Abstract
1. The maximum activities of hexokinase, phosphorylase and phosphofructokinase have been measured in extracts from a variety of muscles and they have been used to estimate the maximum rates of operation of glycolysis in muscle. These estimated rates of glycolysis are compared with those calculated for the intact muscle from such information as oxygen uptake, glycogen degradation and lactate formation. Reasonable agreement between these determinations is observed, and this suggests that such enzyme activity measurements may provide a useful method for comparative investigations into quantitative aspects of maximum glycolytic flux in muscle. 2. The enzyme activities from insect flight muscle confirm and extend much of the earlier work and indicate the type of fuel that can support insect flight. The maximum activity of hexokinase in some insect flight muscles is about tenfold higher than that in vertebrate muscles. The activity of phosphorylase is greater, in general, in vertebrate muscle (particularly white muscle) than in insect flight muscle. This is probably related to the role of glycogen breakdown in vertebrate muscle (particularly white muscle) for the provision of ATP from anaerobic glycolysis and not from complete oxidation of the glucose residues. The activity of hexokinase was found to be higher in red than in white vertebrate muscle, thus confirming and extending earlier reports. 3. The maximum activity of the mitochondrial glycerophosphate dehydrogenase was always much lower than that of the cytoplasmic enzyme, indicating that the former enzyme is rate-limiting for the glycerol 3-phosphate cycle. From the maximum activity of the mitochondrial enzyme it can be calculated that the operation of this cycle would account for the reoxidation of all the glycolytically produced NADH in insect flight muscle but it could account for only a small amount in vertebrate muscle. Other mechanisms for this NADH reoxidation in vertebrate muscle are discussed briefly.
