Abstract
1. It is well known that anoxia induces an increase in extracellular K+. The underlying mechanisms for the increase, however, are not well understood. In the present study, we performed electrophysiological, pharmacological and receptor autoradiographic experiments in an attempt to examine K+ ionic homeostasis during anoxia. Ion-selective microelectrodes were employed to measure intracellular and extracellular K+ activity from hypoglossal neurons in brain slices. 2. During 3-4 min anoxia, adult hypoglossal neurons lose a large amount of their intracellular K+ and this contributes in a major way to the 8-fold increase in extracellular K+. 3. Loss of intracellular K+ from hypoglossal neurons is, to a great extent, due to activation of certain specific K+ channels. Glibenclamide, a potential sulphonylurea ligand and a specific blocker of ATP-sensitive K+ (KATP) channels, has no effect on K+ homeostasis during oxygenated states, but almost halves the anoxia-induced increase in extracellular K+ in the adult rat. 4. [3H]glibenclamide autoradiography shows that the hypoglossal nucleus in the adult rat has high sulphonylurea receptor density, a finding that is consistent with our electrophysiological observation. 5. Since we have previously shown that newborn mammals and reptiles are more resistant to O2 deprivation than adult mammals, we performed comparative studies among adult rat, newborn rat and adult turtle. In sharp contrast