Abstract
1. The function of ubiquinone in Escherichia coli was studied by using whole cells and membrane preparations of normal E. coli and of a mutant lacking ubiquinone. 2. The mutant lacking ubiquinone, strain AN59 (Ubi−), when grown under aerobic conditions, gave an anaerobic type of growth yield and produced large quantities of lactic acid, indicating that ubiquinone plays a vital role in electron transport. 3. NADH and lactate oxidase activities in membranes from strain AN59 (Ubi−) were greatly impaired and activity was restored by the addition of ubiquinone (Q-1). 4. Comparison of the percentage reduction of flavin, cytochrome b1 and cytochrome a2 in the aerobic steady state in membranes from the normal strain (AN62) and strain AN59 (Ubi−) and the effect of respiratory inhibitors on these percentages in membranes from strain AN62 suggest that ubiquinone functions at more than one site in the electron-transport chain. 5. Membranes from strain AN62, in the absence of substrate, showed an electron-spin-resonance signal attributed to ubisemiquinone. The amount of reduced ubiquinone (50%) found after rapid solvent extraction is consistent with the existence of ubiquinone in membranes as a stabilized ubisemiquinone. 6. The effects of piericidin A on membranes from strain AN62 suggest that this inhibitor acts at the ubiquinone sites: thus inhibition of electron transport is reversed by ubiquinone (Q-1); the aerobic steady-state oxidation–reduction levels of flavins and cytochrome b1 in the presence of the inhibitor are raised to values approximating those found in the membranes of strain AN59 (Ubi−); the inhibitor rapidly eliminates the electron-spin-resonance signal attributed to ubisemiquinone and allows slow oxidation of endogenous ubiquinol in the absence of substrate and prevents reduction of ubiquinone in the presence of substrate. It is concluded that piericidin A separates ubiquinone from the remainder of the electron-transport chain. 7. A scheme is proposed in which ubisemiquinone, complexed to an electron carrier, functions in at least two positions in the electron-transport sequence.
Full text
PDF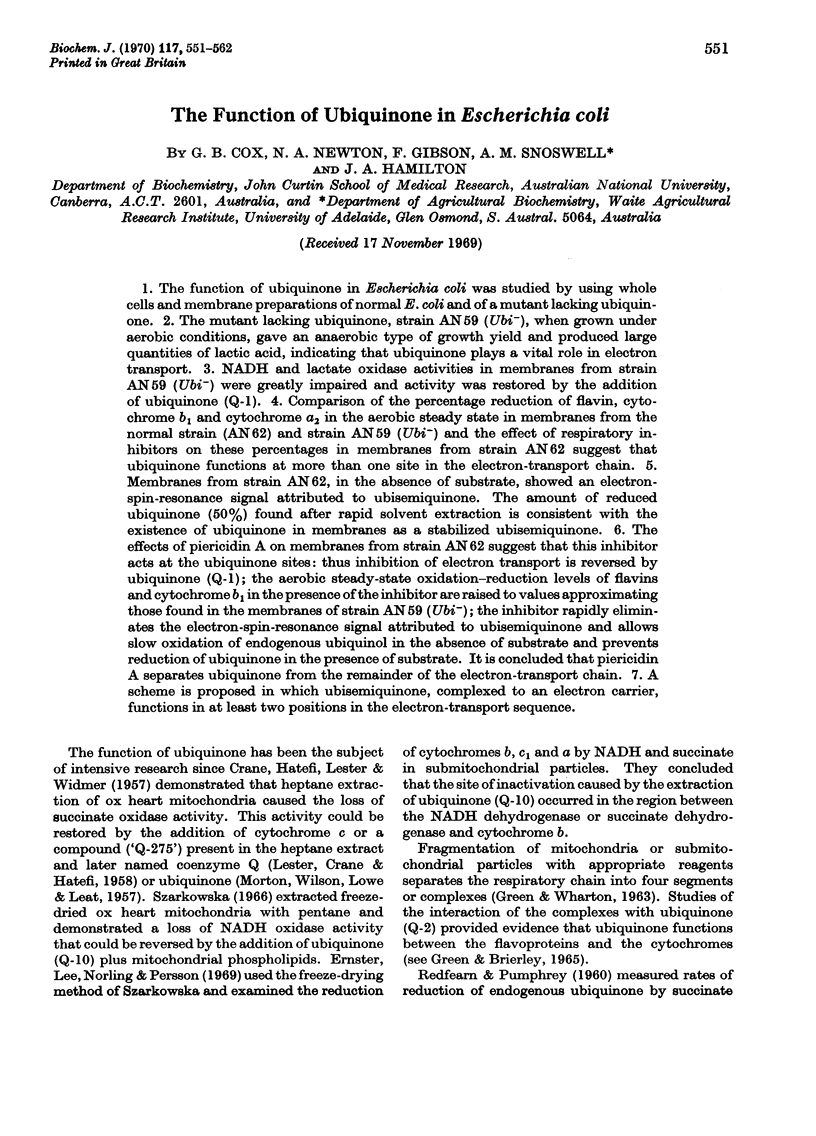
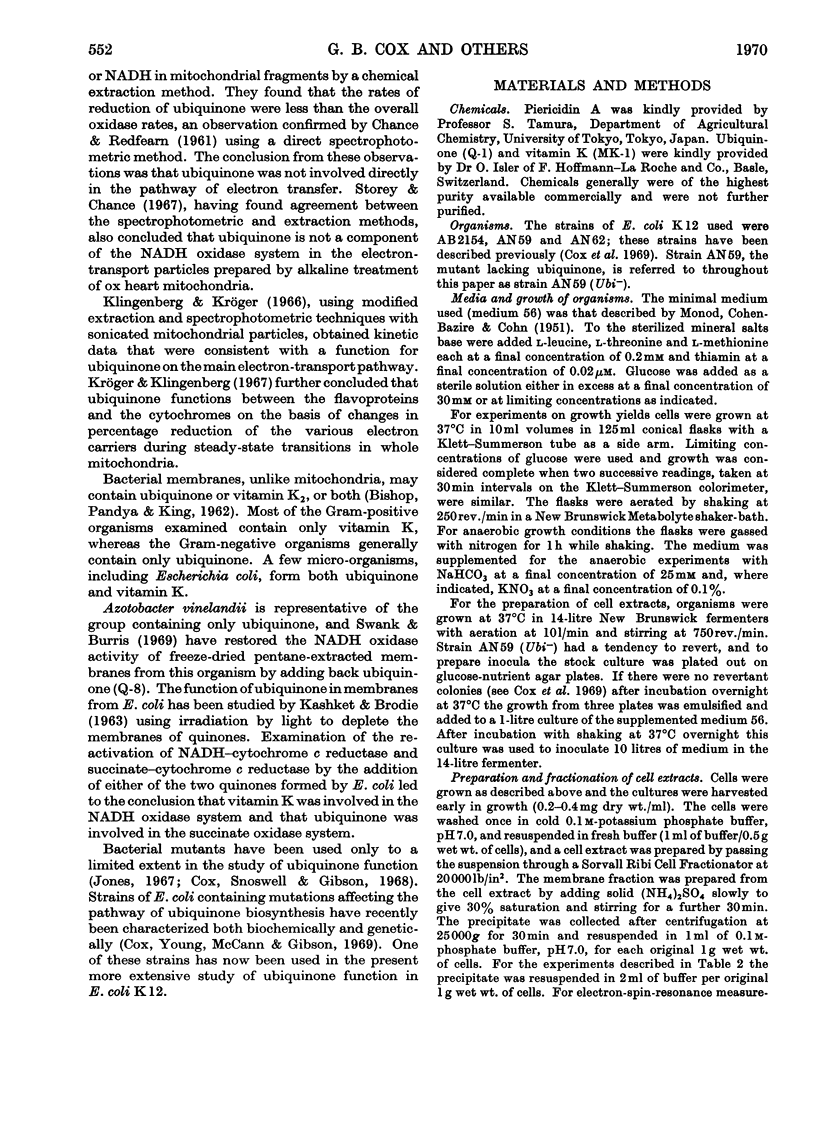
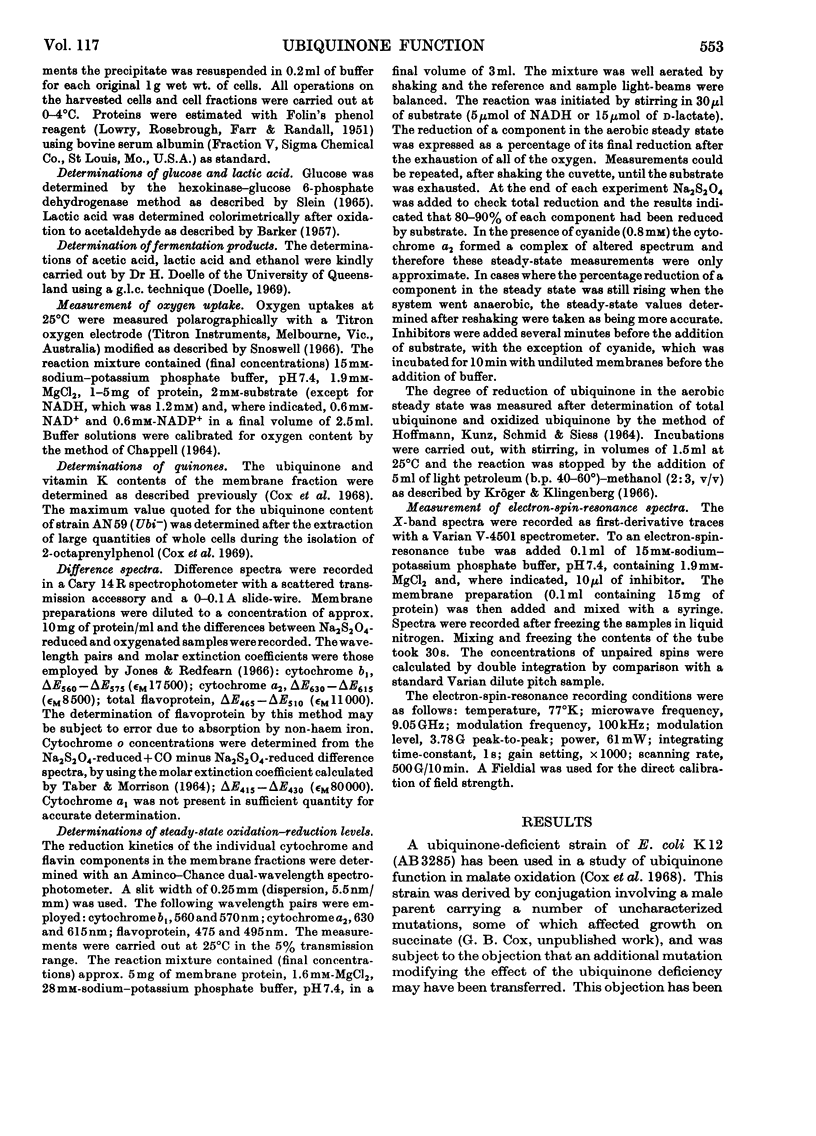
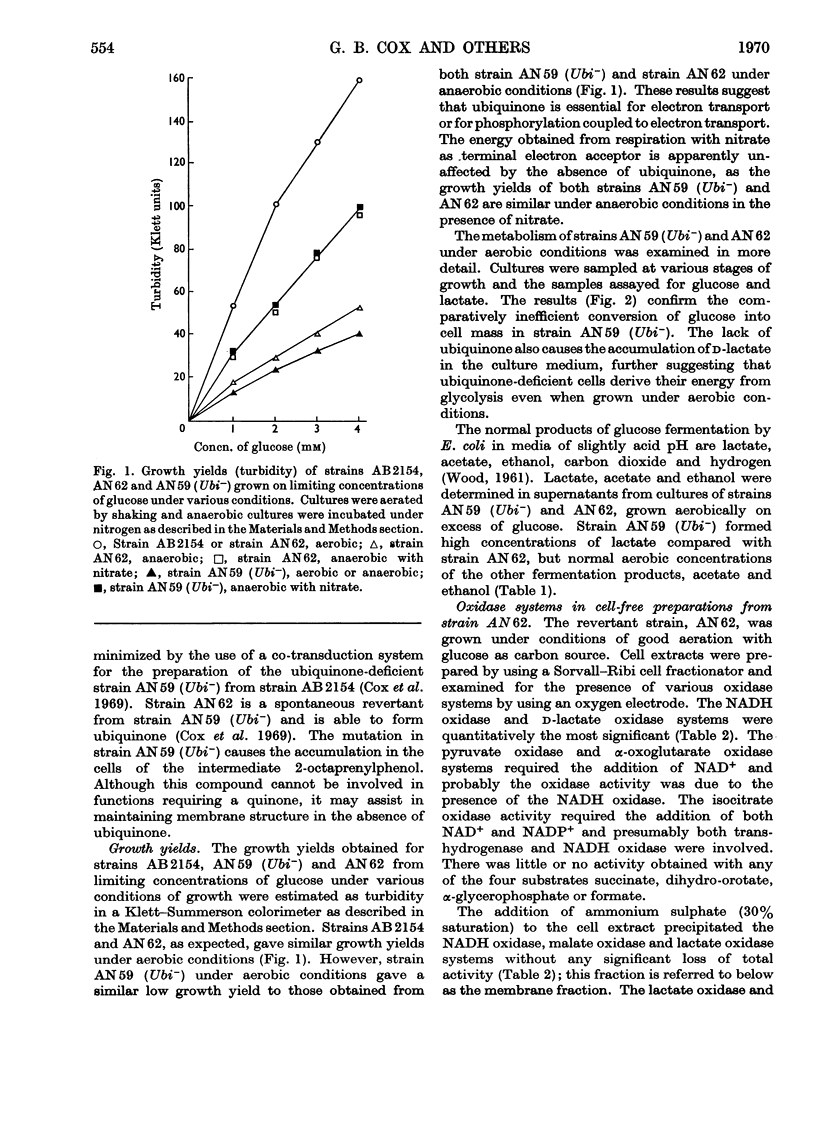
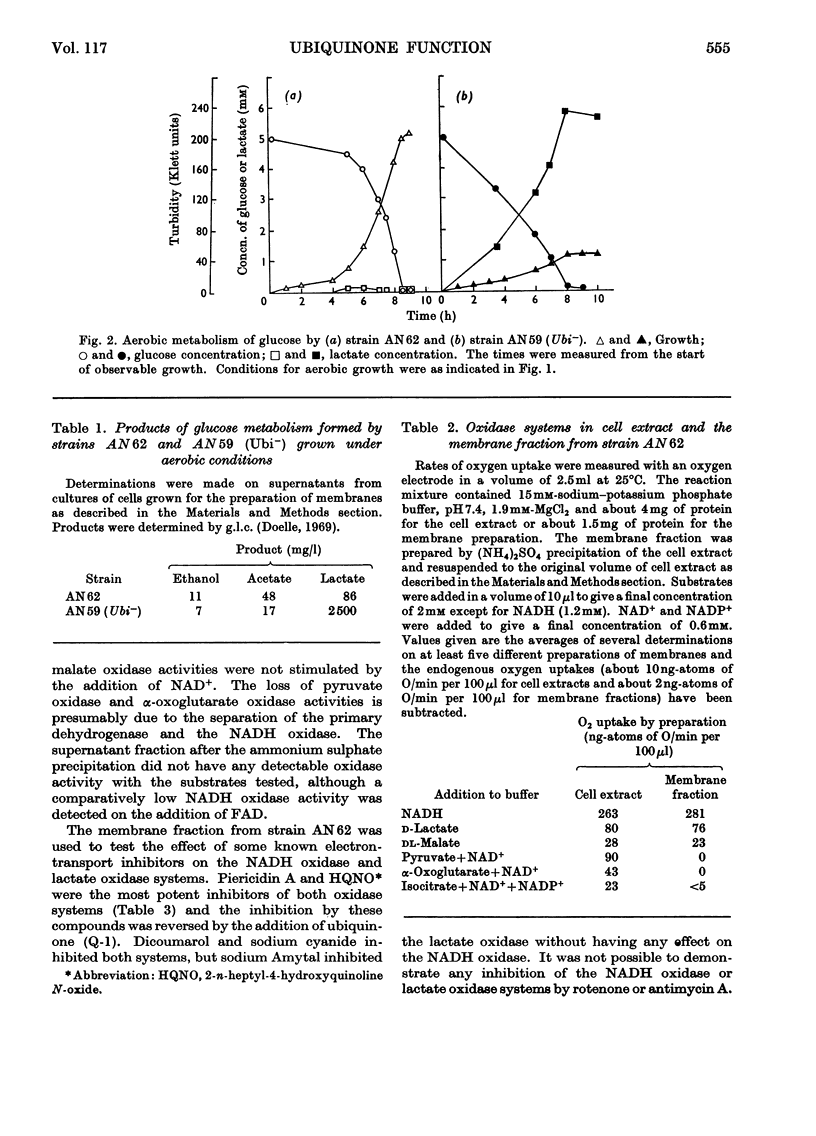
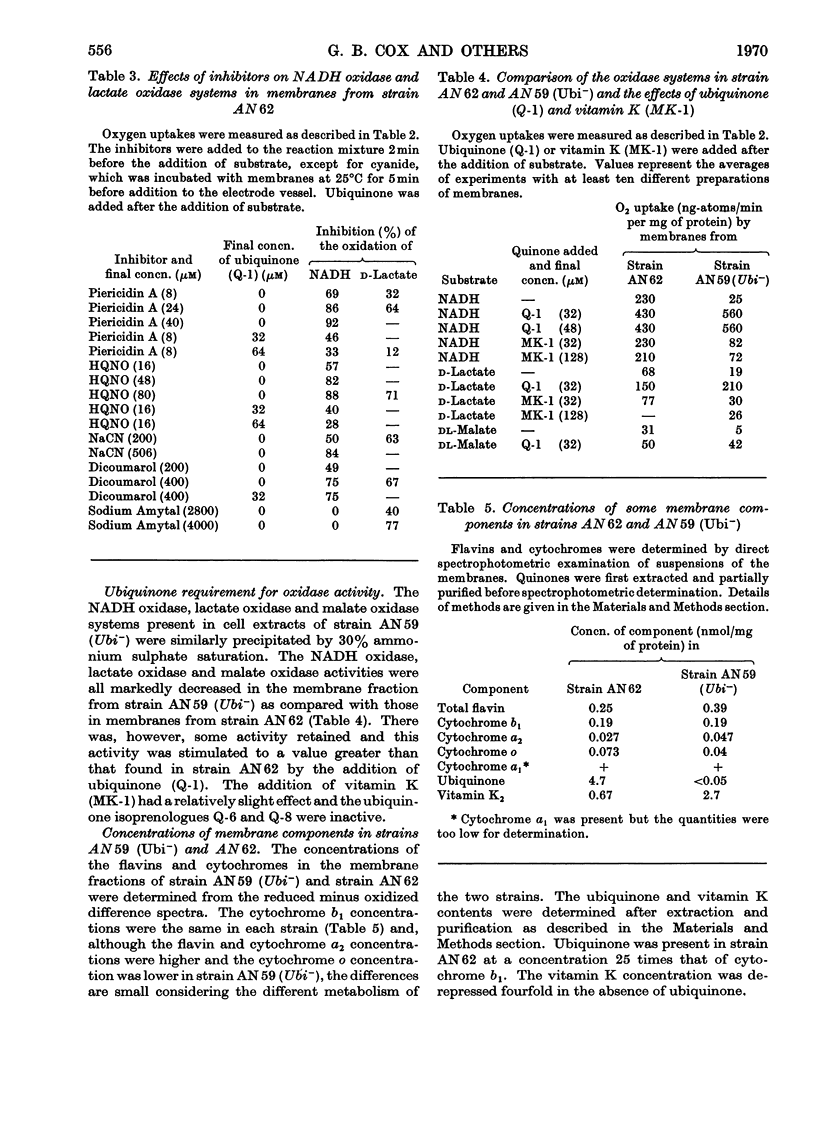
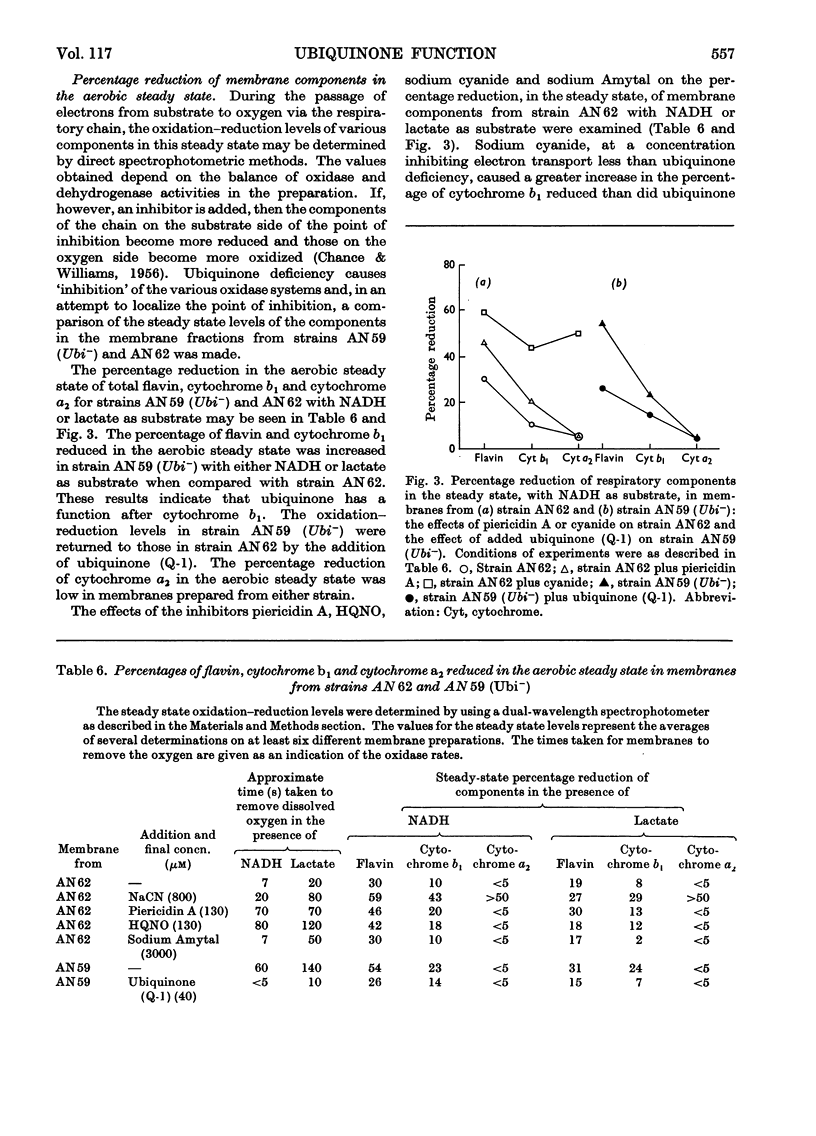
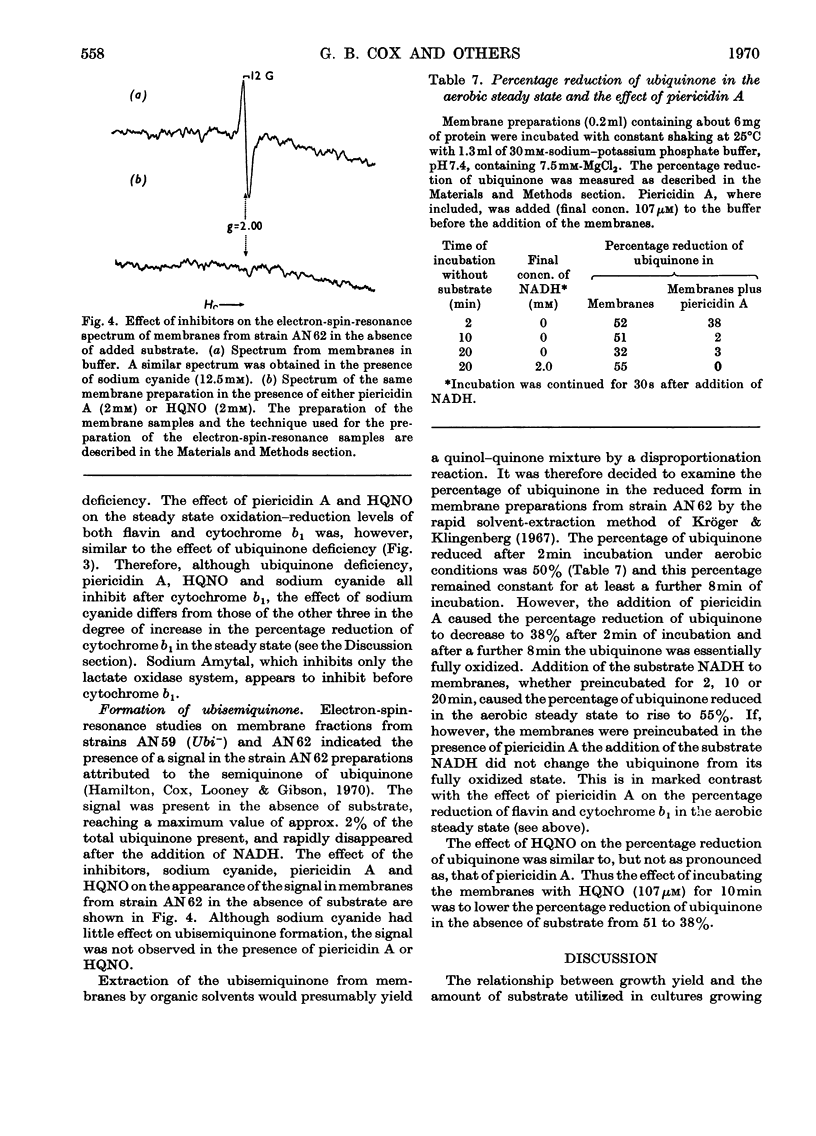
