Abstract
1. Potassium (K+) contractures have been used to characterize the processes of activation and inactivation of excitation-contraction coupling during prolonged depolarization of fibres in small bundles dissected from rat soleus muscles at 23 degrees C. 2. The smallest measurable K+ contracture tension was recorded with depolarization to -40 mV in 30 mM-K+ and maximum tension was achieved between -26 mV in 80 mM-K+ and -19 mV in 120 mM-K+. 3. The rate of inactivation of K+ contracture tension was voltage dependent. Tension decayed from 80 to 20% of the peak amplitude within 44.0 +/- 2.2 s at -26 mV (in 80 mM-K+), compared with 66.7 +/- 4.8 s at -35 mV (in 40 mM-K+). Results are given as mean +/- 1 S.E.M. 4. The effect of inactivation on maximum tension was determined using a two pulse protocol in which a 'conditioning' depolarization in solutions containing 20-120 mM-K+ was applied for 0.5-10 min before a 'test' depolarization to -8 mV in 200 mM-K+. The amplitude of the test contracture was compared with the mean amplitude of 'control' 200 mM-K+ contractures elicited in normally polarized fibres immediately before and after the two pulse protocol. Conditioning depolarization to -47 mV (in 20 mM-K+) did not reduce test 200 mM-K+ contracture tension. Significant inactivation was seen with further conditioning depolarization to more positive potentials: after 10 min at -40 mV (in 30 mM-K+), or -35 mV (in 40 mM-K+), test 200 mM-K+ contracture tension was reduced by 33 and 70% respectively. 