Abstract
1. Mechanical properties of permeabilized single fibres from rabbit psoas and soleus muscle were determined by measuring the length responses due to abrupt changes in load and the force responses due to isovelocity length changes at different phosphate and Ca2+ concentrations. 2. The length responses due to abrupt increases in load from psoas fibres showed a rapid lengthening during the change in load followed by a phase of lengthening during which the velocity gradually decreased. In soleus fibres an abrupt lengthening during the change in load was followed by a phase of lengthening during which the velocity remained constant or decreased slightly for increases in load to less than 1.45 of the isometric force (F0). For larger increases in load the velocity during this later phase first increased and thereafter decreased. 3. The initial force-velocity curve, derived from the early part of the isotonic responses after the change in load, as well as the late force-velocity curve derived from the force level attained during isovelocity length changes, were sensitive to phosphate. Phosphate caused a shift of the absolute force-velocity curves of both psoas and soleus fibres towards lower values of force. In psoas fibres, the relative force-velocity curves derived by normalization of the force level to the force developed isometrically was shifted by phosphate to smaller velocities. In soleus fibres, the initial velocity at low and intermediate relative loads (less than 1.75 F0) was increased by phosphate but at higher loads it decreased, while the late force-velocity curve showed an overall decrease in velocity. 4. The force responses during isovelocity lengthening of psoas fibres showed an early rapid increase in force followed by a slow rise in force. The position of this break point in force was sensitive to the phosphate concentration. In soleus fibres, the force responses without phosphate showed an overshoot followed by a slow rise in force. The overshoot diminished with increasing phosphate concentration. 