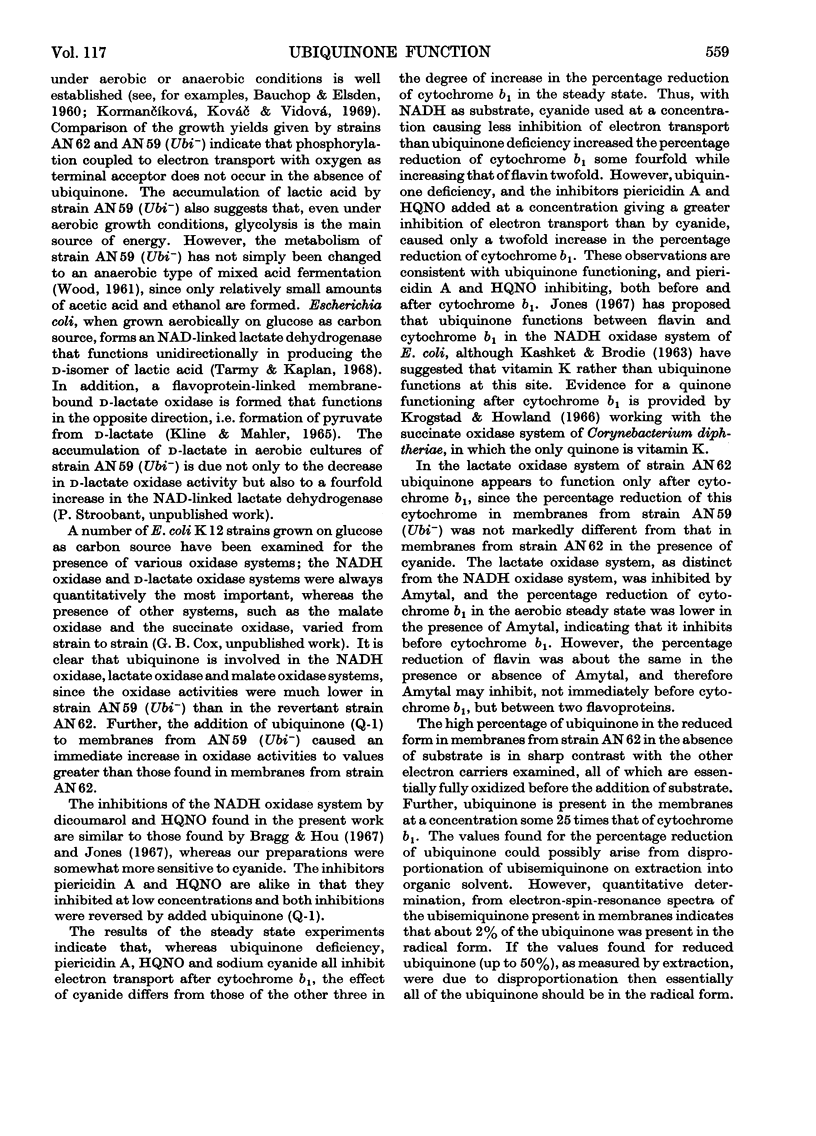
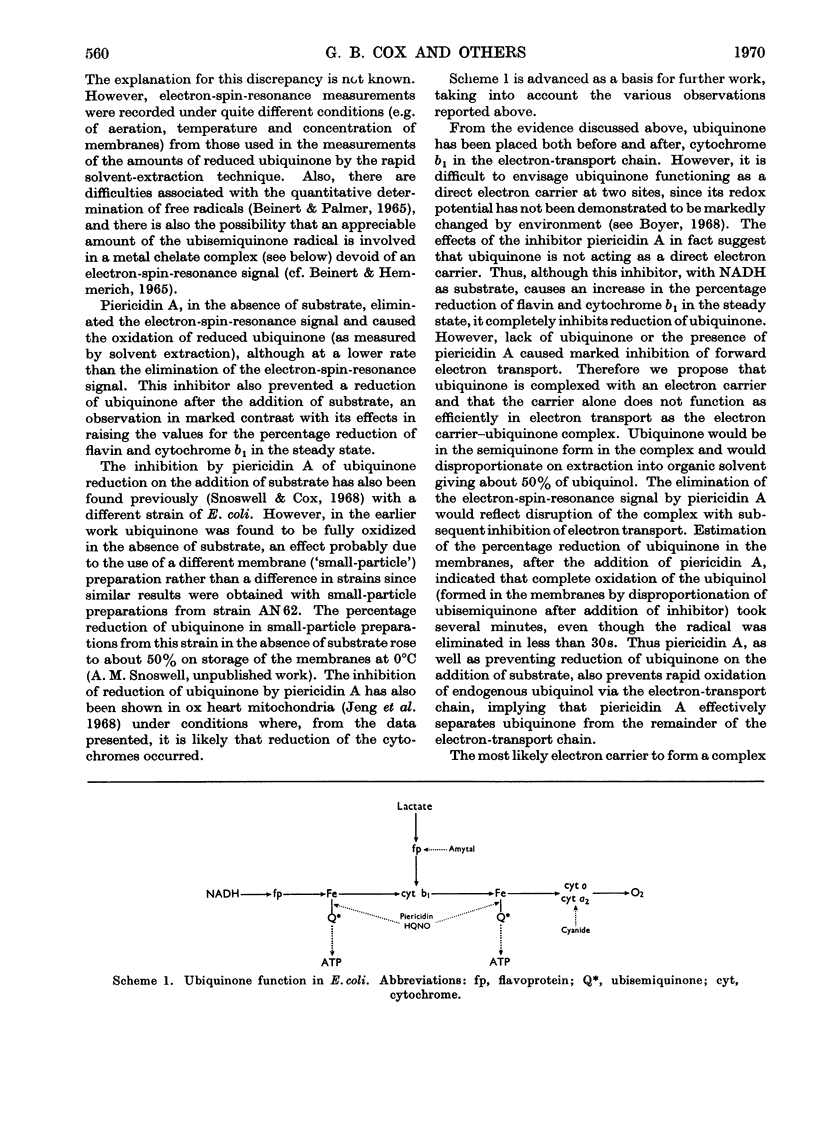
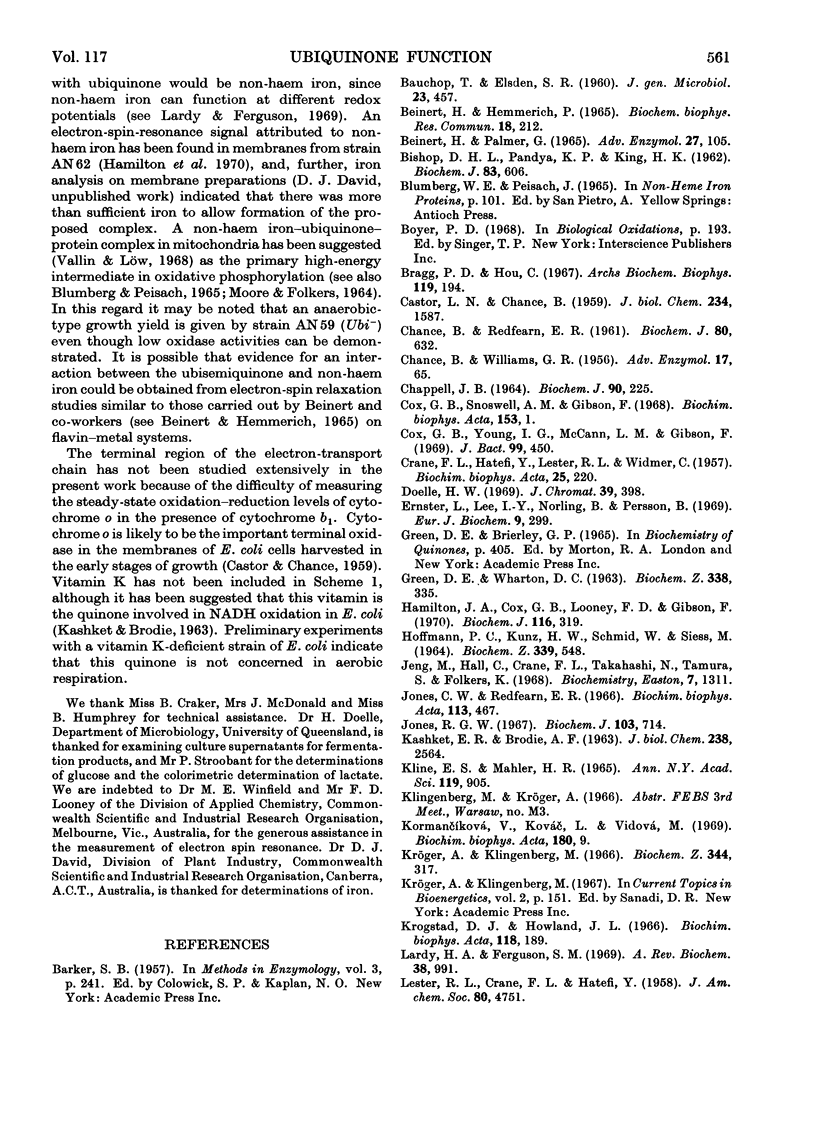
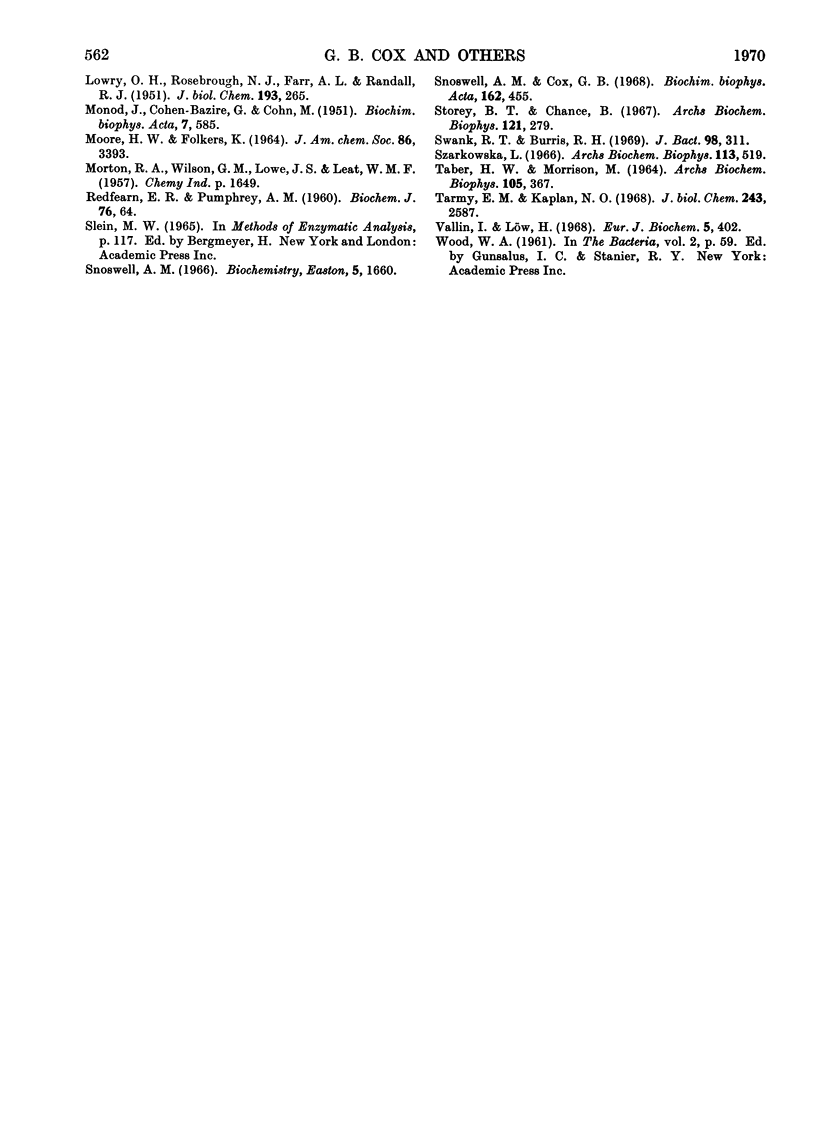
Selected References
These references are in PubMed. This may not be the complete list of references from this article.
- BAUCHOP T., ELSDEN S. R. The growth of micro-organisms in relation to their energy supply. J Gen Microbiol. 1960 Dec;23:457–469. doi: 10.1099/00221287-23-3-457. [DOI] [PubMed] [Google Scholar]
- BEINERT H., HEMMERICH P. EVIDENCE FOR SEMIQUINONE-METAL INTERACTION IN METAL-FLAVOPROTEINS. Biochem Biophys Res Commun. 1965 Jan 18;18:212–220. doi: 10.1016/0006-291x(65)90742-4. [DOI] [PubMed] [Google Scholar]
- BISHOP D. H., PANDYA K. P., KING H. K. Ubiquinone and vitamin K in bacteria. Biochem J. 1962 Jun;83:606–614. doi: 10.1042/bj0830606. [DOI] [PMC free article] [PubMed] [Google Scholar]
- Beinert H., Palmer G. Contributions of EPR spectroscopy to our knowledge of oxidative enzymes. Adv Enzymol Relat Areas Mol Biol. 1965;27:105–198. doi: 10.1002/9780470122723.ch3. [DOI] [PubMed] [Google Scholar]