to the adult rat, extracellular K+ activity in newborn rat and adult turtle brain increases little (10 to 100 times less than the adult rat) and glibenclamide has a small and insignificant effect on K+ efflux in the newborn rat and none in the turtle. Glibenclamide receptor binding sites are much lower in the newborn rat than in the adult rat central nervous system (CNS) and barely detectable in the turtle brain. 6. These results support the hypothesis that in the adult rat, K+ is lost during anoxia from neurons through sulphonylurea receptor or KATP channels in a major way. Generally, however, KATP channels are poorly expressed in the newborn rat and adult turtle CNS and have little role to play during O2 deprivation.
Full text
PDF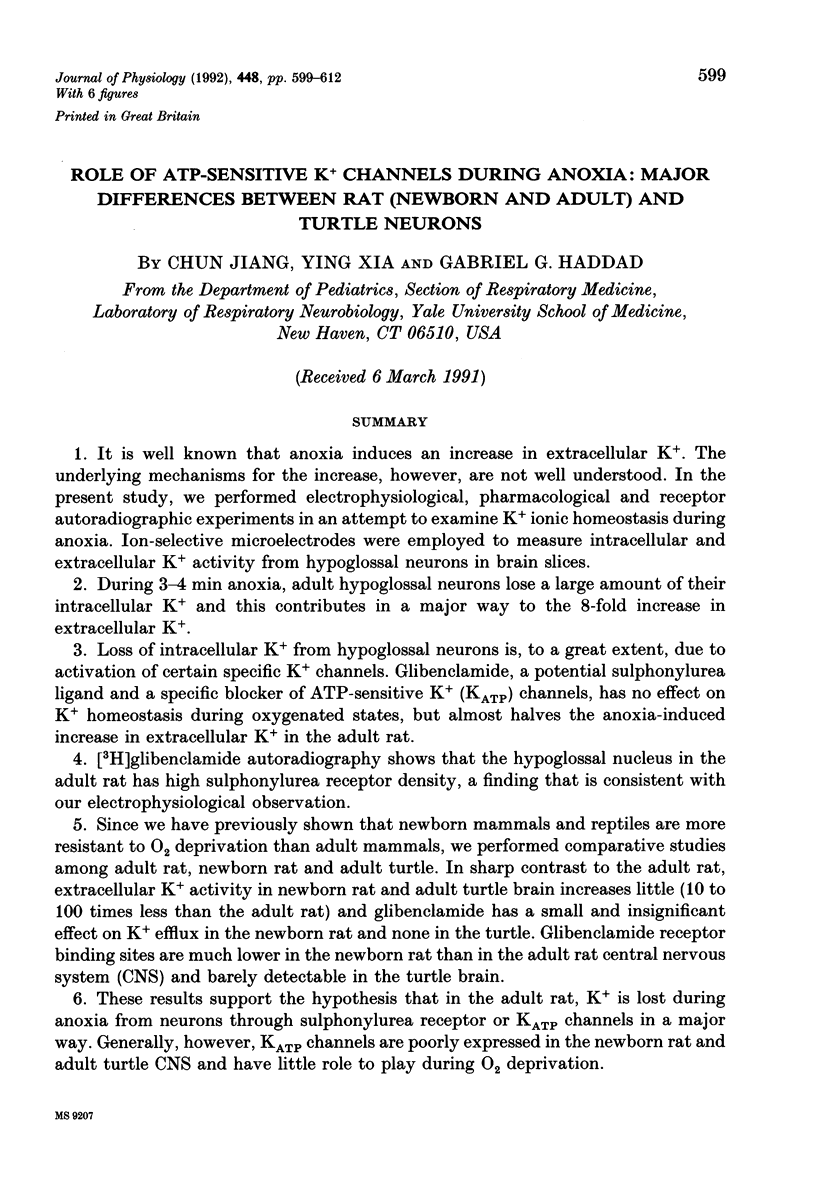
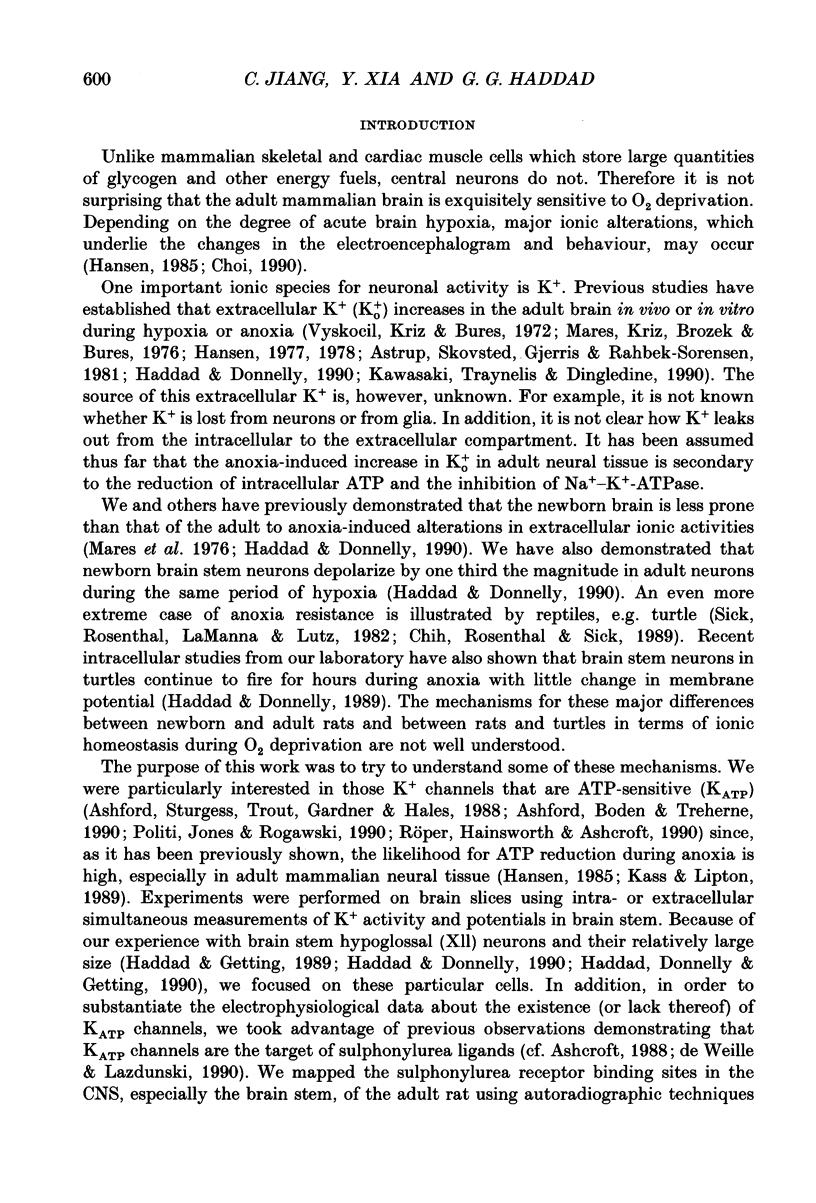
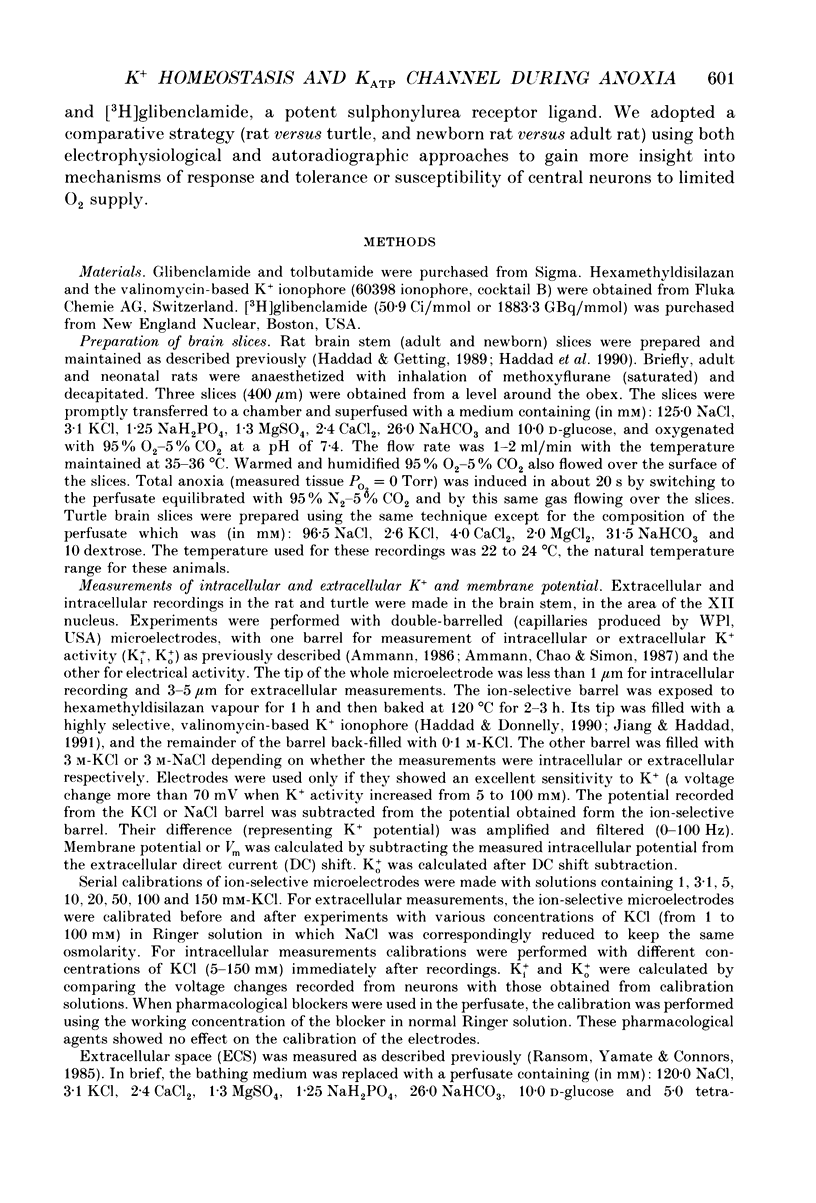
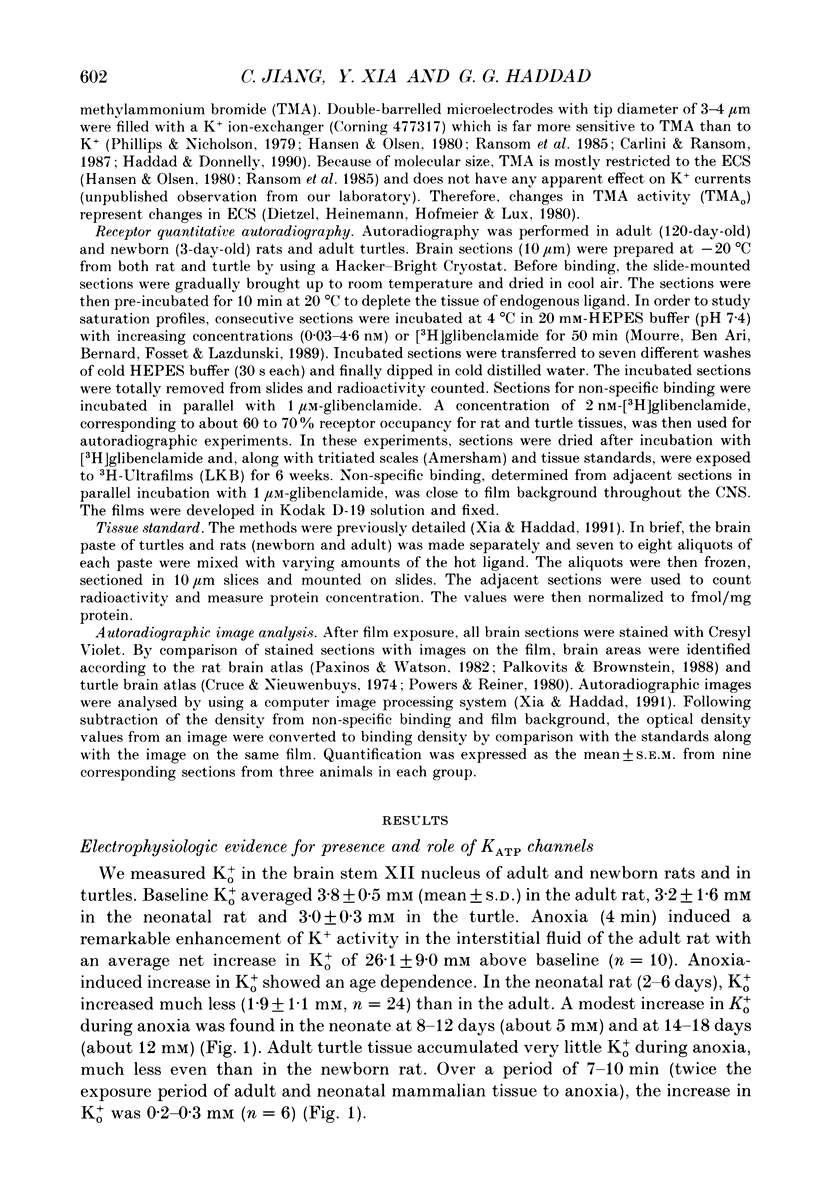
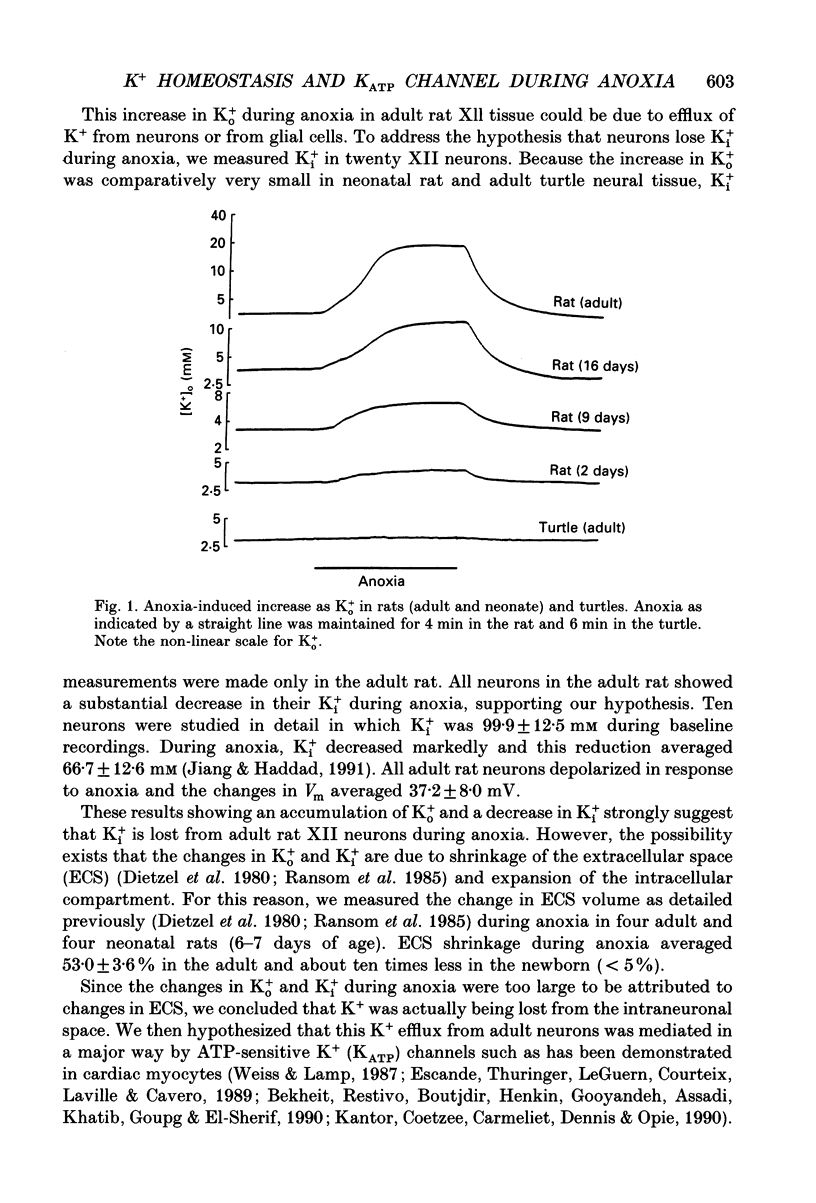
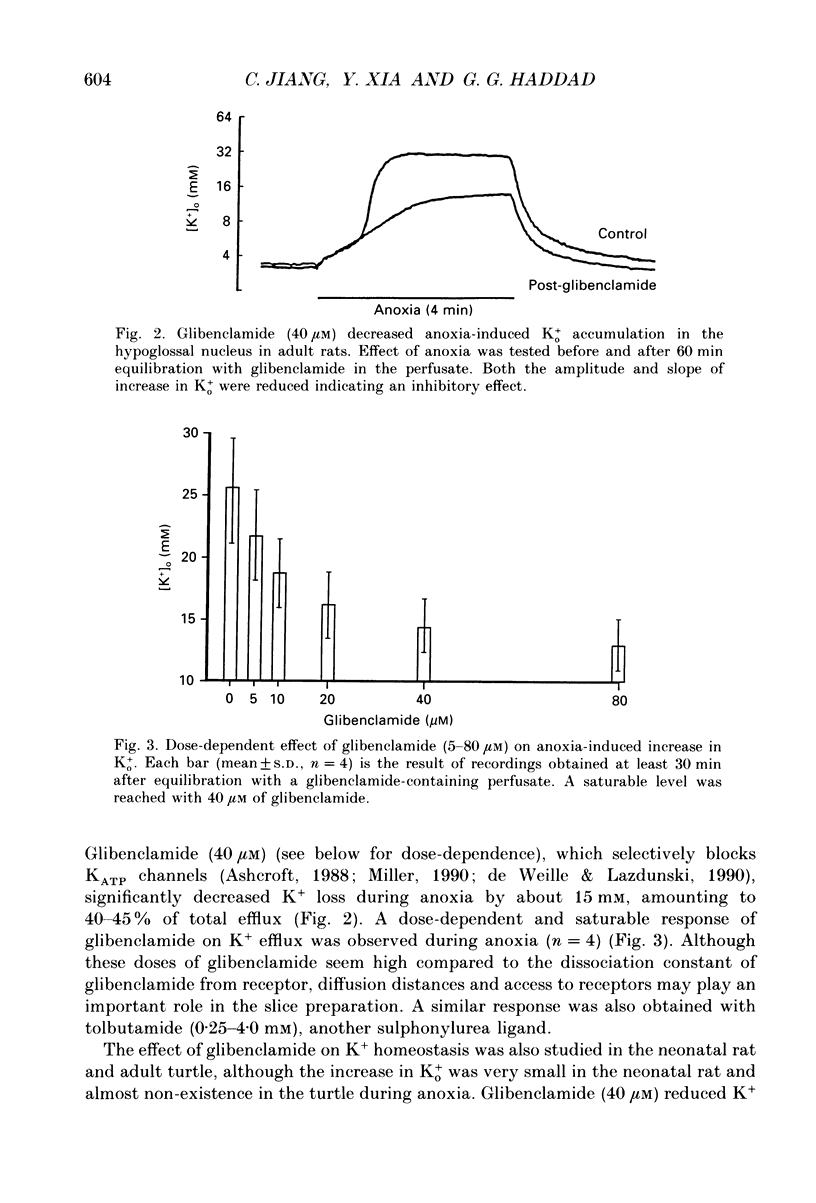