5. In contrast to amphibian muscle, where maximum tension falls to zero within a few minutes of depolarization to potentials positive to -50 mV, test 200 mM-K+ contracture tension in rat soleus fibres fell initially rapidly and then slowly, but was not reduced to zero, even after 10 min at -19 mV in 120 mM-K+. 6. The fast phase of inactivation of test 200 mM-K+ contracture tension occurred during the decay of the conditioning K+ contracture. The slow phase of inactivation reached completion after 10 min of conditioning depolarization and occurred during the period when conditioning tension was reduced to zero or to a plateau level. Both phases of inactivation in rat soleus fibres are slow compared with fast and slow inactivation times of 5-100 s respectively reported for amphibian muscle. 7. When repolarized after prolonged depolarization, the muscle fibres were initially refractory, i.e. unable to produce tension in response to electrical stimulation.(ABSTRACT TRUNCATED AT 400 WORDS)
Full text
PDF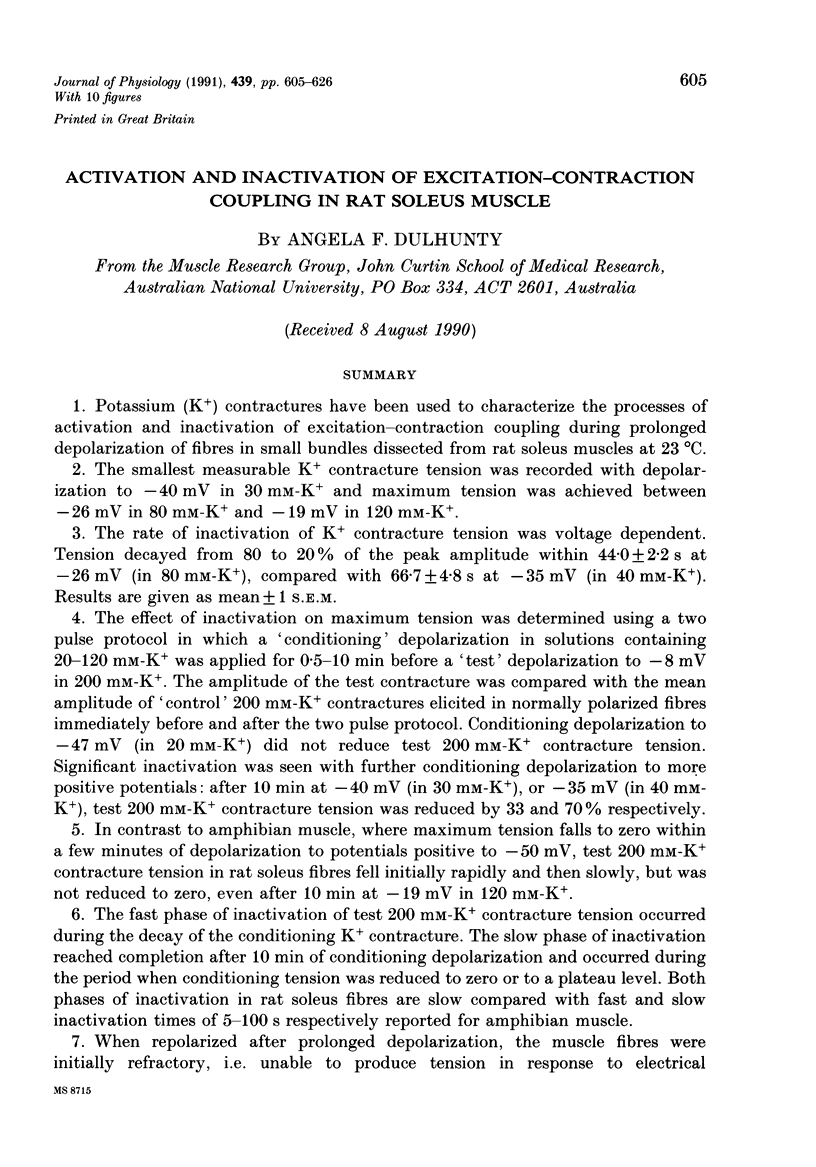
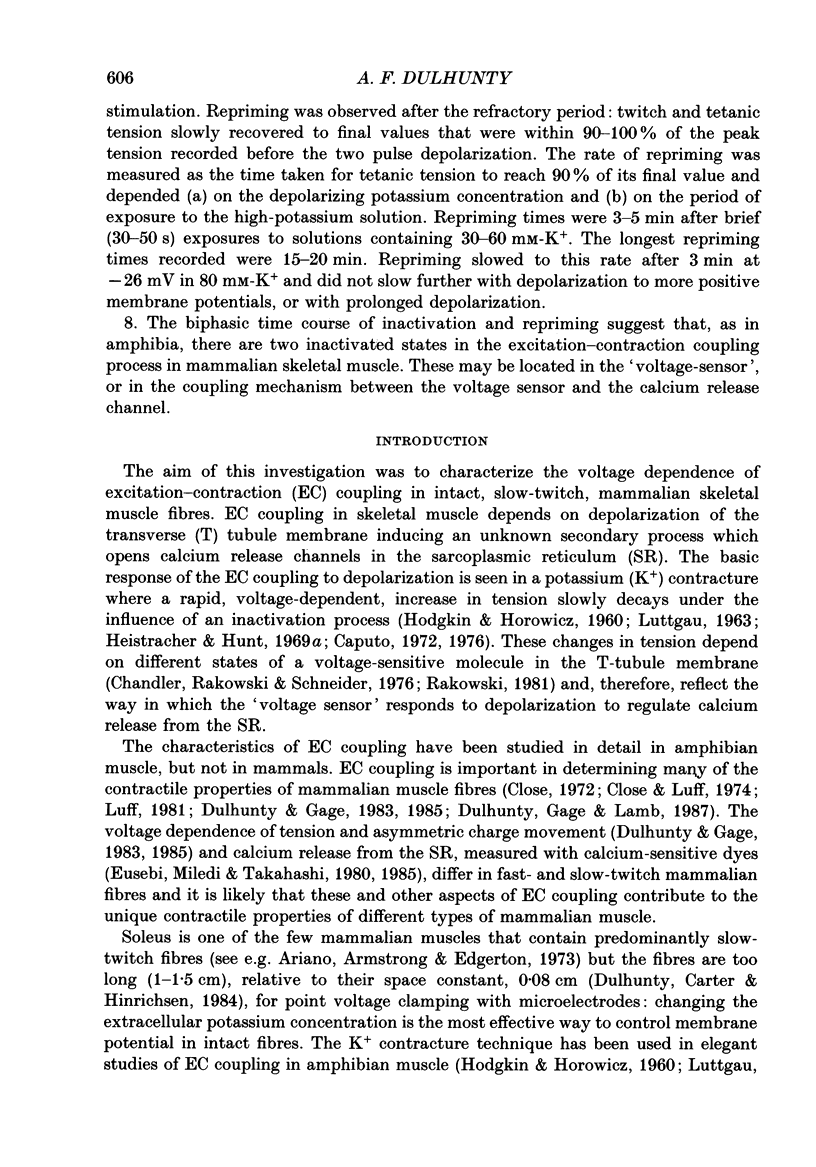
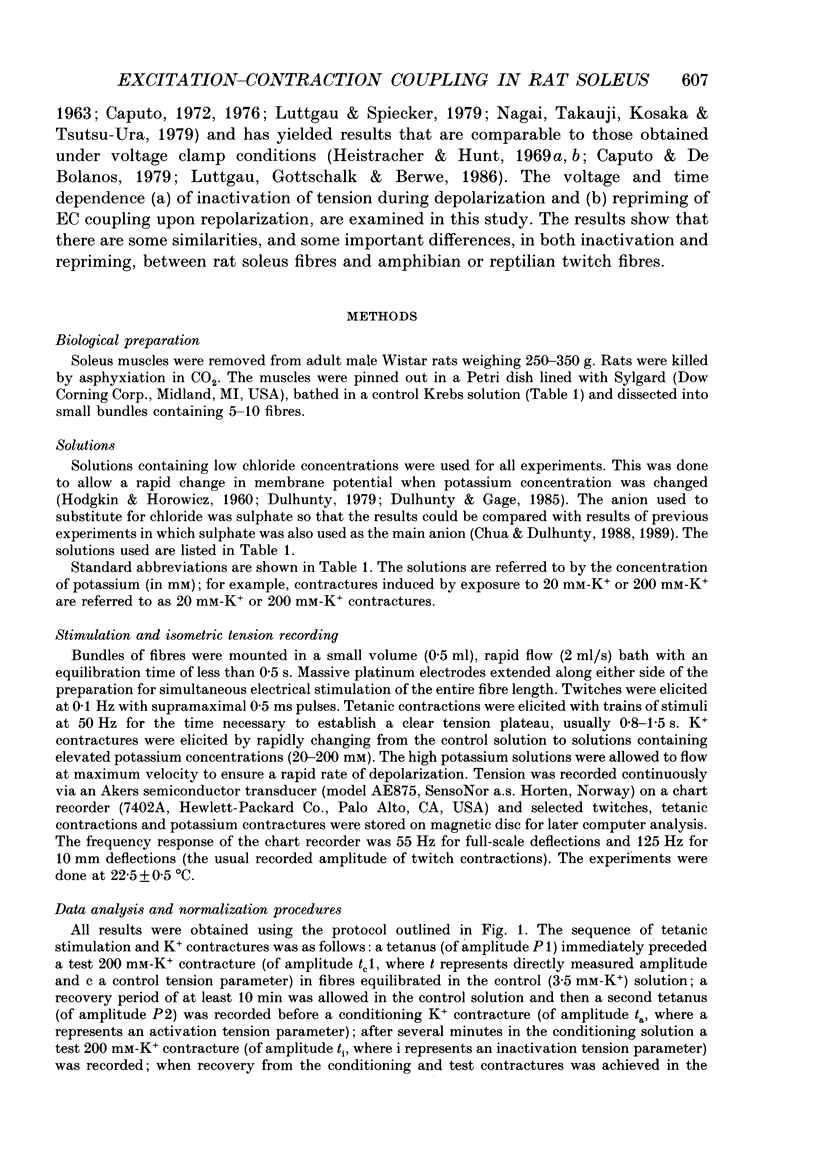
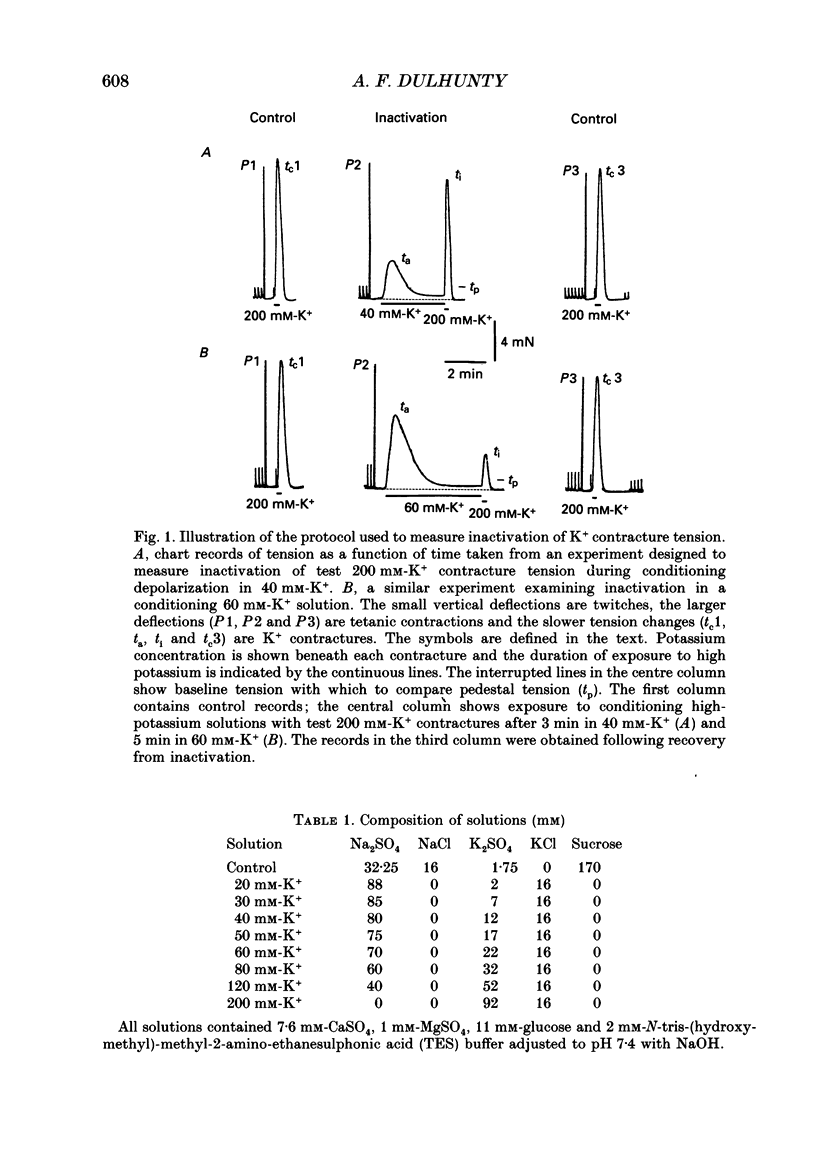
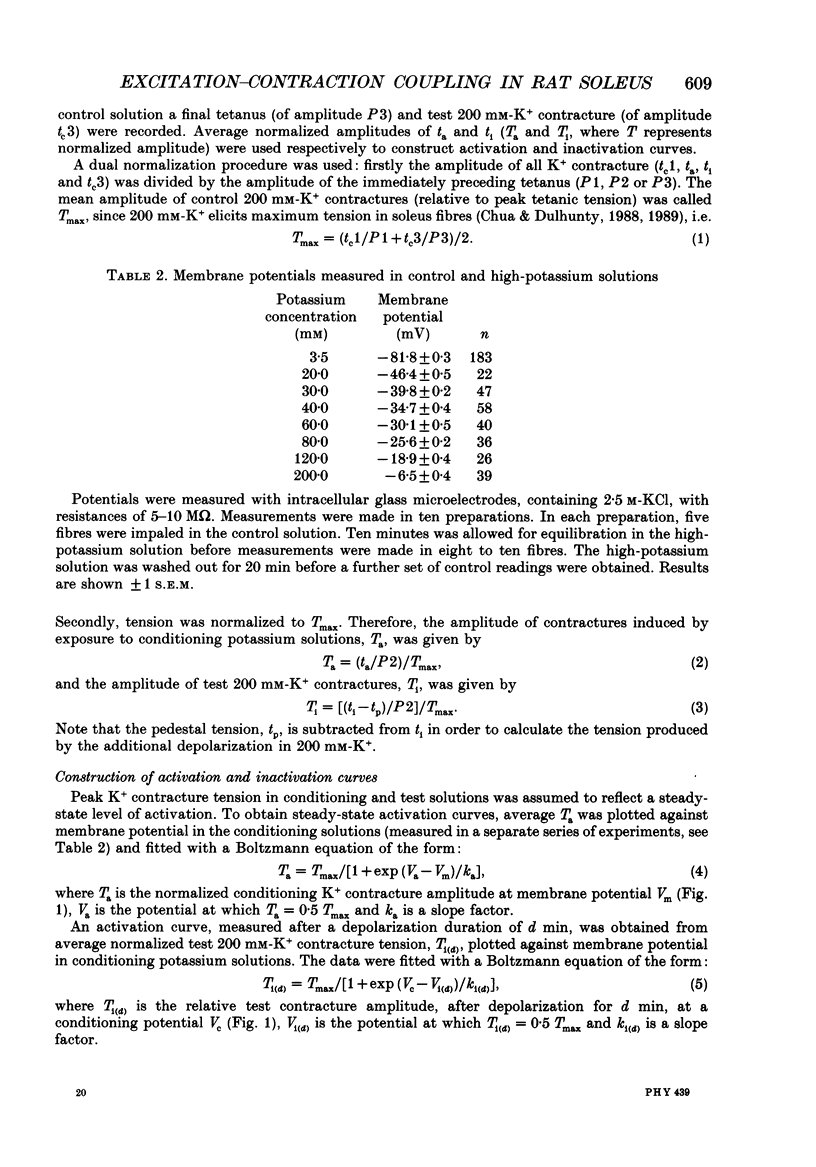
