Abstract
1. A voltage-sensitive dye and multiple-site optical recording of changes in membrane potential were used to reveal the postsynaptic potentials in the early embryonic chick brain stem slice preparation. 2. Vagus-brain stem preparations were isolated from 8-day-old chick embryos and then transverse slice preparations were prepared with both the right and left vagus nerve fibres intact. The slice preparations were stained with a voltage-sensitive merocyanine-rhodanine dye (NK2761). 3. Voltage-related optical (absorbance) changes evoked by vagus nerve stimulation with positive square current pulses using a suction electrode were recorded simultaneously from 127 contiguous loci in the preparation, using a 12 x 12-element photodiode array. Optical responses appeared in a limited area near the dorsal surface of the stimulated side. 4. When relatively large stimulating currents were applied, optical changes having two (or sometimes three) components were recorded. One component was the fast spike-like signal and another the delayed, long-lasting slow signal. 5. The size of the slow signal was decreased by continuous stimulation, reduced by low external calcium ion concentrations and eliminated in the presence of manganese or cadmium ions. 6. The slow signals were eliminated in the presence of kynurenic acid, and they were reduced by 2-APV (DL-2-amino-5-phosphono-valeric acid) and by CNQX (6-cyano-7-nitroquinoxaline-2,3-dione). We conclude that the slow signals correspond to excitatory postsynaptic potentials which are glutamate mediated.
Full text
PDF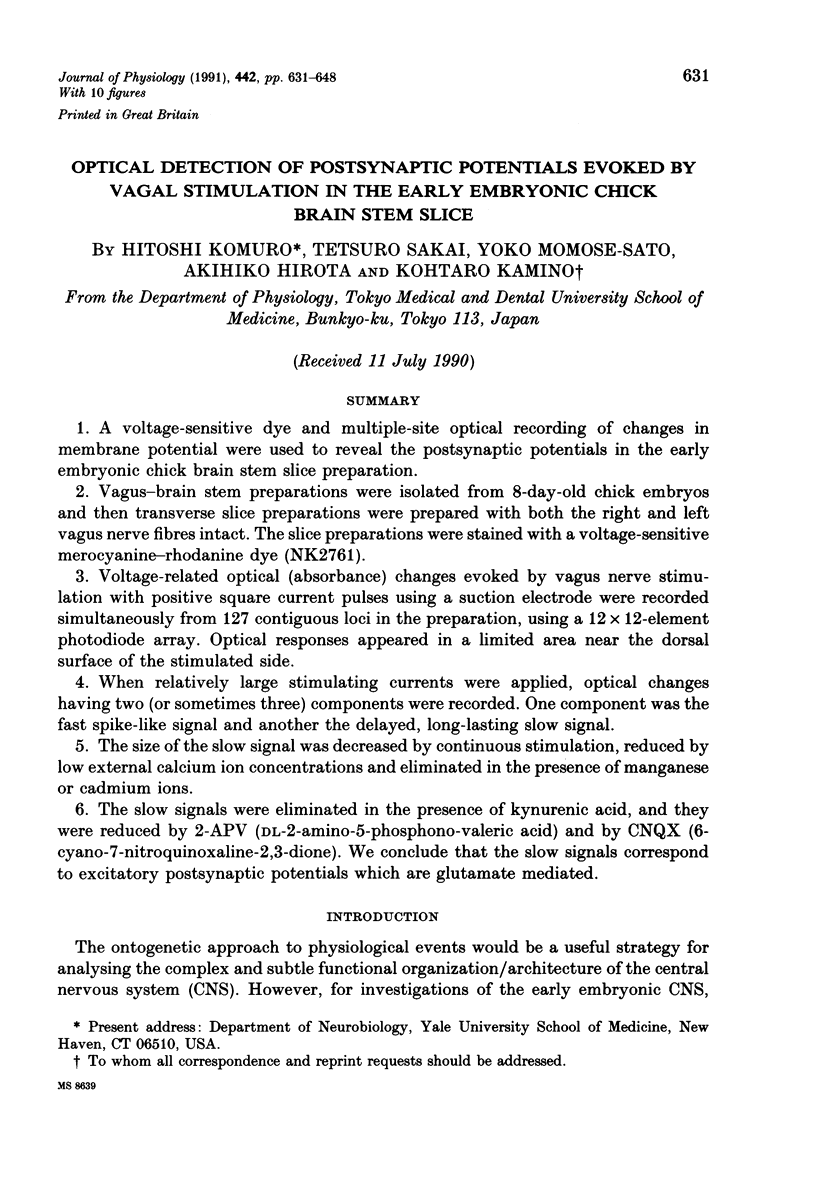
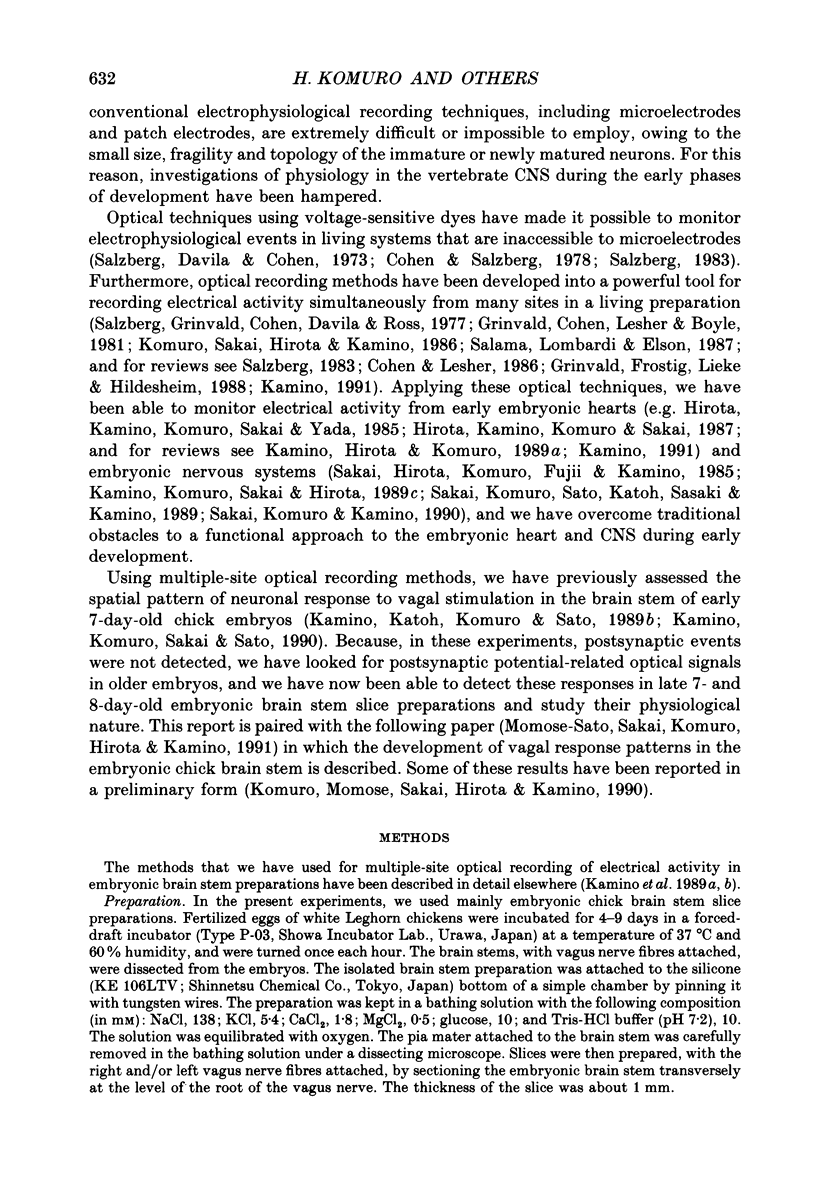
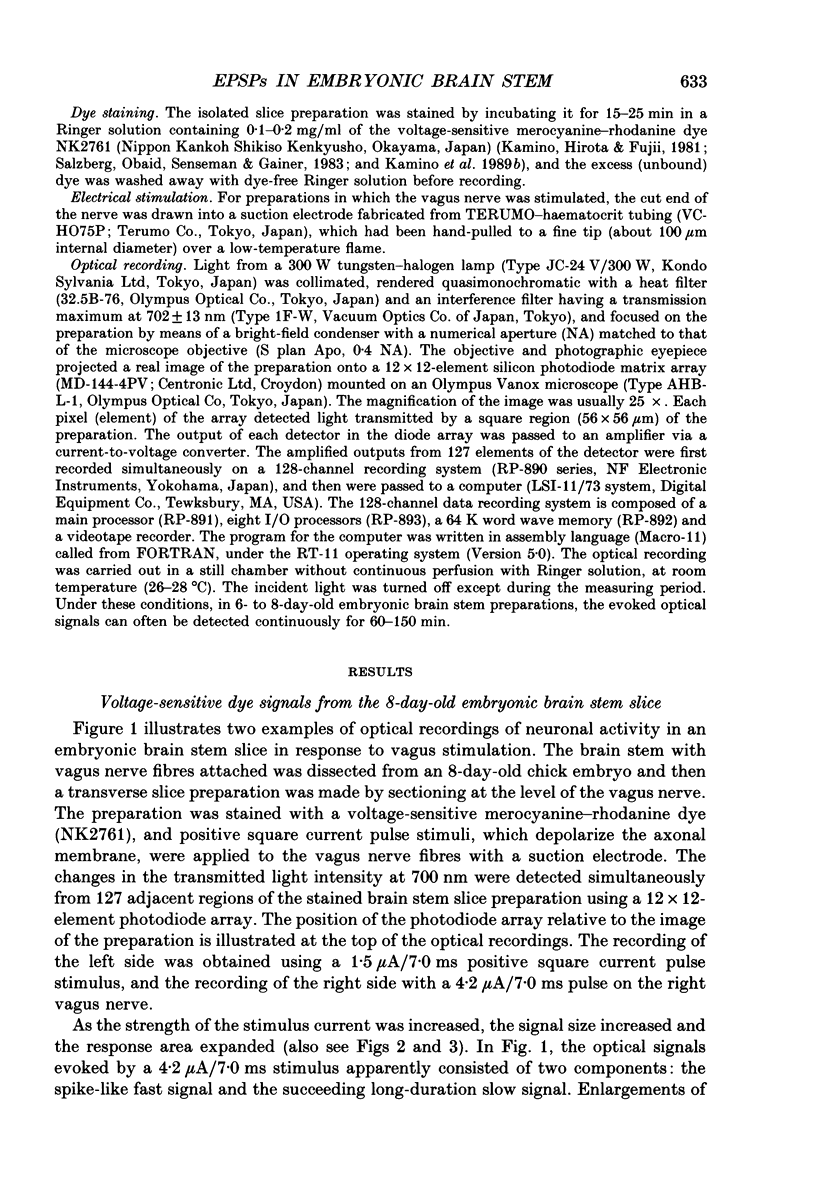
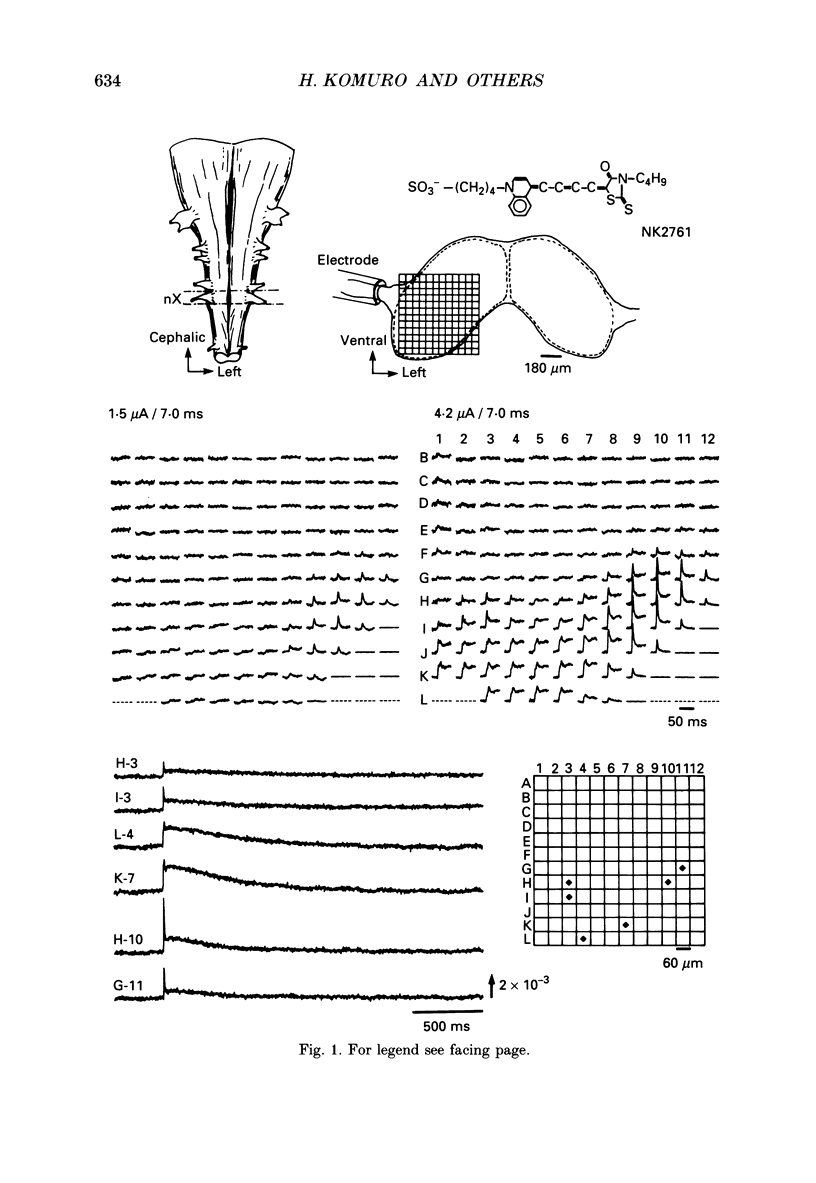
