Abstract
1. A method is described for producing high yields of calcium-tolerant ventricular myocytes from guinea-pig hearts (73.4% rod-shaped cells, n = 19). Their action potential (AP) and membrane currents were recorded using conventional microelectrodes and cell shortening was measured optically using a linear photodiode array. 2. The sensitivity of the guinea-pig Na(+)-K+ pump to strophanthidin (a rapidly acting digitalis analogue) was determined by measuring the inhibition of outward pump current by different doses. The pump was found to have a dissociation constant (KD) for strophanthidin of 1.11 x 10(-5) M, and 5 x 10(-4) M-strophanthidin inhibited the pump maximally. 3. Exposure to strophanthidin resulted in an initial lengthening followed by a shortening of the AP, and an increased contraction. Initial AP lengthening was associated with a more positive AP plateau which became more negative as the AP shortened. 4. There was a reversible reduction of Ca2+ current (ICa) during exposure to strophanthidin. ICa changed reciprocally with contraction and with a similar time course. 5. Strophanthidin exposure caused a reduction of ICa at all activating voltages, suggesting that it resulted in a reduction of Ca2+ conductance with little change of its voltage dependence. 6. The role of an increase of intracellular calcium (Cai2+) was investigated by impaling myocytes with microelectrodes containing BAPTA 1,2-bis (2-amino-phenoxy)ethane-N,N,N',N'-tetraacetic acid, a calcium chelator) to increase Cai2+ buffering. Strophanthidin