Full text
PDF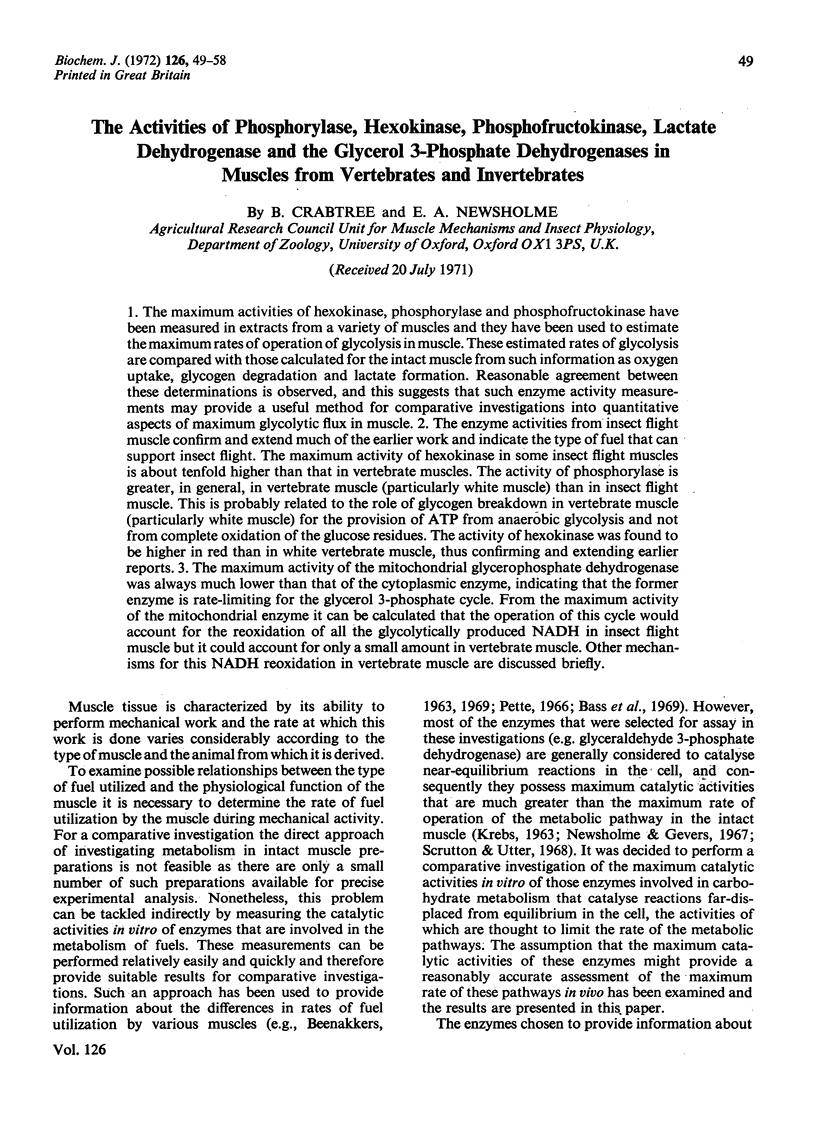
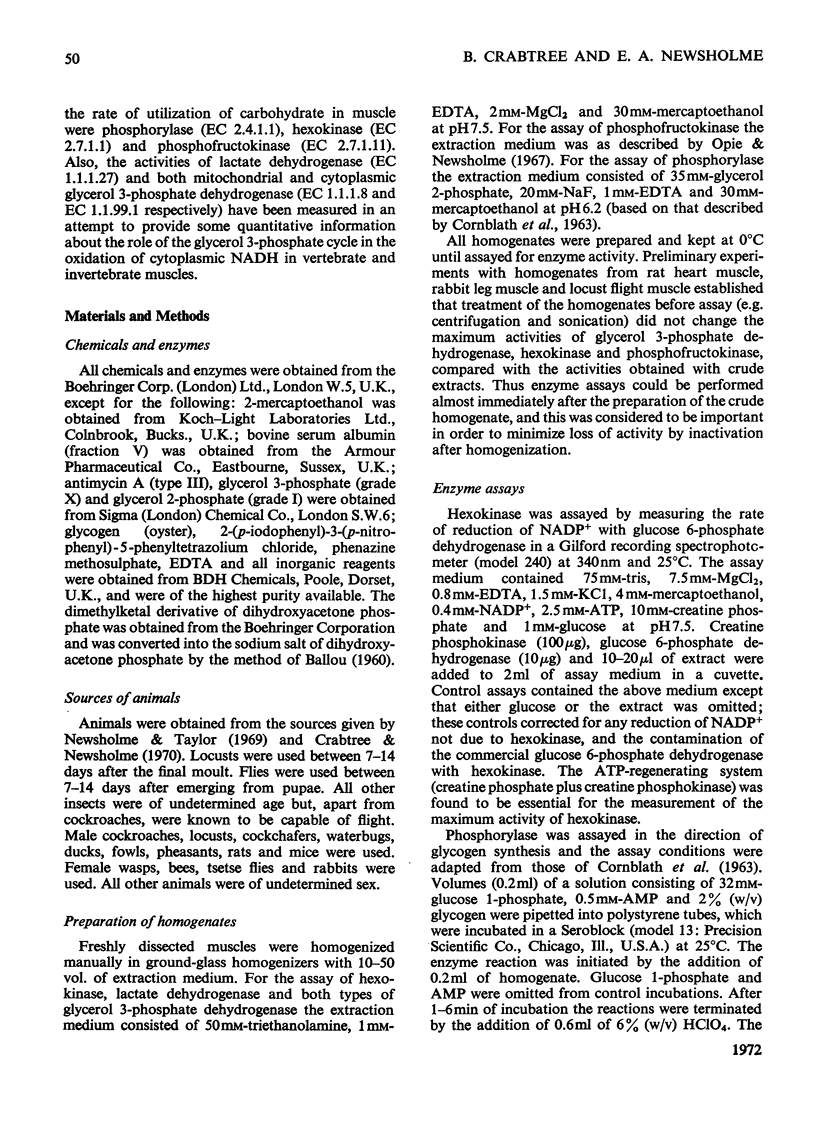
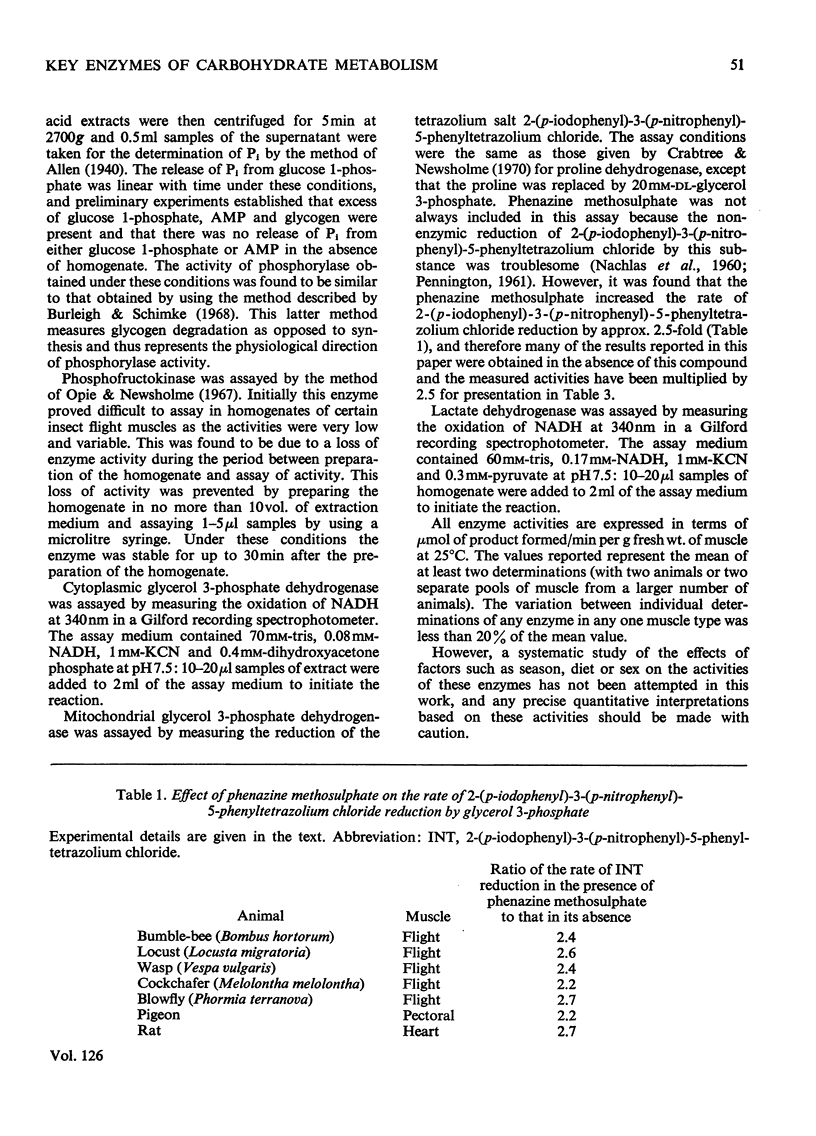
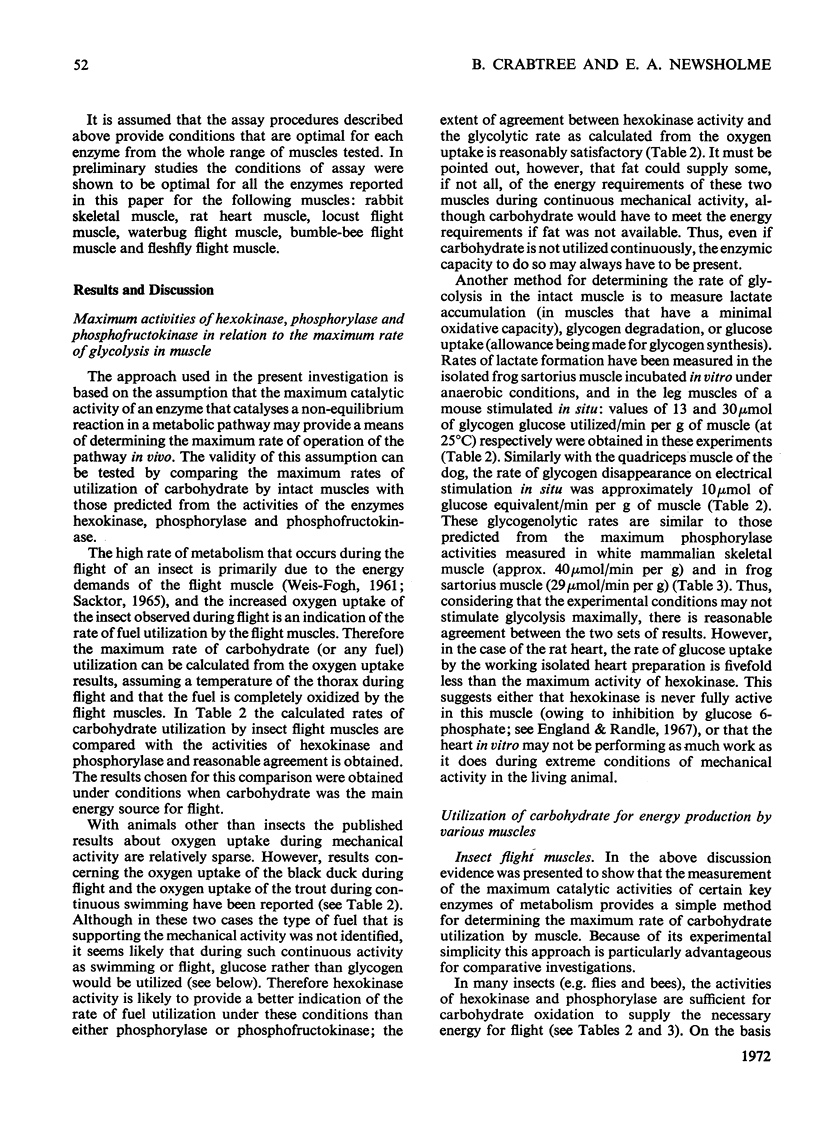
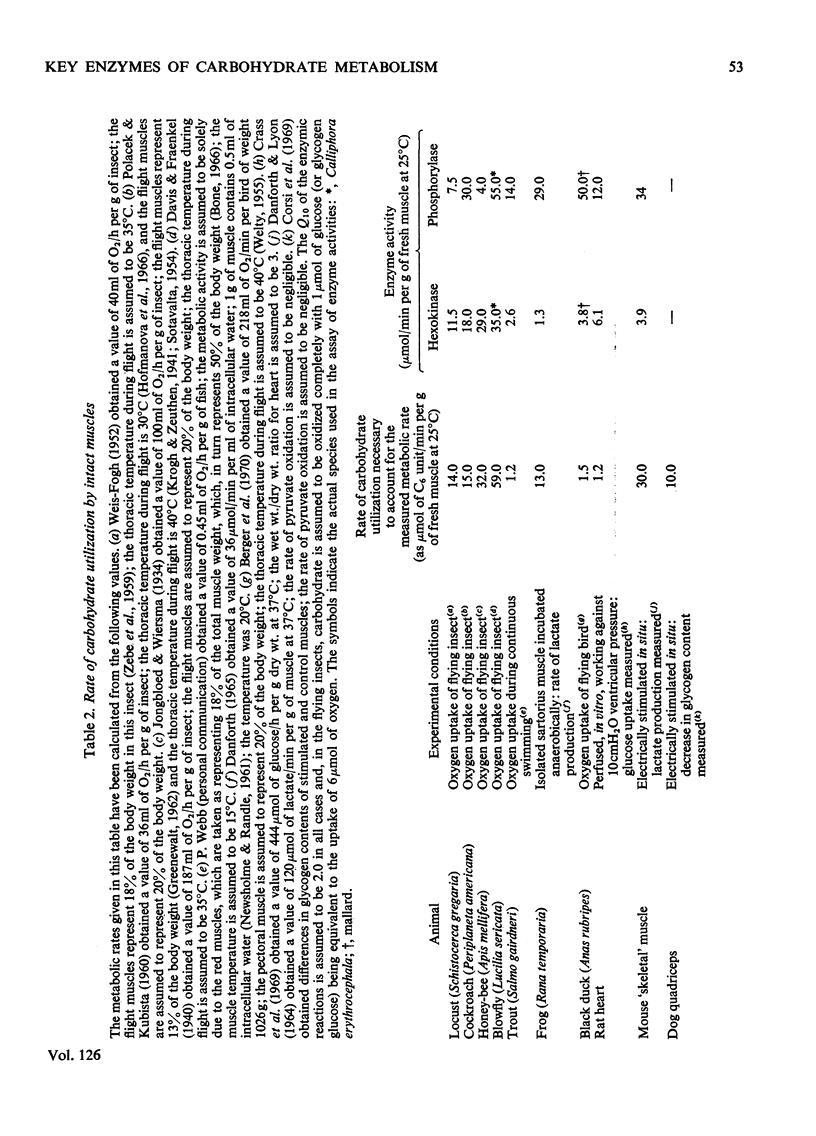
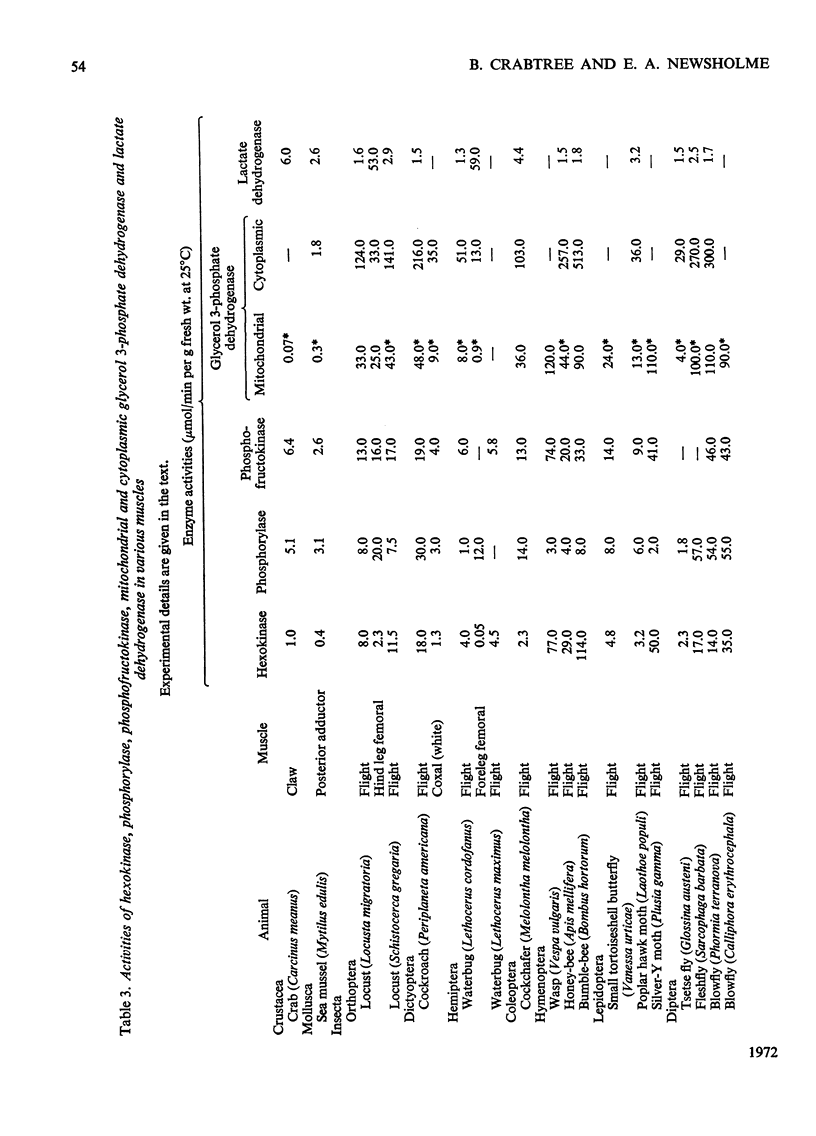
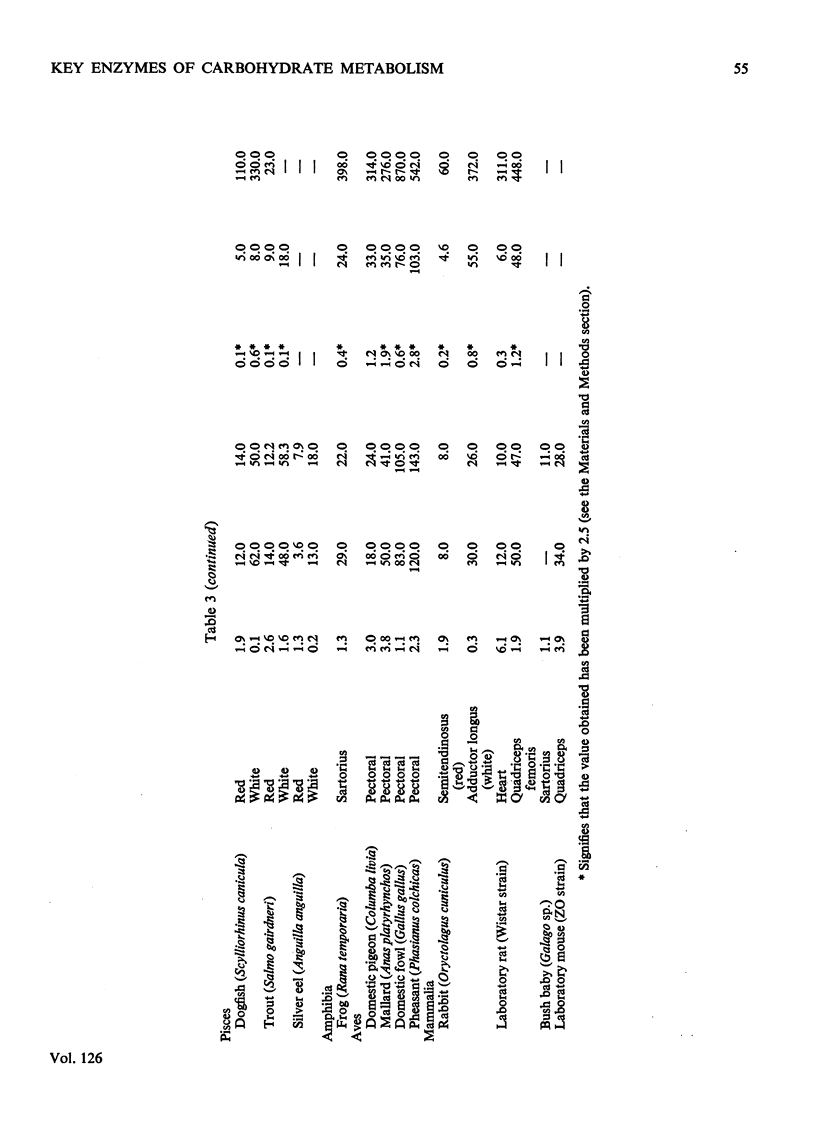
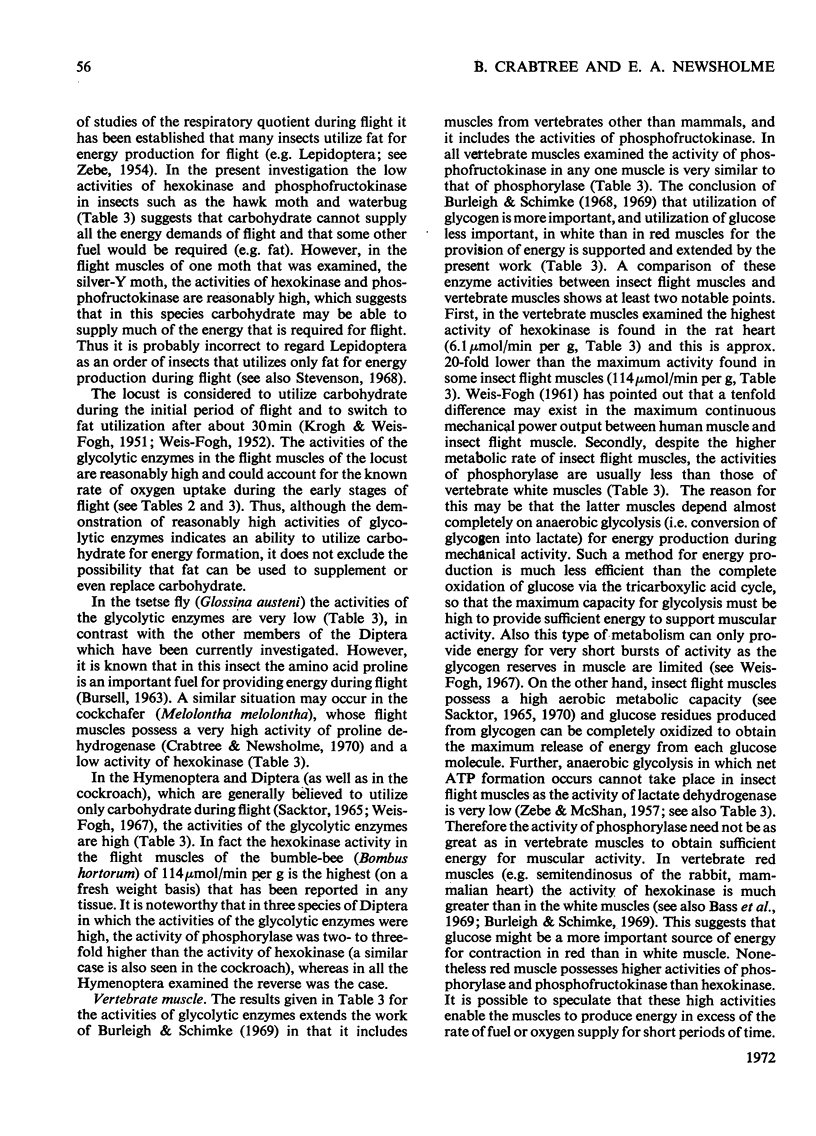
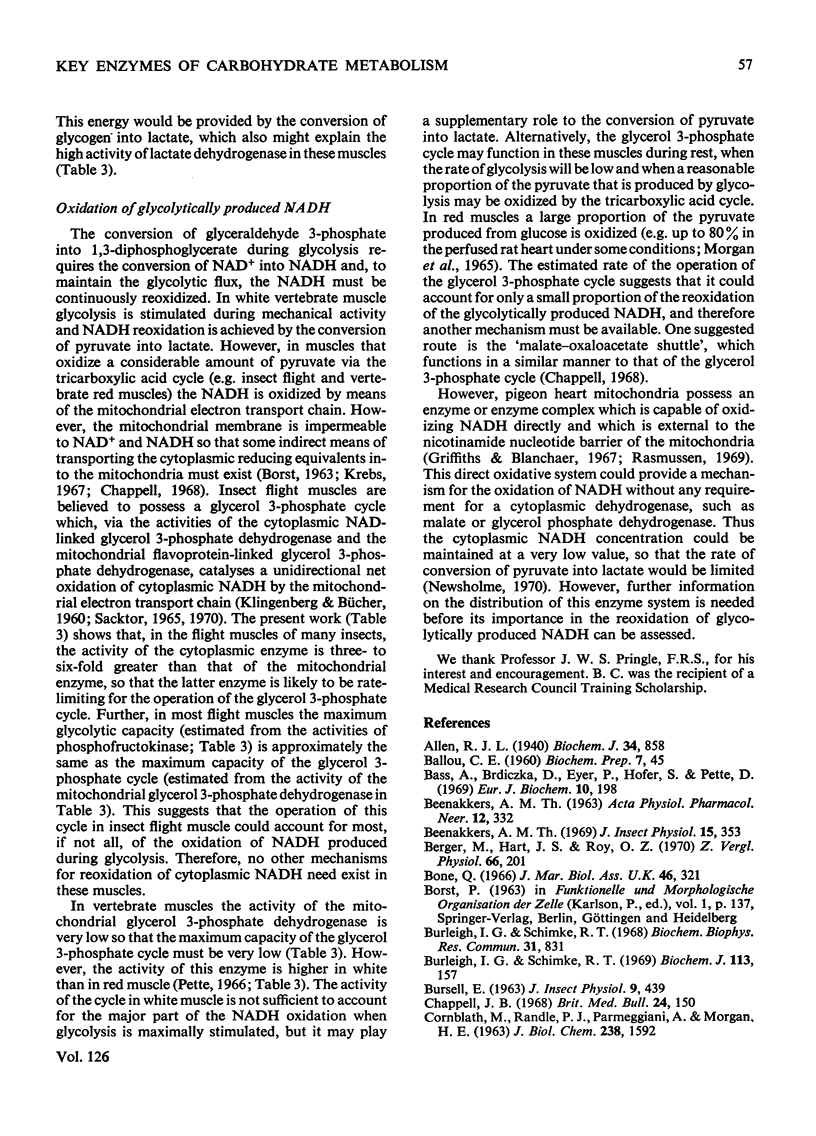
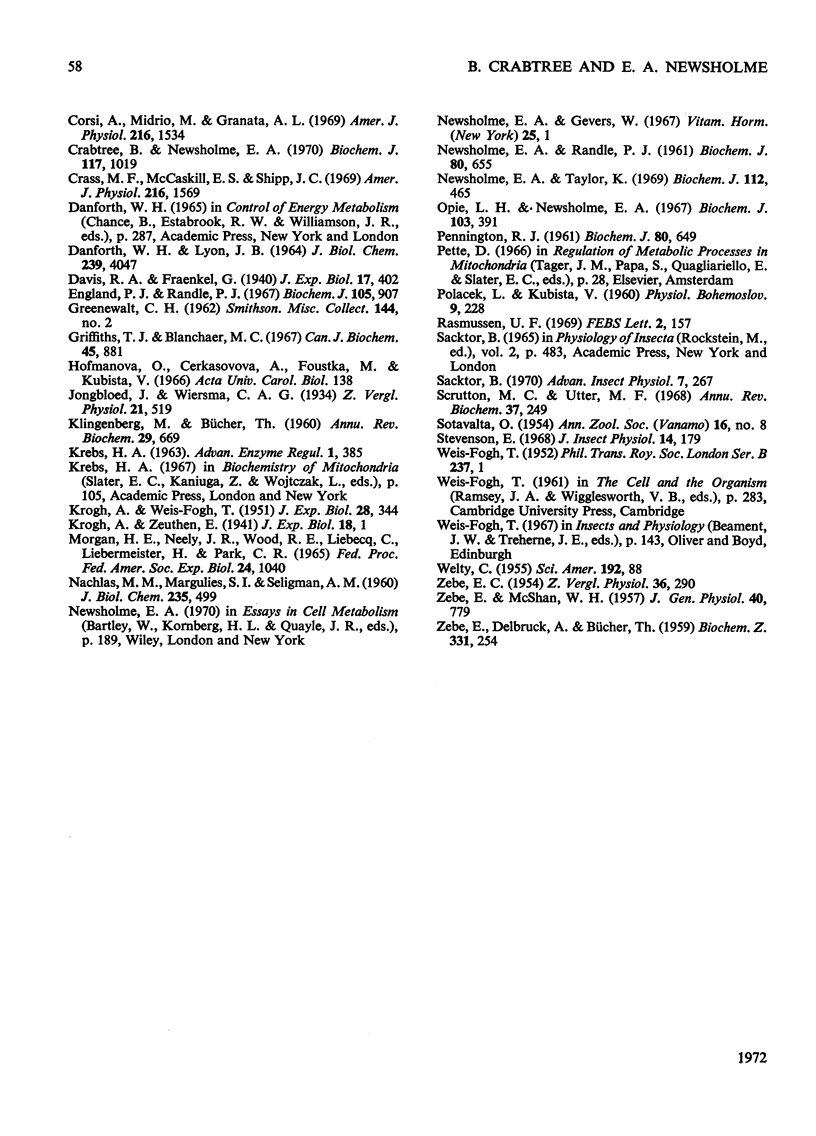
Selected References
These references are in PubMed. This may not be the complete list of references from this article.
- Allen R. J. The estimation of phosphorus. Biochem J. 1940 Jun;34(6):858–865. doi: 10.1042/bj0340858. [DOI] [PMC free article] [PubMed] [Google Scholar]
- Bass A., Brdiczka D., Eyer P., Hofer S., Pette D. Metabolic differentiation of distinct muscle types at the level of enzymatic organization. Eur J Biochem. 1969 Sep;10(2):198–206. doi: 10.1111/j.1432-1033.1969.tb00674.x. [DOI] [PubMed] [Google Scholar]