5. Phosphate and Ca2+ affected the force responses in psoas and soleus fibres in different ways. When the isometric starting levels were the same, force during and after the length change at submaximal activation was always less than at maximal activation in the presence of 15 mM-phosphate. 6. The changes in the mechanical performance during lengthening caused by phosphate in psoas as well as in soleus fibres, are in agreement with a decrease in the average force per attached crossbridge.(ABSTRACT TRUNCATED AT 400 WORDS)
Full text
PDF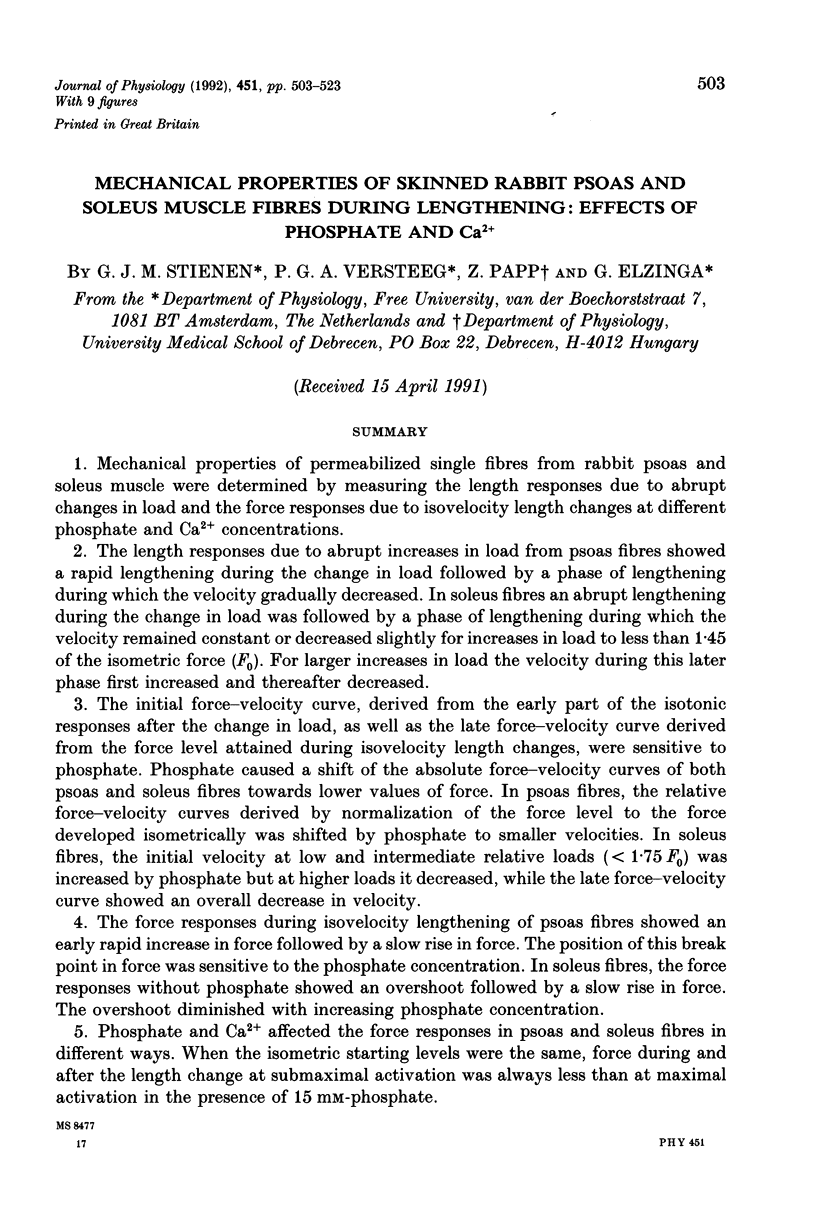
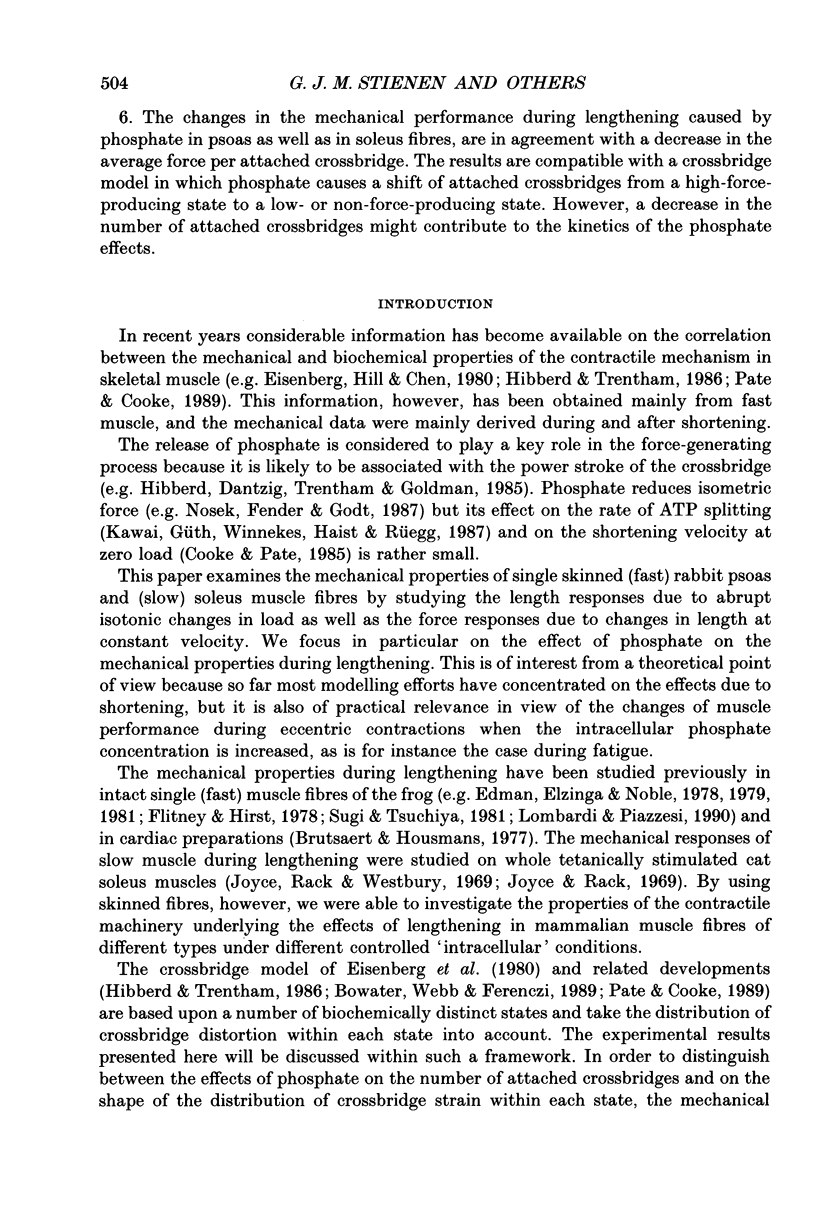
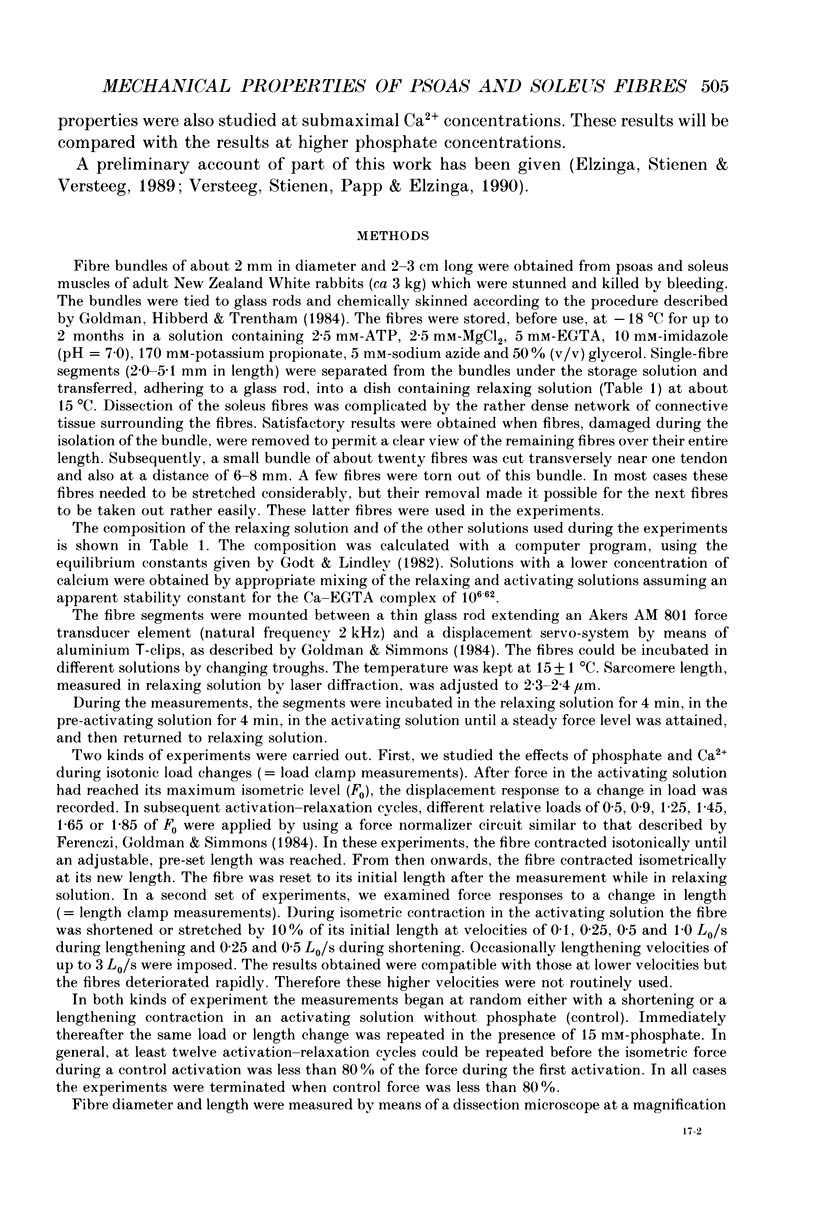
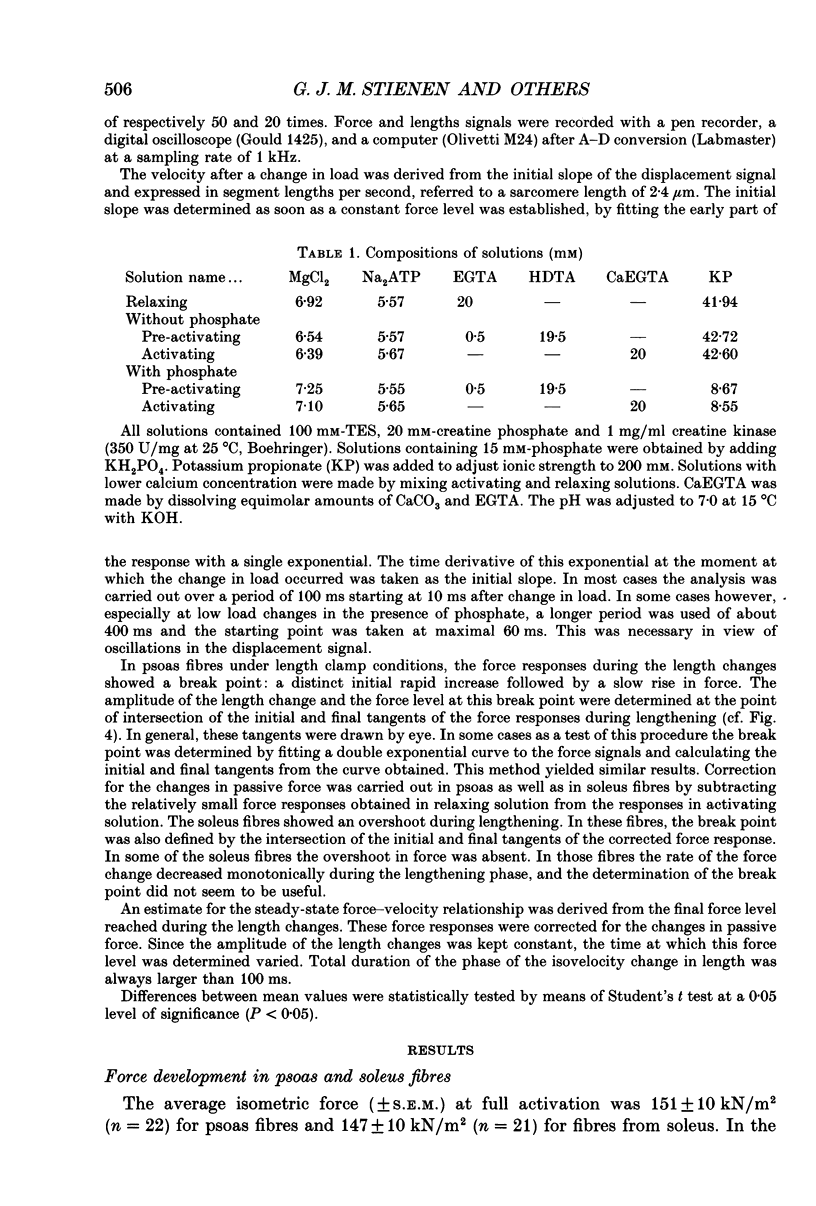
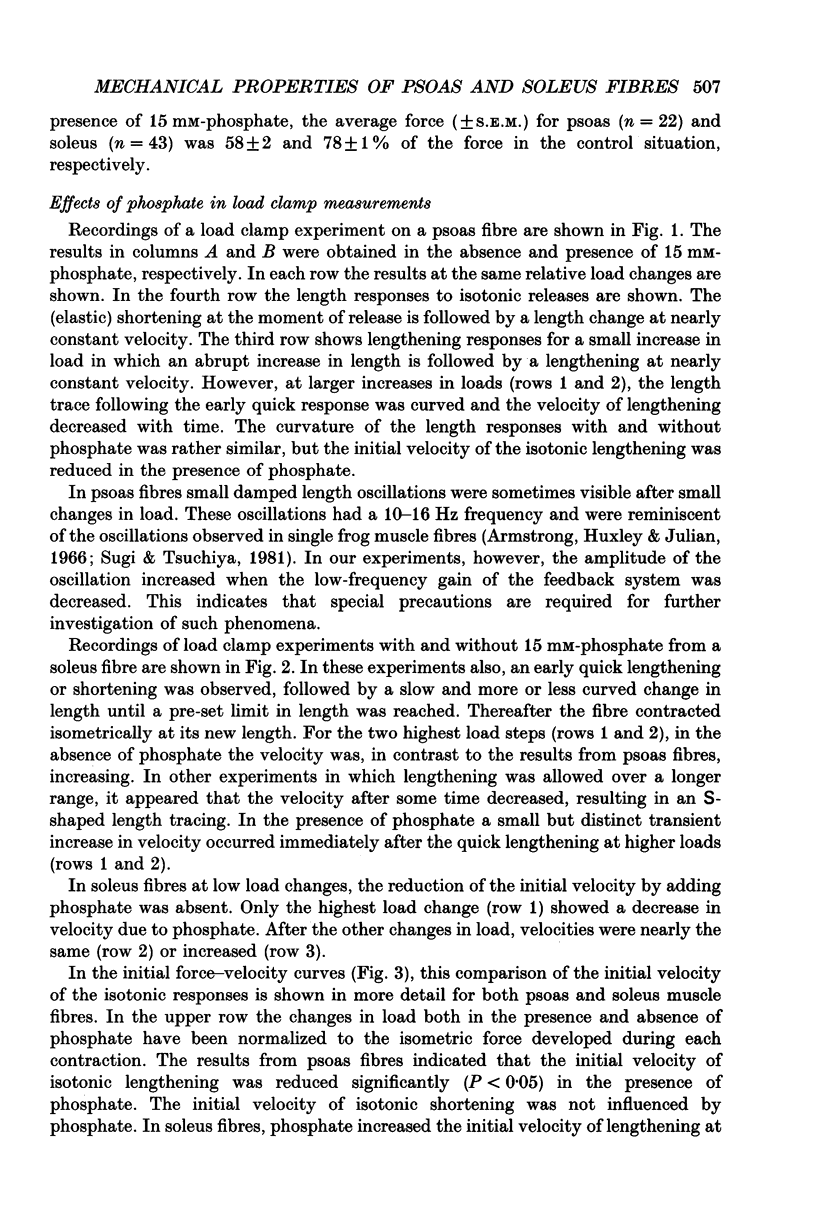
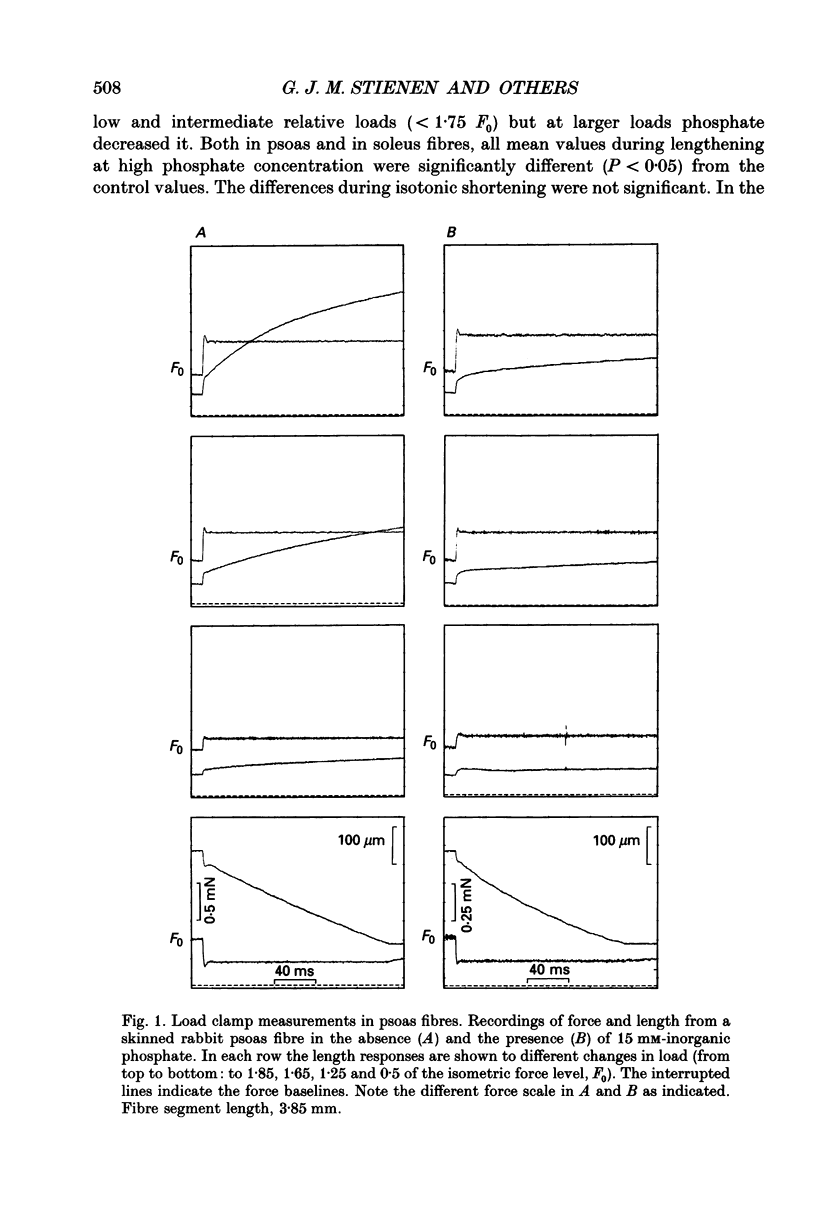
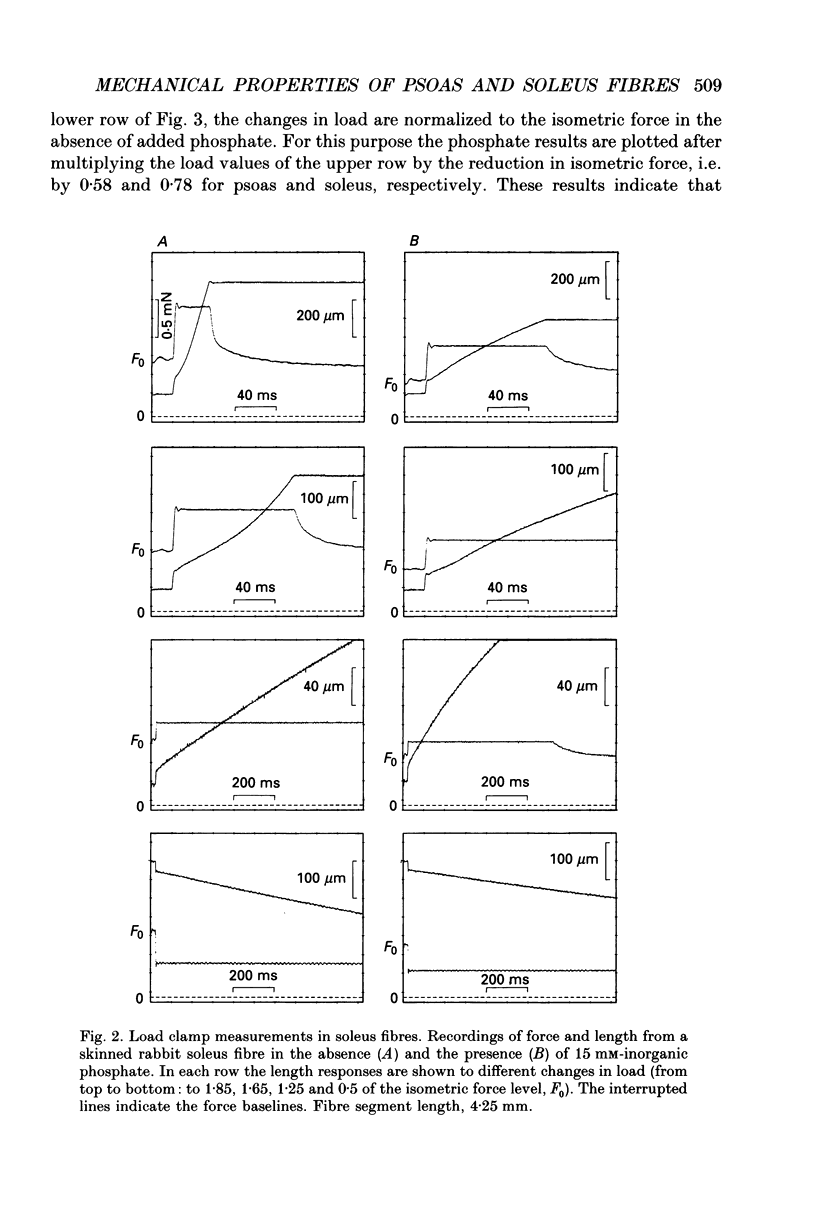
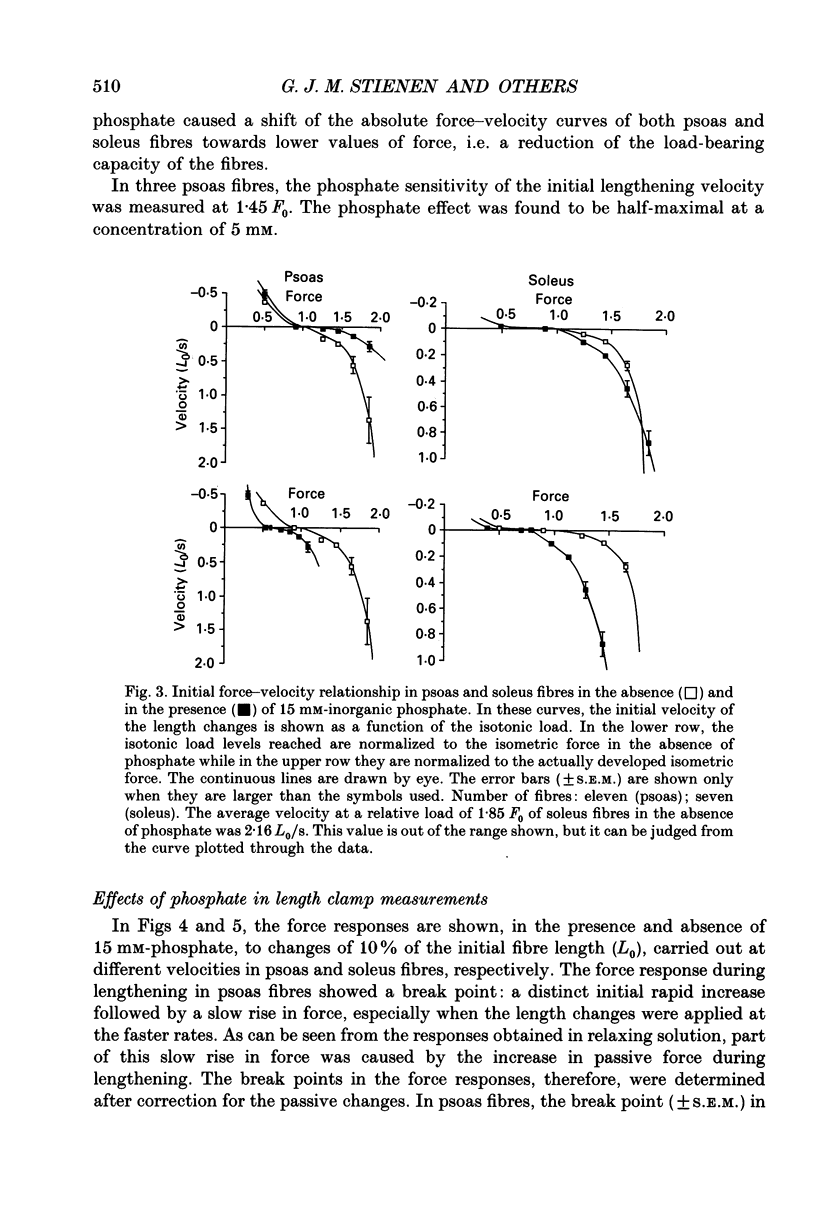
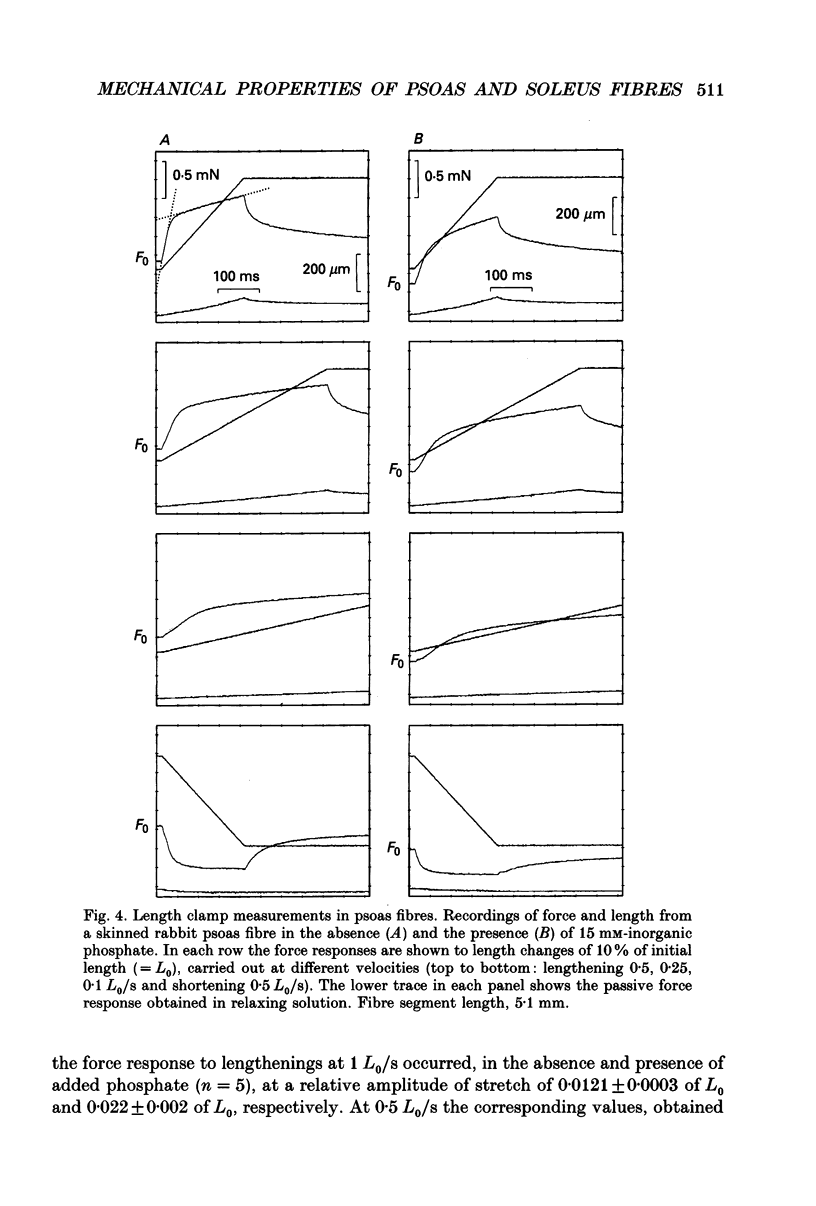
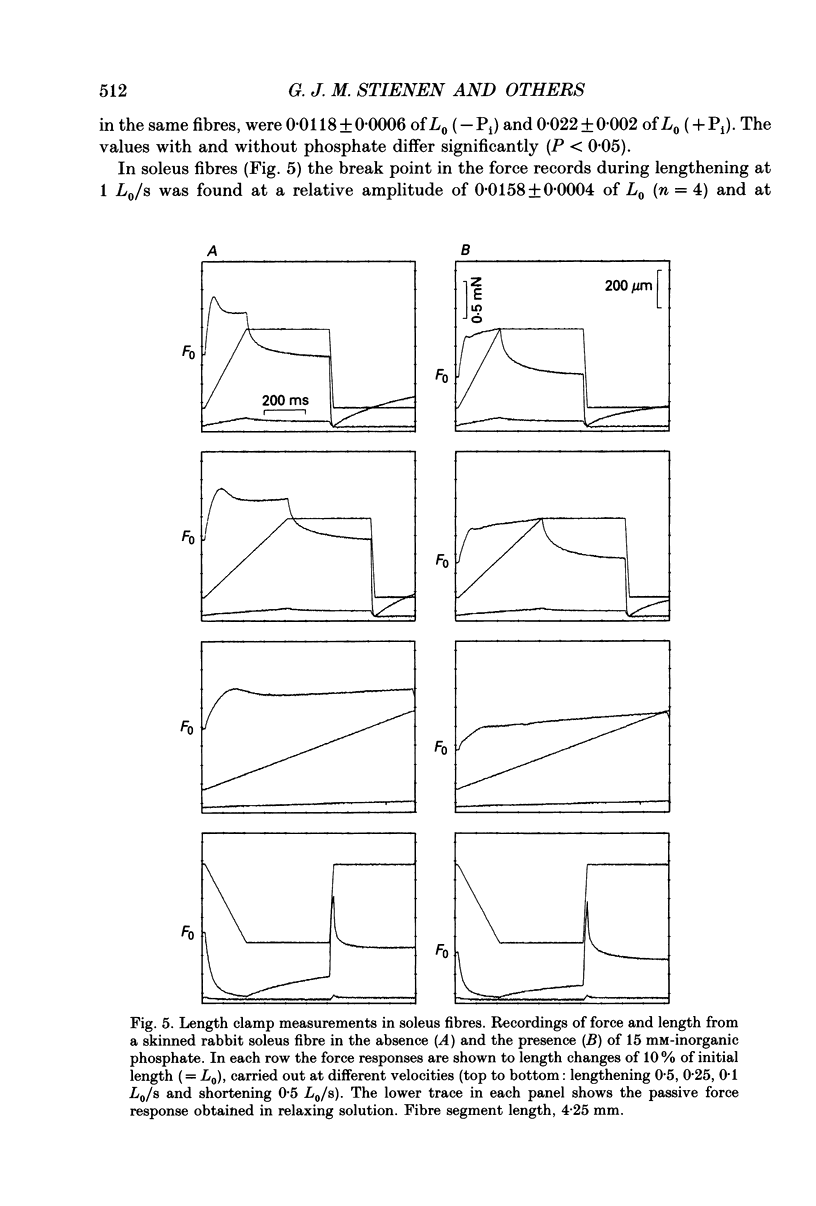
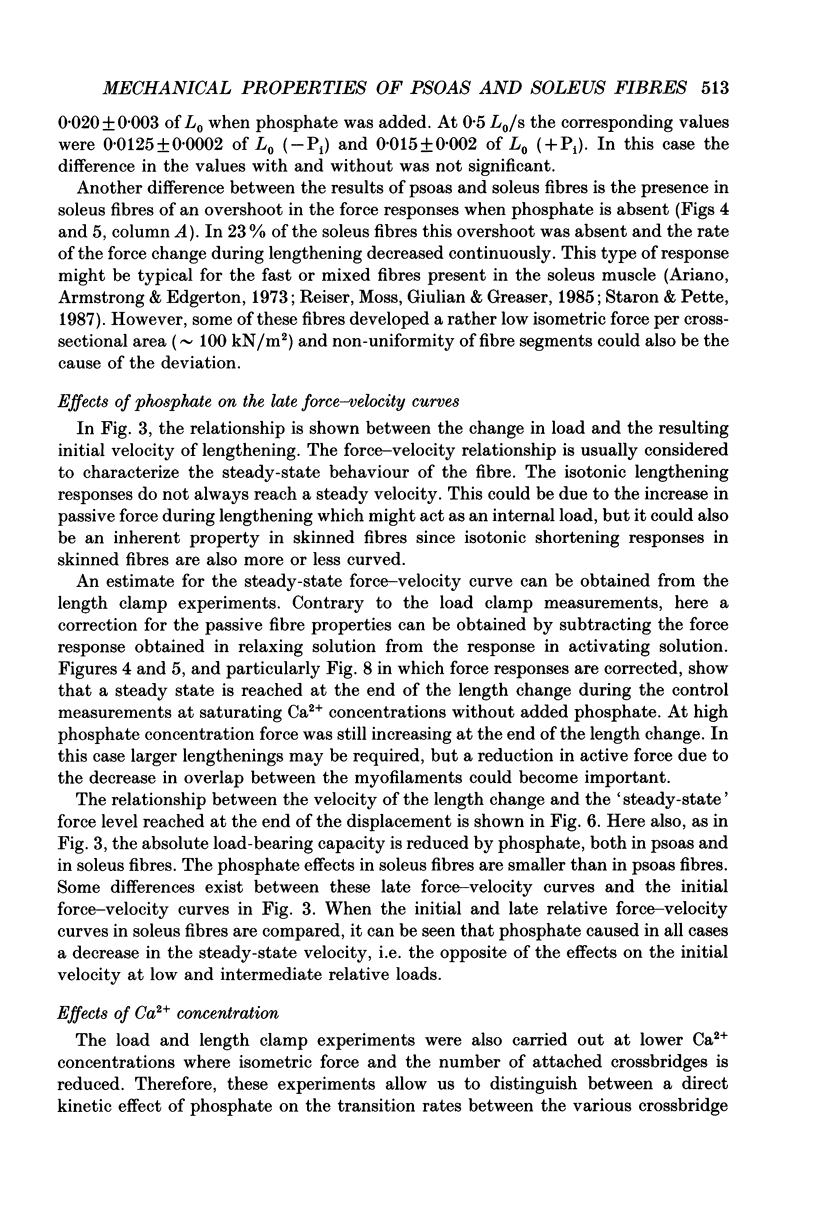
