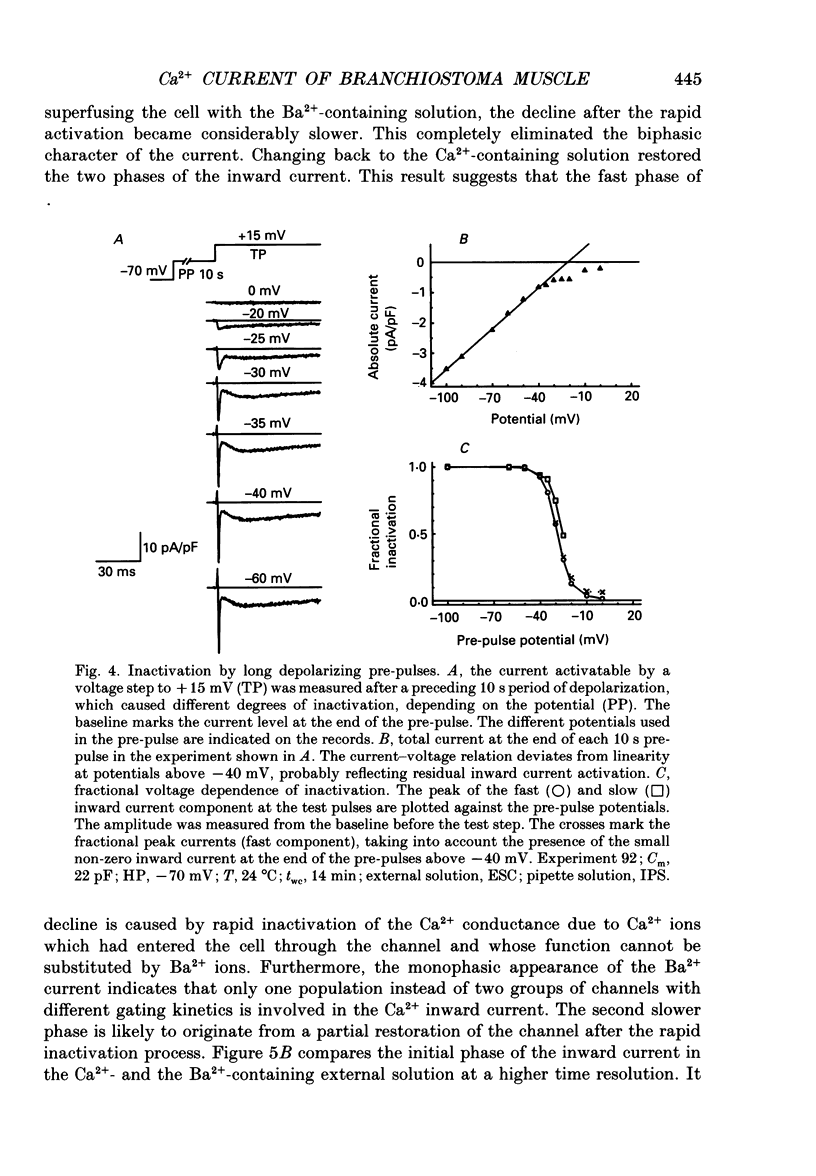
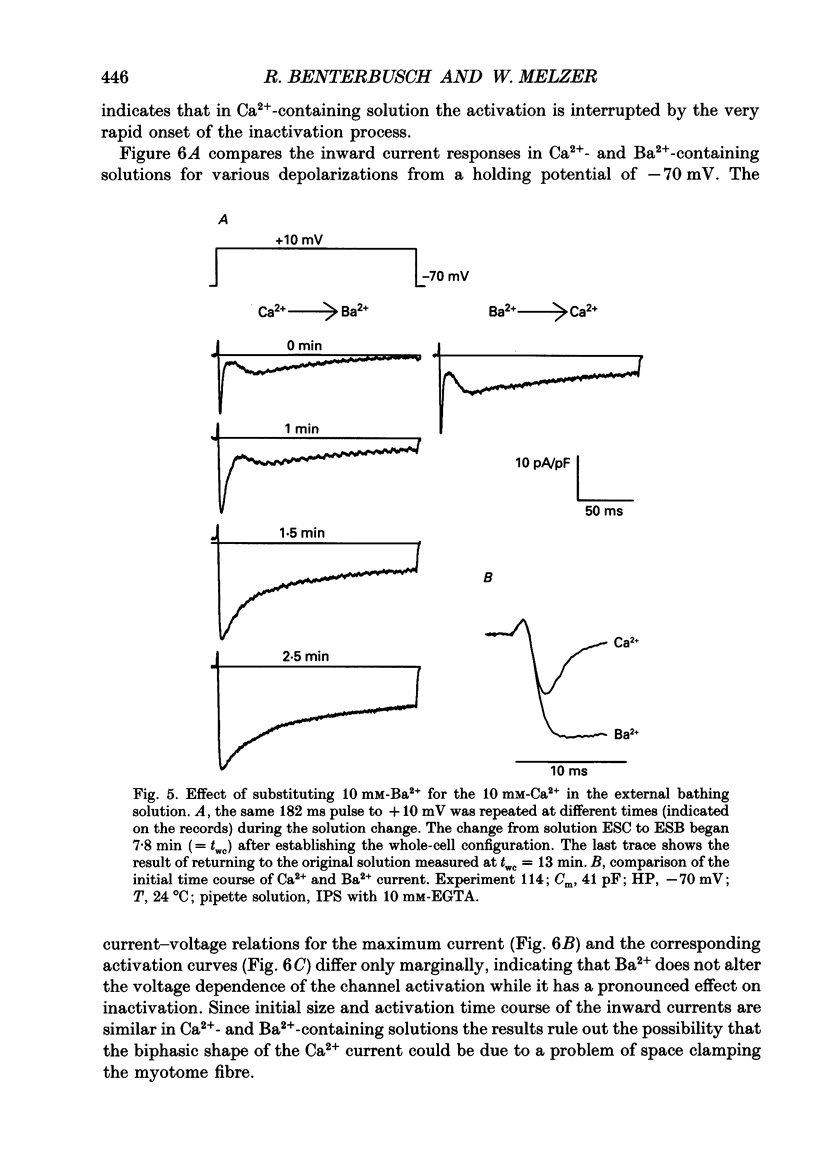
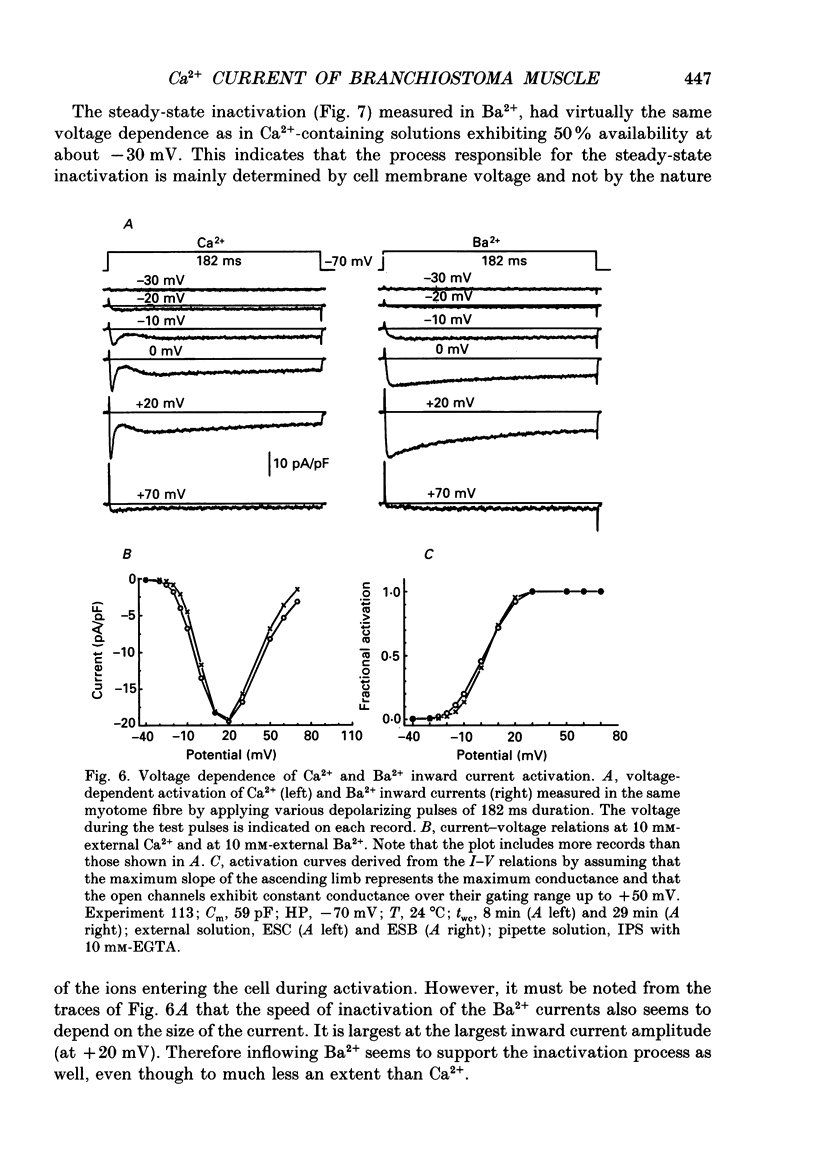
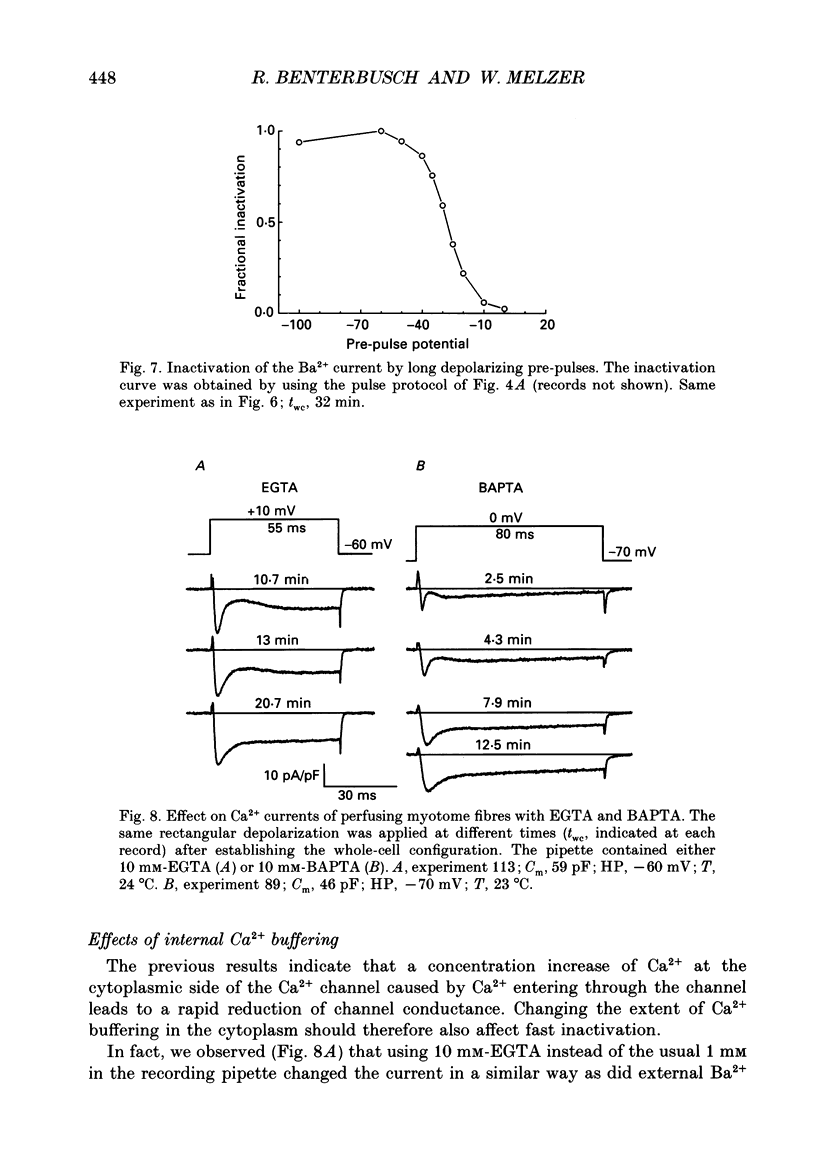
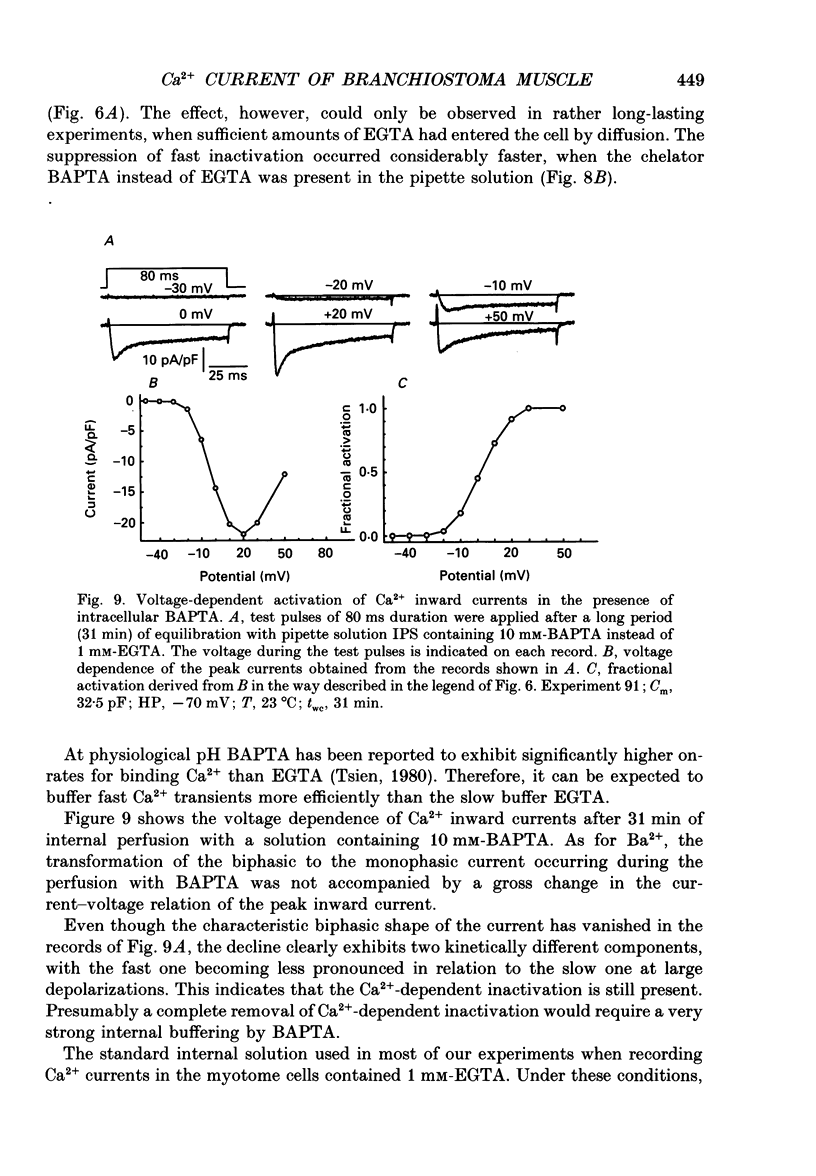
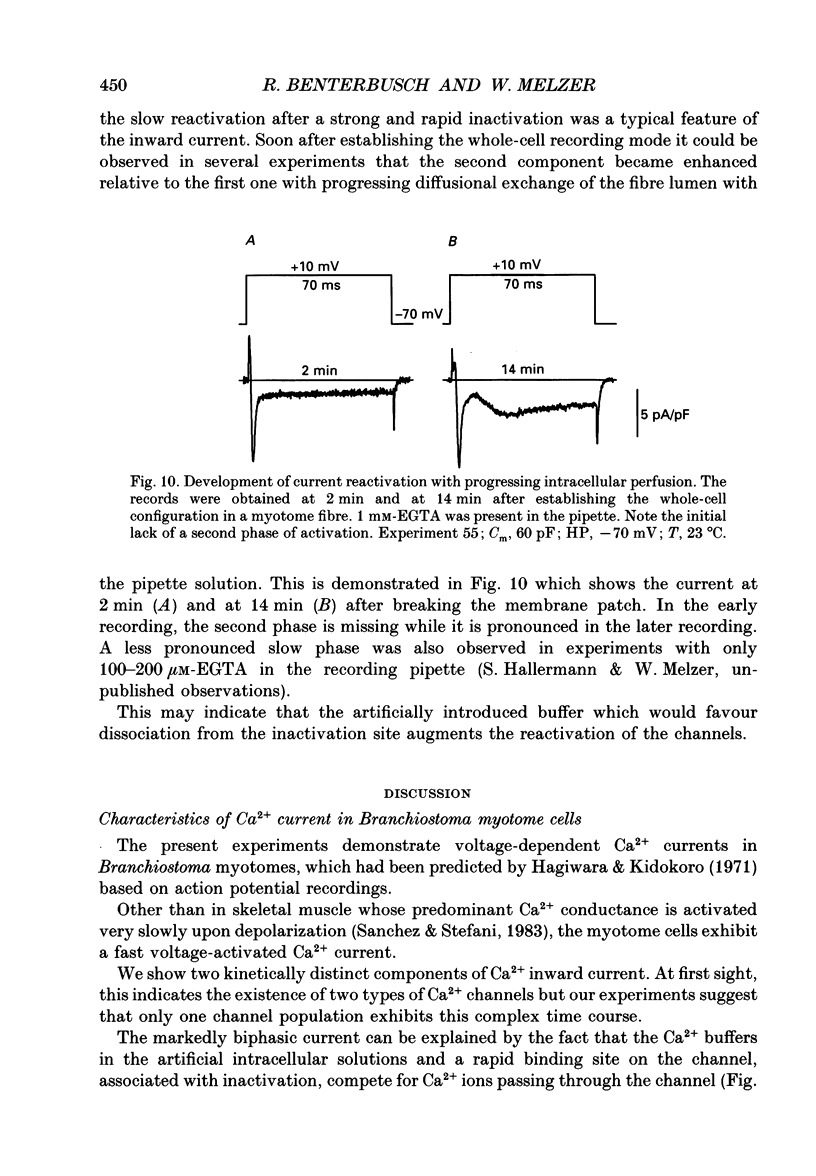
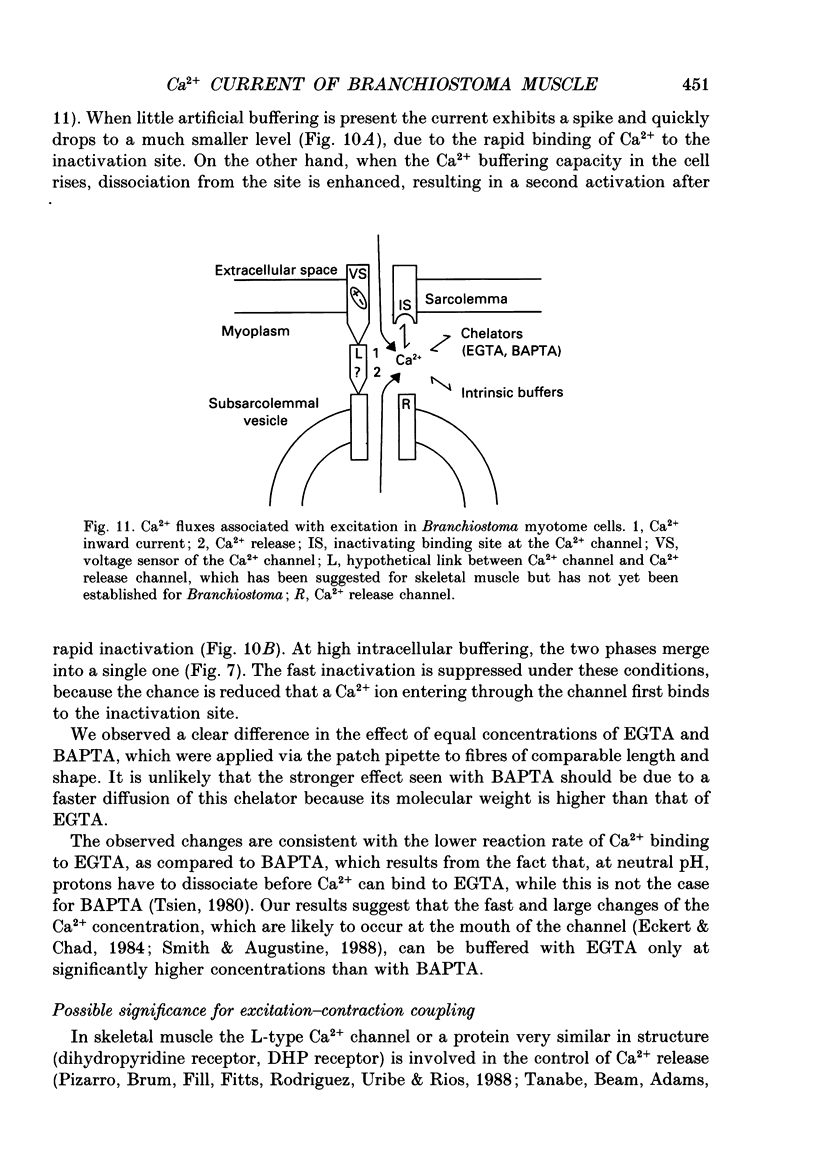
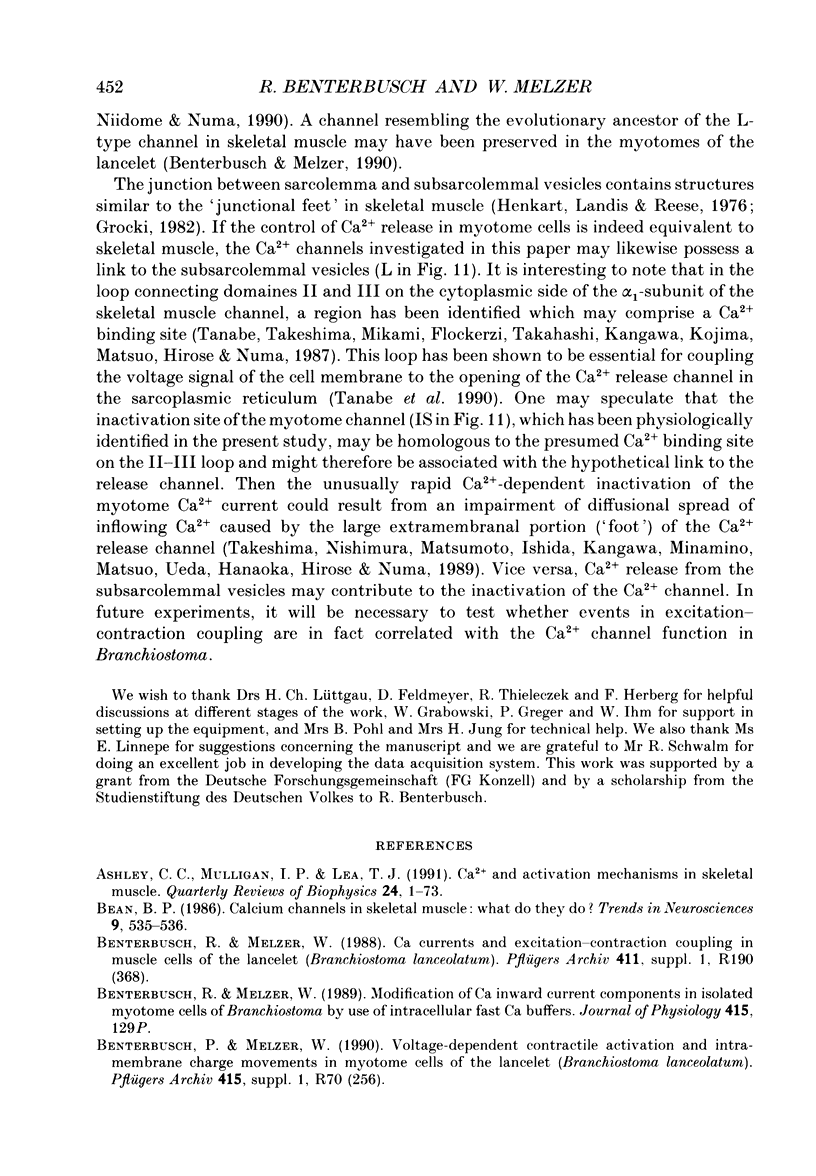
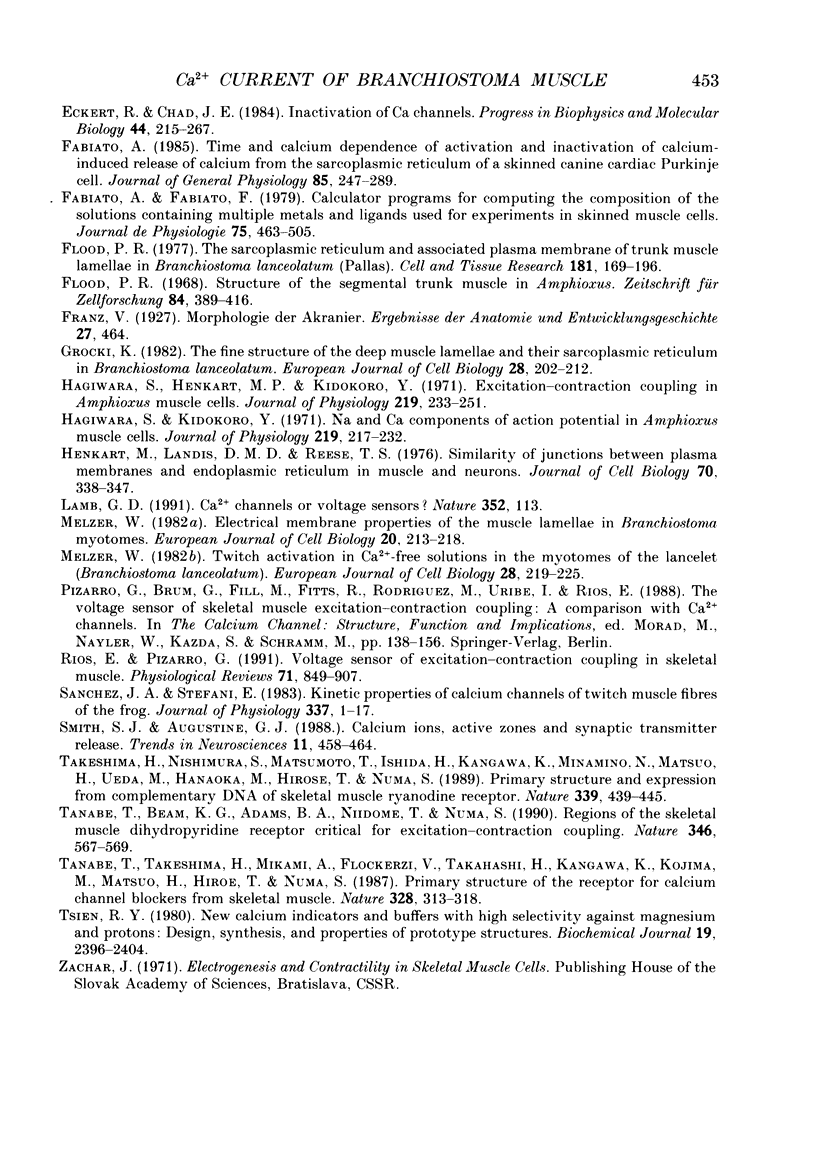
Images in this article
Selected References
These references are in PubMed. This may not be the complete list of references from this article.
- Ashley C. C., Mulligan I. P., Lea T. J. Ca2+ and activation mechanisms in skeletal muscle. Q Rev Biophys. 1991 Feb;24(1):1–73. doi: 10.1017/s0033583500003267. [DOI] [PubMed] [Google Scholar]