- Bragg P. D., Hou C. Reduced nicotinamide adenine dinucleotide oxidation in Escherichia coli particles. I. Properties and cleavage of the electron transport chain. Arch Biochem Biophys. 1967 Mar;119(1):194–201. doi: 10.1016/0003-9861(67)90446-8. [DOI] [PubMed] [Google Scholar]
- CASTOR L. N., CHANCE B. Photochemical determinations of the oxidases of bacteria. J Biol Chem. 1959 Jun;234(6):1587–1592. [PubMed] [Google Scholar]
- CHANCE B., REDFEARN E. R. Direct spectrophotometric studies of the kinetics of oxidation and reduction of ubiquinone of heart-muscle particles. Biochem J. 1961 Sep;80:632–644. doi: 10.1042/bj0800632. [DOI] [PMC free article] [PubMed] [Google Scholar]
- CHANCE B., WILLIAMS G. R. The respiratory chain and oxidative phosphorylation. Adv Enzymol Relat Subj Biochem. 1956;17:65–134. doi: 10.1002/9780470122624.ch2. [DOI] [PubMed] [Google Scholar]
- CRANE F. L., HATEFI Y., LESTER R. L., WIDMER C. Isolation of a quinone from beef heart mitochondria. Biochim Biophys Acta. 1957 Jul;25(1):220–221. doi: 10.1016/0006-3002(57)90457-2. [DOI] [PubMed] [Google Scholar]
- Chappell J. B. The oxidation of citrate, isocitrate and cis-aconitate by isolated mitochondria. Biochem J. 1964 Feb;90(2):225–237. doi: 10.1042/bj0900225. [DOI] [PMC free article] [PubMed] [Google Scholar]