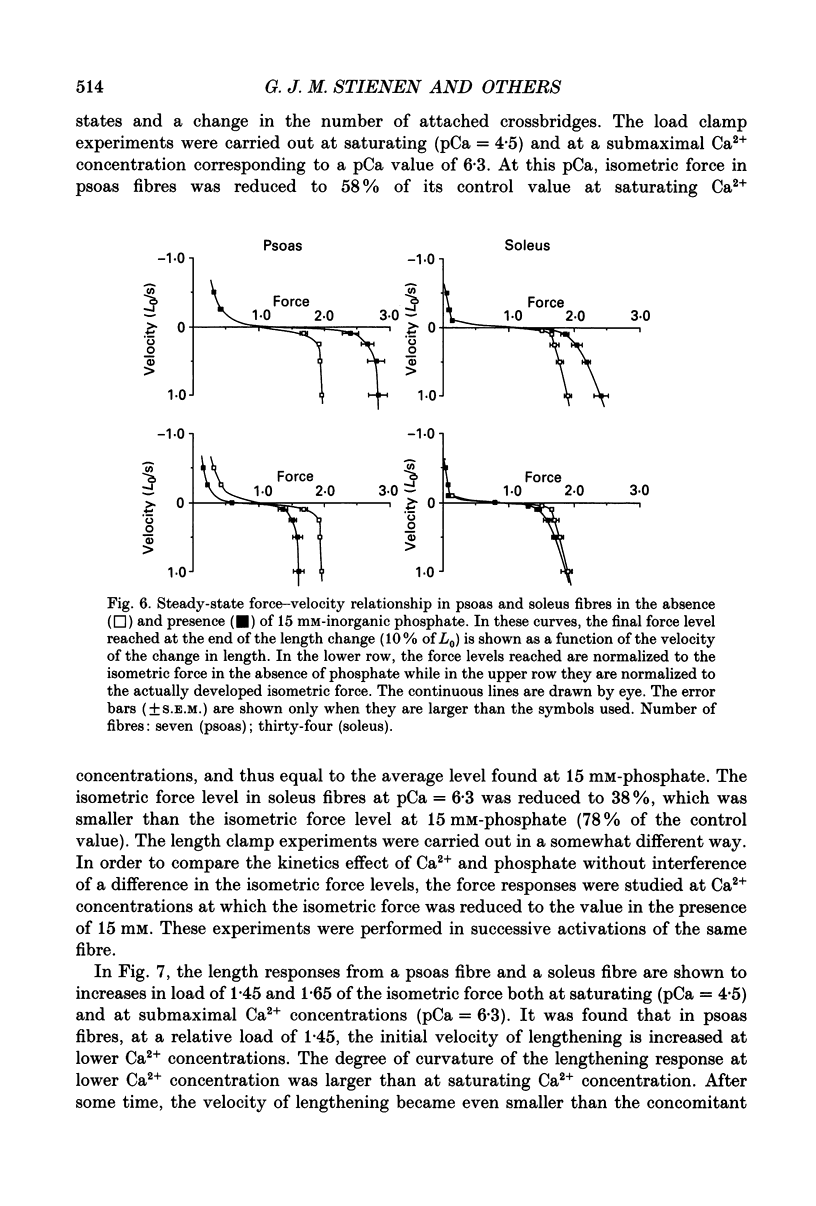
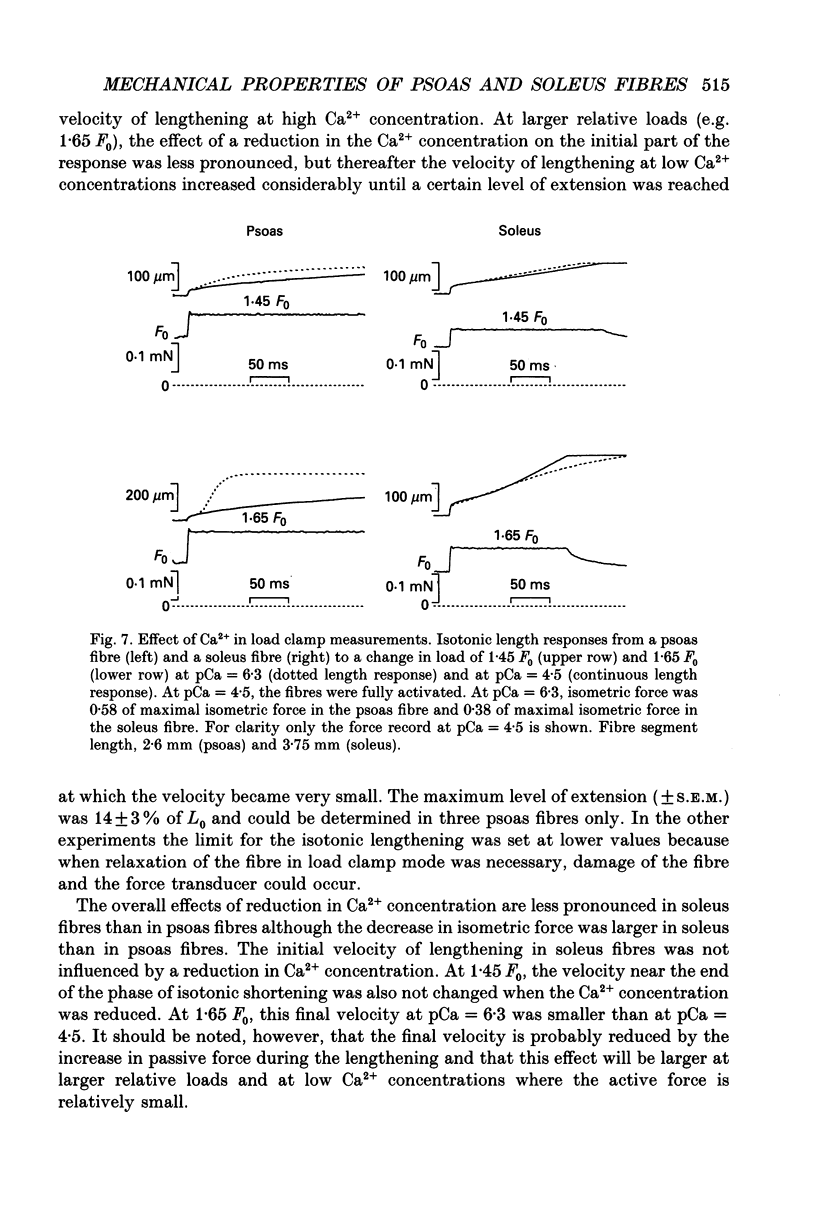
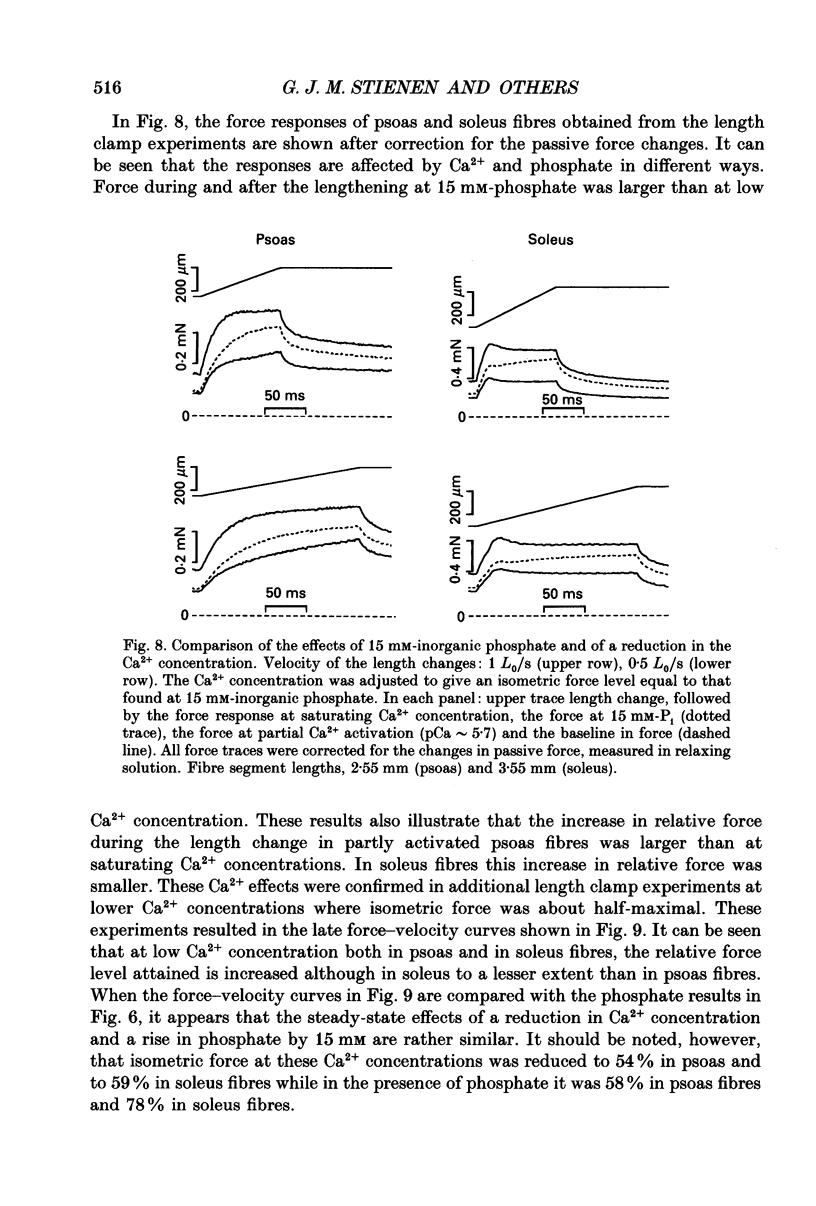
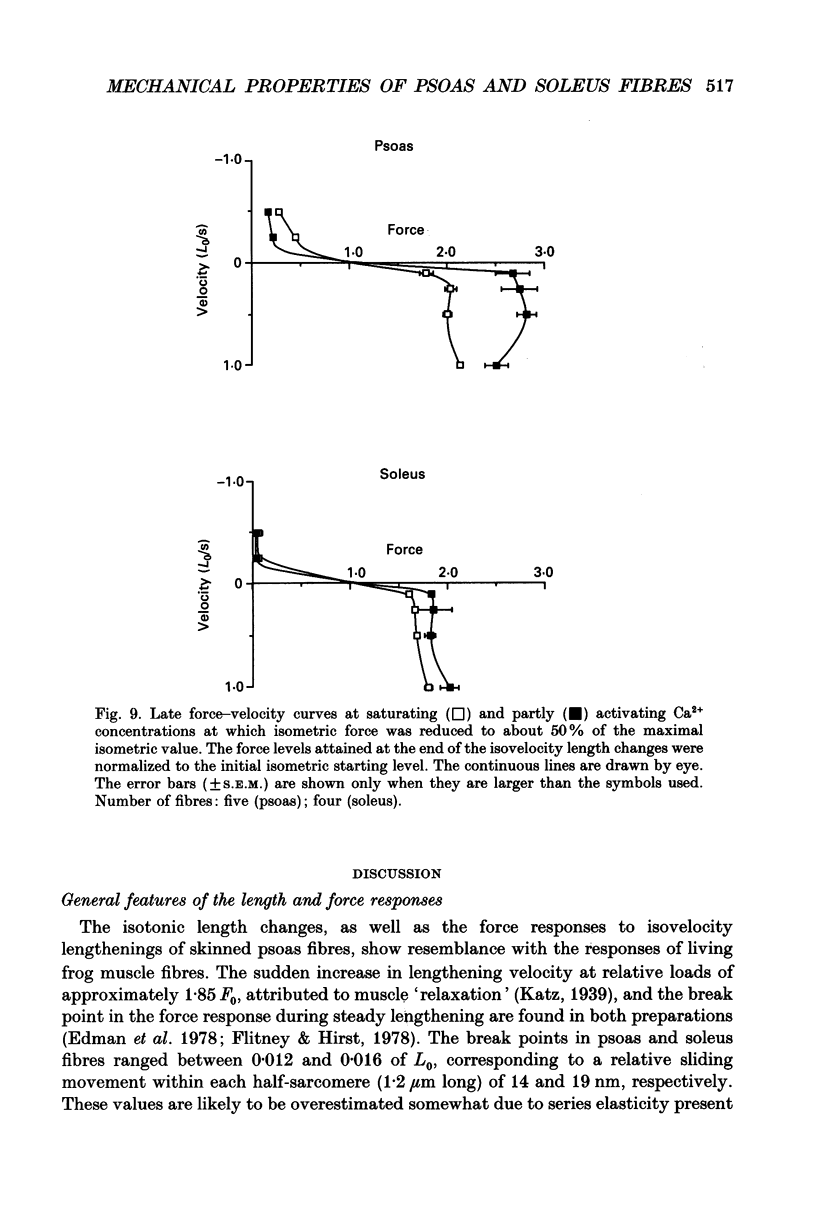
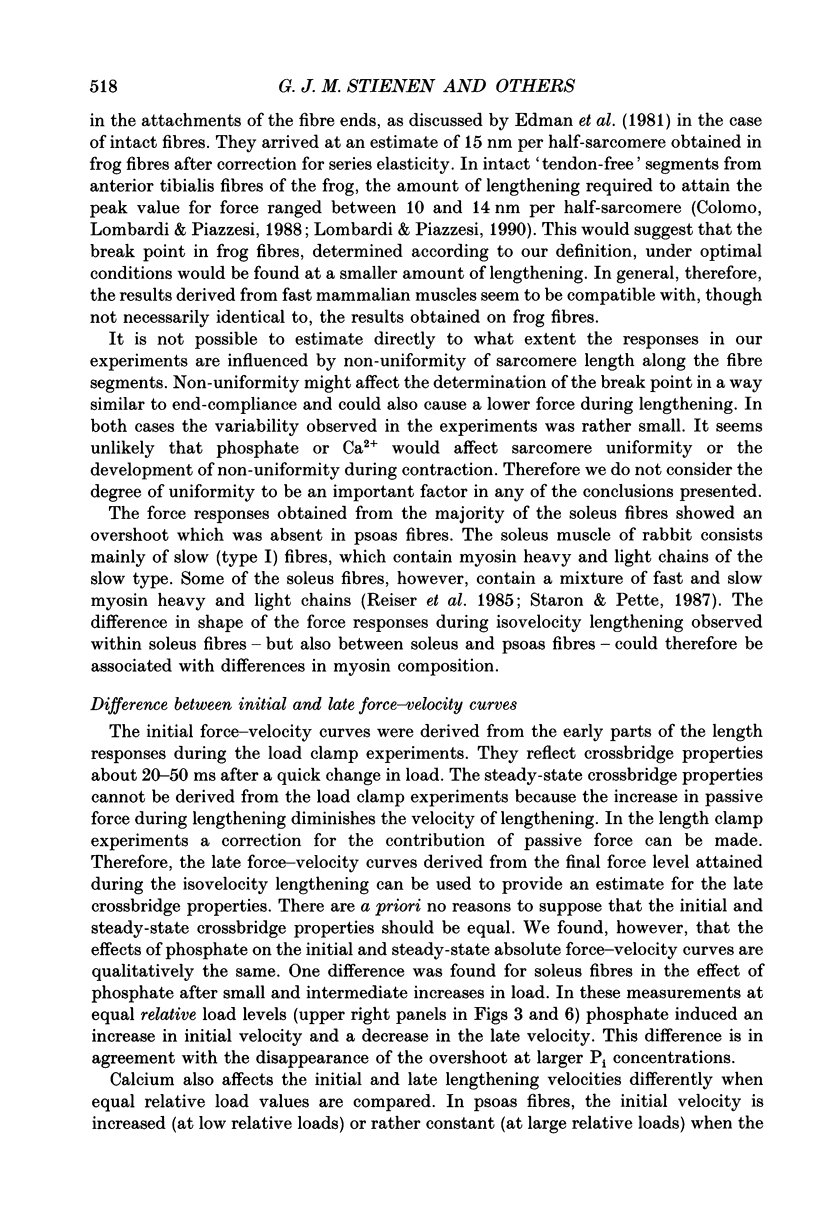
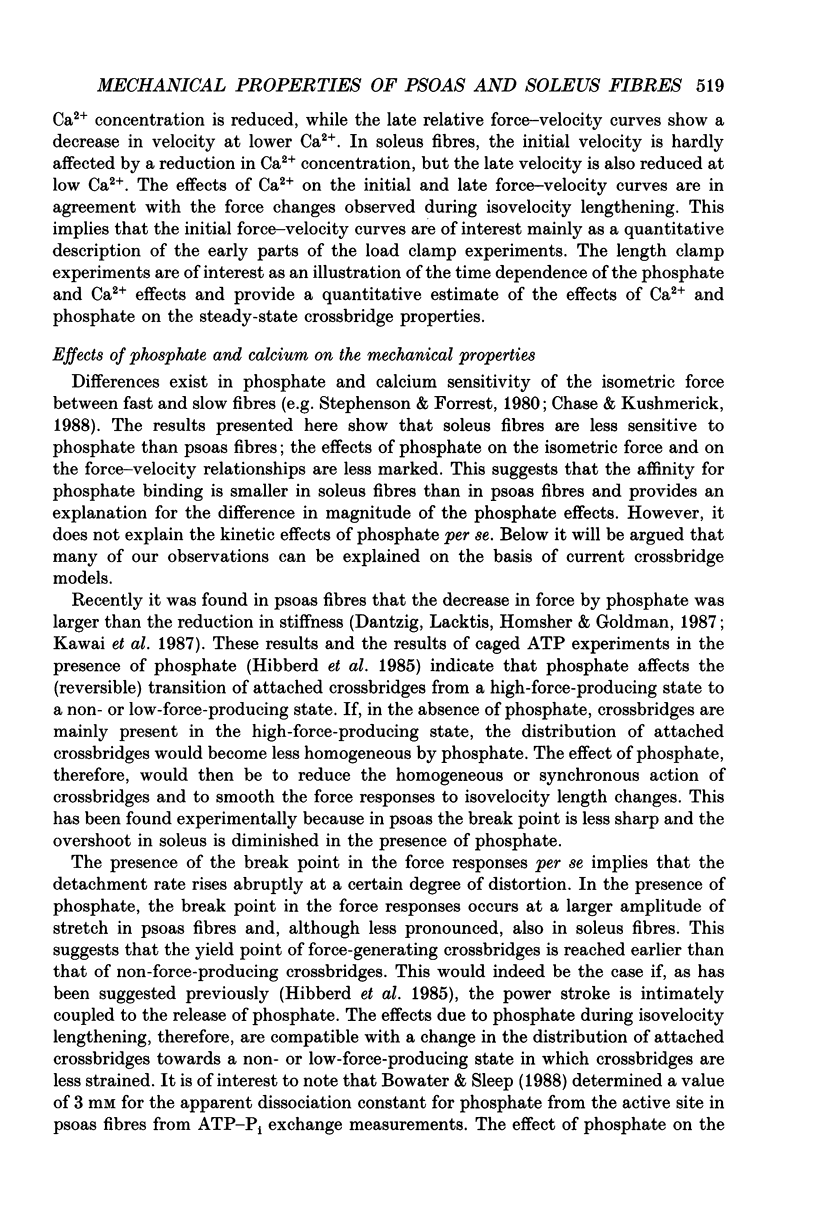
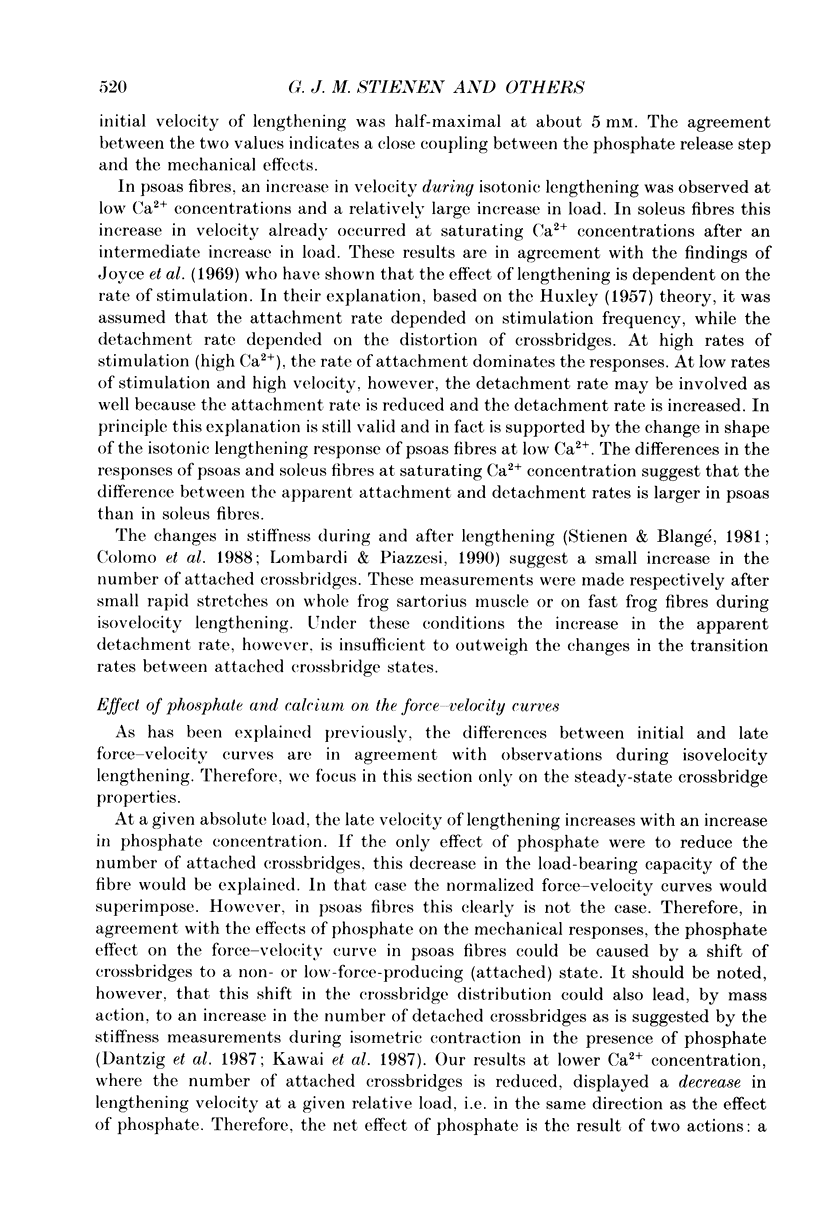
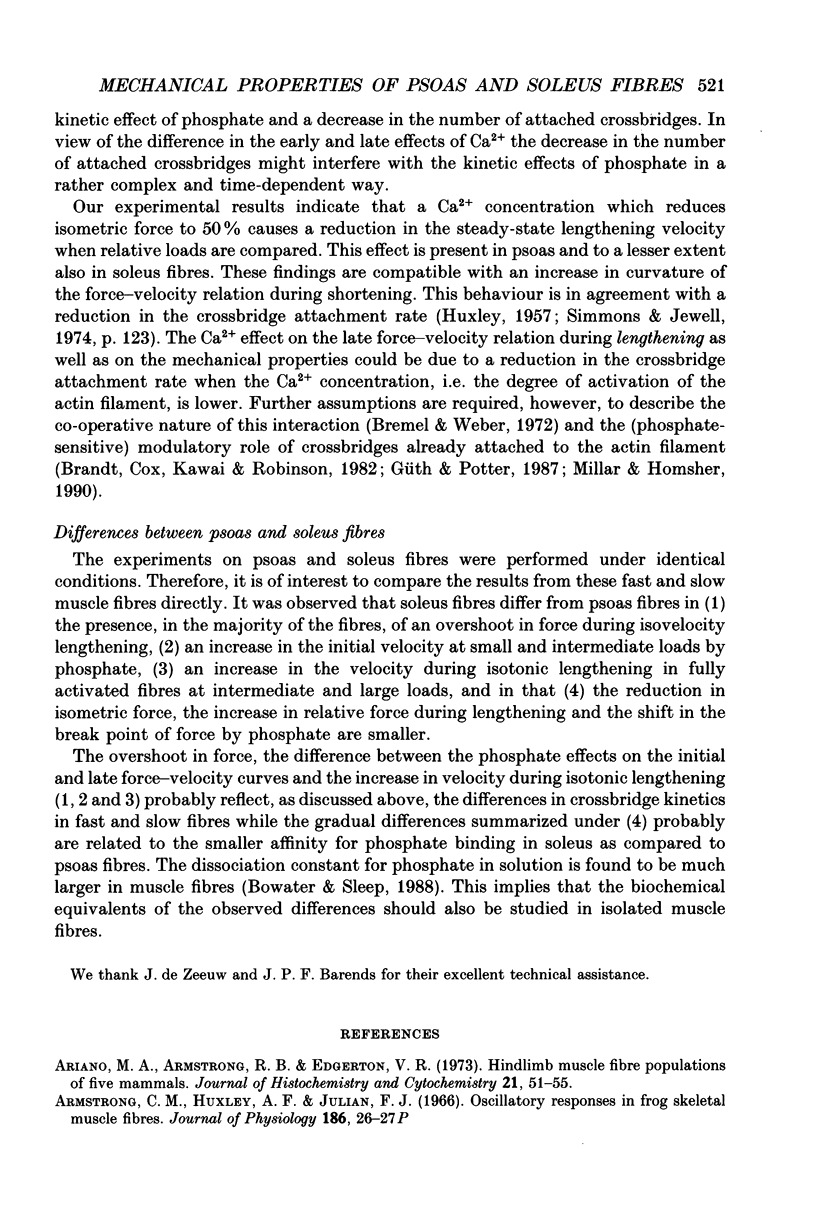
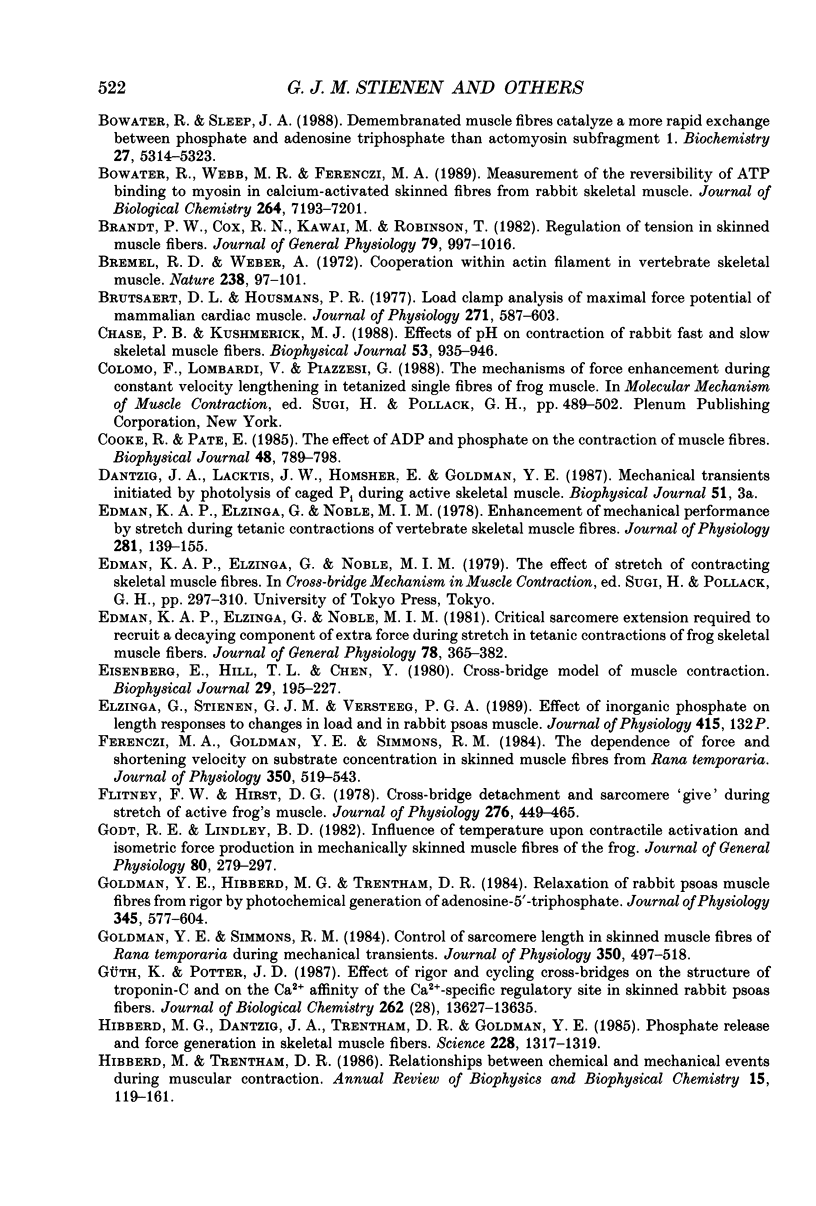
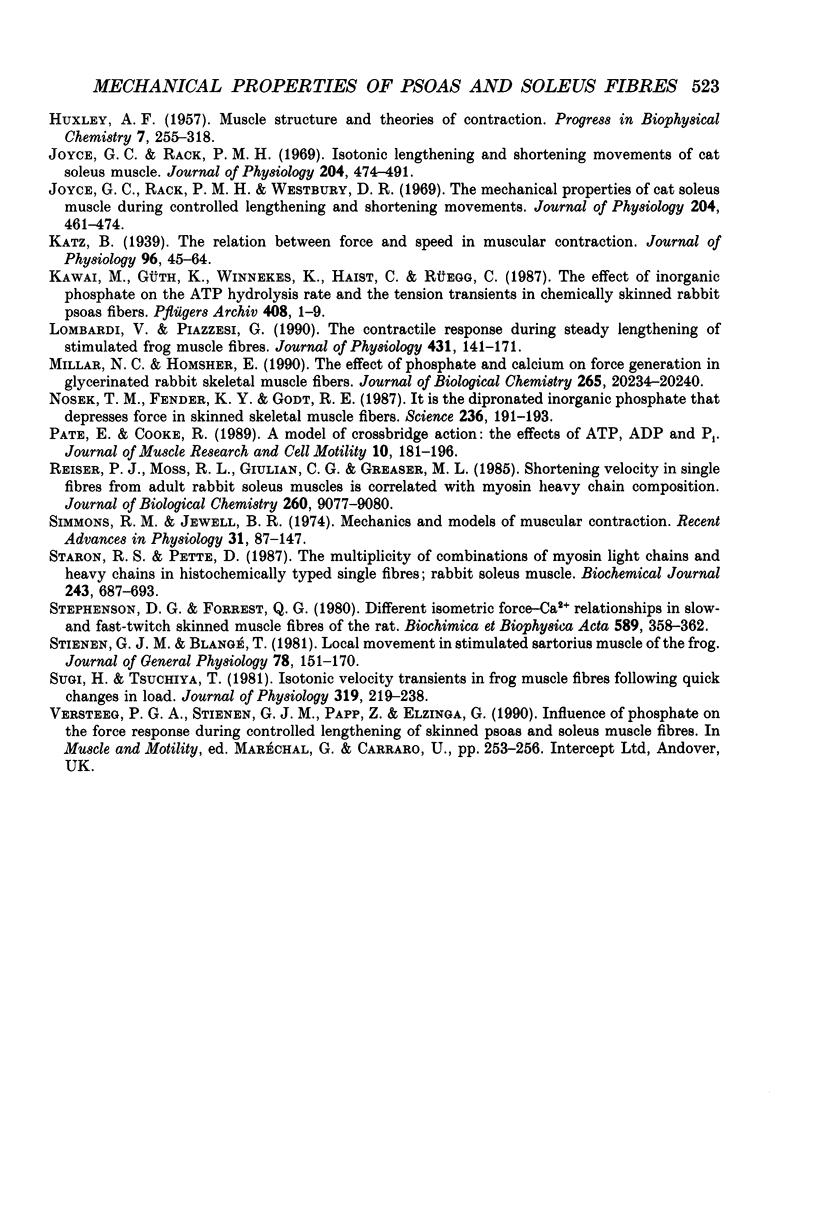
Selected References
These references are in PubMed. This may not be the complete list of references from this article.