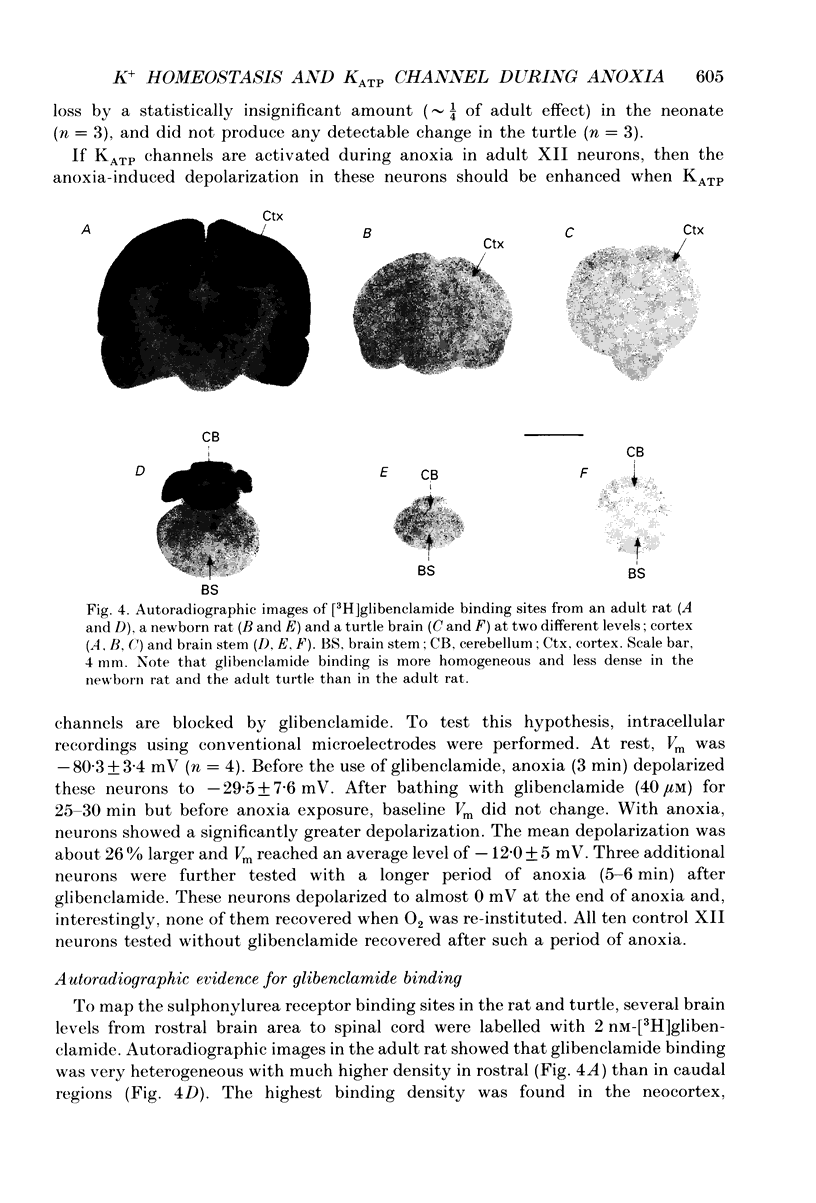
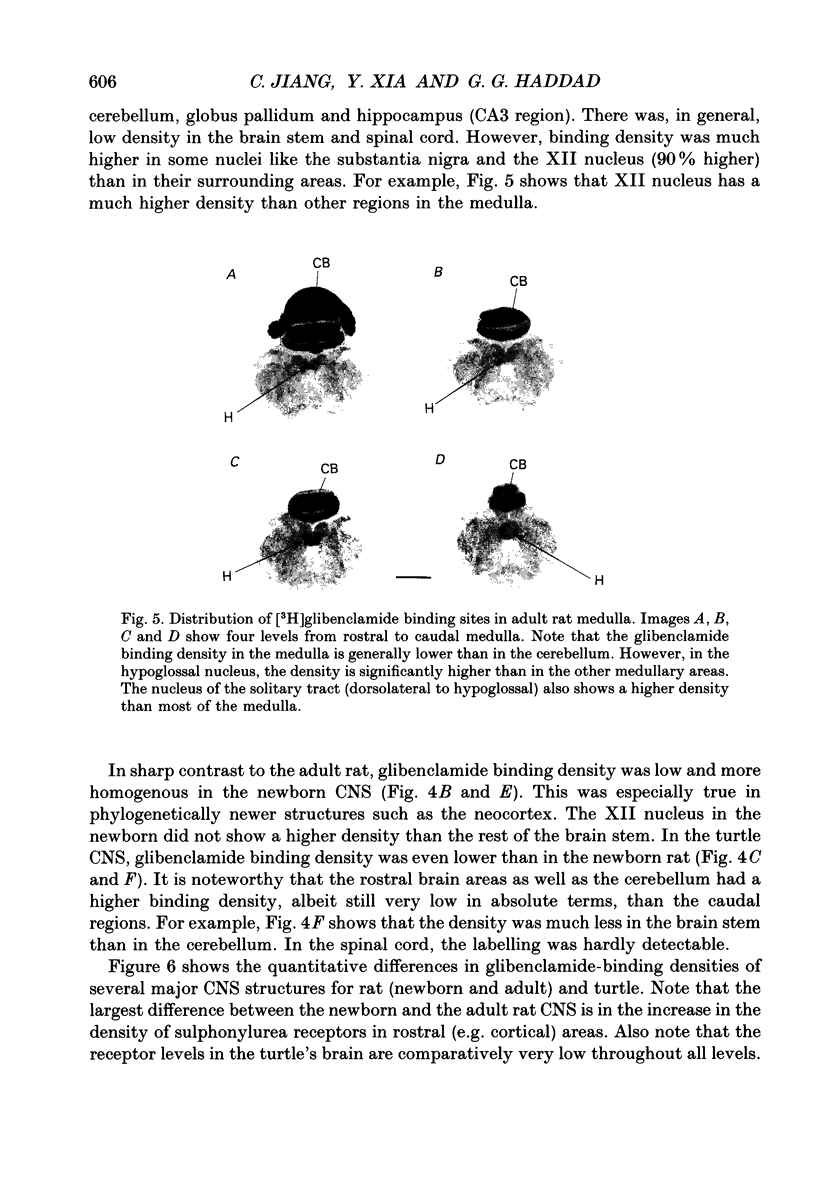
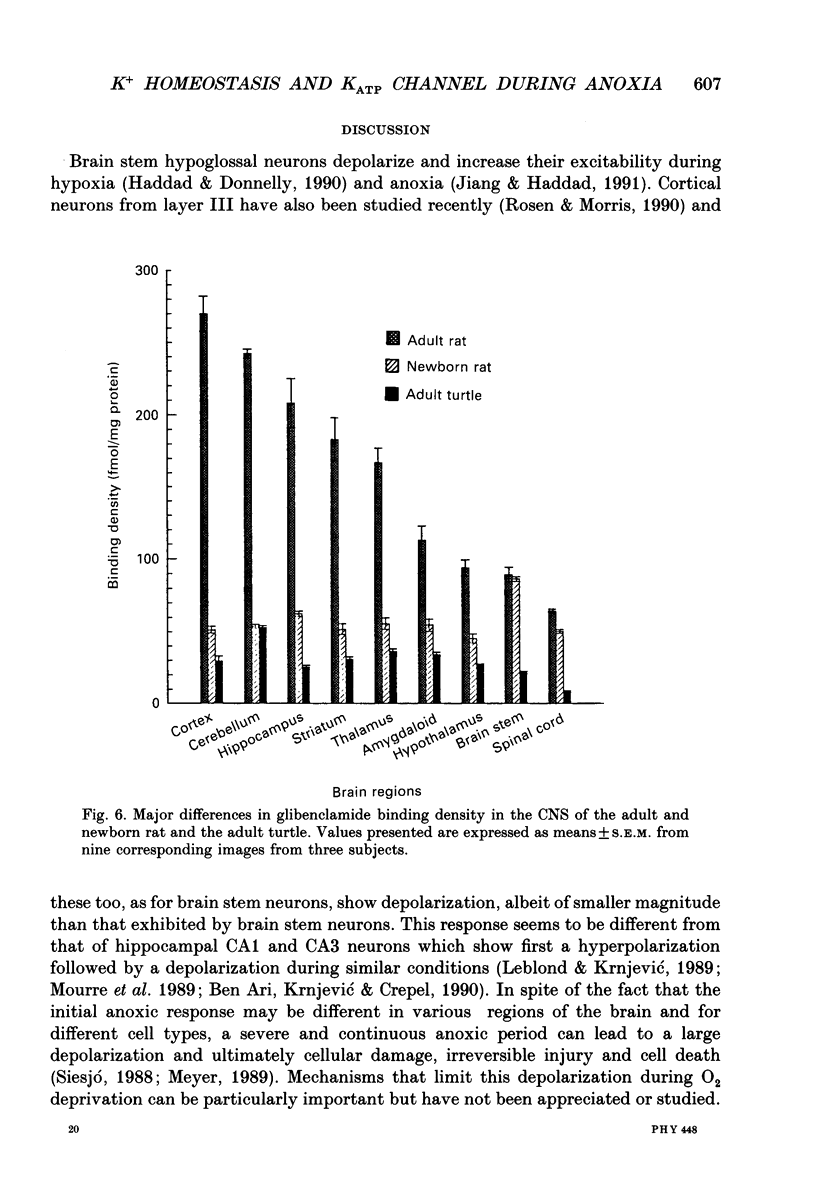
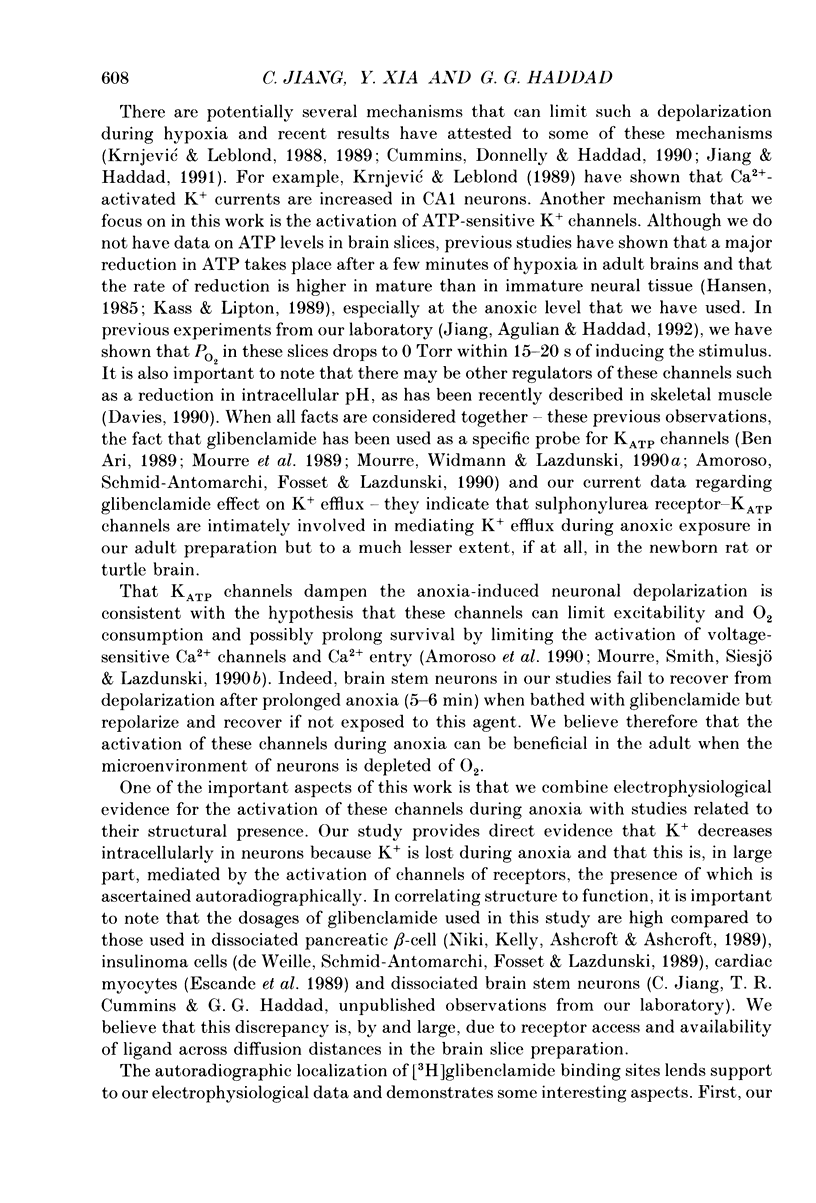
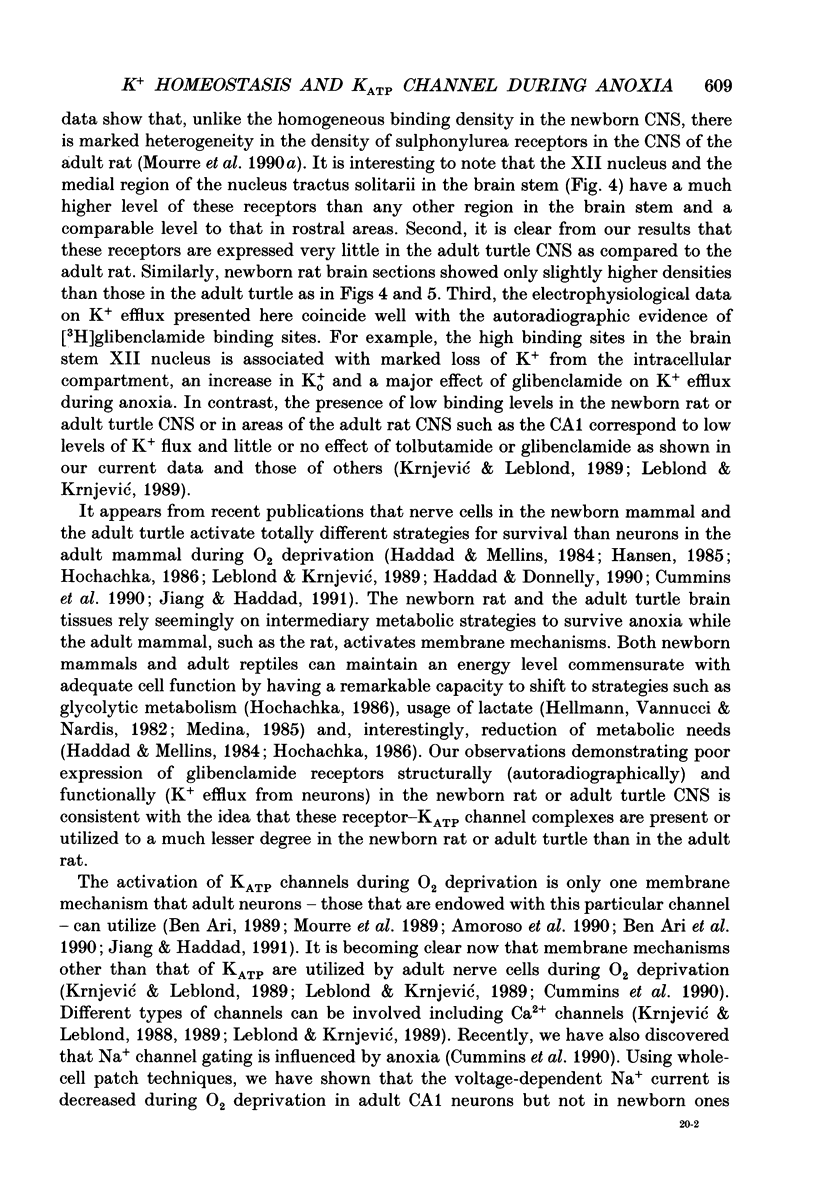
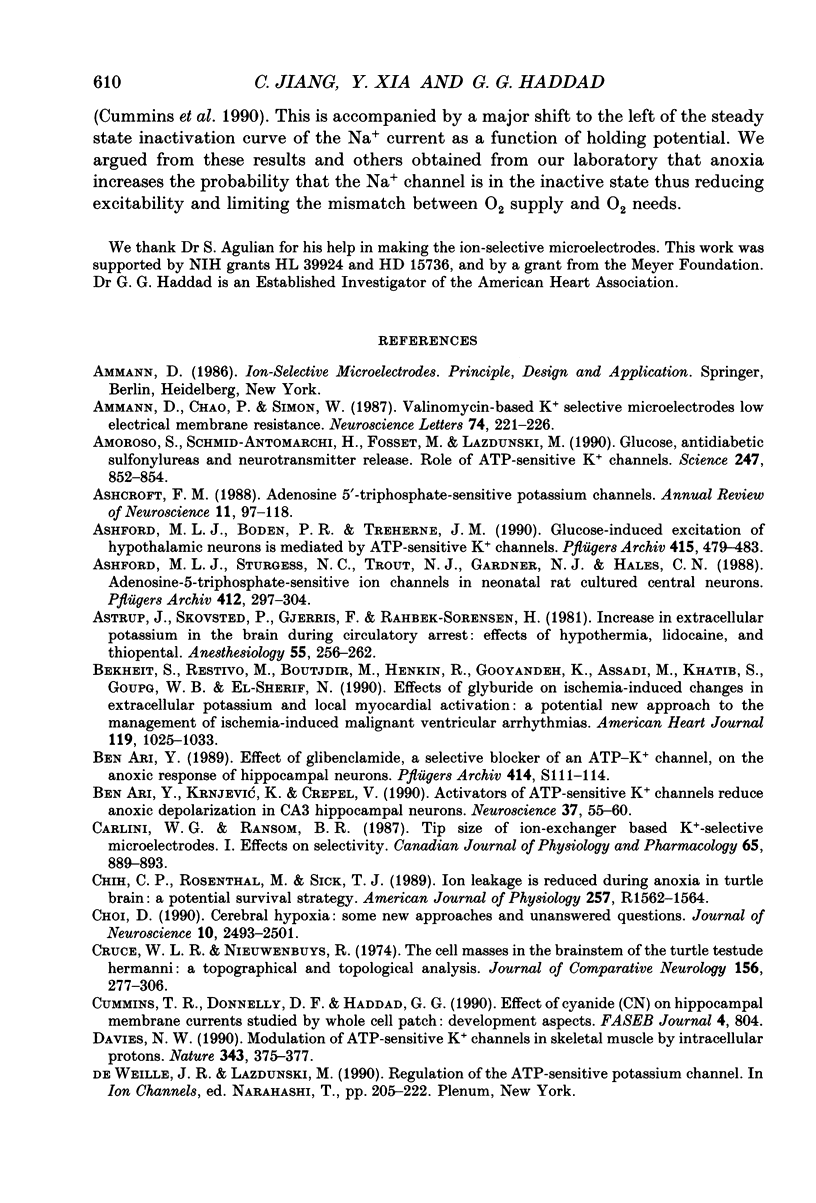
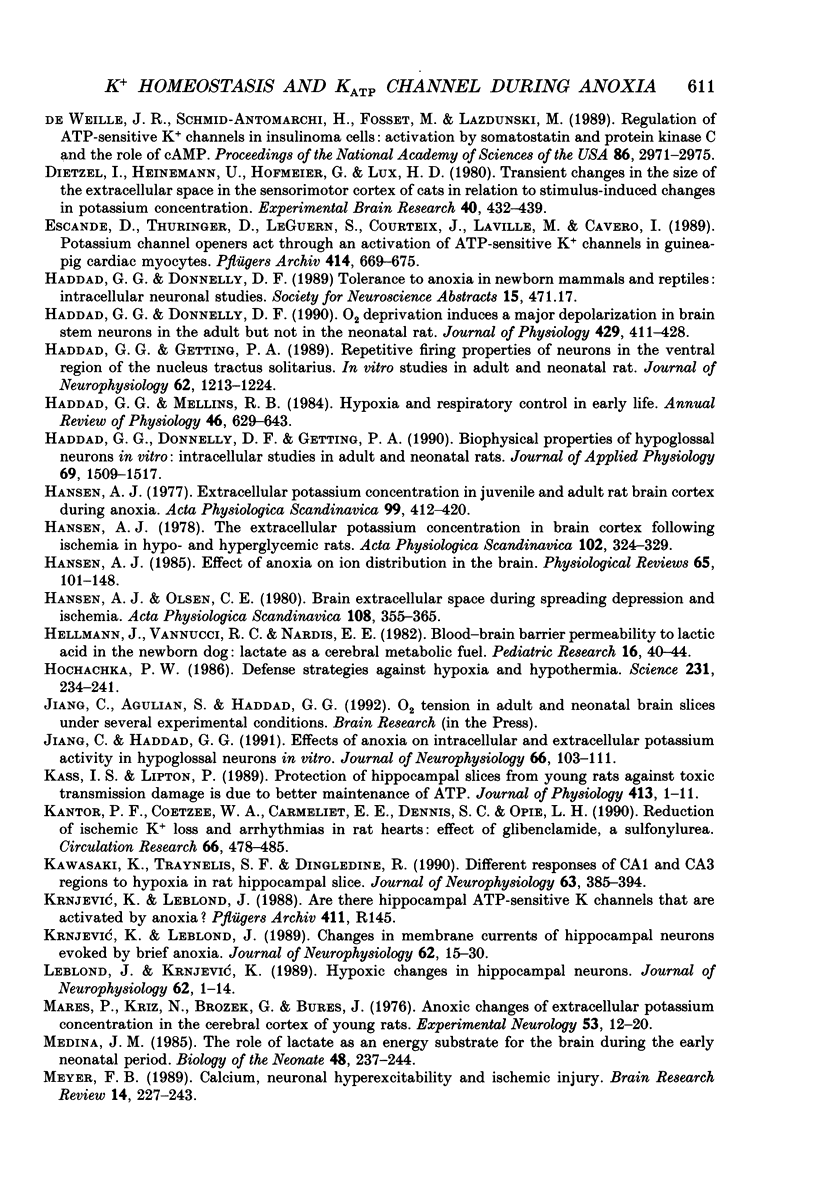
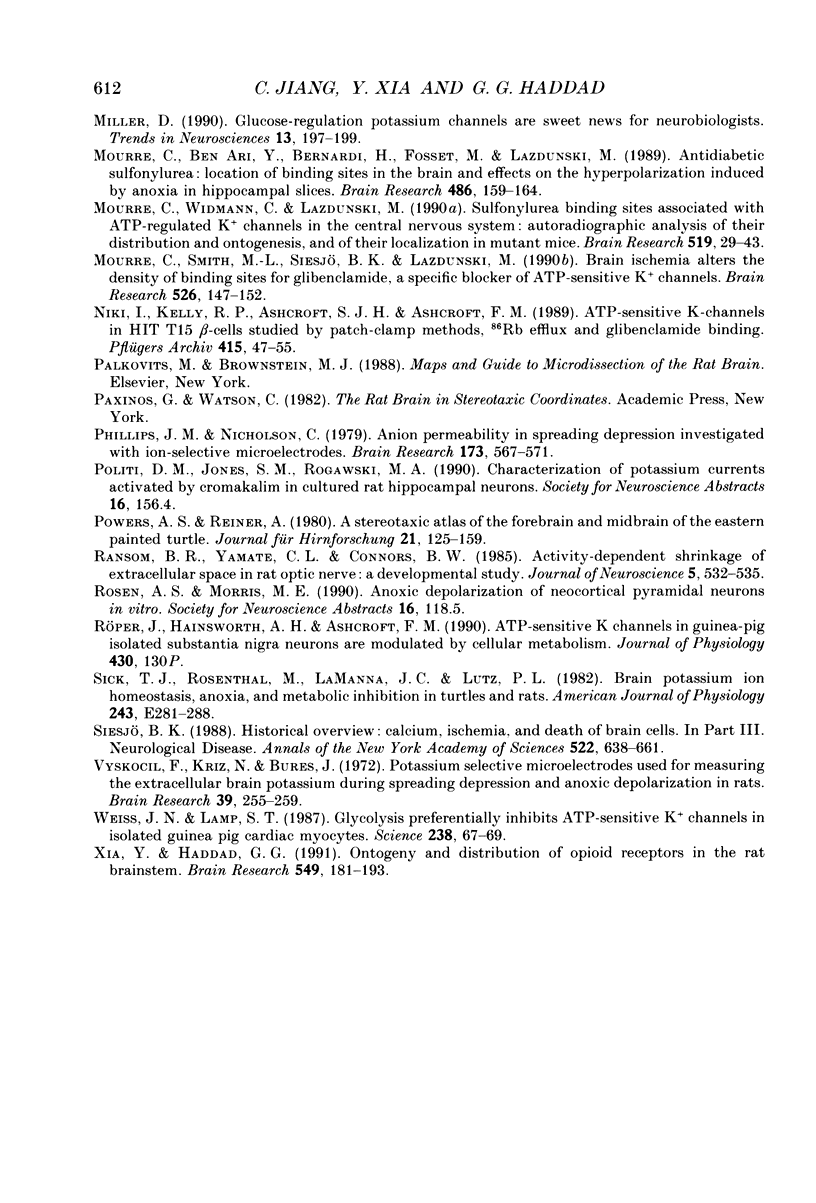
Images in this article
Selected References
These references are in PubMed. This may not be the complete list of references from this article.