- Eckert R., Chad J. E. Inactivation of Ca channels. Prog Biophys Mol Biol. 1984;44(3):215–267. doi: 10.1016/0079-6107(84)90009-9. [DOI] [PubMed] [Google Scholar]
- Fabiato A., Fabiato F. Calculator programs for computing the composition of the solutions containing multiple metals and ligands used for experiments in skinned muscle cells. J Physiol (Paris) 1979;75(5):463–505. [PubMed] [Google Scholar]
- Fabiato A. Time and calcium dependence of activation and inactivation of calcium-induced release of calcium from the sarcoplasmic reticulum of a skinned canine cardiac Purkinje cell. J Gen Physiol. 1985 Feb;85(2):247–289. doi: 10.1085/jgp.85.2.247. [DOI] [PMC free article] [PubMed] [Google Scholar]
- Flood P. R. Structure of the segmental trunk muscle in amphioxus. With notes on the course and "endings" of the so-called ventral root fibres. Z Zellforsch Mikrosk Anat. 1968;84(3):389–416. [PubMed] [Google Scholar]
- Flood P. R. The sarcoplasmic reticulum and associated plasma membrane of trunk muscle lamellae in Branchiostoma lanceolatum (pallas). A transmission and scanning electrom microscopic study including freeze-fractures, direct replicas and x-ray microanalysis of calcium oxalate deposits. Cell Tissue Res. 1977 Jul 11;181(2):169–196. doi: 10.1007/BF00219979. [DOI] [PubMed] [Google Scholar]