- Cox G. B., Young I. G., McCann L. M., Gibson F. Biosynthesis of ubiquinone in Escherichia coli K-12: location of genes affecting the metabolism of 3-octaprenyl-4-hydroxybenzoic acid and 2-octaprenylphenol. J Bacteriol. 1969 Aug;99(2):450–458. doi: 10.1128/jb.99.2.450-458.1969. [DOI] [PMC free article] [PubMed] [Google Scholar]
- Doelle H. W. Gas chromatographic separation and determination of micro quantities of C1-C7 branched and straight-chain saturated fatty acids. J Chromatogr. 1969 Feb 25;39(4):398–406. doi: 10.1016/s0021-9673(01)98028-8. [DOI] [PubMed] [Google Scholar]
- Ernster L., Lee I. Y., Norling B., Persson B. Studies with ubiquinone-depleted submitochondrial particles. Essentiality of ubiquinone for the interaction of succinate dehydrogenase, NADH dehydrogenase, and cytochrome b. Eur J Biochem. 1969 Jun;9(3):299–310. doi: 10.1111/j.1432-1033.1969.tb00609.x. [DOI] [PubMed] [Google Scholar]
- HOFFMANN P. C., KUNZ H. W., SCHMID W., SIESS M. THE DETERMINATION OF THE LEVEL AND REDOX QUOTIENT OF UBIQUINONE50 (COENZYME Q10) IN GUINEA PIG HEART IN VIVO. Biochem Z. 1964 Jun 16;339:548–558. [PubMed] [Google Scholar]
- Hamilton J. A., Cox G. B., Looney F. D., Gibson F. Ubisemiquinone in membranes from Escherichia coli. Biochem J. 1970 Jan;116(2):319–320. doi: 10.1042/bj1160319. [DOI] [PMC free article] [PubMed] [Google Scholar]