still shortened the AP when BAPTA was present, suggesting that a rise of Cai2+ is not a major cause of AP shortening. 7. Although AP shortening was little affected, the decline of ICa with strophanthidin was markedly reduced when BAPTA was present, suggesting that a rise of Cai2+ was the cause of the ICa decline with strophanthidin. 8. When barium ions carried the current through Ca2+ channels, strophanthidin did not reduce Ca2+ channel current, suggesting that this compound does not have a direct inhibitory effect on the channel. 9. The results suggest that strophanthidin causes a reduction of ICa by increasing Cai2+, via the mechanism of Cai(2+)-dependent inactivation of ICa. The reduction of ICa at least partially explains the AP shortening and more negative plateau with strophanthidin. 10. The shortening of the AP, more negative plateau and reduced ICa have negative inotropic effects which oppose the direct positive inotropic effect of strophanthidin.
Full text
PDF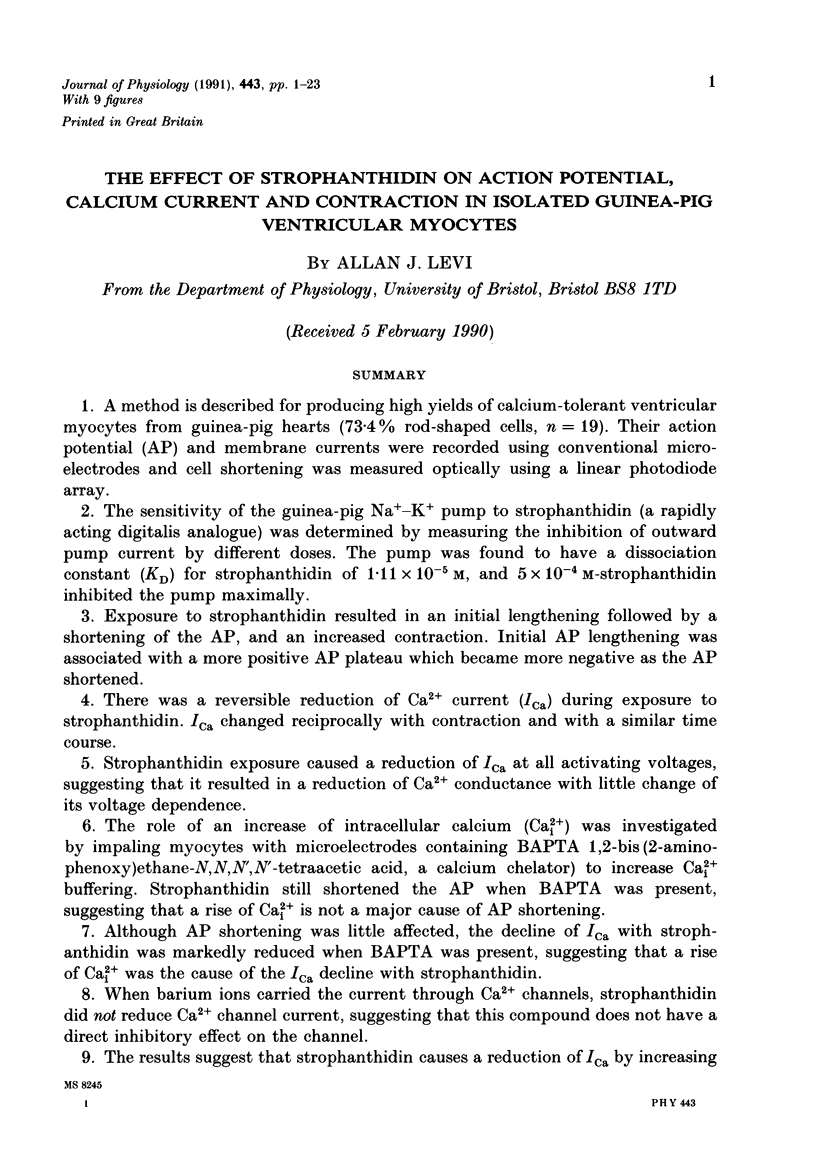