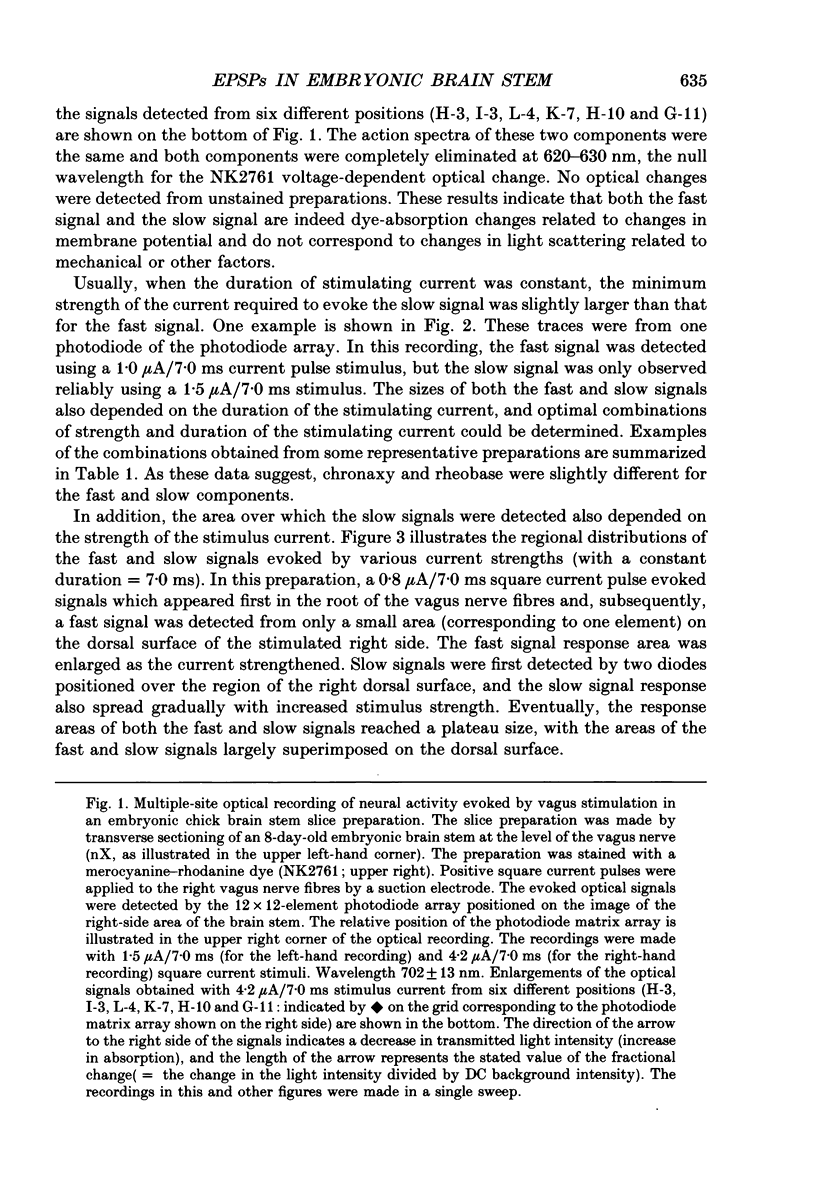
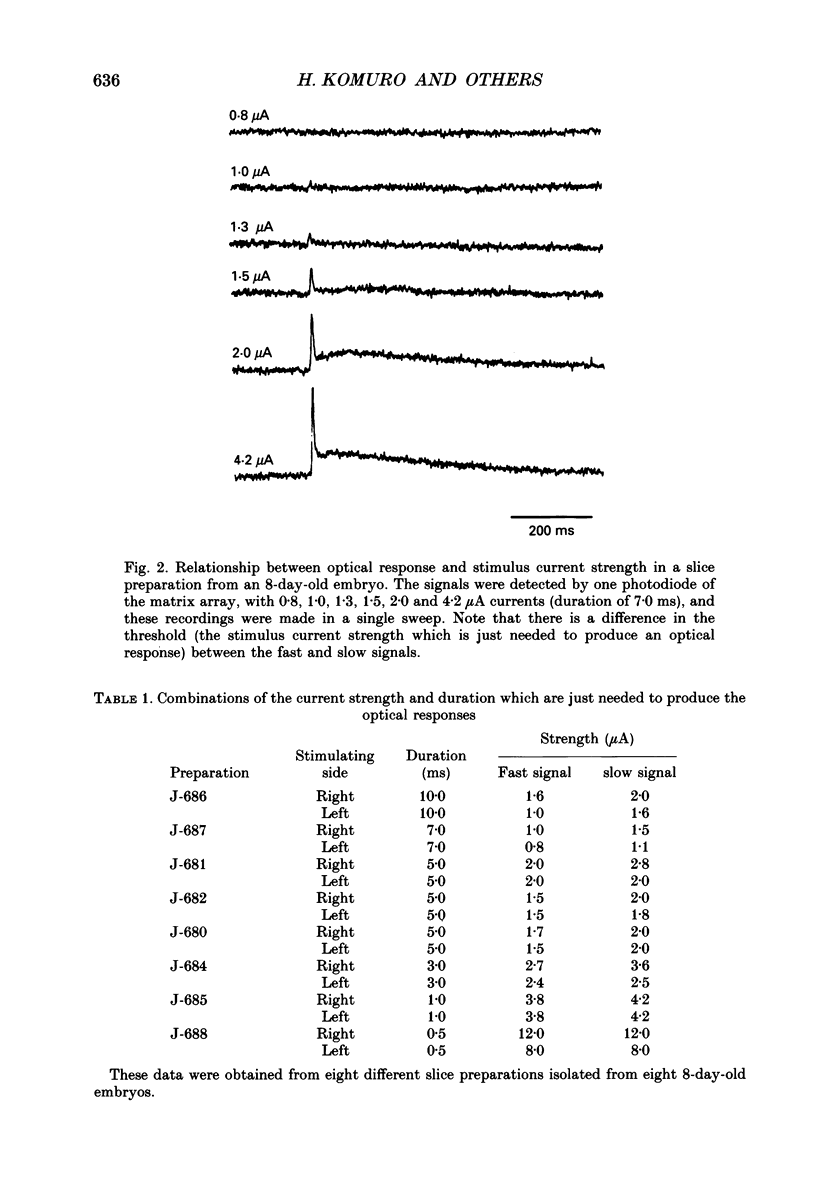
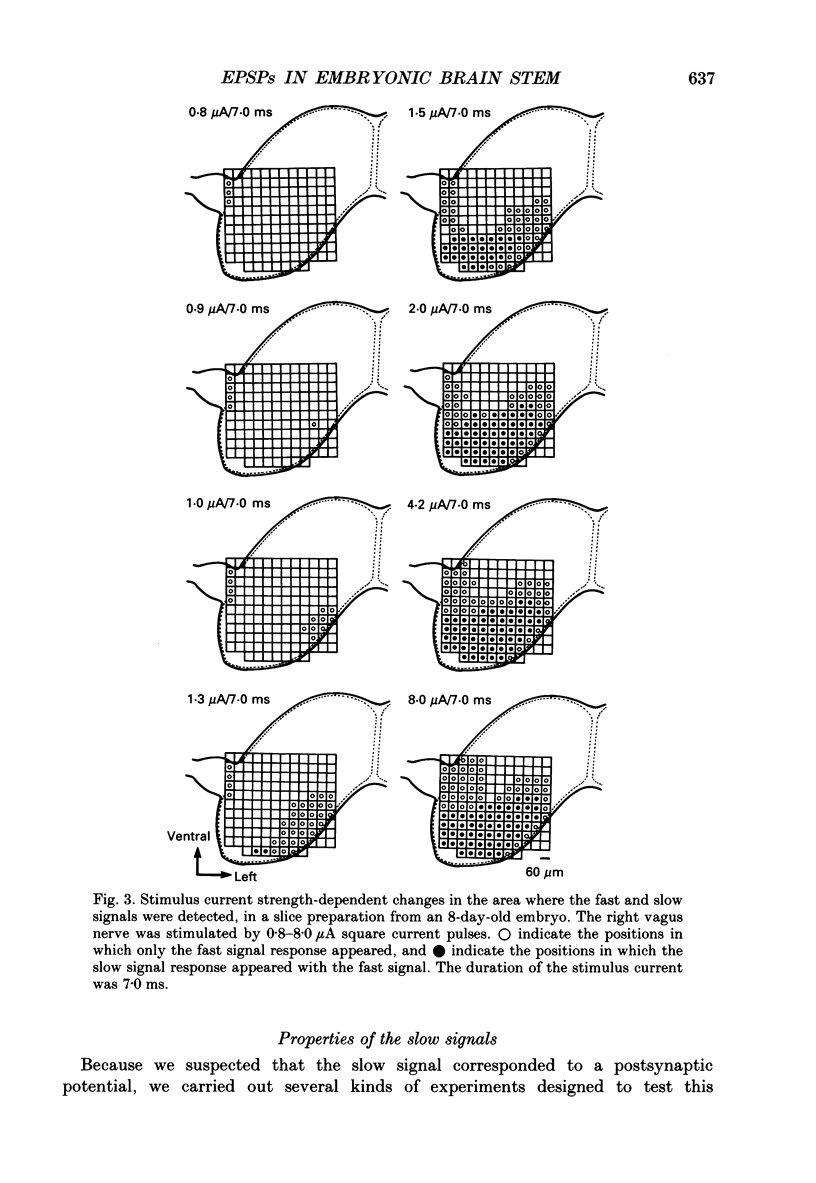
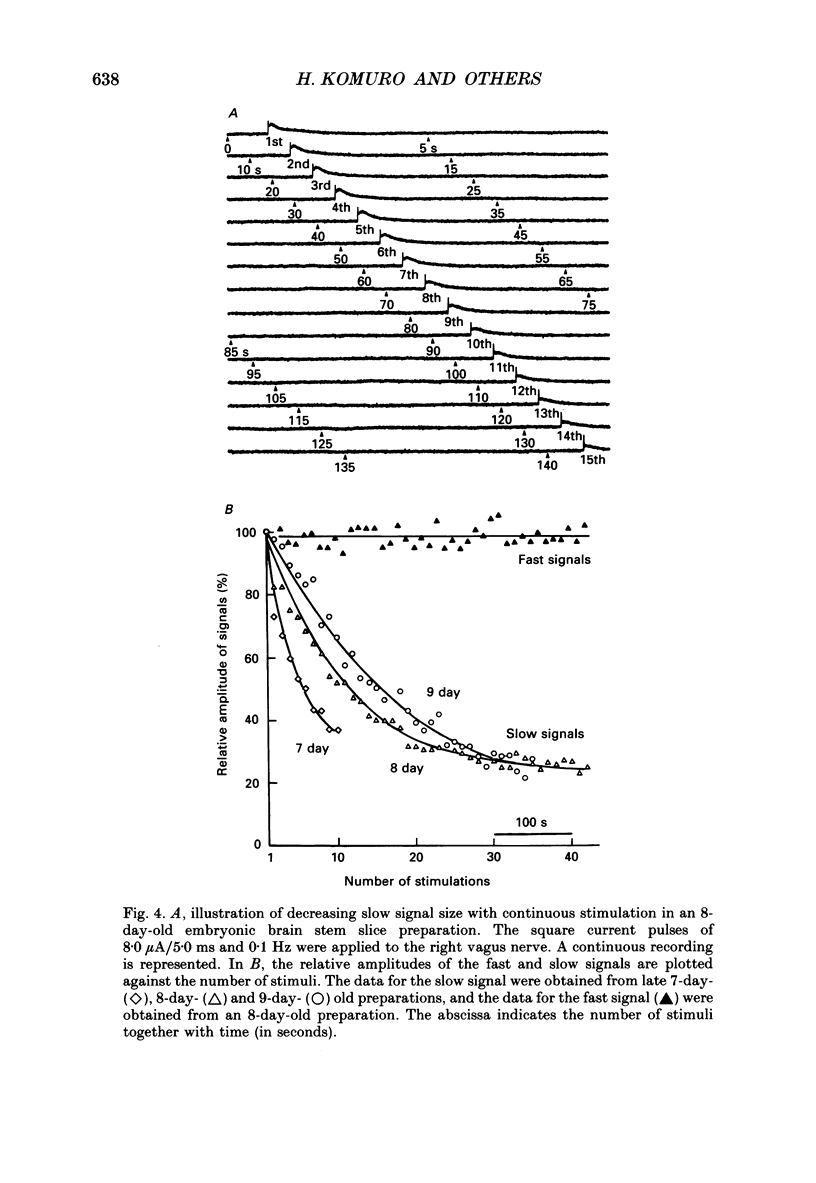
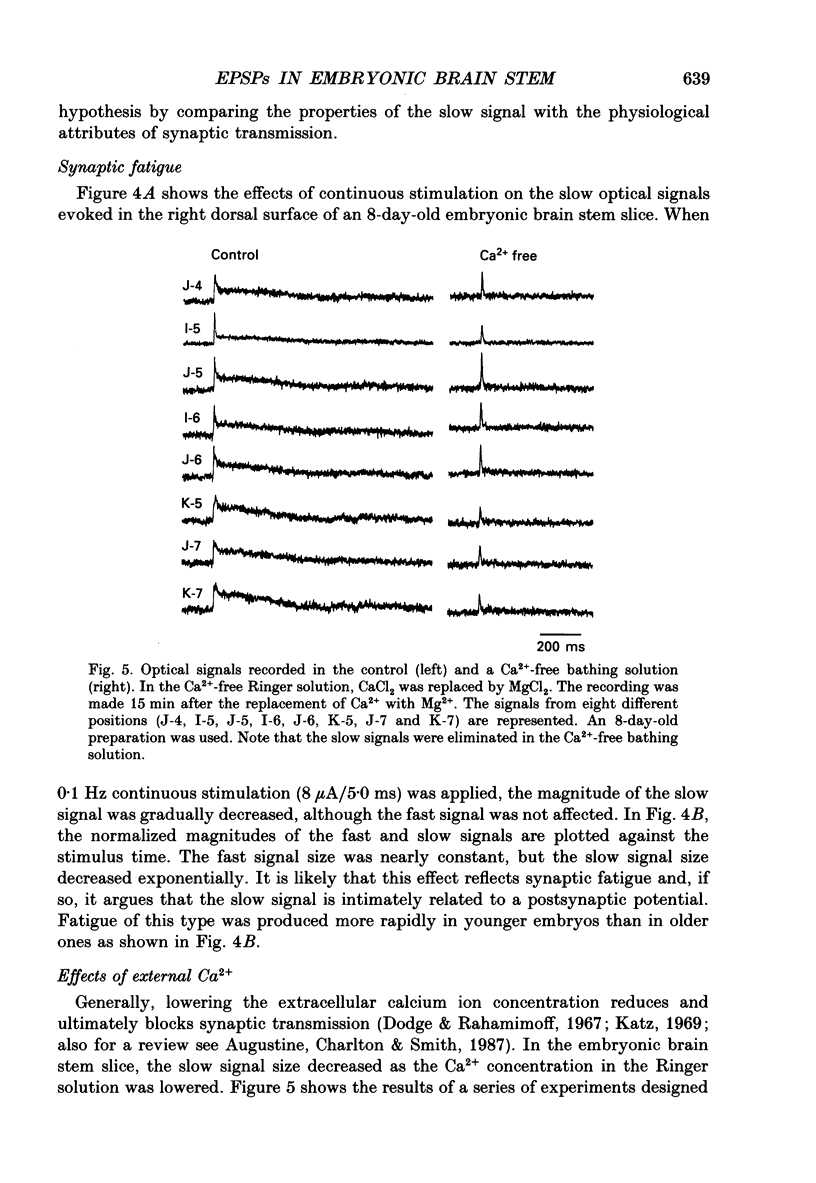
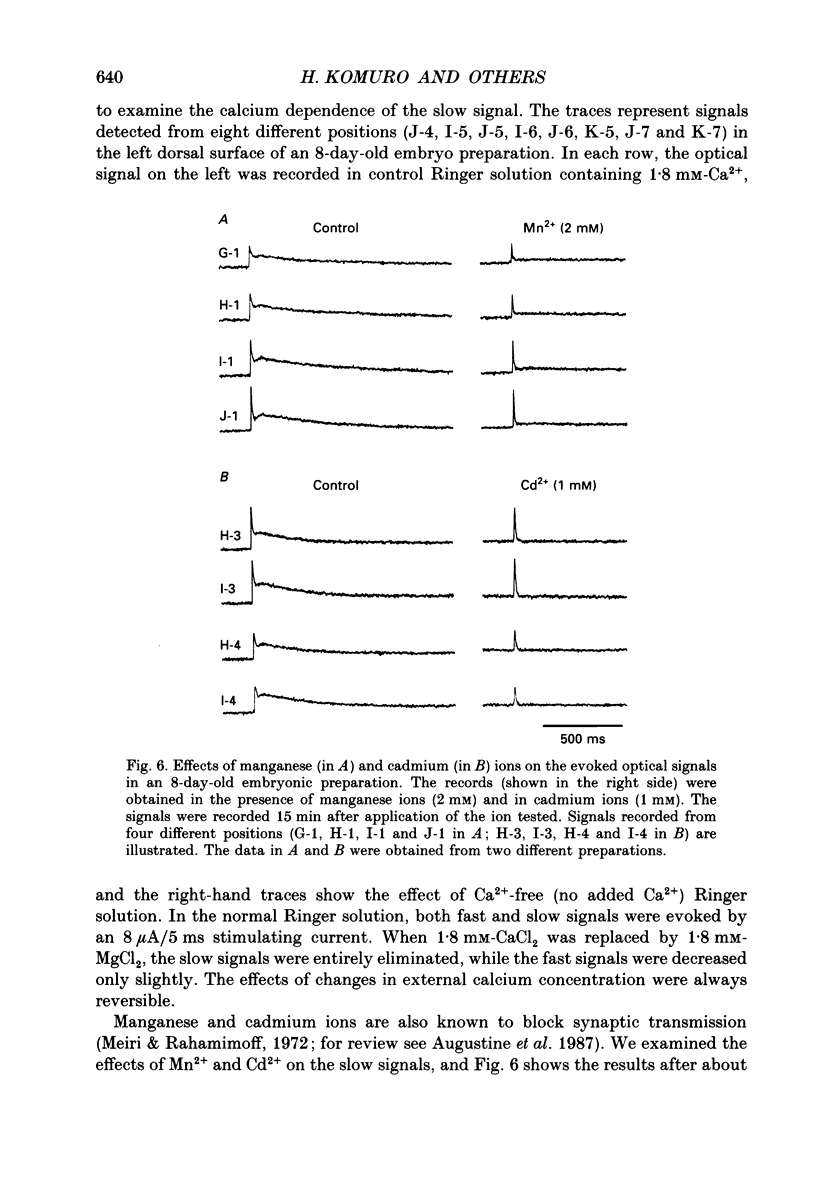
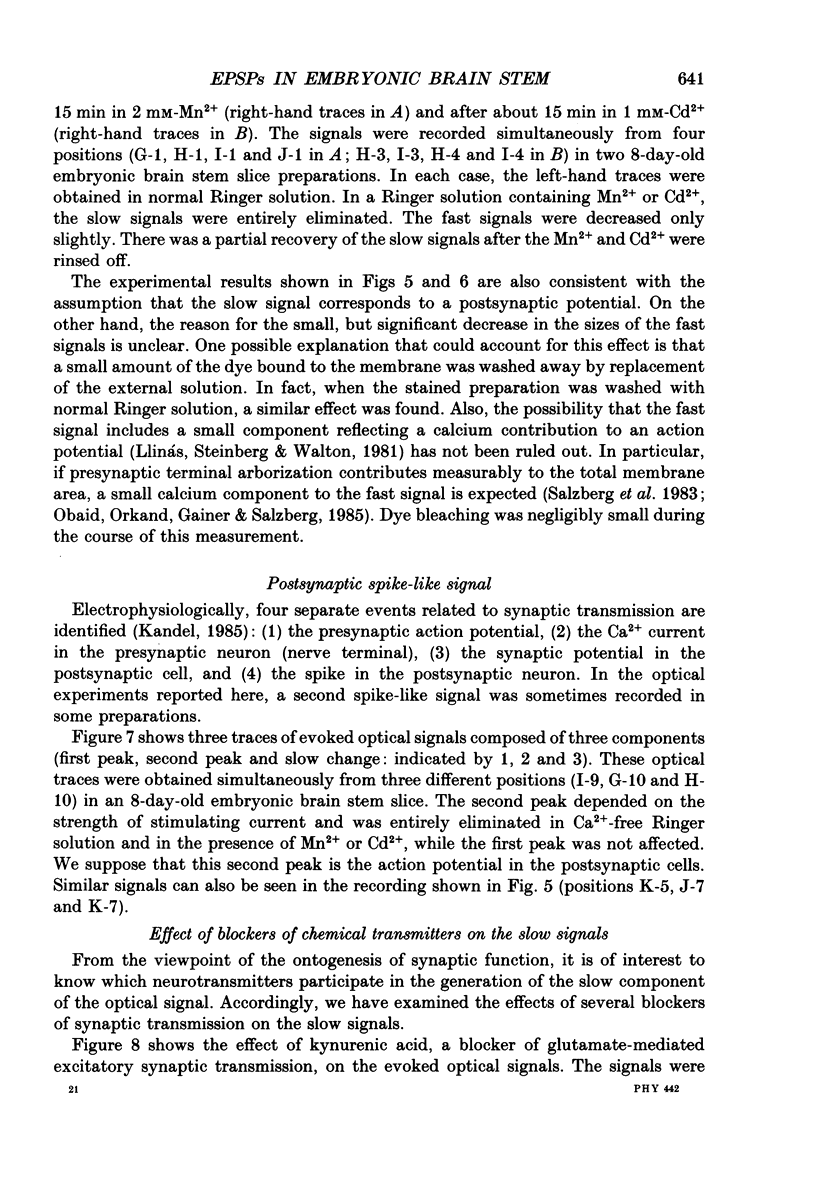
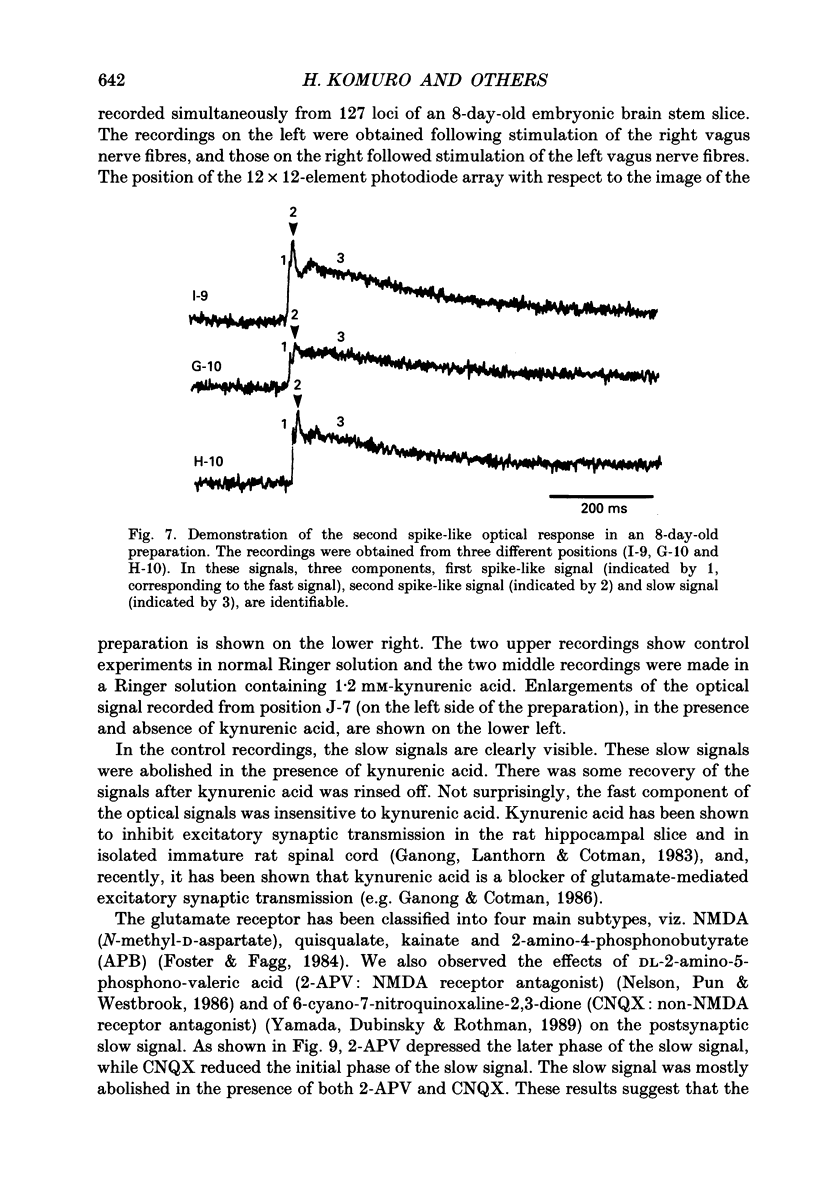