- Jeng M., Hall C., Crane F. L., Takahashi N., Tamura S., Folkers K. Inhibition of mitochondrial electron transport by piericidin A and related compounds. Biochemistry. 1968 Apr;7(4):1311–1322. doi: 10.1021/bi00844a010. [DOI] [PubMed] [Google Scholar]
- Jones C. W., Redfearn E. R. Electron transport in Azotobacter vinelandii. Biochim Biophys Acta. 1966 Mar 7;113(3):467–481. doi: 10.1016/s0926-6593(66)80005-x. [DOI] [PubMed] [Google Scholar]
- Jones R. G. Ubiquinone deficiency in an auxotroph of Escherichia coli requiring 4-hydroxybenzoic acid. Biochem J. 1967 Jun;103(3):714–719. doi: 10.1042/bj1030714. [DOI] [PMC free article] [PubMed] [Google Scholar]
- Kline E. S., Mahler H. R. The lactic dehydrogenases of E. coli. Ann N Y Acad Sci. 1965 Jul 31;119(3):905–919. doi: 10.1111/j.1749-6632.1965.tb47451.x. [DOI] [PubMed] [Google Scholar]
- Krogstad D. J., Howland J. L. Role of menaquinone in Corynebacterium diphtheriae electron transport. Biochim Biophys Acta. 1966 Apr 12;118(1):189–191. doi: 10.1016/s0926-6593(66)80155-8. [DOI] [PubMed] [Google Scholar]