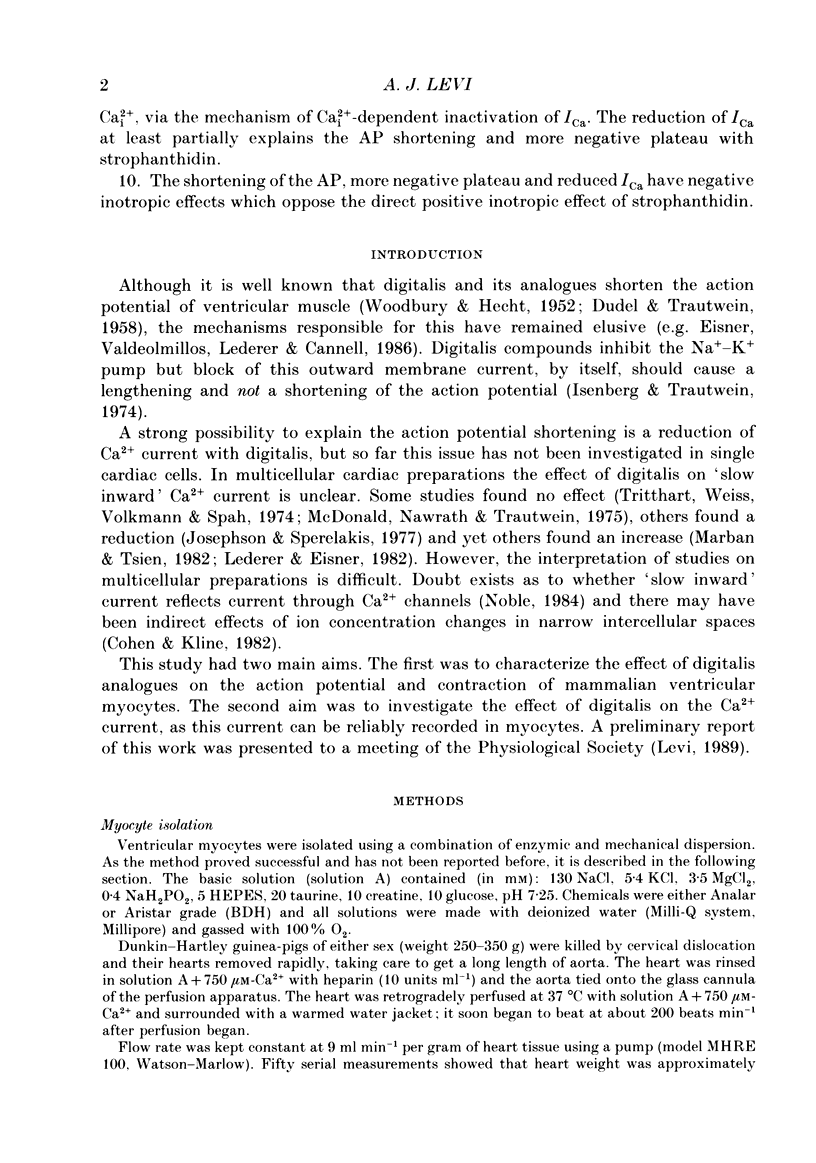
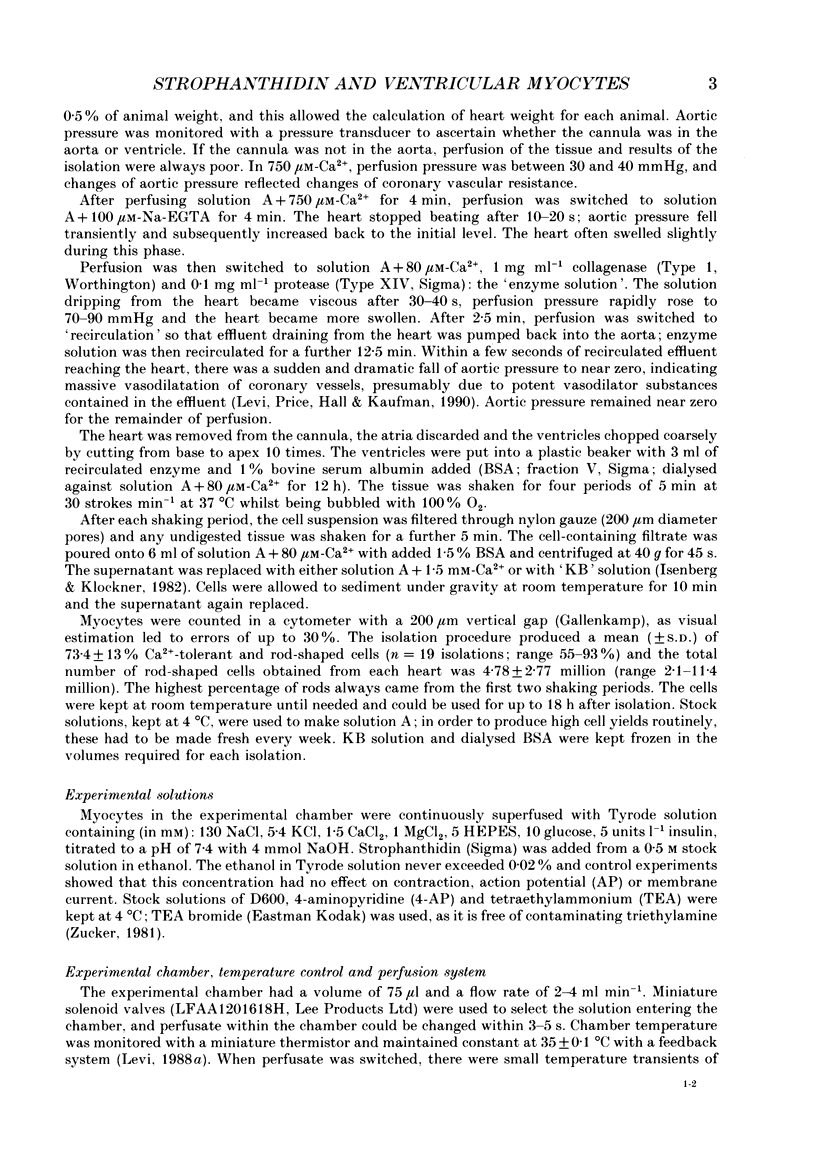
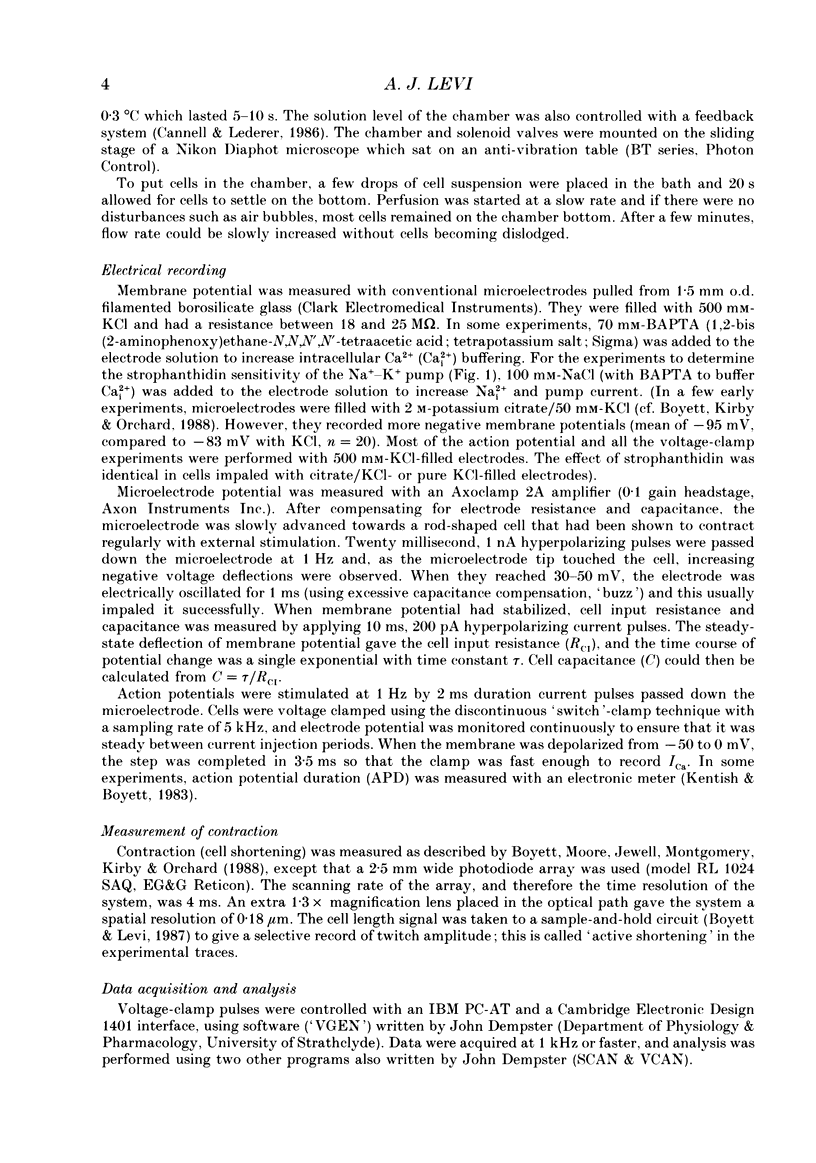
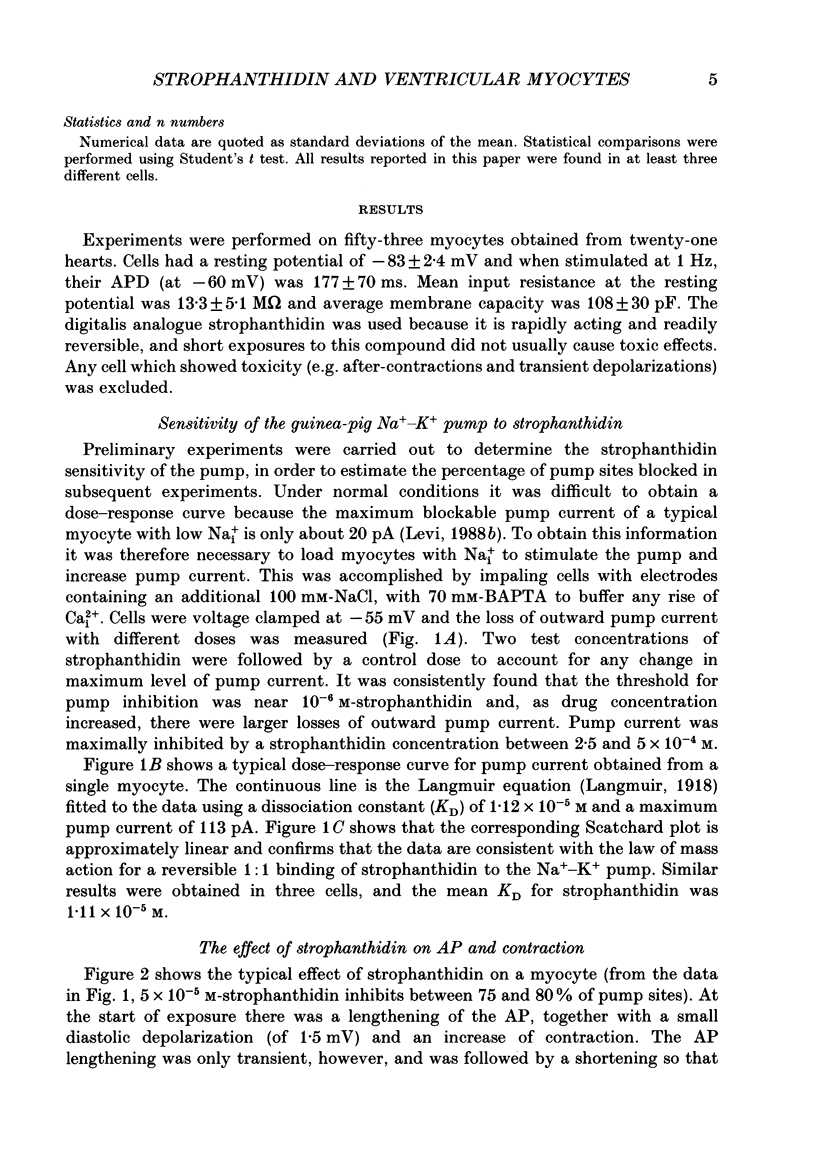
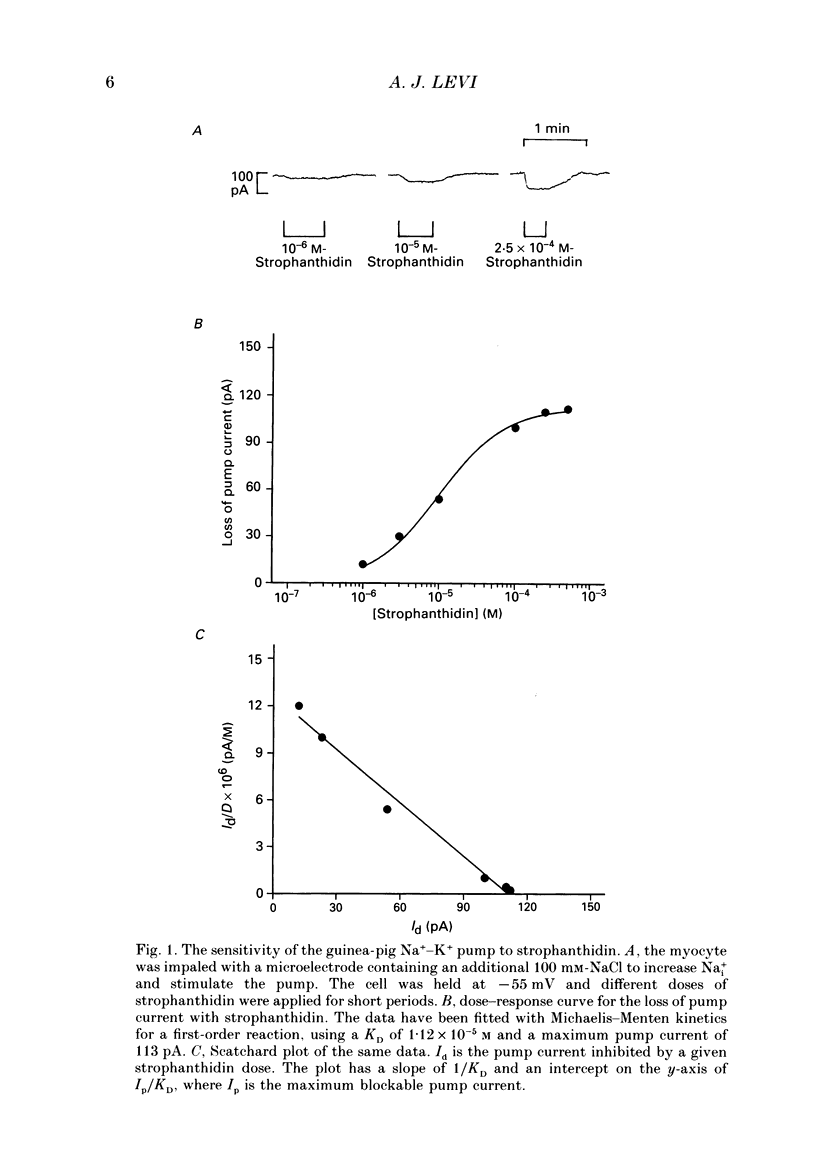
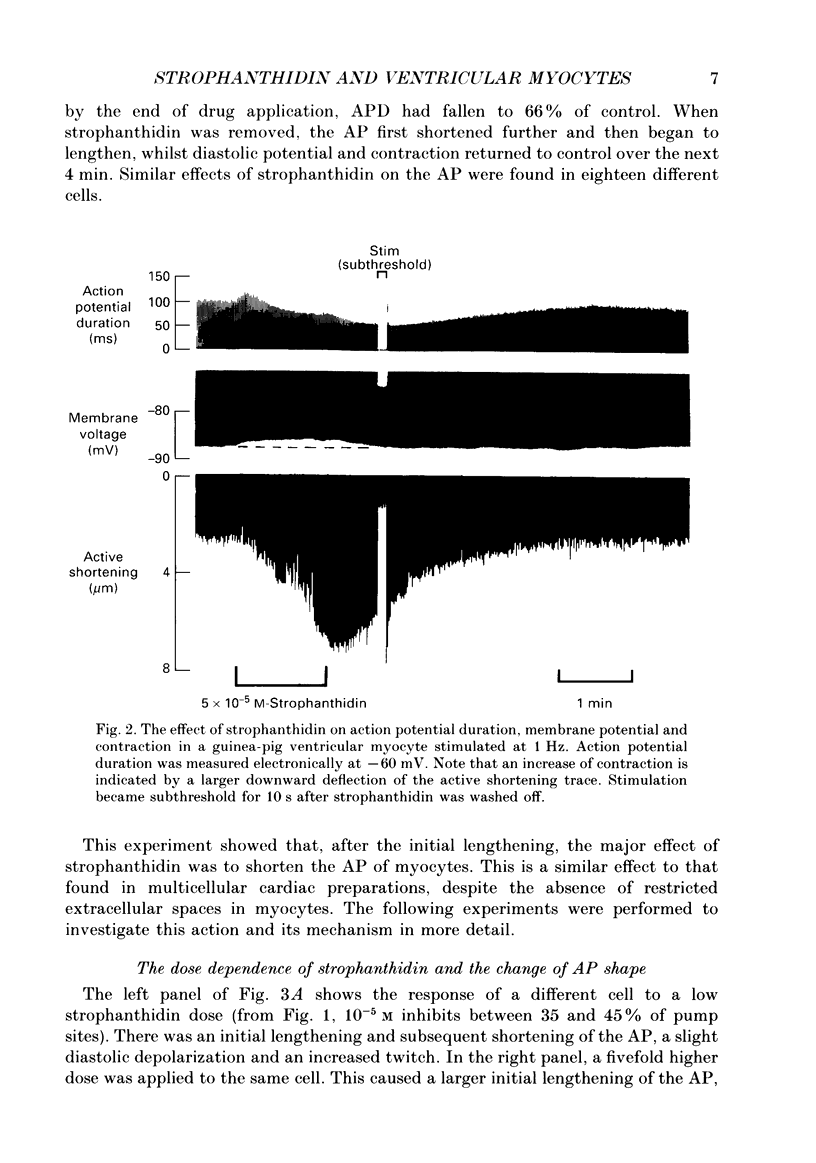
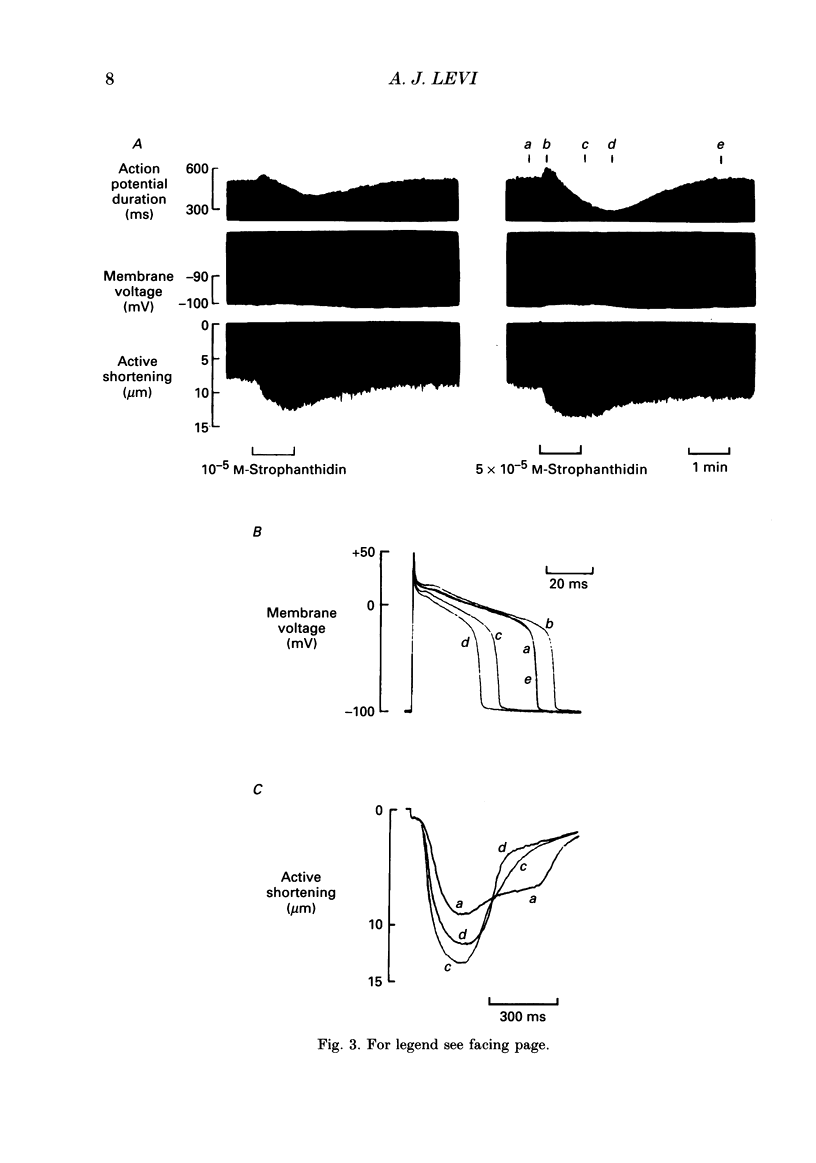
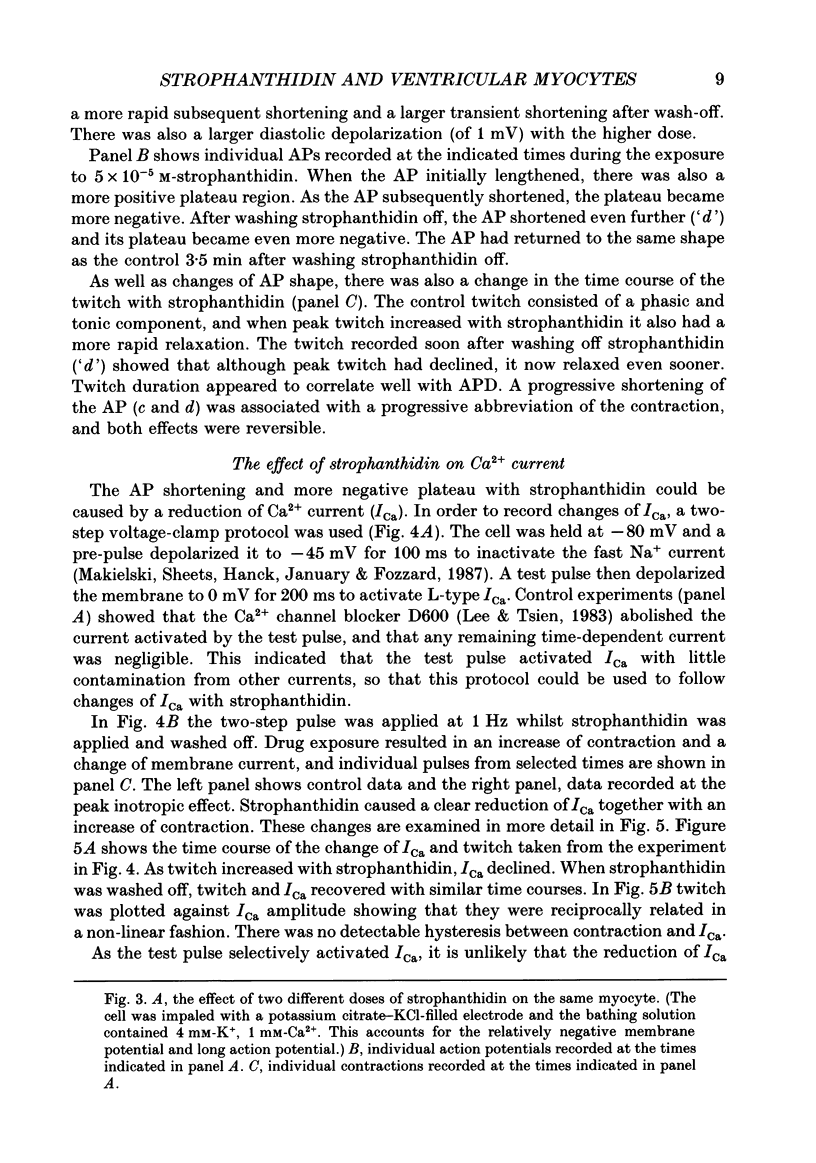
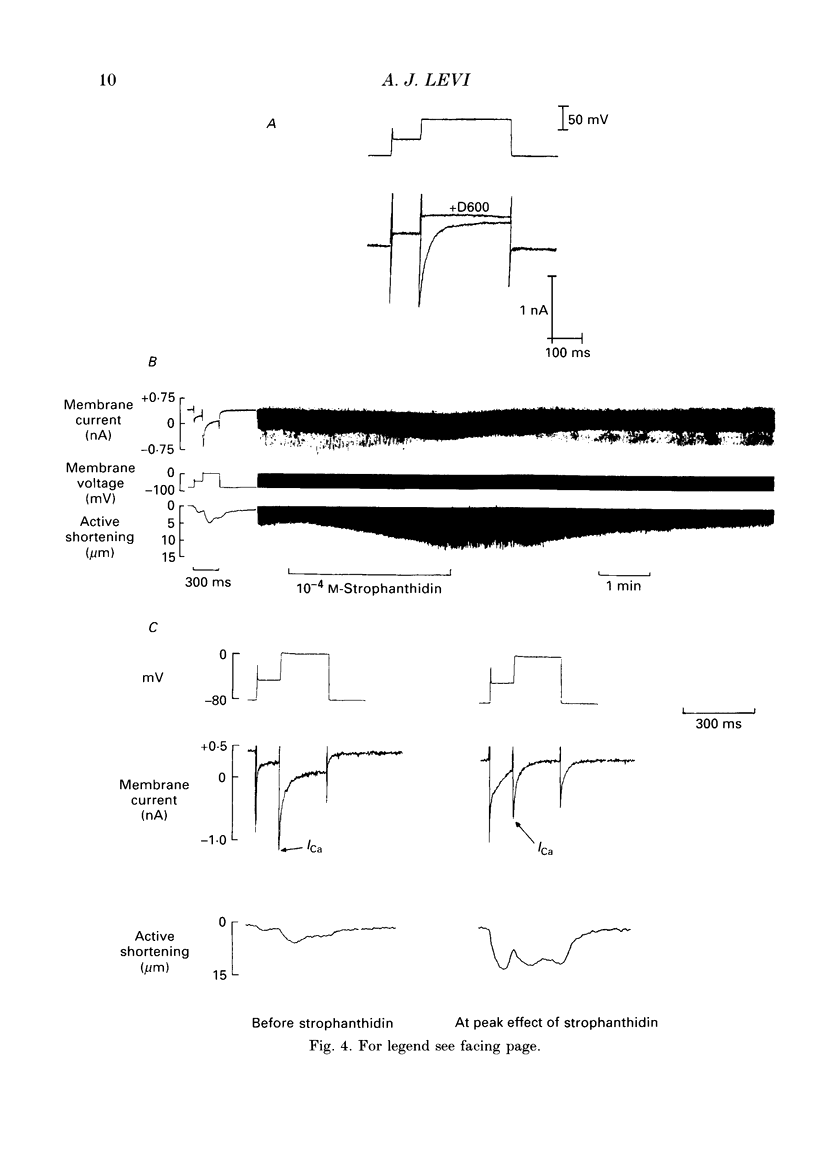
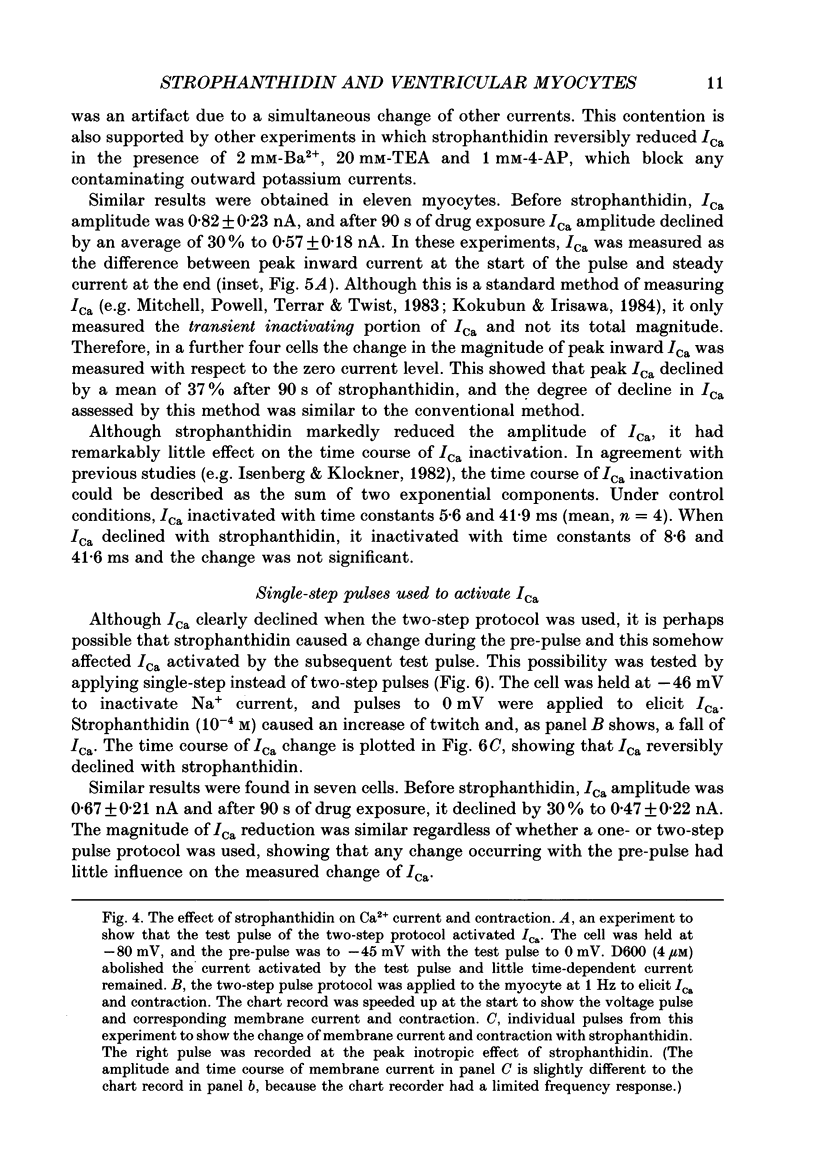
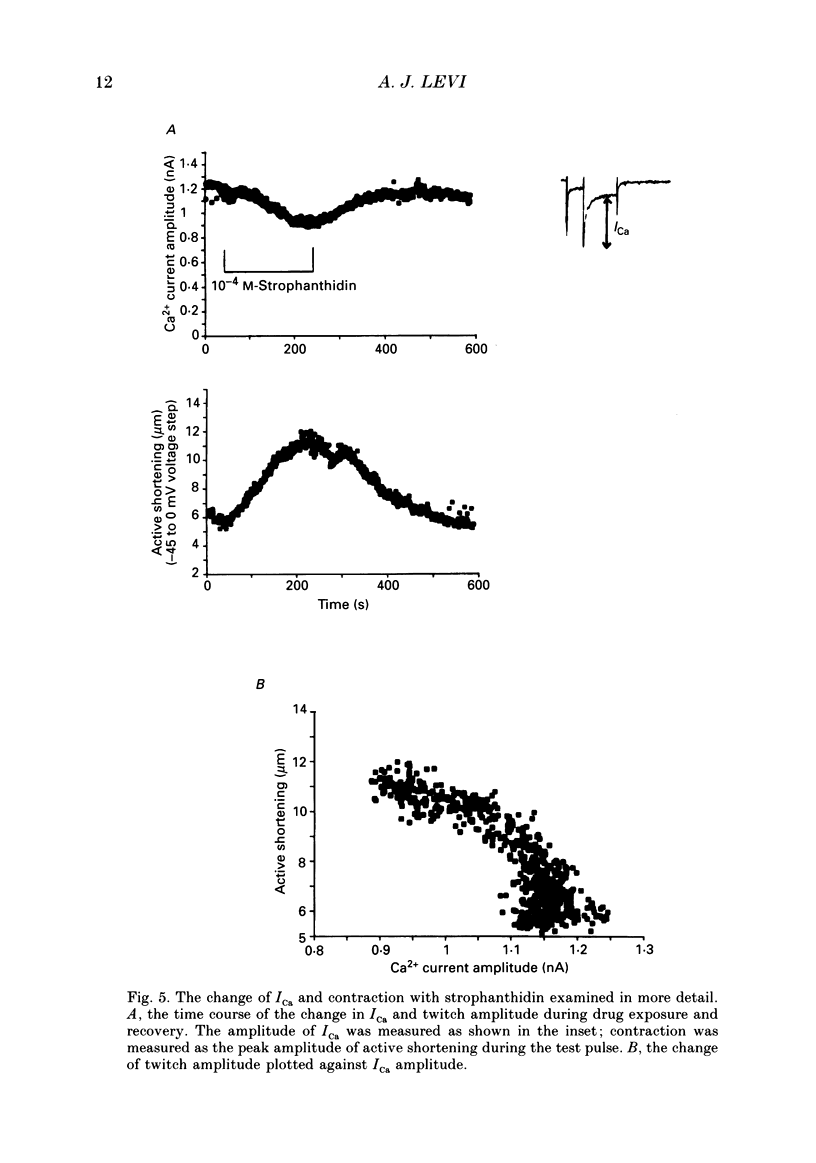
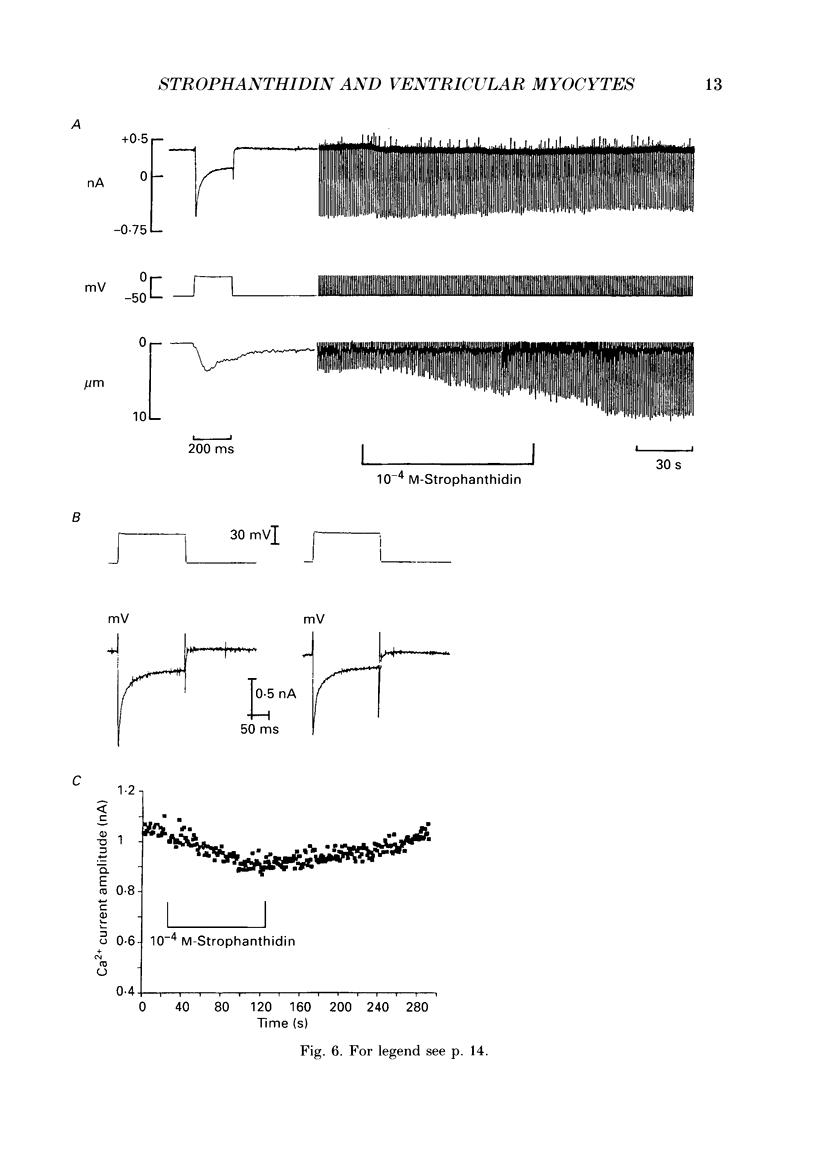
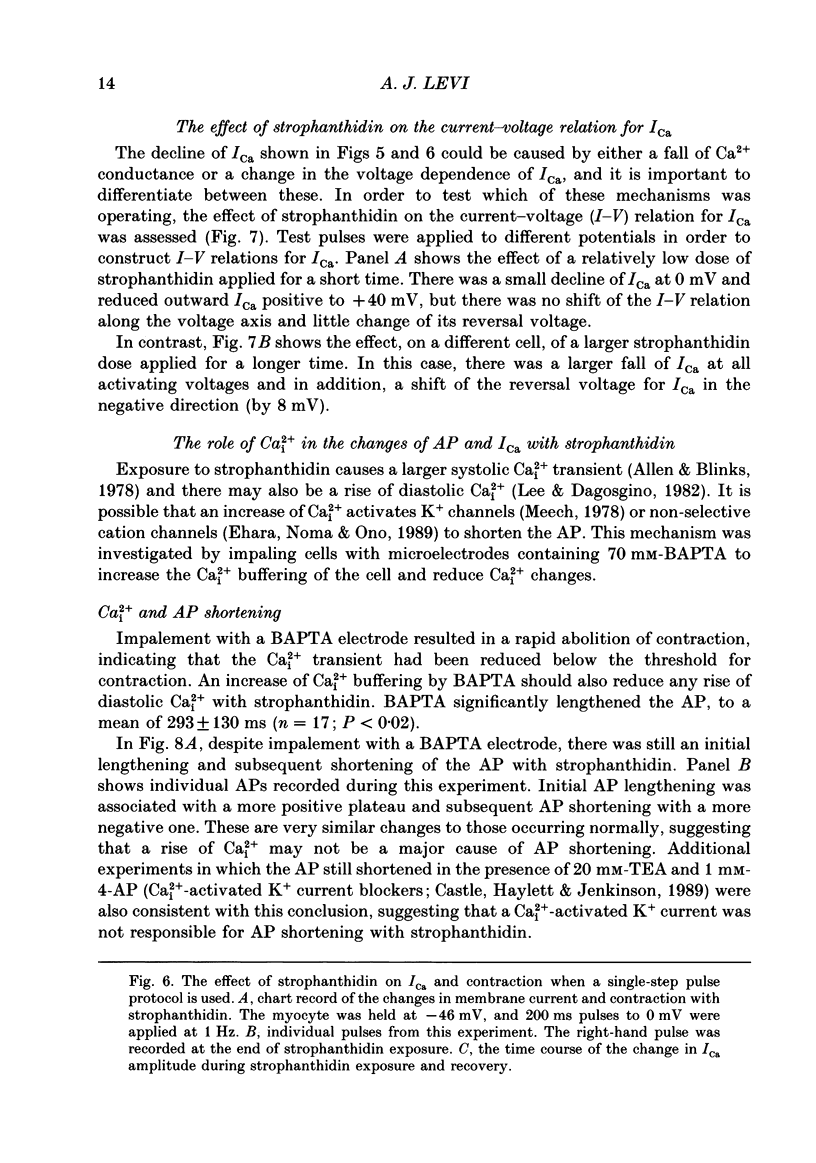