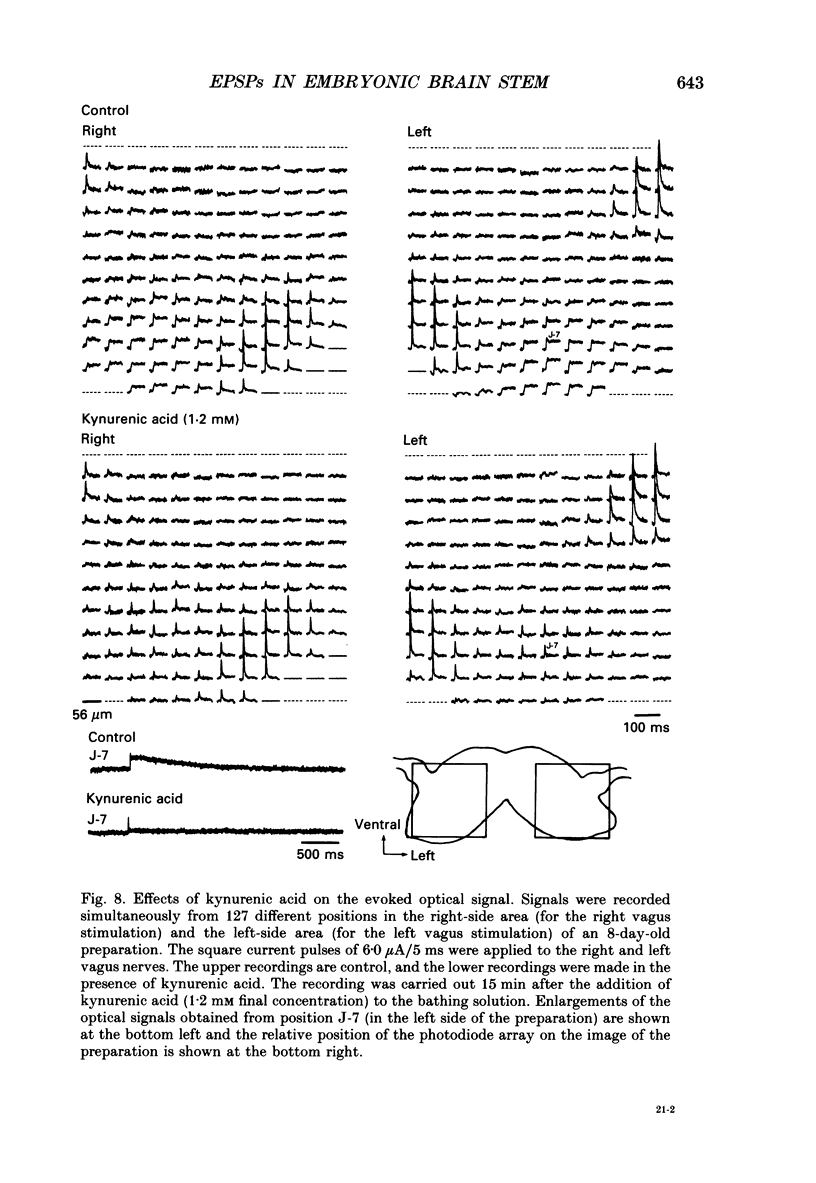
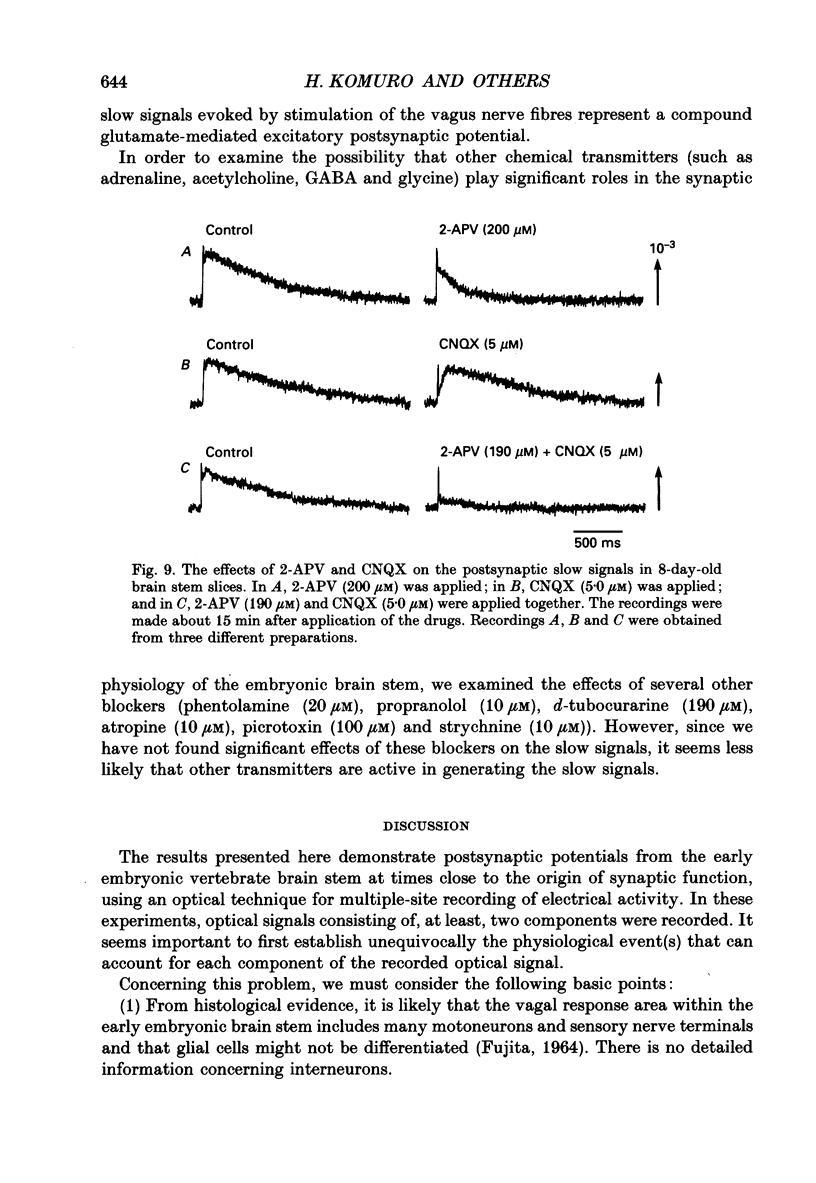
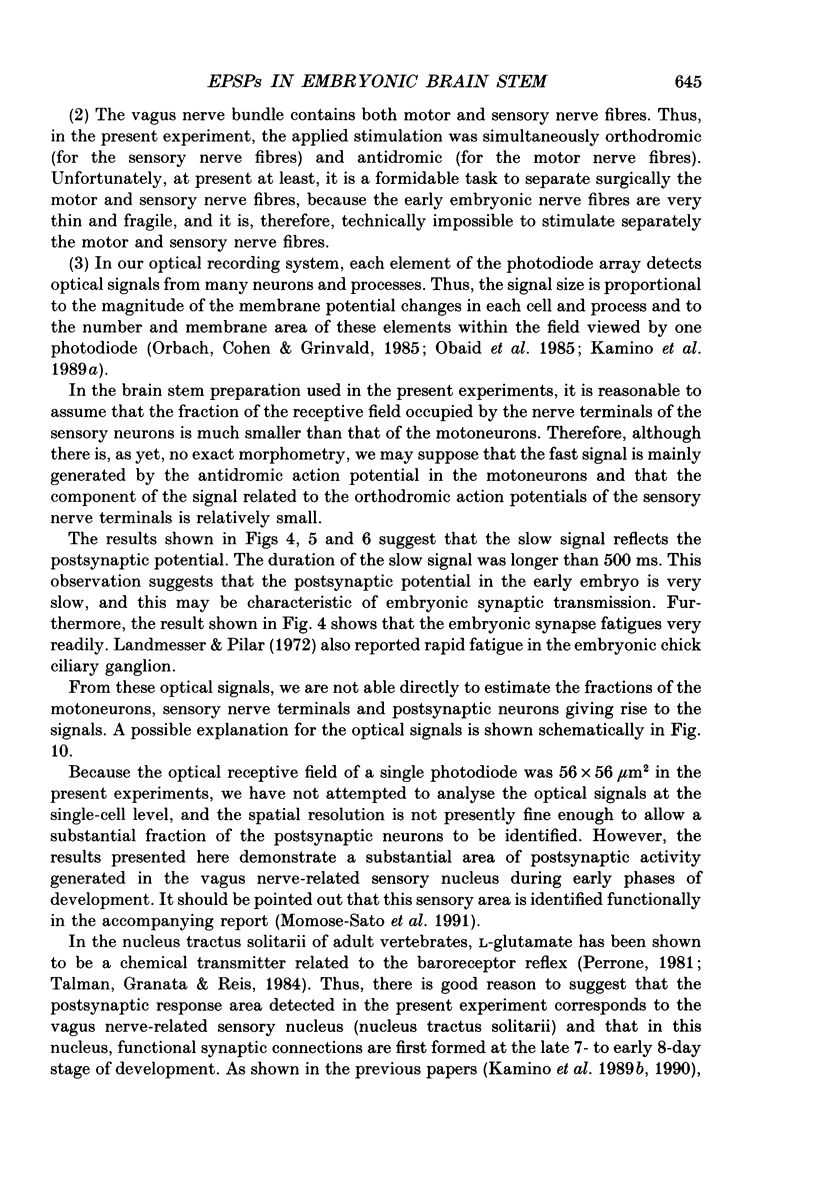
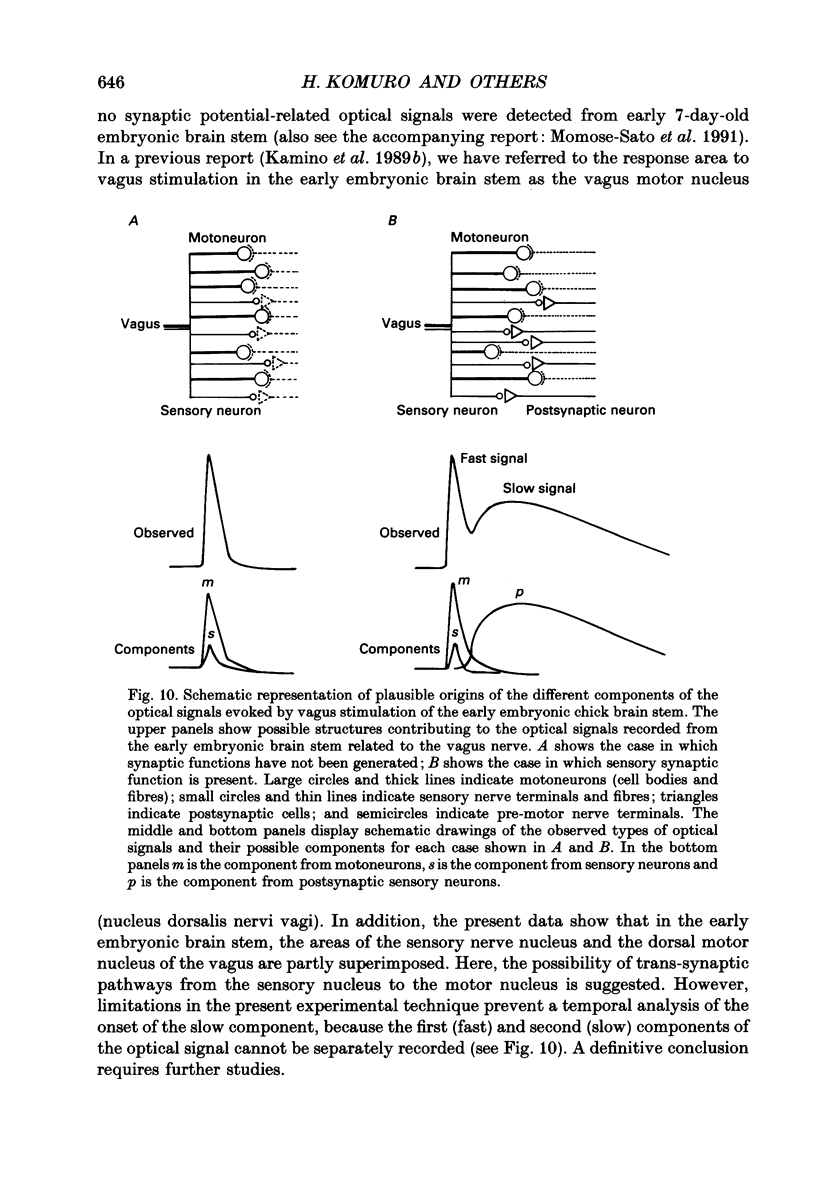
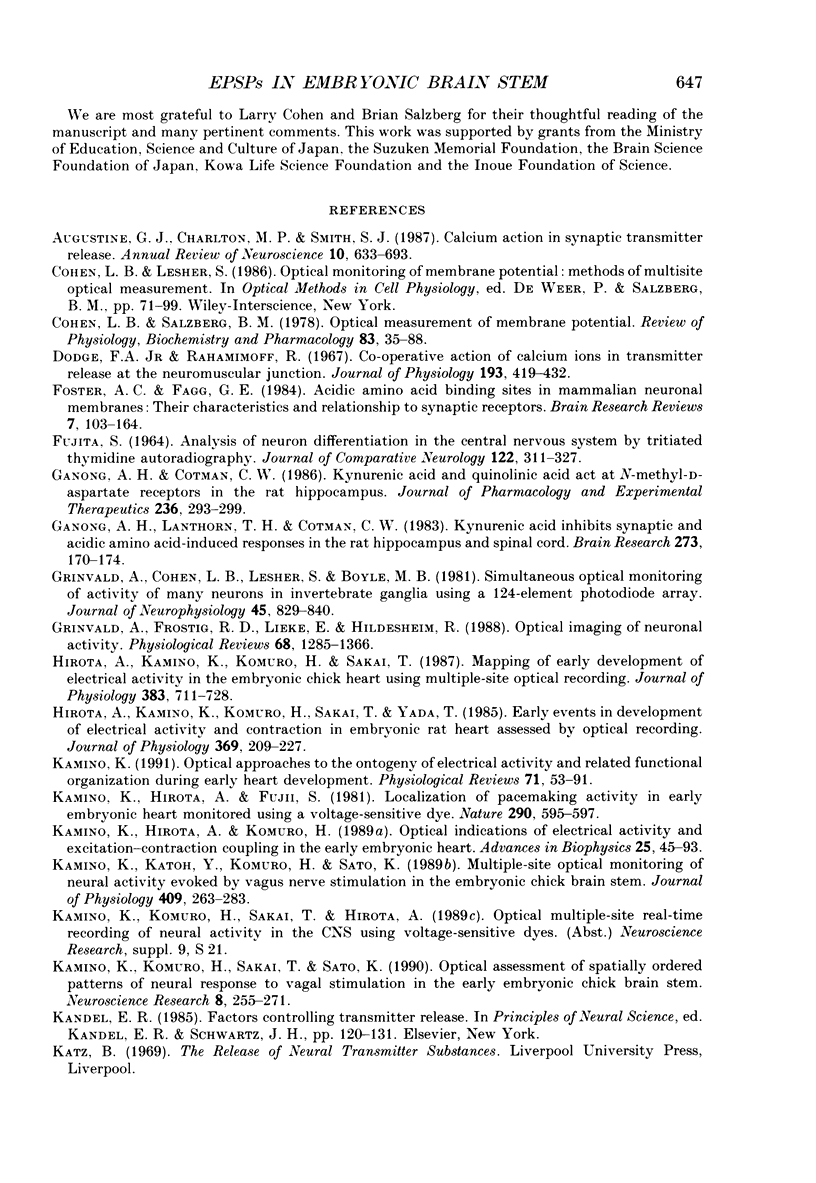
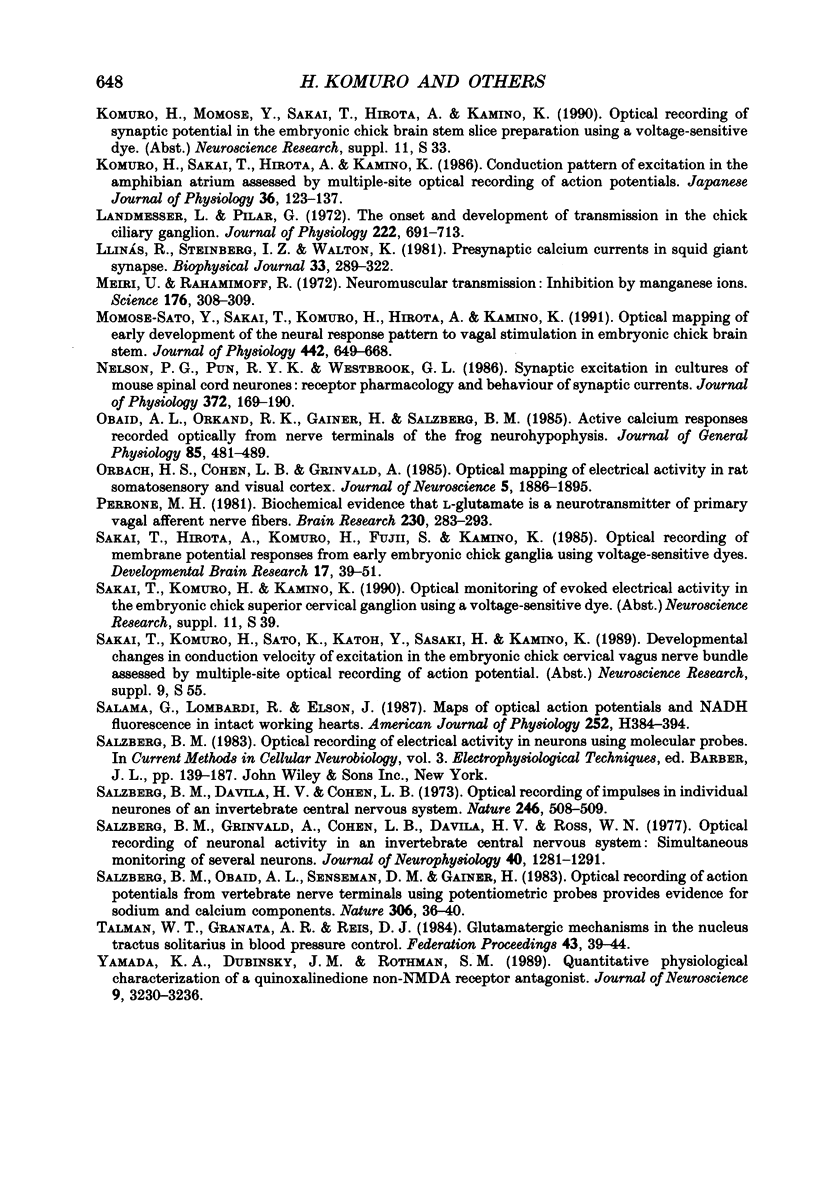
Selected References
These references are in PubMed. This may not be the complete list of references from this article.