- LOWRY O. H., ROSEBROUGH N. J., FARR A. L., RANDALL R. J. Protein measurement with the Folin phenol reagent. J Biol Chem. 1951 Nov;193(1):265–275. [PubMed] [Google Scholar]
- Lardy H. A., Ferguson S. M. Oxidative phosphorylation in mitochondria. Annu Rev Biochem. 1969;38:991–1034. doi: 10.1146/annurev.bi.38.070169.005015. [DOI] [PubMed] [Google Scholar]
- MONOD J., COHEN-BAZIRE G., COHN M. Sur la biosynthèse de la beta-galactosidase (lactase) chez Escherichia coli; la spécificité de l'induction. Biochim Biophys Acta. 1951 Nov;7(4):585–599. doi: 10.1016/0006-3002(51)90072-8. [DOI] [PubMed] [Google Scholar]
- REDFEARN E. R., PUMPHREY A. M. The kinetics of ubiquinone reactions in heart-muscle preparations. Biochem J. 1960 Jul;76:64–71. doi: 10.1042/bj0760064. [DOI] [PMC free article] [PubMed] [Google Scholar]
- Snoswell A. M., Cox G. B. Piericidin A and inhibition of respiratory chain activity in Escherichia coli K12. Biochim Biophys Acta. 1968 Oct 1;162(3):455–458. doi: 10.1016/0005-2728(68)90132-1. [DOI] [PubMed] [Google Scholar]
- Snoswell A. M. Respiration-dependent proton movements in rat liver mitochondria. Biochemistry. 1966 May;5(5):1660–1666. doi: 10.1021/bi00869a031. [DOI] [PubMed] [Google Scholar]