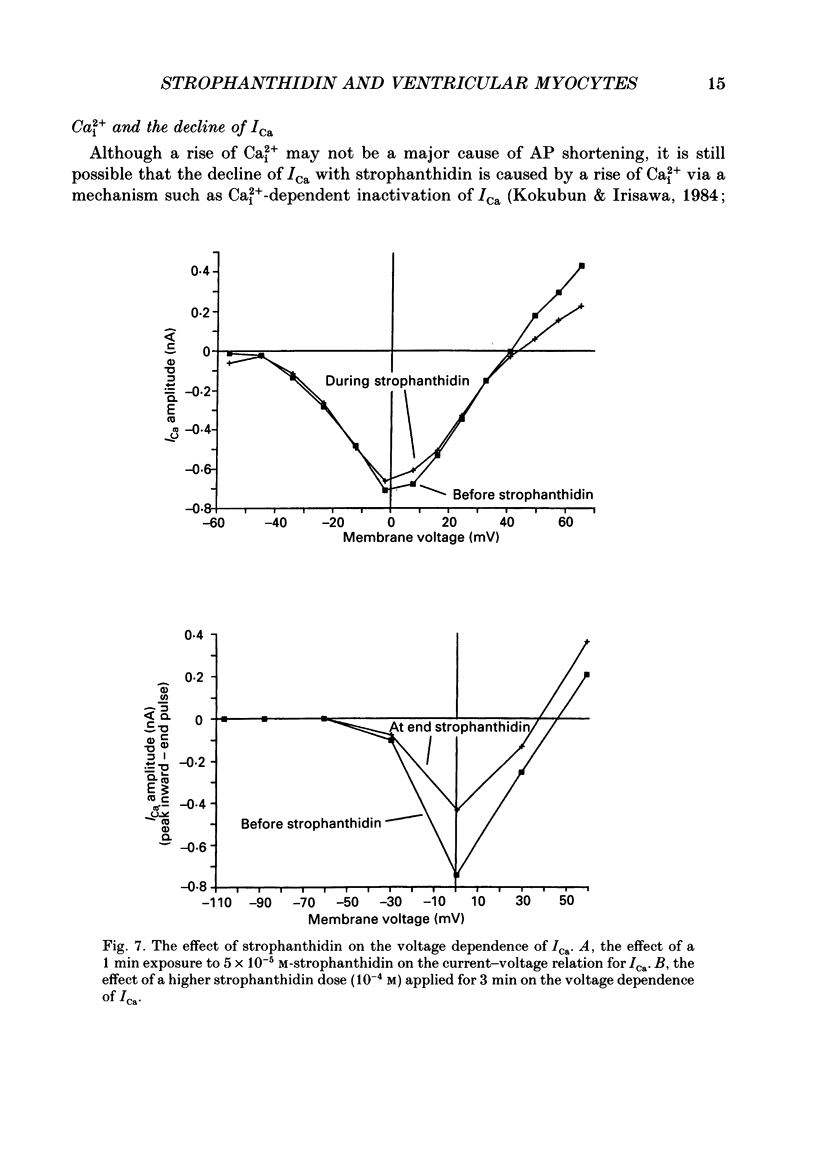
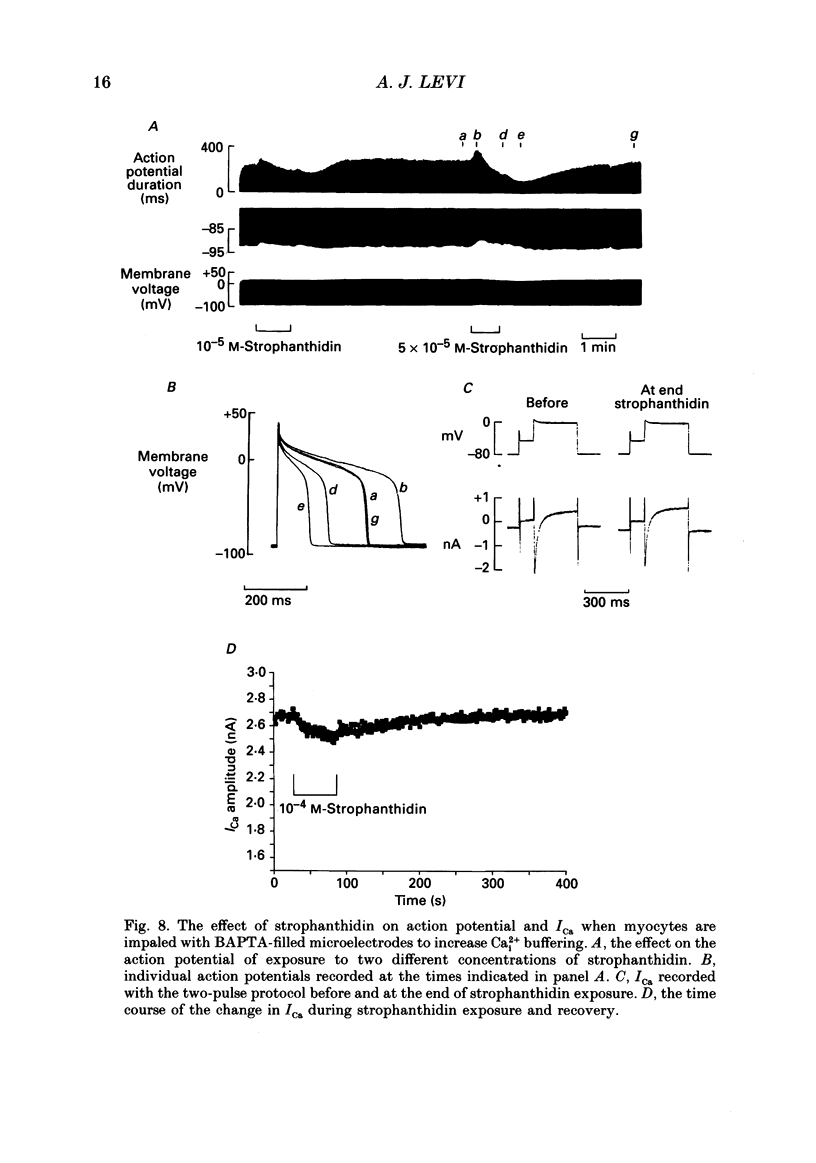
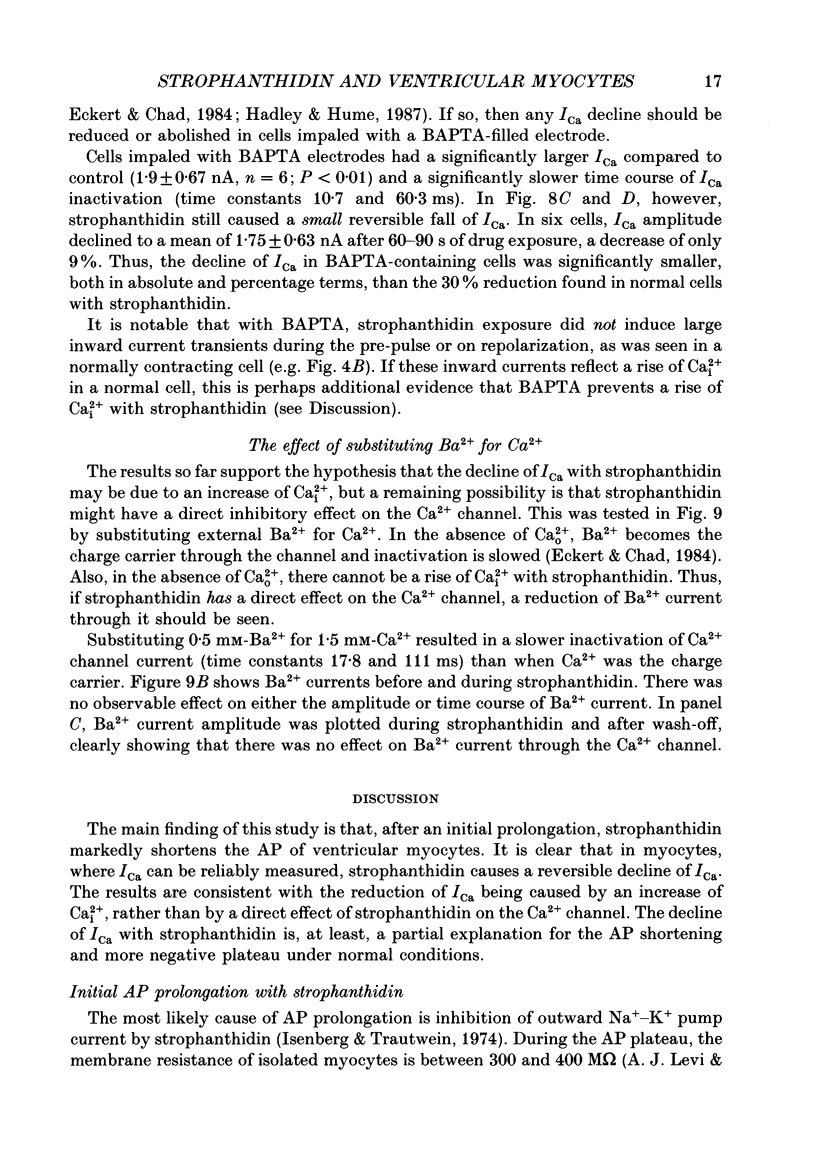
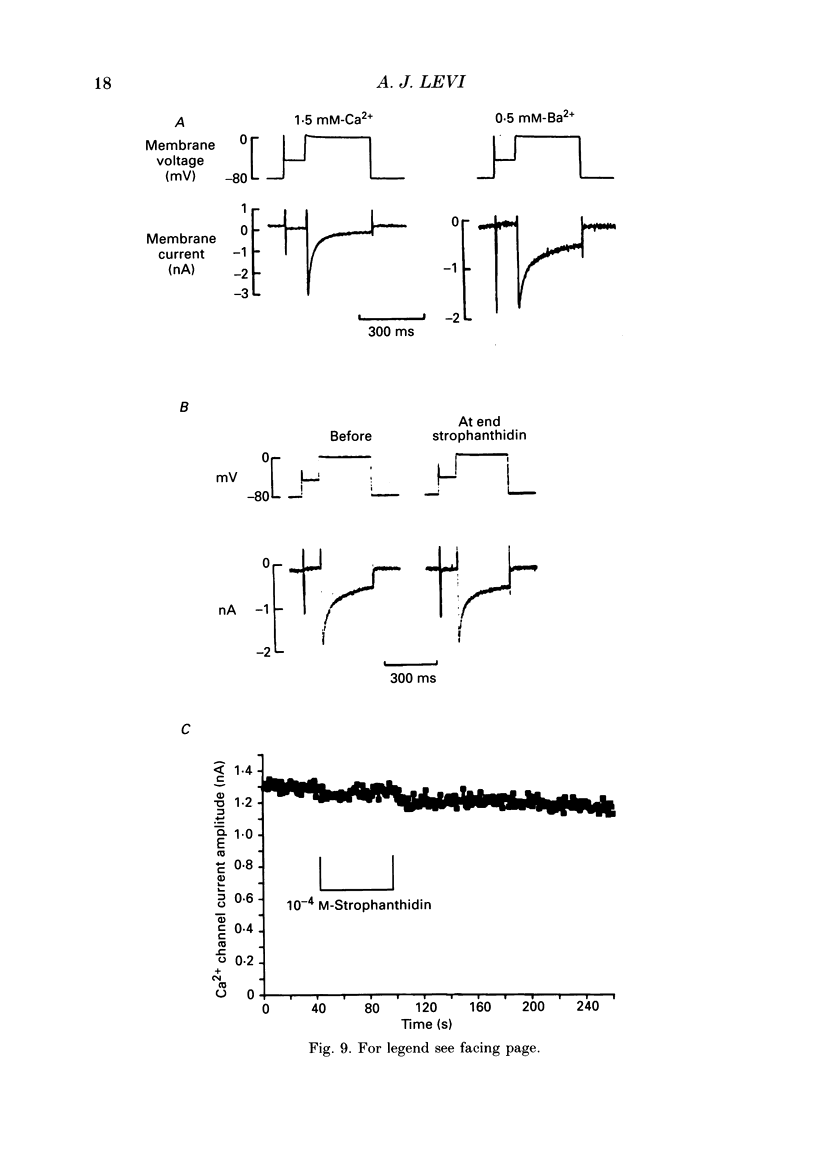
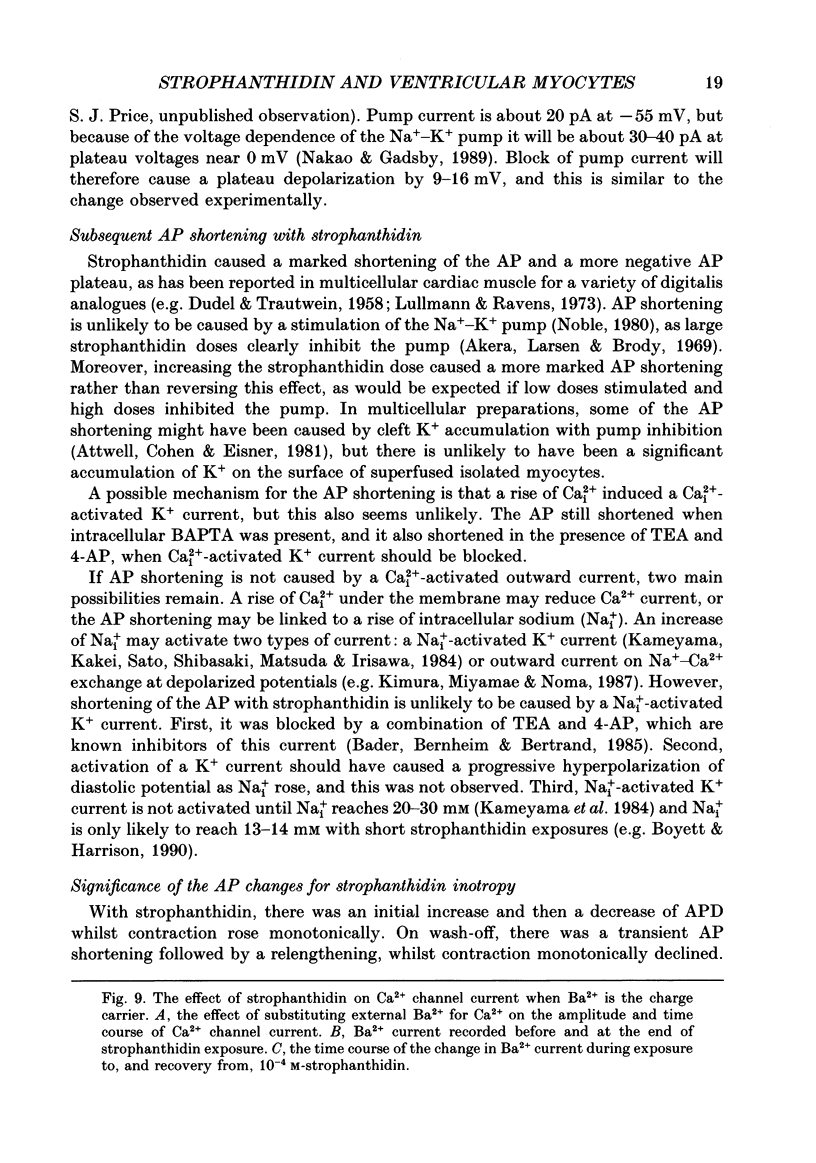
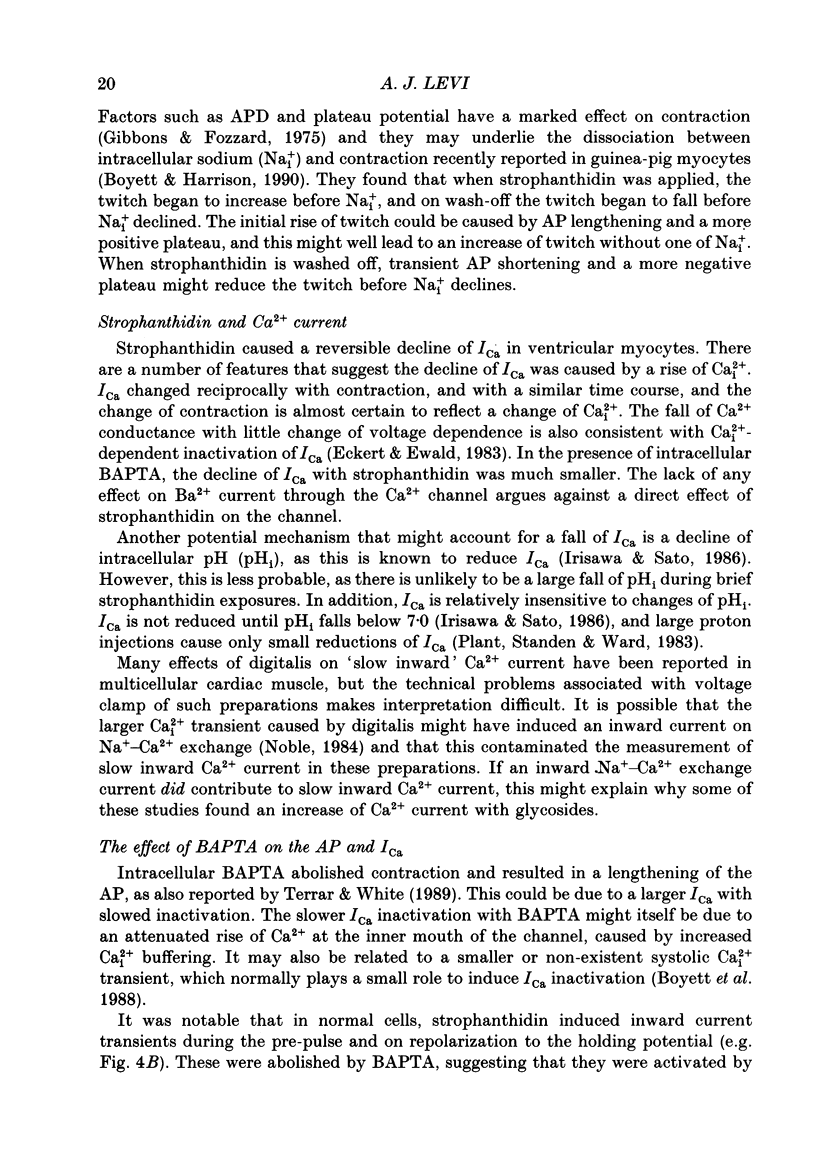
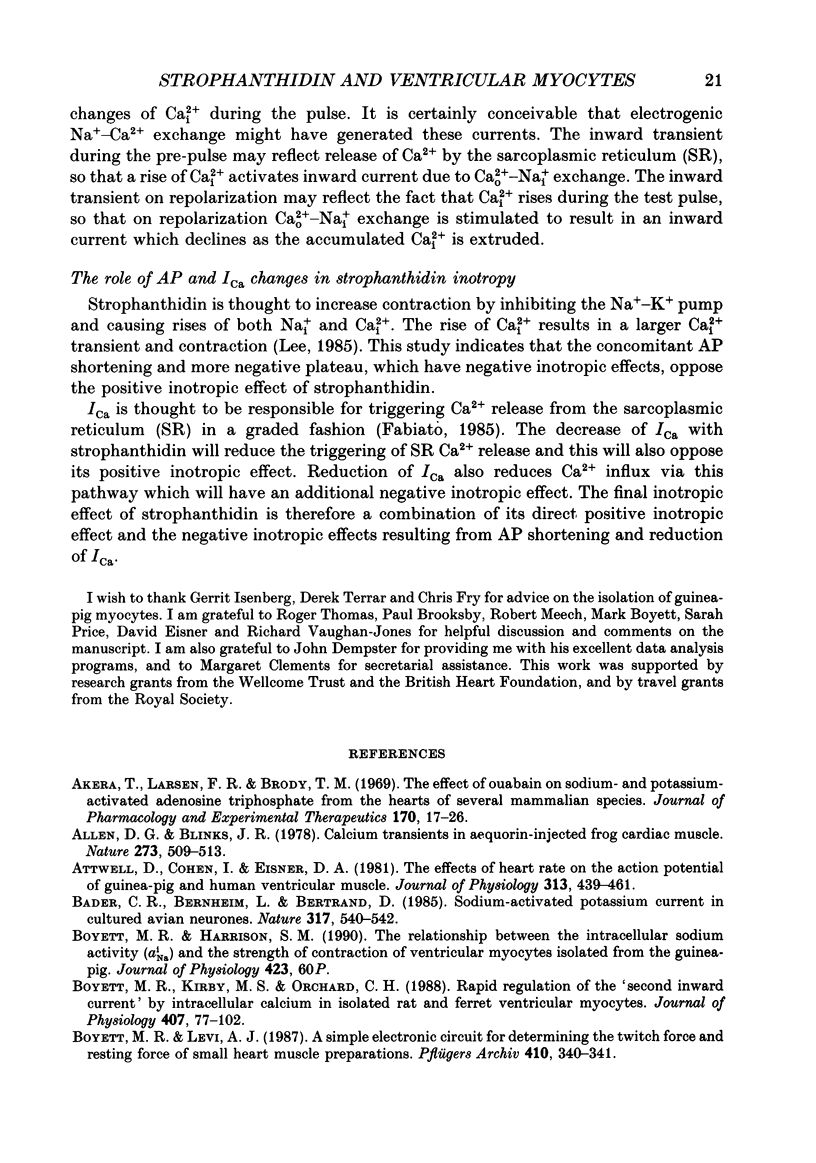
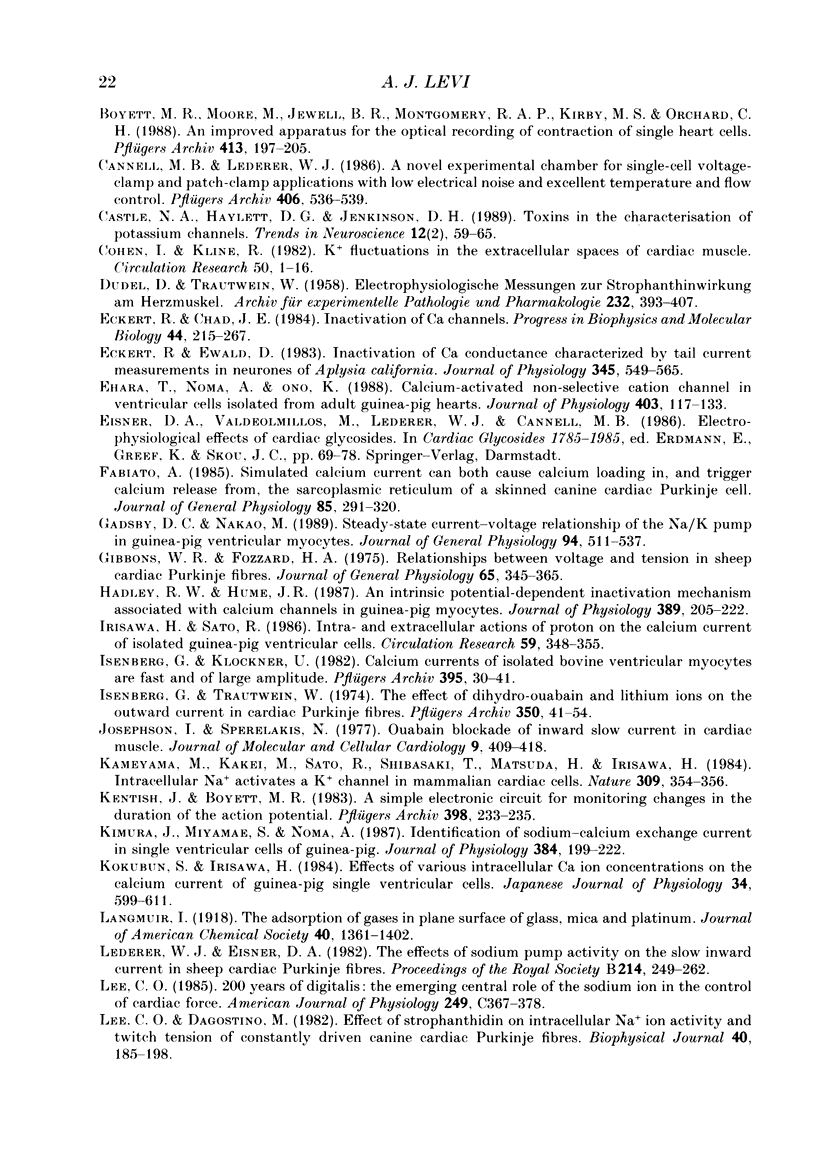
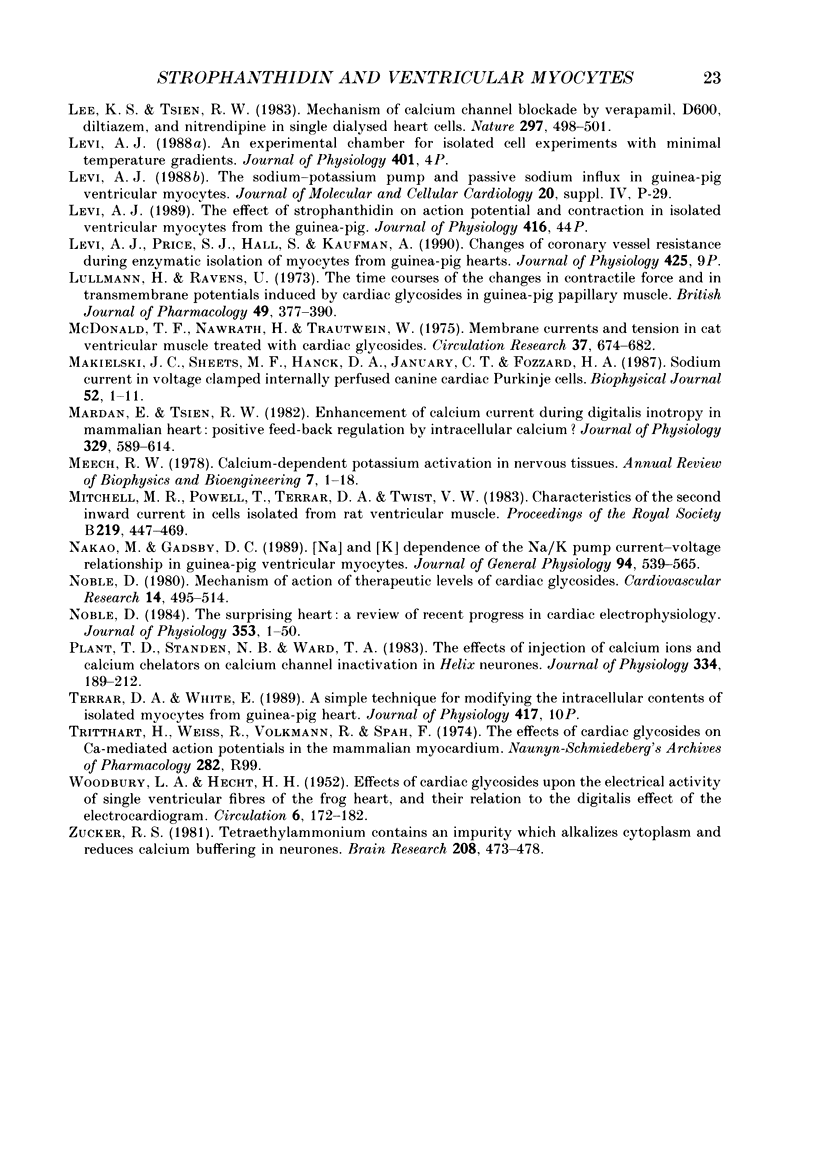
Images in this article
Selected References
These references are in PubMed. This may not be the complete list of references from this article.