- Beenakkers A. M. Carbohydrate and fat as a fuel for insect flight. A comparative study. J Insect Physiol. 1969 Mar;15(3):353–361. doi: 10.1016/0022-1910(69)90281-9. [DOI] [PubMed] [Google Scholar]
- Burleigh G., Schimke R. T. On the activities of some enzymes concerned with glycolysis and glycogenolysis in extracts of rabbit skeletal muscles. Biochem Biophys Res Commun. 1968 Jun 10;31(5):831–836. doi: 10.1016/0006-291x(68)90638-4. [DOI] [PubMed] [Google Scholar]
- Burleigh I. G., Schimke R. T. The activities of some enzymes concerned with energy metabolism in mammalian muscles of differing pigmentation. Biochem J. 1969 Jun;113(1):157–166. doi: 10.1042/bj1130157. [DOI] [PMC free article] [PubMed] [Google Scholar]
- CORNBLATH M., RANDLE P. J., PARMEGGIANI A., MORGAN H. E. Regulation of glycogenolysis in muscle. Effects of glucagon and anoxia on lactate production, glycogen content, and phosphorylase activity in the perfused isolated rat heart. J Biol Chem. 1963 May;238:1592–1597. [PubMed] [Google Scholar]
- Chappell J. B. Systems used for the transport of substrates into mitochondria. Br Med Bull. 1968 May;24(2):150–157. doi: 10.1093/oxfordjournals.bmb.a070618. [DOI] [PubMed] [Google Scholar]
- Corsi A., Midrio M., Granata A. L. In situ utilization of glycogen and blood glucose by skeletal muscle during tetanus. Am J Physiol. 1969 Jun;216(6):1534–1541. doi: 10.1152/ajplegacy.1969.216.6.1534. [DOI] [PubMed] [Google Scholar]
- Crabtree B., Newsholme E. A. The activities of proline dehydrogenase, glutamate dehydrogenase, aspartate-oxoglutarate aminotransferase and alanine-oxoglutarate aminotransferase in some insect flight muscles. Biochem J. 1970 May;117(5):1019–1021. doi: 10.1042/bj1171019. [DOI] [PMC free article] [PubMed] [Google Scholar]
- Crass M. F., 3rd, McCaskill E. S., Shipp J. C. Effect of pressure development on glucose and palmitate metabolism in perfused heart. Am J Physiol. 1969 Jun;216(6):1569–1576. doi: 10.1152/ajplegacy.1969.216.6.1569. [DOI] [PubMed] [Google Scholar]