- Ammann D., Chao P. S., Simon W. Valinomycin-based K+ selective microelectrodes with low electrical membrane resistance. Neurosci Lett. 1987 Feb 24;74(2):221–226. doi: 10.1016/0304-3940(87)90153-4. [DOI] [PubMed] [Google Scholar]
- Amoroso S., Schmid-Antomarchi H., Fosset M., Lazdunski M. Glucose, sulfonylureas, and neurotransmitter release: role of ATP-sensitive K+ channels. Science. 1990 Feb 16;247(4944):852–854. doi: 10.1126/science.2305257. [DOI] [PubMed] [Google Scholar]
- Ashcroft F. M. Adenosine 5'-triphosphate-sensitive potassium channels. Annu Rev Neurosci. 1988;11:97–118. doi: 10.1146/annurev.ne.11.030188.000525. [DOI] [PubMed] [Google Scholar]
- Ashford M. L., Boden P. R., Treherne J. M. Glucose-induced excitation of hypothalamic neurones is mediated by ATP-sensitive K+ channels. Pflugers Arch. 1990 Jan;415(4):479–483. doi: 10.1007/BF00373626. [DOI] [PubMed] [Google Scholar]
- Ashford M. L., Sturgess N. C., Trout N. J., Gardner N. J., Hales C. N. Adenosine-5'-triphosphate-sensitive ion channels in neonatal rat cultured central neurones. Pflugers Arch. 1988 Aug;412(3):297–304. doi: 10.1007/BF00582512. [DOI] [PubMed] [Google Scholar]
- Astrup J., Skovsted P., Gjerris F., Sørensen H. R. Increase in extracellular potassium in the brain during circulatory arrest: effects of hypothermia, lidocaine, and thiopental. Anesthesiology. 1981 Sep;55(3):256–262. doi: 10.1097/00000542-198109000-00012. [DOI] [PubMed] [Google Scholar]
- Bekheit S. S., Restivo M., Boutjdir M., Henkin R., Gooyandeh K., Assadi M., Khatib S., Gough W. B., el-Sherif N. Effects of glyburide on ischemia-induced changes in extracellular potassium and local myocardial activation: a potential new approach to the management of ischemia-induced malignant ventricular arrhythmias. Am Heart J. 1990 May;119(5):1025–1033. doi: 10.1016/s0002-8703(05)80231-5. [DOI] [PubMed] [Google Scholar]
- Ben Ari Y. Effect of glibenclamide, a selective blocker of an ATP-K+ channel, on the anoxic response of hippocampal neurones. Pflugers Arch. 1989;414 (Suppl 1):S111–S114. doi: 10.1007/BF00582258. [DOI] [PubMed] [Google Scholar]
- Ben-Ari Y., Krnjević K., Crépel V. Activators of ATP-sensitive K+ channels reduce anoxic depolarization in CA3 hippocampal neurons. Neuroscience. 1990;37(1):55–60. doi: 10.1016/0306-4522(90)90191-6. [DOI] [PubMed] [Google Scholar]
- Carlini W. G., Ransom B. R. Tip size of ion-exchanger based K+-selective microelectrodes. I. Effects on selectivity. Can J Physiol Pharmacol. 1987 May;65(5):889–893. doi: 10.1139/y87-143. [DOI] [PubMed] [Google Scholar]
- Chih C. P., Rosenthal M., Sick T. J. Ion leakage is reduced during anoxia in turtle brain: a potential survival strategy. Am J Physiol. 1989 Dec;257(6 Pt 2):R1562–R1564. doi: 10.1152/ajpregu.1989.257.6.R1562. [DOI] [PubMed] [Google Scholar]
- Choi D. W. Cerebral hypoxia: some new approaches and unanswered questions. J Neurosci. 1990 Aug;10(8):2493–2501. doi: 10.1523/JNEUROSCI.10-08-02493.1990. [DOI] [PMC free article] [PubMed] [Google Scholar]
- Cruce W. L., Nieuwenhuys R. The cell masses in the brain stem of the turtle Testudo hermanni; a topographical and topological analysis. J Comp Neurol. 1974 Aug 1;156(3):277–306. doi: 10.1002/cne.901560303. [DOI] [PubMed] [Google Scholar]
- Davies N. W. Modulation of ATP-sensitive K+ channels in skeletal muscle by intracellular protons. Nature. 1990 Jan 25;343(6256):375–377. doi: 10.1038/343375a0. [DOI] [PubMed] [Google Scholar]
- Dietzel I., Heinemann U., Hofmeier G., Lux H. D. Transient changes in the size of the extracellular space in the sensorimotor cortex of cats in relation to stimulus-induced changes in potassium concentration. Exp Brain Res. 1980;40(4):432–439. doi: 10.1007/BF00236151. [DOI] [PubMed] [Google Scholar]
- Escande D., Thuringer D., Le Guern S., Courteix J., Laville M., Cavero I. Potassium channel openers act through an activation of ATP-sensitive K+ channels in guinea-pig cardiac myocytes. Pflugers Arch. 1989 Sep;414(6):669–675. doi: 10.1007/BF00582134. [DOI] [PubMed] [Google Scholar]
- Haddad G. G., Donnelly D. F., Getting P. A. Biophysical properties of hypoglossal neurons in vitro: intracellular studies in adult and neonatal rats. J Appl Physiol (1985) 1990 Oct;69(4):1509–1517. doi: 10.1152/jappl.1990.69.4.1509. [DOI] [PubMed] [Google Scholar]
- Haddad G. G., Donnelly D. F. O2 deprivation induces a major depolarization in brain stem neurons in the adult but not in the neonatal rat. J Physiol. 1990 Oct;429:411–428. doi: 10.1113/jphysiol.1990.sp018265. [DOI] [PMC free article] [PubMed] [Google Scholar]
- Haddad G. G., Getting P. A. Repetitive firing properties of neurons in the ventral region of nucleus tractus solitarius. In vitro studies in adult and neonatal rat. J Neurophysiol. 1989 Dec;62(6):1213–1224. doi: 10.1152/jn.1989.62.6.1213. [DOI] [PubMed] [Google Scholar]
- Haddad G. G., Mellins R. B. Hypoxia and respiratory control in early life. Annu Rev Physiol. 1984;46:629–643. doi: 10.1146/annurev.ph.46.030184.003213. [DOI] [PubMed] [Google Scholar]
- Hansen A. J. Effect of anoxia on ion distribution in the brain. Physiol Rev. 1985 Jan;65(1):101–148. doi: 10.1152/physrev.1985.65.1.101. [DOI] [PubMed] [Google Scholar]
- Hansen A. J. Extracellular potassium concentration in juvenile and adult rat brain cortex during anoxia. Acta Physiol Scand. 1977 Apr;99(4):412–420. doi: 10.1111/j.1748-1716.1977.tb10394.x. [DOI] [PubMed] [Google Scholar]
- Hansen A. J., Olsen C. E. Brain extracellular space during spreading depression and ischemia. Acta Physiol Scand. 1980 Apr;108(4):355–365. doi: 10.1111/j.1748-1716.1980.tb06544.x. [DOI] [PubMed] [Google Scholar]
- Hansen A. J. The extracellular potassium concentration in brain cortex following ischemia in hypo- and hyperglycemic rats. Acta Physiol Scand. 1978 Mar;102(3):324–329. doi: 10.1111/j.1748-1716.1978.tb06079.x. [DOI] [PubMed] [Google Scholar]