- Augustine G. J., Charlton M. P., Smith S. J. Calcium action in synaptic transmitter release. Annu Rev Neurosci. 1987;10:633–693. doi: 10.1146/annurev.ne.10.030187.003221. [DOI] [PubMed] [Google Scholar]
- Cohen L. B., Salzberg B. M. Optical measurement of membrane potential. Rev Physiol Biochem Pharmacol. 1978;83:35–88. doi: 10.1007/3-540-08907-1_2. [DOI] [PubMed] [Google Scholar]
- Dodge F. A., Jr, Rahamimoff R. Co-operative action a calcium ions in transmitter release at the neuromuscular junction. J Physiol. 1967 Nov;193(2):419–432. doi: 10.1113/jphysiol.1967.sp008367. [DOI] [PMC free article] [PubMed] [Google Scholar]
- FUJITA S. ANALYSIS OF NEURON DIFFERENTIATION IN THE CENTRAL NERVOUS SYSTEM BY TRITIATED THYMIDINE AUTORADIOGRAPHY. J Comp Neurol. 1964 Jun;122:311–327. doi: 10.1002/cne.901220303. [DOI] [PubMed] [Google Scholar]
- Foster A. C., Fagg G. E. Acidic amino acid binding sites in mammalian neuronal membranes: their characteristics and relationship to synaptic receptors. Brain Res. 1984 May;319(2):103–164. doi: 10.1016/0165-0173(84)90020-1. [DOI] [PubMed] [Google Scholar]
- Ganong A. H., Cotman C. W. Kynurenic acid and quinolinic acid act at N-methyl-D-aspartate receptors in the rat hippocampus. J Pharmacol Exp Ther. 1986 Jan;236(1):293–299. [PubMed] [Google Scholar]
- Ganong A. H., Lanthorn T. H., Cotman C. W. Kynurenic acid inhibits synaptic and acidic amino acid-induced responses in the rat hippocampus and spinal cord. Brain Res. 1983 Aug 22;273(1):170–174. doi: 10.1016/0006-8993(83)91108-3. [DOI] [PubMed] [Google Scholar]
- Grinvald A., Cohen L. B., Lesher S., Boyle M. B. Simultaneous optical monitoring of activity of many neurons in invertebrate ganglia using a 124-element photodiode array. J Neurophysiol. 1981 May;45(5):829–840. doi: 10.1152/jn.1981.45.5.829. [DOI] [PubMed] [Google Scholar]
- Grinvald A., Frostig R. D., Lieke E., Hildesheim R. Optical imaging of neuronal activity. Physiol Rev. 1988 Oct;68(4):1285–1366. doi: 10.1152/physrev.1988.68.4.1285. [DOI] [PubMed] [Google Scholar]
- Hirota A., Kamino K., Komuro H., Sakai T. Mapping of early development of electrical activity in the embryonic chick heart using multiple-site optical recording. J Physiol. 1987 Feb;383:711–728. doi: 10.1113/jphysiol.1987.sp016437. [DOI] [PMC free article] [PubMed] [Google Scholar]
- Hirota A., Kamino K., Komuro H., Sakai T., Yada T. Early events in development of electrical activity and contraction in embryonic rat heart assessed by optical recording. J Physiol. 1985 Dec;369:209–227. doi: 10.1113/jphysiol.1985.sp015897. [DOI] [PMC free article] [PubMed] [Google Scholar]
- Kamino K., Hirota A., Fujii S. Localization of pacemaking activity in early embryonic heart monitored using voltage-sensitive dye. Nature. 1981 Apr 16;290(5807):595–597. doi: 10.1038/290595a0. [DOI] [PubMed] [Google Scholar]
- Kamino K., Hirota A., Komuro H. Optical indications of electrical activity and excitation-contraction coupling in the early embryonic heart. Adv Biophys. 1989;25:45–93. doi: 10.1016/0065-227x(89)90004-x. [DOI] [PubMed] [Google Scholar]
- Kamino K., Katoh Y., Komuro H., Sato K. Multiple-site optical monitoring of neural activity evoked by vagus nerve stimulation in the embryonic chick brain stem. J Physiol. 1989 Feb;409:263–283. doi: 10.1113/jphysiol.1989.sp017496. [DOI] [PMC free article] [PubMed] [Google Scholar]
- Kamino K., Komuro H., Sakai T., Sato K. Optical assessment of spatially ordered patterns of neural response to vagal stimulation in the early embryonic chick brainstem. Neurosci Res. 1990 Aug;8(4):255–271. doi: 10.1016/0168-0102(90)90032-a. [DOI] [PubMed] [Google Scholar]
- Kamino K. Optical approaches to ontogeny of electrical activity and related functional organization during early heart development. Physiol Rev. 1991 Jan;71(1):53–91. doi: 10.1152/physrev.1991.71.1.53. [DOI] [PubMed] [Google Scholar]
- Komuro H., Sakai T., Hirota A., Kamino K. Conduction pattern of excitation in the amphibian atrium assessed by multiple-site optical recording of action potentials. Jpn J Physiol. 1986;36(1):123–137. doi: 10.2170/jjphysiol.36.123. [DOI] [PubMed] [Google Scholar]
- Landmesser L., Pilar G. The onset and development of transmission in the chick ciliary ganglion. J Physiol. 1972 May;222(3):691–713. doi: 10.1113/jphysiol.1972.sp009822. [DOI] [PMC free article] [PubMed] [Google Scholar]
- Llinás R., Steinberg I. Z., Walton K. Presynaptic calcium currents in squid giant synapse. Biophys J. 1981 Mar;33(3):289–321. doi: 10.1016/S0006-3495(81)84898-9. [DOI] [PMC free article] [PubMed] [Google Scholar]
- Meiri U., Rahamimoff R. Neuromuscular transmission: inhibition by manganese ions. Science. 1972 Apr 21;176(4032):308–309. doi: 10.1126/science.176.4032.308. [DOI] [PubMed] [Google Scholar]
- Momose-Sato Y., Sakai T., Komuro H., Hirota A., Kamino K. Optical mapping of the early development of the response pattern to vagal stimulation in embryonic chick brain stem. J Physiol. 1991 Oct;442:649–668. doi: 10.1113/jphysiol.1991.sp018813. [DOI] [PMC free article] [PubMed] [Google Scholar]
- Nelson P. G., Pun R. Y., Westbrook G. L. Synaptic excitation in cultures of mouse spinal cord neurones: receptor pharmacology and behaviour of synaptic currents. J Physiol. 1986 Mar;372:169–190. doi: 10.1113/jphysiol.1986.sp016003. [DOI] [PMC free article] [PubMed] [Google Scholar]
- Obaid A. L., Orkand R. K., Gainer H., Salzberg B. M. Active calcium responses recorded optically from nerve terminals of the frog neurohypophysis. J Gen Physiol. 1985 Apr;85(4):481–489. doi: 10.1085/jgp.85.4.481. [DOI] [PMC free article] [PubMed] [Google Scholar]
- Orbach H. S., Cohen L. B., Grinvald A. Optical mapping of electrical activity in rat somatosensory and visual cortex. J Neurosci. 1985 Jul;5(7):1886–1895. doi: 10.1523/JNEUROSCI.05-07-01886.1985. [DOI] [PMC free article] [PubMed] [Google Scholar]
- Perrone M. H. Biochemical evidence that L-glutamate is a neurotransmitter of primary vagal afferent nerve fibers. Brain Res. 1981 Dec 28;230(1-2):283–293. doi: 10.1016/0006-8993(81)90407-8. [DOI] [PubMed] [Google Scholar]
- Sakai T., Hirota A., Komuro H., Fujii S., Kamino K. Optical recording of membrane potential responses from early embryonic chick ganglia using voltage-sensitive dyes. Brain Res. 1985 Jan;349(1-2):39–51. doi: 10.1016/0165-3806(85)90130-0. [DOI] [PubMed] [Google Scholar]
- Salama G., Lombardi R., Elson J. Maps of optical action potentials and NADH fluorescence in intact working hearts. Am J Physiol. 1987 Feb;252(2 Pt 2):H384–H394. doi: 10.1152/ajpheart.1987.252.2.H384. [DOI] [PubMed] [Google Scholar]
- Salzberg B. M., Davila H. V., Cohen L. B. Optical recording of impulses in individual neurones of an invertebrate central nervous system. Nature. 1973 Dec 21;246(5434):508–509. doi: 10.1038/246508a0. [DOI] [PubMed] [Google Scholar]
- Salzberg B. M., Grinvald A., Cohen L. B., Davila H. V., Ross W. N. Optical recording of neuronal activity in an invertebrate central nervous system: simultaneous monitoring of several neurons. J Neurophysiol. 1977 Nov;40(6):1281–1291. doi: 10.1152/jn.1977.40.6.1281. [DOI] [PubMed] [Google Scholar]
- Salzberg B. M., Obaid A. L., Senseman D. M., Gainer H. Optical recording of action potentials from vertebrate nerve terminals using potentiometric probes provides evidence for sodium and calcium components. Nature. 1983 Nov 3;306(5938):36–40. doi: 10.1038/306036a0. [DOI] [PubMed] [Google Scholar]
- Talman W. T., Granata A. R., Reis D. J. Glutamatergic mechanisms in the nucleus tractus solitarius in blood pressure control. Fed Proc. 1984 Jan;43(1):39–44. [PubMed] [Google Scholar]
- Yamada K. A., Dubinsky J. M., Rothman S. M. Quantitative physiological characterization of a quinoxalinedione non-NMDA receptor antagonist. J Neurosci. 1989 Sep;9(9):3230–3236. doi: 10.1523/JNEUROSCI.09-09-03230.1989. [DOI] [PMC free article] [PubMed] [Google Scholar]