- Grocki K. The fine structure of the deep muscle lamellae and their sarcoplasmic reticulum in Branchiostoma lanceolatum. Eur J Cell Biol. 1982 Oct;28(2):202–212. [PubMed] [Google Scholar]
- Hagiwara S., Henkart M. P., Kidokoro Y. Excitation-contraction coupling in amphioxus muscle cells. J Physiol. 1971 Dec;219(1):233–251. doi: 10.1113/jphysiol.1971.sp009659. [DOI] [PMC free article] [PubMed] [Google Scholar]
- Hagiwara S., Kidokoro Y. Na and Ca components of action potential in amphioxus muscle cells. J Physiol. 1971 Dec;219(1):217–232. doi: 10.1113/jphysiol.1971.sp009658. [DOI] [PMC free article] [PubMed] [Google Scholar]
- Henkart M., Landis D. M., Reese T. S. Similarity of junctions between plasma membranes and endoplasmic reticulum in muscle and neurons. J Cell Biol. 1976 Aug;70(2 Pt 1):338–347. doi: 10.1083/jcb.70.2.338. [DOI] [PMC free article] [PubMed] [Google Scholar]
- Lamb G. D. Ca2+ channels or voltage sensors? Nature. 1991 Jul 11;352(6331):113–113. doi: 10.1038/352113b0. [DOI] [PubMed] [Google Scholar]
- Melzer W. Electrical membrane properties of the muscle lamellae in Branchiostoma myotomes. Eur J Cell Biol. 1982 Oct;28(2):213–218. [PubMed] [Google Scholar]