- Hellmann J., Vannucci R. C., Nardis E. E. Blood-brain barrier permeability to lactic acid in the newborn dog: lactate as a cerebral metabolic fuel. Pediatr Res. 1982 Jan;16(1):40–44. doi: 10.1203/00006450-198201001-00008. [DOI] [PubMed] [Google Scholar]
- Hochachka P. W. Defense strategies against hypoxia and hypothermia. Science. 1986 Jan 17;231(4735):234–241. doi: 10.1126/science.2417316. [DOI] [PubMed] [Google Scholar]
- Jiang C., Haddad G. G. Effect of anoxia on intracellular and extracellular potassium activity in hypoglossal neurons in vitro. J Neurophysiol. 1991 Jul;66(1):103–111. doi: 10.1152/jn.1991.66.1.103. [DOI] [PubMed] [Google Scholar]
- Kantor P. F., Coetzee W. A., Carmeliet E. E., Dennis S. C., Opie L. H. Reduction of ischemic K+ loss and arrhythmias in rat hearts. Effect of glibenclamide, a sulfonylurea. Circ Res. 1990 Feb;66(2):478–485. doi: 10.1161/01.res.66.2.478. [DOI] [PubMed] [Google Scholar]
- Kass I. S., Lipton P. Protection of hippocampal slices from young rats against anoxic transmission damage is due to better maintenance of ATP. J Physiol. 1989 Jun;413:1–11. doi: 10.1113/jphysiol.1989.sp017638. [DOI] [PMC free article] [PubMed] [Google Scholar]
- Kawasaki K., Traynelis S. F., Dingledine R. Different responses of CA1 and CA3 regions to hypoxia in rat hippocampal slice. J Neurophysiol. 1990 Mar;63(3):385–394. doi: 10.1152/jn.1990.63.3.385. [DOI] [PubMed] [Google Scholar]
- Krnjević K., Leblond J. Changes in membrane currents of hippocampal neurons evoked by brief anoxia. J Neurophysiol. 1989 Jul;62(1):15–30. doi: 10.1152/jn.1989.62.1.15. [DOI] [PubMed] [Google Scholar]
- Leblond J., Krnjevic K. Hypoxic changes in hippocampal neurons. J Neurophysiol. 1989 Jul;62(1):1–14. doi: 10.1152/jn.1989.62.1.1. [DOI] [PubMed] [Google Scholar]
- Mares P., Kríz N., Brozek G., Bures J. Anoxic changes of extracellular potassium concentration in the cerebral cortex of young rats. Exp Neurol. 1976 Oct;53(1):12–20. doi: 10.1016/0014-4886(76)90277-6. [DOI] [PubMed] [Google Scholar]
- Medina J. M. The role of lactate as an energy substrate for the brain during the early neonatal period. Biol Neonate. 1985;48(4):237–244. doi: 10.1159/000242176. [DOI] [PubMed] [Google Scholar]
- Meyer F. B. Calcium, neuronal hyperexcitability and ischemic injury. Brain Res Brain Res Rev. 1989 Jul-Sep;14(3):227–243. doi: 10.1016/0165-0173(89)90002-7. [DOI] [PubMed] [Google Scholar]
- Miller R. J. Glucose-regulated potassium channels are sweet news for neurobiologists. Trends Neurosci. 1990 Jun;13(6):197–199. doi: 10.1016/0166-2236(90)90158-7. [DOI] [PubMed] [Google Scholar]
- Mourre C., Ben Ari Y., Bernardi H., Fosset M., Lazdunski M. Antidiabetic sulfonylureas: localization of binding sites in the brain and effects on the hyperpolarization induced by anoxia in hippocampal slices. Brain Res. 1989 May 1;486(1):159–164. doi: 10.1016/0006-8993(89)91288-2. [DOI] [PubMed] [Google Scholar]
- Mourre C., Smith M. L., Siesjö B. K., Lazdunski M. Brain ischemia alters the density of binding sites for glibenclamide, a specific blocker of ATP-sensitive K+ channels. Brain Res. 1990 Aug 27;526(1):147–152. doi: 10.1016/0006-8993(90)90262-a. [DOI] [PubMed] [Google Scholar]
- Mourre C., Widmann C., Lazdunski M. Sulfonylurea binding sites associated with ATP-regulated K+ channels in the central nervous system: autoradiographic analysis of their distribution and ontogenesis, and of their localization in mutant mice cerebellum. Brain Res. 1990 Jun 11;519(1-2):29–43. doi: 10.1016/0006-8993(90)90057-i. [DOI] [PubMed] [Google Scholar]
- Niki I., Kelly R. P., Ashcroft S. J., Ashcroft F. M. ATP-sensitive K-channels in HIT T15 beta-cells studied by patch-clamp methods, 86Rb efflux and glibenclamide binding. Pflugers Arch. 1989 Oct;415(1):47–55. doi: 10.1007/BF00373140. [DOI] [PubMed] [Google Scholar]
- Phillips J. M., Nicholson C. Anion permeability in spreading depression investigated with ion-sensitive microelectrodes. Brain Res. 1979 Sep 21;173(3):567–571. doi: 10.1016/0006-8993(79)90254-3. [DOI] [PubMed] [Google Scholar]
- Powers A. S., Reiner A. A stereotaxic atlas of the forebrain and midbrain of the eastern painted turtle (Chrysemys picta picta). J Hirnforsch. 1980;21(2):125–159. [PubMed] [Google Scholar]
- Ransom B. R., Yamate C. L., Connors B. W. Activity-dependent shrinkage of extracellular space in rat optic nerve: a developmental study. J Neurosci. 1985 Feb;5(2):532–535. doi: 10.1523/JNEUROSCI.05-02-00532.1985. [DOI] [PMC free article] [PubMed] [Google Scholar]
- Vyskocil F., Kritz N., Bures J. Potassium-selective microelectrodes used for measuring the extracellular brain potassium during spreading depression and anoxic depolarization in rats. Brain Res. 1972 Apr 14;39(1):255–259. doi: 10.1016/0006-8993(72)90802-5. [DOI] [PubMed] [Google Scholar]
- Weiss J. N., Lamp S. T. Glycolysis preferentially inhibits ATP-sensitive K+ channels in isolated guinea pig cardiac myocytes. Science. 1987 Oct 2;238(4823):67–69. doi: 10.1126/science.2443972. [DOI] [PubMed] [Google Scholar]
- Xia Y., Haddad G. G. Ontogeny and distribution of opioid receptors in the rat brainstem. Brain Res. 1991 May 24;549(2):181–193. doi: 10.1016/0006-8993(91)90457-7. [DOI] [PubMed] [Google Scholar]
- de Weille J. R., Lazdunski M. Regulation of the ATP-sensitive potassium channel. Ion Channels. 1990;2:205–222. doi: 10.1007/978-1-4615-7305-0_6. [DOI] [PubMed] [Google Scholar]
- de Weille J. R., Schmid-Antomarchi H., Fosset M., Lazdunski M. Regulation of ATP-sensitive K+ channels in insulinoma cells: activation by somatostatin and protein kinase C and the role of cAMP. Proc Natl Acad Sci U S A. 1989 Apr;86(8):2971–2975. doi: 10.1073/pnas.86.8.2971. [DOI] [PMC free article] [PubMed] [Google Scholar]