- DANFORTH W. H., LYON J. B., Jr GLYCOGENOLYSIS DURING TETANIC CONTRACTION OF ISOLATED MOUSE MUSCLES IN THE PRESENCE AND ABSENCE OF PHOSPHORYLASE A. J Biol Chem. 1964 Dec;239:4047–4050. [PubMed] [Google Scholar]
- England P. J., Randle P. J. Effectors of rat-heart hexokinases and the control of rates of glucose phosphorylation in the perfused rat heart. Biochem J. 1967 Dec;105(3):907–920. doi: 10.1042/bj1050907. [DOI] [PMC free article] [PubMed] [Google Scholar]
- Griffith T. J., Blanchaer M. C. Kinetics of NADH oxidation by pigeon-heart mitochondria. Can J Biochem. 1967 Jun;45(6):881–888. doi: 10.1139/o67-098. [DOI] [PubMed] [Google Scholar]
- KLINGENBERG M., BUECHER T. Biological oxidations. Annu Rev Biochem. 1960;29:669–708. doi: 10.1146/annurev.bi.29.070160.003321. [DOI] [PubMed] [Google Scholar]
- KREBS H. A. RENAL GLUCONEOGENESIS. Adv Enzyme Regul. 1963;1:385–400. doi: 10.1016/0065-2571(63)90034-7. [DOI] [PubMed] [Google Scholar]
- Morgan H. E., Neely J. R., Wood R. E., Liébecq C., Liebermeister H., Park C. R. Factors affecting glucose transport in heart muscle and erythrocytes. Fed Proc. 1965 Sep-Oct;24(5):1040–1045. [PubMed] [Google Scholar]
- NACHLAS M. M., MARGULIES S. I., SELIGMAN A. M. A colorimetric method for the estimation of succinic dehydrogenase activity. J Biol Chem. 1960 Feb;235:499–503. [PubMed] [Google Scholar]
- NEWSHOLME E. A., RANDLE P. J. Regulation of glucose uptake by muscle. 5. Effects of anoxia, insulin, adrenaline and prolonged starving on concentrations of hexose phosphates in isolated rat diaphragm and perfused isolated rat heart. Biochem J. 1961 Sep;80:655–662. doi: 10.1042/bj0800655. [DOI] [PMC free article] [PubMed] [Google Scholar]
- Newsholme E. A., Taylor K. Glycerol kinase activities in muscles from vertebrates and invertebrates. Biochem J. 1969 May;112(4):465–474. doi: 10.1042/bj1120465. [DOI] [PMC free article] [PubMed] [Google Scholar]
- Opie L. H., Newsholme E. A. The activities of fructose 1,6-diphosphatase, phosphofructokinase and phosphoenolpyruvate carboxykinase in white muscle and red muscle. Biochem J. 1967 May;103(2):391–399. doi: 10.1042/bj1030391. [DOI] [PMC free article] [PubMed] [Google Scholar]
- PENNINGTON R. J. Biochemistry of dystrophic muscle. Mitochondrial succinate-tetrazolium reductase and adenosine triphosphatase. Biochem J. 1961 Sep;80:649–654. doi: 10.1042/bj0800649. [DOI] [PMC free article] [PubMed] [Google Scholar]
- Rasmussen U. F. The oxidation of added NADH by intact heart mitochondria. FEBS Lett. 1969 Jan;2(3):157–162. doi: 10.1016/0014-5793(69)80006-2. [DOI] [PubMed] [Google Scholar]
- ZEBE E. C., MCSHAN W. H. Lactic and alpha-glycerophosphate dehydrogenases in insects. J Gen Physiol. 1957 May 20;40(5):779–790. doi: 10.1085/jgp.40.5.779. [DOI] [PMC free article] [PubMed] [Google Scholar]