- Melzer W. Twitch activation in Ca2+ -free solutions in the myotomes of the lancelet (Branchiostoma lanceolatum). Eur J Cell Biol. 1982 Oct;28(2):219–225. [PubMed] [Google Scholar]
- Ríos E., Pizarro G. Voltage sensor of excitation-contraction coupling in skeletal muscle. Physiol Rev. 1991 Jul;71(3):849–908. doi: 10.1152/physrev.1991.71.3.849. [DOI] [PubMed] [Google Scholar]
- Smith S. J., Augustine G. J. Calcium ions, active zones and synaptic transmitter release. Trends Neurosci. 1988 Oct;11(10):458–464. doi: 10.1016/0166-2236(88)90199-3. [DOI] [PubMed] [Google Scholar]
- Sánchez J. A., Stefani E. Kinetic properties of calcium channels of twitch muscle fibres of the frog. J Physiol. 1983 Apr;337:1–17. doi: 10.1113/jphysiol.1983.sp014607. [DOI] [PMC free article] [PubMed] [Google Scholar]
- Takeshima H., Nishimura S., Matsumoto T., Ishida H., Kangawa K., Minamino N., Matsuo H., Ueda M., Hanaoka M., Hirose T. Primary structure and expression from complementary DNA of skeletal muscle ryanodine receptor. Nature. 1989 Jun 8;339(6224):439–445. doi: 10.1038/339439a0. [DOI] [PubMed] [Google Scholar]
- Tanabe T., Beam K. G., Adams B. A., Niidome T., Numa S. Regions of the skeletal muscle dihydropyridine receptor critical for excitation-contraction coupling. Nature. 1990 Aug 9;346(6284):567–569. doi: 10.1038/346567a0. [DOI] [PubMed] [Google Scholar]
- Tanabe T., Takeshima H., Mikami A., Flockerzi V., Takahashi H., Kangawa K., Kojima M., Matsuo H., Hirose T., Numa S. Primary structure of the receptor for calcium channel blockers from skeletal muscle. Nature. 1987 Jul 23;328(6128):313–318. doi: 10.1038/328313a0. [DOI] [PubMed] [Google Scholar]
- Tsien R. Y. New calcium indicators and buffers with high selectivity against magnesium and protons: design, synthesis, and properties of prototype structures. Biochemistry. 1980 May 27;19(11):2396–2404. doi: 10.1021/bi00552a018. [DOI] [PubMed] [Google Scholar]