- Ariano M. A., Armstrong R. B., Edgerton V. R. Hindlimb muscle fiber populations of five mammals. J Histochem Cytochem. 1973 Jan;21(1):51–55. doi: 10.1177/21.1.51. [DOI] [PubMed] [Google Scholar]
- Bowater R., Sleep J. Demembranated muscle fibers catalyze a more rapid exchange between phosphate and adenosine triphosphate than actomyosin subfragment 1. Biochemistry. 1988 Jul 12;27(14):5314–5323. doi: 10.1021/bi00414a055. [DOI] [PubMed] [Google Scholar]
- Bowater R., Webb M. R., Ferenczi M. A. Measurement of the reversibility of ATP binding to myosin in calcium-activated skinned fibers from rabbit skeletal muscle. Oxygen exchange between water and ATP released to the solution. J Biol Chem. 1989 May 5;264(13):7193–7201. [PubMed] [Google Scholar]
- Brandt P. W., Cox R. N., Kawai M., Robinson T. Effect of cross-bridge kinetics on apparent Ca2+ sensitivity. J Gen Physiol. 1982 Jun;79(6):997–1016. doi: 10.1085/jgp.79.6.997. [DOI] [PMC free article] [PubMed] [Google Scholar]
- Bremel R. D., Weber A. Cooperation within actin filament in vertebrate skeletal muscle. Nat New Biol. 1972 Jul 26;238(82):97–101. doi: 10.1038/newbio238097a0. [DOI] [PubMed] [Google Scholar]
- Brutsaert D. L., Housmans P. R. Load clamp analysis of maximal force potential of mammalian cardiac muscle. J Physiol. 1977 Oct;271(3):587–603. doi: 10.1113/jphysiol.1977.sp012016. [DOI] [PMC free article] [PubMed] [Google Scholar]
- Chase P. B., Kushmerick M. J. Effects of pH on contraction of rabbit fast and slow skeletal muscle fibers. Biophys J. 1988 Jun;53(6):935–946. doi: 10.1016/S0006-3495(88)83174-6. [DOI] [PMC free article] [PubMed] [Google Scholar]
- Colomo F., Lombardi V., Piazzesi G. The mechanisms of force enhancement during constant velocity lengthening in tetanized single fibres of frog muscle. Adv Exp Med Biol. 1988;226:489–502. [PubMed] [Google Scholar]
- Cooke R., Pate E. The effects of ADP and phosphate on the contraction of muscle fibers. Biophys J. 1985 Nov;48(5):789–798. doi: 10.1016/S0006-3495(85)83837-6. [DOI] [PMC free article] [PubMed] [Google Scholar]
- Edman K. A., Elzinga G., Noble M. I. Critical sarcomere extension required to recruit a decaying component of extra force during stretch in tetanic contractions of frog skeletal muscle fibers. J Gen Physiol. 1981 Oct;78(4):365–382. doi: 10.1085/jgp.78.4.365. [DOI] [PMC free article] [PubMed] [Google Scholar]
- Edman K. A., Elzinga G., Noble M. I. Enhancement of mechanical performance by stretch during tetanic contractions of vertebrate skeletal muscle fibres. J Physiol. 1978 Aug;281:139–155. doi: 10.1113/jphysiol.1978.sp012413. [DOI] [PMC free article] [PubMed] [Google Scholar]
- Eisenberg E., Hill T. L., Chen Y. Cross-bridge model of muscle contraction. Quantitative analysis. Biophys J. 1980 Feb;29(2):195–227. doi: 10.1016/S0006-3495(80)85126-5. [DOI] [PMC free article] [PubMed] [Google Scholar]
- Ferenczi M. A., Goldman Y. E., Simmons R. M. The dependence of force and shortening velocity on substrate concentration in skinned muscle fibres from Rana temporaria. J Physiol. 1984 May;350:519–543. doi: 10.1113/jphysiol.1984.sp015216. [DOI] [PMC free article] [PubMed] [Google Scholar]
- Flitney F. W., Hirst D. G. Cross-bridge detachment and sarcomere 'give' during stretch of active frog's muscle. J Physiol. 1978 Mar;276:449–465. doi: 10.1113/jphysiol.1978.sp012246. [DOI] [PMC free article] [PubMed] [Google Scholar]
- Godt R. E., Lindley B. D. Influence of temperature upon contractile activation and isometric force production in mechanically skinned muscle fibers of the frog. J Gen Physiol. 1982 Aug;80(2):279–297. doi: 10.1085/jgp.80.2.279. [DOI] [PMC free article] [PubMed] [Google Scholar]
- Goldman Y. E., Hibberd M. G., Trentham D. R. Relaxation of rabbit psoas muscle fibres from rigor by photochemical generation of adenosine-5'-triphosphate. J Physiol. 1984 Sep;354:577–604. doi: 10.1113/jphysiol.1984.sp015394. [DOI] [PMC free article] [PubMed] [Google Scholar]
- Goldman Y. E., Simmons R. M. Control of sarcomere length in skinned muscle fibres of Rana temporaria during mechanical transients. J Physiol. 1984 May;350:497–518. doi: 10.1113/jphysiol.1984.sp015215. [DOI] [PMC free article] [PubMed] [Google Scholar]
- Güth K., Potter J. D. Effect of rigor and cycling cross-bridges on the structure of troponin C and on the Ca2+ affinity of the Ca2+-specific regulatory sites in skinned rabbit psoas fibers. J Biol Chem. 1987 Oct 5;262(28):13627–13635. [PubMed] [Google Scholar]
- HUXLEY A. F. Muscle structure and theories of contraction. Prog Biophys Biophys Chem. 1957;7:255–318. [PubMed] [Google Scholar]
- Hibberd M. G., Dantzig J. A., Trentham D. R., Goldman Y. E. Phosphate release and force generation in skeletal muscle fibers. Science. 1985 Jun 14;228(4705):1317–1319. doi: 10.1126/science.3159090. [DOI] [PubMed] [Google Scholar]
- Hibberd M. G., Trentham D. R. Relationships between chemical and mechanical events during muscular contraction. Annu Rev Biophys Biophys Chem. 1986;15:119–161. doi: 10.1146/annurev.bb.15.060186.001003. [DOI] [PubMed] [Google Scholar]
- Joyce G. C., Rack P. M. Isotonic lengthening and shortening movements of cat soleus muscle. J Physiol. 1969 Oct;204(2):475–491. doi: 10.1113/jphysiol.1969.sp008925. [DOI] [PMC free article] [PubMed] [Google Scholar]
- Joyce G. C., Rack P. M., Westbury D. R. The mechanical properties of cat soleus muscle during controlled lengthening and shortening movements. J Physiol. 1969 Oct;204(2):461–474. doi: 10.1113/jphysiol.1969.sp008924. [DOI] [PMC free article] [PubMed] [Google Scholar]
- Katz B. The relation between force and speed in muscular contraction. J Physiol. 1939 Jun 14;96(1):45–64. doi: 10.1113/jphysiol.1939.sp003756. [DOI] [PMC free article] [PubMed] [Google Scholar]
- Lombardi V., Piazzesi G. The contractile response during steady lengthening of stimulated frog muscle fibres. J Physiol. 1990 Dec;431:141–171. doi: 10.1113/jphysiol.1990.sp018324. [DOI] [PMC free article] [PubMed] [Google Scholar]
- Millar N. C., Homsher E. The effect of phosphate and calcium on force generation in glycerinated rabbit skeletal muscle fibers. A steady-state and transient kinetic study. J Biol Chem. 1990 Nov 25;265(33):20234–20240. [PubMed] [Google Scholar]
- Nosek T. M., Fender K. Y., Godt R. E. It is diprotonated inorganic phosphate that depresses force in skinned skeletal muscle fibers. Science. 1987 Apr 10;236(4798):191–193. doi: 10.1126/science.3563496. [DOI] [PubMed] [Google Scholar]
- Pate E., Cooke R. A model of crossbridge action: the effects of ATP, ADP and Pi. J Muscle Res Cell Motil. 1989 Jun;10(3):181–196. doi: 10.1007/BF01739809. [DOI] [PubMed] [Google Scholar]
- Reiser P. J., Moss R. L., Giulian G. G., Greaser M. L. Shortening velocity in single fibers from adult rabbit soleus muscles is correlated with myosin heavy chain composition. J Biol Chem. 1985 Aug 5;260(16):9077–9080. [PubMed] [Google Scholar]
- Staron R. S., Pette D. The multiplicity of combinations of myosin light chains and heavy chains in histochemically typed single fibres. Rabbit soleus muscle. Biochem J. 1987 May 1;243(3):687–693. doi: 10.1042/bj2430687. [DOI] [PMC free article] [PubMed] [Google Scholar]
- Stephenson D. G., Forrest Q. G. Different isometric force - [Ca2+] relationships in slow and fast twitch skinned muscle fibres of the rat. Biochim Biophys Acta. 1980 Feb 8;589(2):358–362. doi: 10.1016/0005-2728(80)90052-3. [DOI] [PubMed] [Google Scholar]
- Stienen G. J., Blangé T. Local movement in stimulated frog sartorius muscle. J Gen Physiol. 1981 Aug;78(2):151–170. doi: 10.1085/jgp.78.2.151. [DOI] [PMC free article] [PubMed] [Google Scholar]
- Sugi H., Tsuchiya T. Isotonic velocity transients in frog muscle fibres following quick changes in load. J Physiol. 1981;319:219–238. doi: 10.1113/jphysiol.1981.sp013903. [DOI] [PMC free article] [PubMed] [Google Scholar]