- Akera T., Larsen F. S., Brody T. M. The effect of ouabain on sodium- and potassium-activated adenosine triphosphatase from the hearts of several mammalian species. J Pharmacol Exp Ther. 1969 Nov;170(1):17–26. [PubMed] [Google Scholar]
- Allen D. G., Blinks J. R. Calcium transients in aequorin-injected frog cardiac muscle. Nature. 1978 Jun 15;273(5663):509–513. doi: 10.1038/273509a0. [DOI] [PubMed] [Google Scholar]
- Attwell D., Cohen I., Eisner D. A. The effects of heart rate on the action potential of guinea-pig and human ventricular muscle. J Physiol. 1981;313:439–461. doi: 10.1113/jphysiol.1981.sp013675. [DOI] [PMC free article] [PubMed] [Google Scholar]
- Bader C. R., Bernheim L., Bertrand D. Sodium-activated potassium current in cultured avian neurones. Nature. 1985 Oct 10;317(6037):540–542. doi: 10.1038/317540a0. [DOI] [PubMed] [Google Scholar]
- Boyett M. R., Kirby M. S., Orchard C. H. Rapid regulation of the 'second inward current' by intracellular calcium in isolated rat and ferret ventricular myocytes. J Physiol. 1988 Dec;407:77–102. doi: 10.1113/jphysiol.1988.sp017404. [DOI] [PMC free article] [PubMed] [Google Scholar]
- Boyett M. R., Levi A. J. A simple electronic circuit for determining the twitch force and resting force of small heart muscle preparations. Pflugers Arch. 1987 Oct;410(3):340–341. doi: 10.1007/BF00580287. [DOI] [PubMed] [Google Scholar]
- Boyett M. R., Moore M., Jewell B. R., Montgomery R. A., Kirby M. S., Orchard C. H. An improved apparatus for the optical recording of contraction of single heart cells. Pflugers Arch. 1988 Dec;413(2):197–205. doi: 10.1007/BF00582531. [DOI] [PubMed] [Google Scholar]
- Cannell M. B., Lederer W. J. A novel experimental chamber for single-cell voltage-clamp and patch-clamp applications with low electrical noise and excellent temperature and flow control. Pflugers Arch. 1986 May;406(5):536–539. doi: 10.1007/BF00583378. [DOI] [PubMed] [Google Scholar]
- Castle N. A., Haylett D. G., Jenkinson D. H. Toxins in the characterization of potassium channels. Trends Neurosci. 1989 Feb;12(2):59–65. doi: 10.1016/0166-2236(89)90137-9. [DOI] [PubMed] [Google Scholar]
- Cohen I., Kline R. K+ fluctuations in the extracellular spaces of cardiac muscle. Evidence from the voltage clamp and extracellular K+ - selective microelectrodes. Circ Res. 1982 Jan;50(1):1–16. [PubMed] [Google Scholar]
- DUDEL J., TRAUTWEIN W. Elektrophysiologiche Messungen zur Strophanthinwirkung am Herzmuskel. Naunyn Schmiedebergs Arch Exp Pathol Pharmakol. 1958;232(2):393–407. [PubMed] [Google Scholar]
- Eckert R., Chad J. E. Inactivation of Ca channels. Prog Biophys Mol Biol. 1984;44(3):215–267. doi: 10.1016/0079-6107(84)90009-9. [DOI] [PubMed] [Google Scholar]
- Eckert R., Ewald D. Inactivation of calcium conductance characterized by tail current measurements in neurones of Aplysia californica. J Physiol. 1983 Dec;345:549–565. doi: 10.1113/jphysiol.1983.sp014996. [DOI] [PMC free article] [PubMed] [Google Scholar]
- Ehara T., Noma A., Ono K. Calcium-activated non-selective cation channel in ventricular cells isolated from adult guinea-pig hearts. J Physiol. 1988 Sep;403:117–133. doi: 10.1113/jphysiol.1988.sp017242. [DOI] [PMC free article] [PubMed] [Google Scholar]
- Fabiato A. Simulated calcium current can both cause calcium loading in and trigger calcium release from the sarcoplasmic reticulum of a skinned canine cardiac Purkinje cell. J Gen Physiol. 1985 Feb;85(2):291–320. doi: 10.1085/jgp.85.2.291. [DOI] [PMC free article] [PubMed] [Google Scholar]
- Gadsby D. C., Nakao M. Steady-state current-voltage relationship of the Na/K pump in guinea pig ventricular myocytes. J Gen Physiol. 1989 Sep;94(3):511–537. doi: 10.1085/jgp.94.3.511. [DOI] [PMC free article] [PubMed] [Google Scholar]
- Gibbons W. R., Fozzard H. A. Relationships between voltage and tension in sheep cardiac Purkinje fibers. J Gen Physiol. 1975 Mar;65(3):345–365. doi: 10.1085/jgp.65.3.345. [DOI] [PMC free article] [PubMed] [Google Scholar]
- Hadley R. W., Hume J. R. An intrinsic potential-dependent inactivation mechanism associated with calcium channels in guinea-pig myocytes. J Physiol. 1987 Aug;389:205–222. doi: 10.1113/jphysiol.1987.sp016654. [DOI] [PMC free article] [PubMed] [Google Scholar]
- Irisawa H., Sato R. Intra- and extracellular actions of proton on the calcium current of isolated guinea pig ventricular cells. Circ Res. 1986 Sep;59(3):348–355. doi: 10.1161/01.res.59.3.348. [DOI] [PubMed] [Google Scholar]
- Isenberg G., Klöckner U. Calcium currents of isolated bovine ventricular myocytes are fast and of large amplitude. Pflugers Arch. 1982 Oct;395(1):30–41. doi: 10.1007/BF00584965. [DOI] [PubMed] [Google Scholar]
- Isenberg G., Trautwein W. The effect of dihydro-ouabain and lithium-ions on the outward current in cardiac Purkinje fibers. Evidence for electrogenicity of active transport. Pflugers Arch. 1974;350(1):41–54. doi: 10.1007/BF00586737. [DOI] [PubMed] [Google Scholar]
- Josephson I., Sperelakis N. Ouabain blockade of inward slow current in cardiac muscle. J Mol Cell Cardiol. 1977 May;9(5):409–418. doi: 10.1016/s0022-2828(77)80007-2. [DOI] [PubMed] [Google Scholar]
- Kameyama M., Kakei M., Sato R., Shibasaki T., Matsuda H., Irisawa H. Intracellular Na+ activates a K+ channel in mammalian cardiac cells. Nature. 1984 May 24;309(5966):354–356. doi: 10.1038/309354a0. [DOI] [PubMed] [Google Scholar]
- Kentish J. C., Boyett M. R. A simple electronic circuit for monitoring changes in the duration of the action potential. Pflugers Arch. 1983 Aug;398(3):233–235. doi: 10.1007/BF00657157. [DOI] [PubMed] [Google Scholar]
- Kimura J., Miyamae S., Noma A. Identification of sodium-calcium exchange current in single ventricular cells of guinea-pig. J Physiol. 1987 Mar;384:199–222. doi: 10.1113/jphysiol.1987.sp016450. [DOI] [PMC free article] [PubMed] [Google Scholar]
- Kokubun S., Irisawa H. Effects of various intracellular Ca ion concentrations on the calcium current of guinea-pig single ventricular cells. Jpn J Physiol. 1984;34(4):599–611. doi: 10.2170/jjphysiol.34.599. [DOI] [PubMed] [Google Scholar]
- Lederer W. J., Eisner D. A. The effects of sodium pump activity on the slow inward current in sheep cardiac Purkinje fibres. Proc R Soc Lond B Biol Sci. 1982 Jan 22;214(1195):249–262. doi: 10.1098/rspb.1982.0008. [DOI] [PubMed] [Google Scholar]
- Lee C. O. 200 years of digitalis: the emerging central role of the sodium ion in the control of cardiac force. Am J Physiol. 1985 Nov;249(5 Pt 1):C367–C378. doi: 10.1152/ajpcell.1985.249.5.C367. [DOI] [PubMed] [Google Scholar]
- Lee C. O., Dagostino M. Effect of strophanthidin on intracellular Na ion activity and twitch tension of constantly driven canine cardiac Purkinje fibers. Biophys J. 1982 Dec;40(3):185–198. doi: 10.1016/S0006-3495(82)84474-3. [DOI] [PMC free article] [PubMed] [Google Scholar]
- Lee K. S., Tsien R. W. Reversal of current through calcium channels in dialysed single heart cells. Nature. 1982 Jun 10;297(5866):498–501. doi: 10.1038/297498a0. [DOI] [PubMed] [Google Scholar]
- Lüllmann H., Ravens U. The time courses of the changes in contractile force and in transmembfane potentials induced by cardiac glycosides in guinea-pig papillary muscle. Br J Pharmacol. 1973 Nov;49(3):377–390. doi: 10.1111/j.1476-5381.1973.tb17248.x. [DOI] [PMC free article] [PubMed] [Google Scholar]
- Makielski J. C., Sheets M. F., Hanck D. A., January C. T., Fozzard H. A. Sodium current in voltage clamped internally perfused canine cardiac Purkinje cells. Biophys J. 1987 Jul;52(1):1–11. doi: 10.1016/S0006-3495(87)83182-X. [DOI] [PMC free article] [PubMed] [Google Scholar]
- Marban E., Tsien R. W. Enhancement of calcium current during digitalis inotropy in mammalian heart: positive feed-back regulation by intracellular calcium? J Physiol. 1982 Aug;329:589–614. doi: 10.1113/jphysiol.1982.sp014321. [DOI] [PMC free article] [PubMed] [Google Scholar]
- McDonald T. F., Nawrath H., Trautwein W. Membrane currents and tension in cat ventricular muscle treated with cardiac glycosides. Circ Res. 1975 Nov;37(5):674–682. doi: 10.1161/01.res.37.5.674. [DOI] [PubMed] [Google Scholar]
- Meech R. W. Calcium-dependent potassium activation in nervous tissues. Annu Rev Biophys Bioeng. 1978;7:1–18. doi: 10.1146/annurev.bb.07.060178.000245. [DOI] [PubMed] [Google Scholar]
- Mitchell M. R., Powell T., Terrar D. A., Twist V. W. Characteristics of the second inward current in cells isolated from rat ventricular muscle. Proc R Soc Lond B Biol Sci. 1983 Oct 22;219(1217):447–469. doi: 10.1098/rspb.1983.0084. [DOI] [PubMed] [Google Scholar]
- Nakao M., Gadsby D. C. [Na] and [K] dependence of the Na/K pump current-voltage relationship in guinea pig ventricular myocytes. J Gen Physiol. 1989 Sep;94(3):539–565. doi: 10.1085/jgp.94.3.539. [DOI] [PMC free article] [PubMed] [Google Scholar]
- Noble D. Mechanism of action of therapeutic levels of cardiac glycosides. Cardiovasc Res. 1980 Sep;14(9):495–514. doi: 10.1093/cvr/14.9.495. [DOI] [PubMed] [Google Scholar]
- Noble D. The surprising heart: a review of recent progress in cardiac electrophysiology. J Physiol. 1984 Aug;353:1–50. doi: 10.1113/jphysiol.1984.sp015320. [DOI] [PMC free article] [PubMed] [Google Scholar]
- Plant T. D., Standen N. B., Ward T. A. The effects of injection of calcium ions and calcium chelators on calcium channel inactivation in Helix neurones. J Physiol. 1983 Jan;334:189–212. doi: 10.1113/jphysiol.1983.sp014489. [DOI] [PMC free article] [PubMed] [Google Scholar]
- Tritthart H., Weiss R., Volkmann R., Späh F. Proceedings: The effects of cardiac glycosides on Ca-mediated action potentials in the mammalian myocardium. Naunyn Schmiedebergs Arch Pharmacol. 1974;282(Suppl):suppl 282–282:R99. [PubMed] [Google Scholar]
- WOODBURY L. A., HECHT H. H. Effects of cardiac glycosides upon the electrical activity of single ventricular fibers of the frog heart, and their relation to the digitalis effect of the electrocardiogram. Circulation. 1952 Aug;6(2):172–182. doi: 10.1161/01.cir.6.2.172. [DOI] [PubMed] [Google Scholar]
- Zucker R. S. Tetraethylammonium contains an impurity which alkalizes cytoplasm and reduce calcium buffering in neurons. Brain Res. 1981 Mar 16;208(2):473–478. doi: 10.1016/0006-8993(81)90580-1. [DOI] [PubMed] [Google Scholar]