- Storey B. T., Chance B. Rate of reduction of ubiquinone by NADH in electron transport particles. Arch Biochem Biophys. 1967 Aug;121(2):279–289. doi: 10.1016/0003-9861(67)90077-x. [DOI] [PubMed] [Google Scholar]
- Swank R. T., Burris R. H. Restoration by ubiquinone of Azotobacter vinelandii reduced nicotinamide adenine dinucleotide oxidase activity. J Bacteriol. 1969 Apr;98(1):311–313. doi: 10.1128/jb.98.1.311-313.1969. [DOI] [PMC free article] [PubMed] [Google Scholar]
- Szarkowska L. The restoration of DPNH oxidase activity by coenzyme Q (ubiquinone). Arch Biochem Biophys. 1966 Mar;113(3):519–525. doi: 10.1016/0003-9861(66)90228-1. [DOI] [PubMed] [Google Scholar]
- TABER H. W., MORRISON M. ELECTRON TRANSPORT IN STAPHYLOCOCCI. PROPERTIES OF A PARTICLE PREPARATION FROM EXPONENTIAL PHASE STAPHYLOCOCCUS AUREUS. Arch Biochem Biophys. 1964 May;105:367–379. doi: 10.1016/0003-9861(64)90021-9. [DOI] [PubMed] [Google Scholar]
- Tarmy E. M., Kaplan N. O. Kinetics of Escherichia coli B D-lactate dehydrogenase and evidence for pyruvate-controlled change in conformation. J Biol Chem. 1968 May 25;243(10):2587–2596. [PubMed] [Google Scholar]
- Vallin I., Löw H. The effect of piericidin A on energy-linked processes in submitochondrial particles. Eur J Biochem. 1968 Aug;5(3):402–408. [PubMed] [Google Scholar]