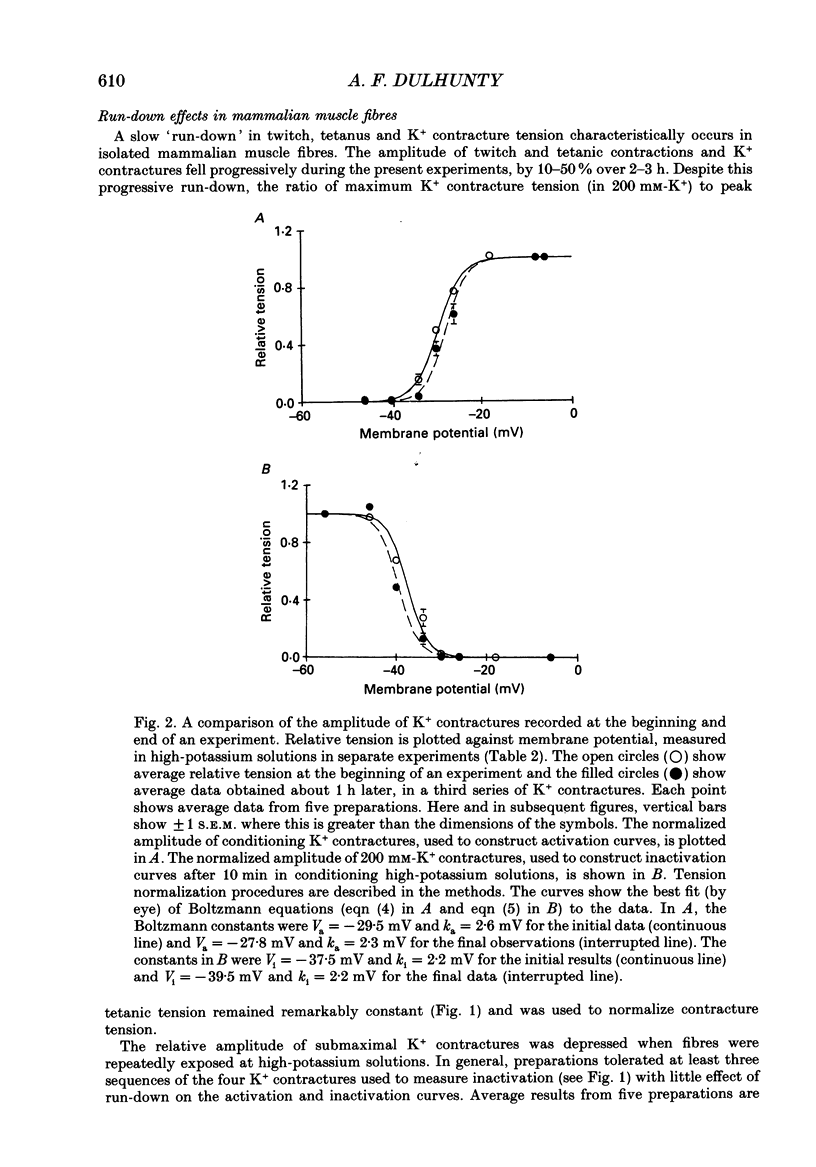
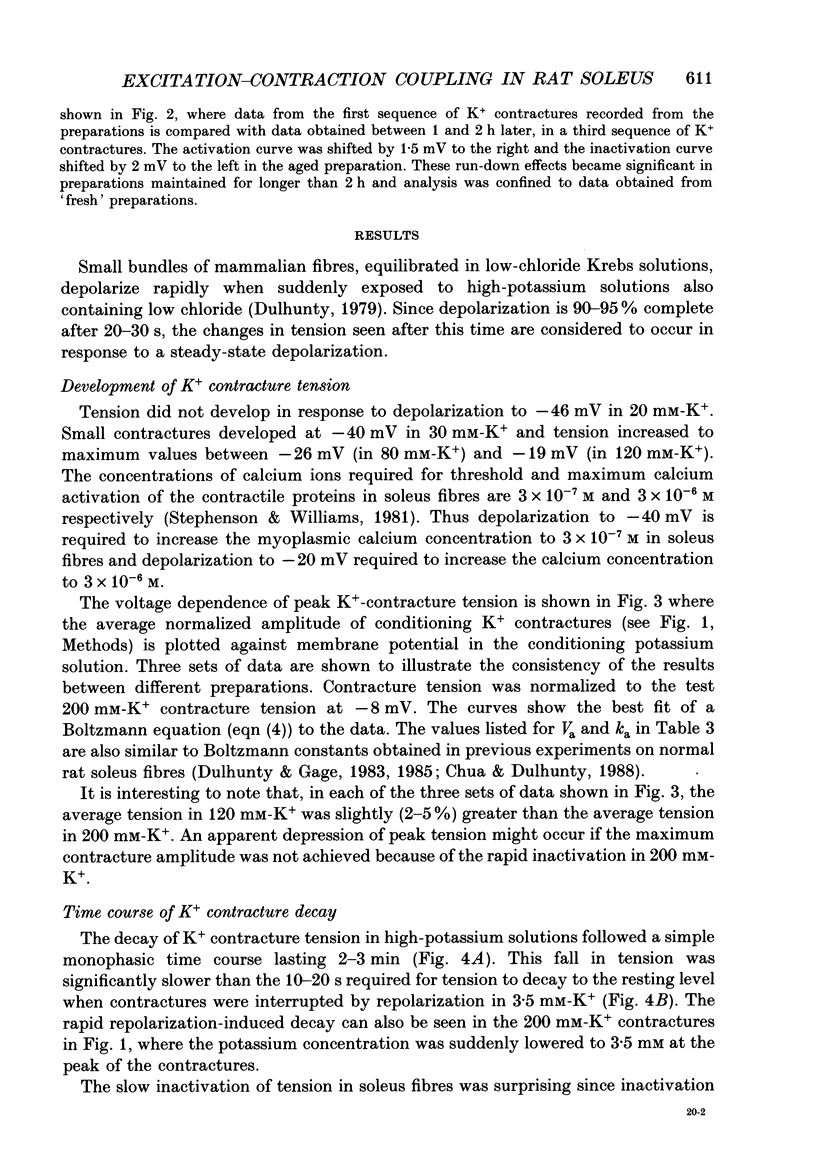
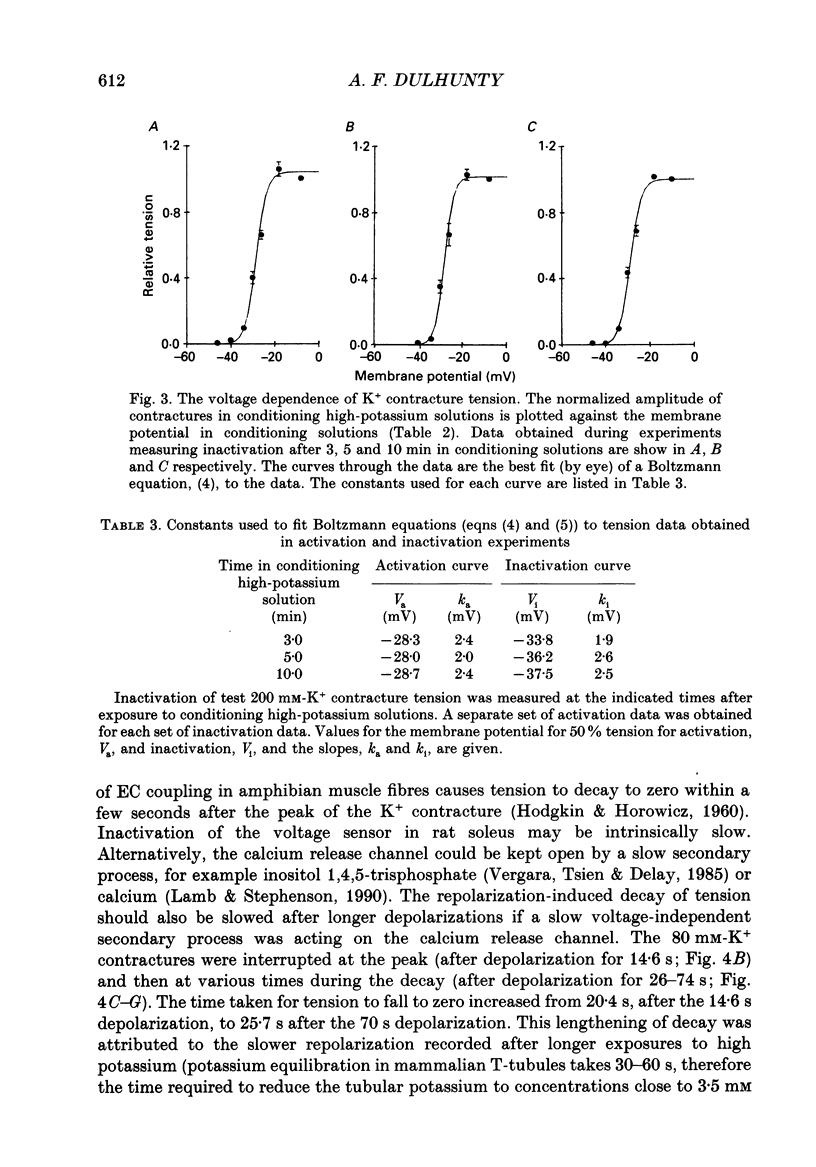
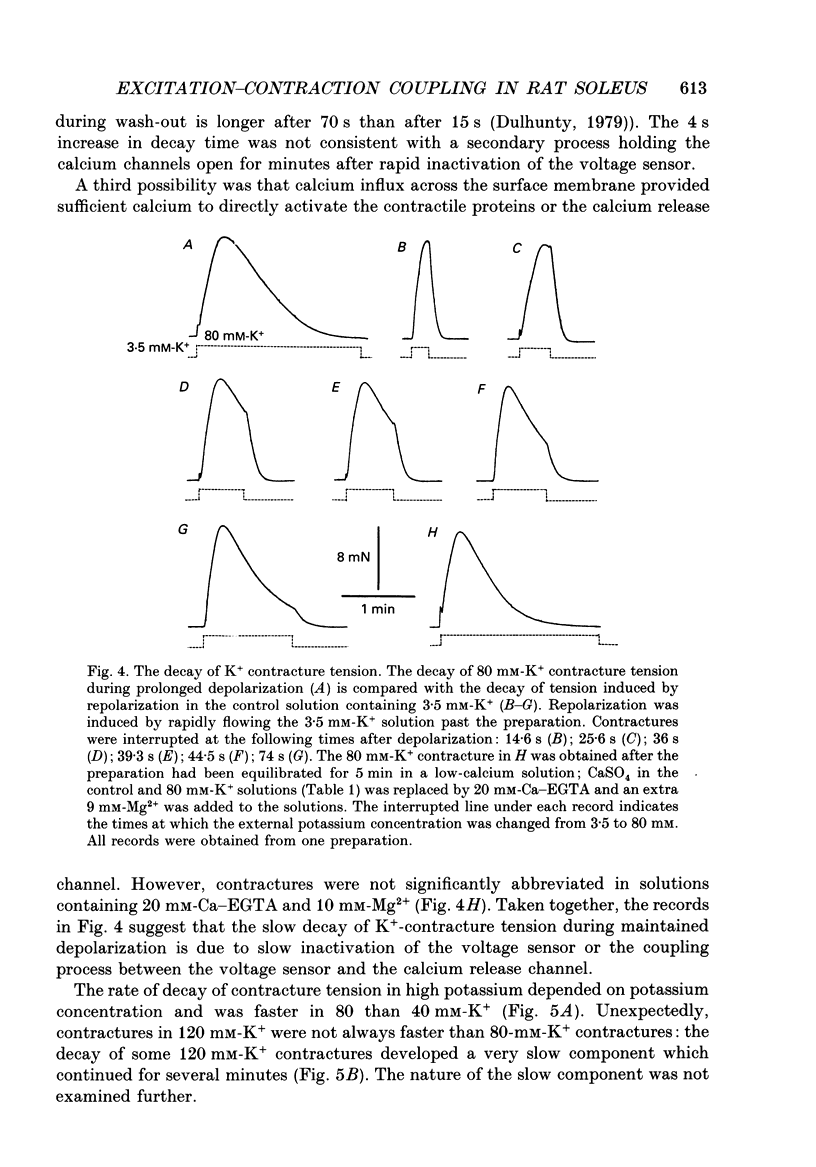
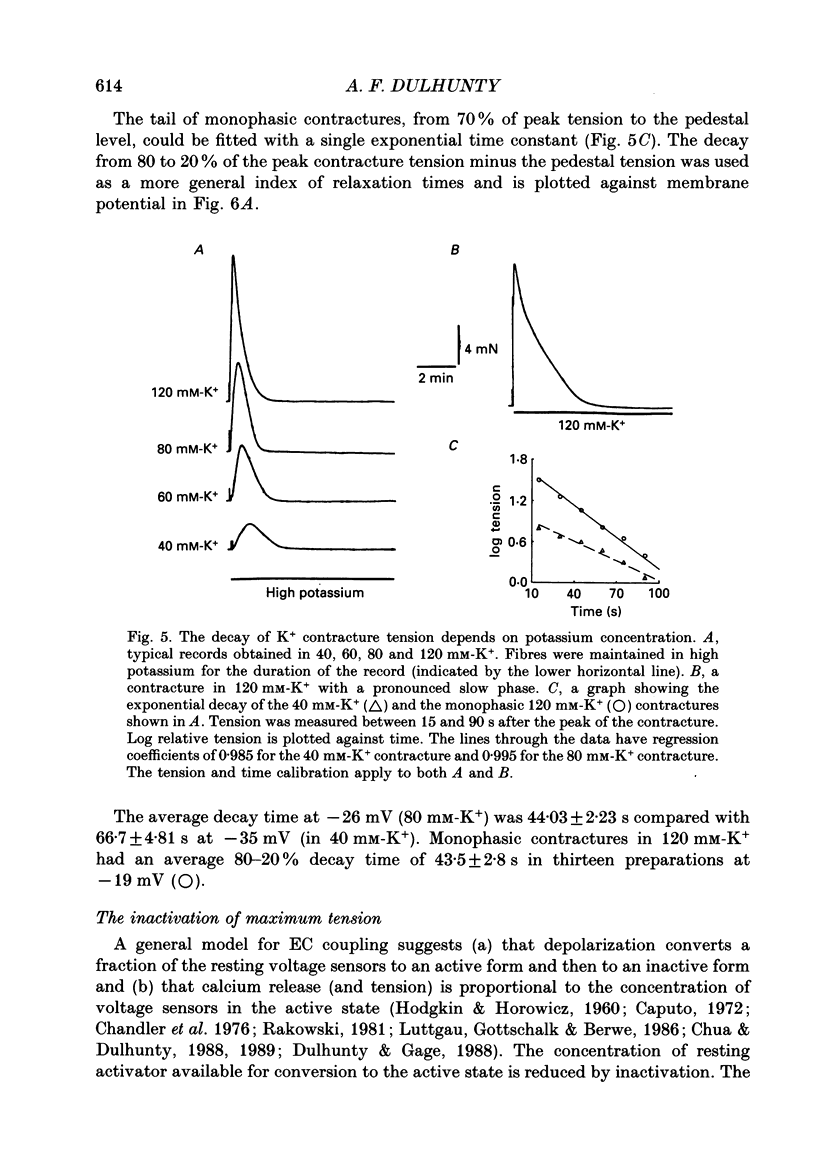
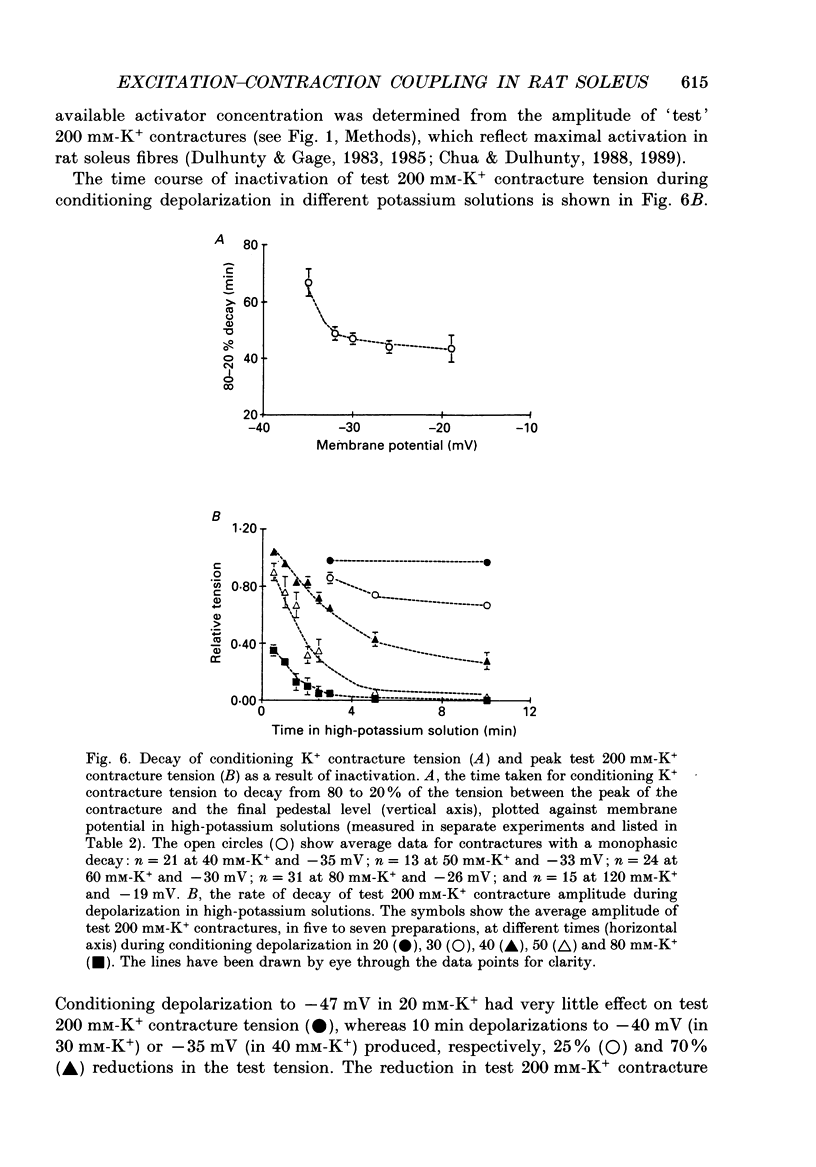
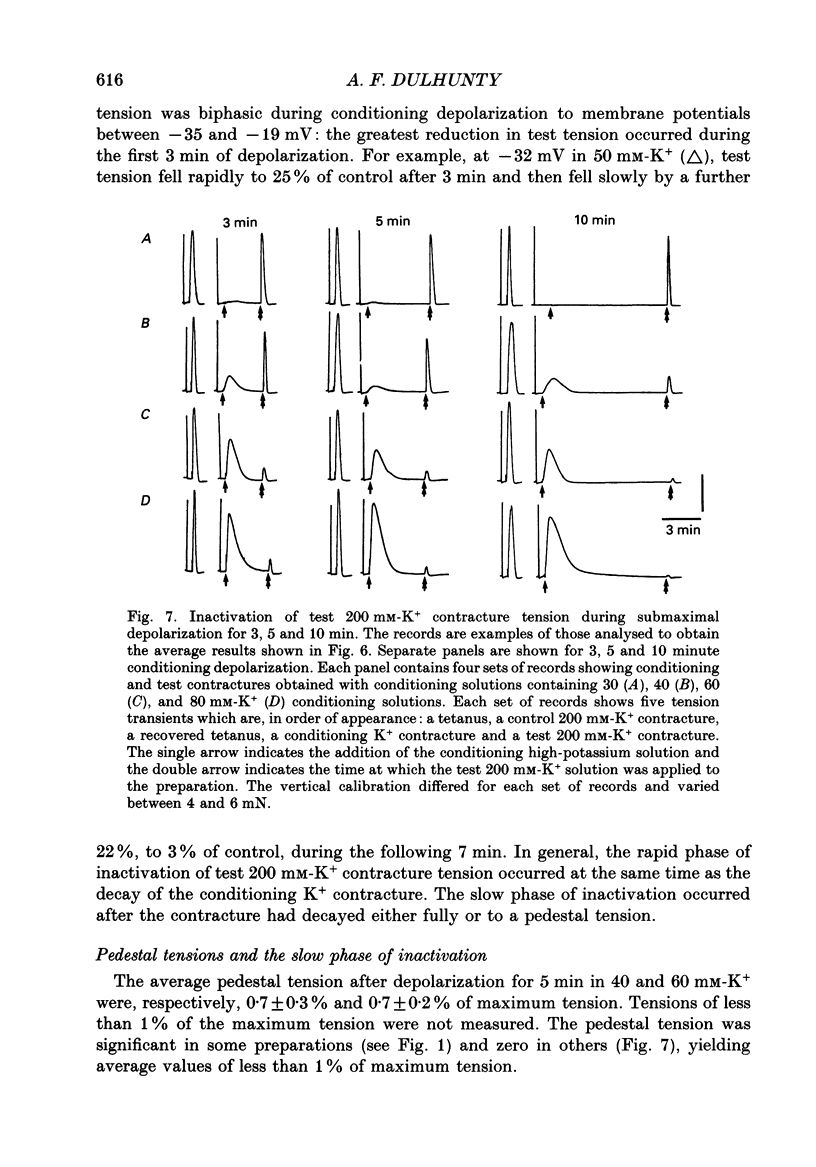
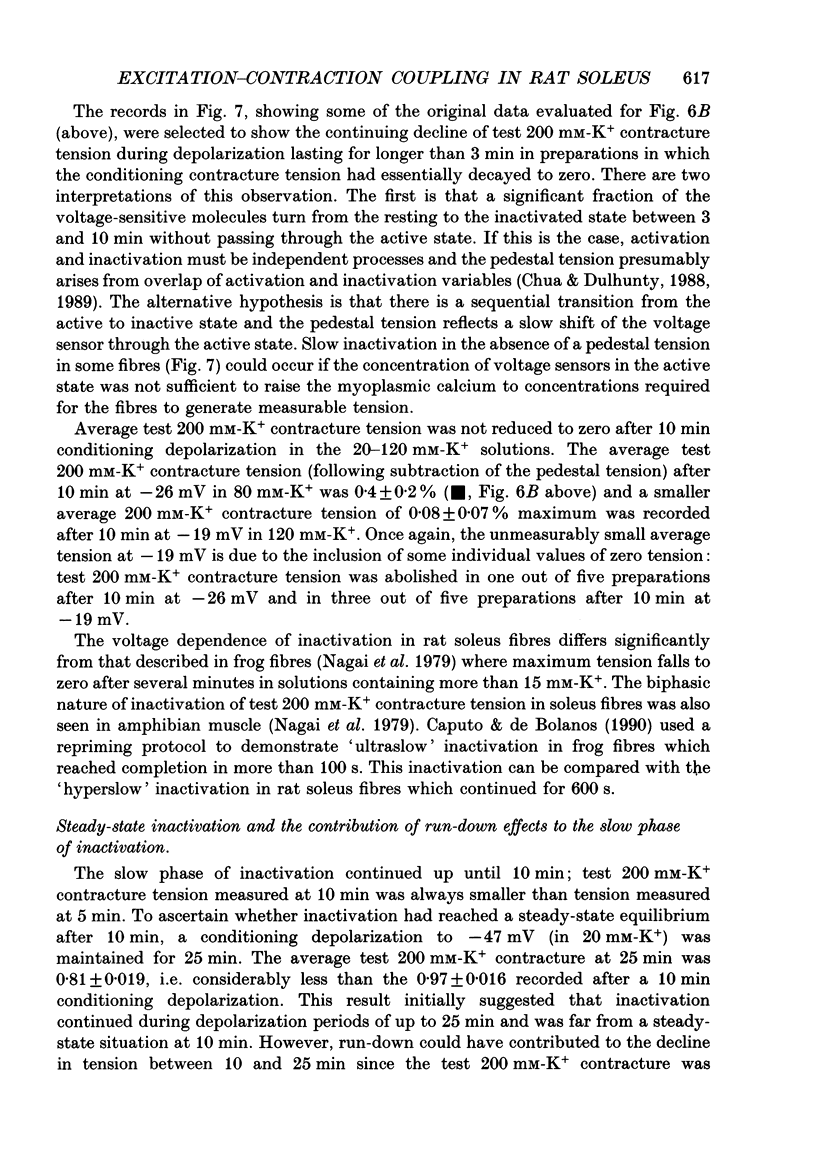
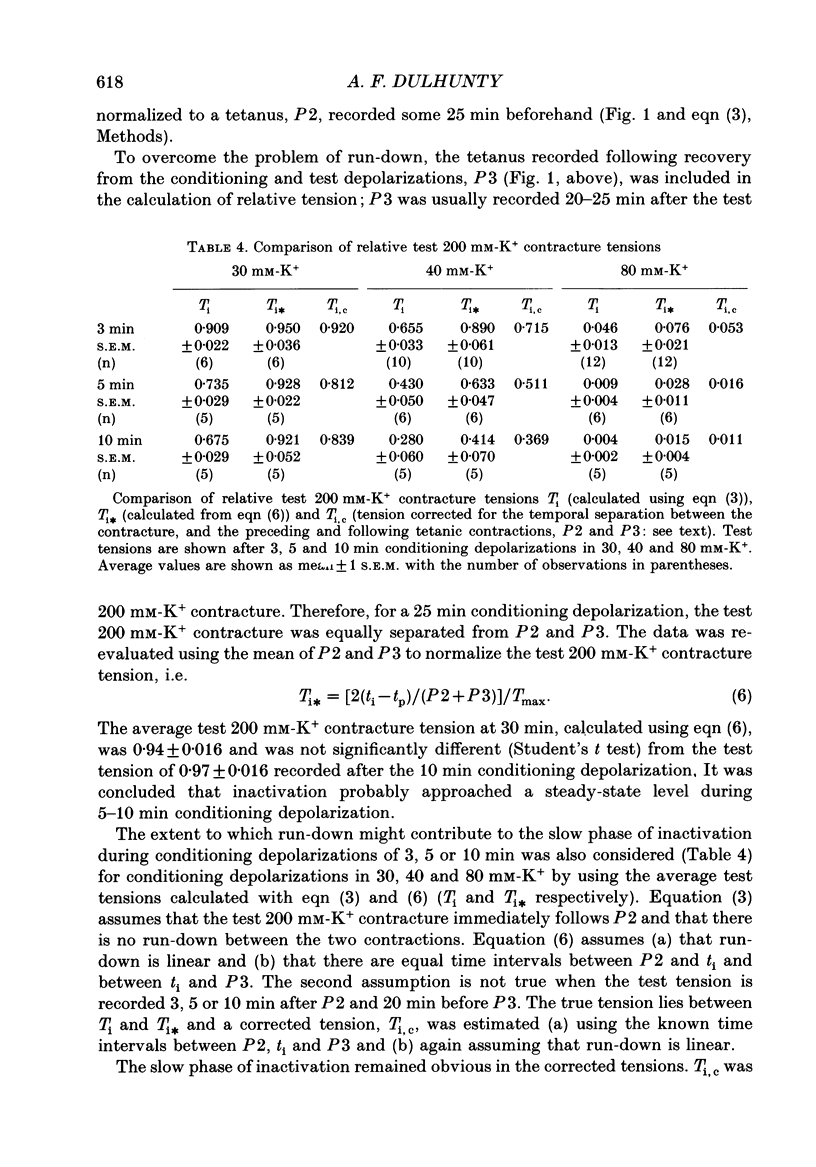
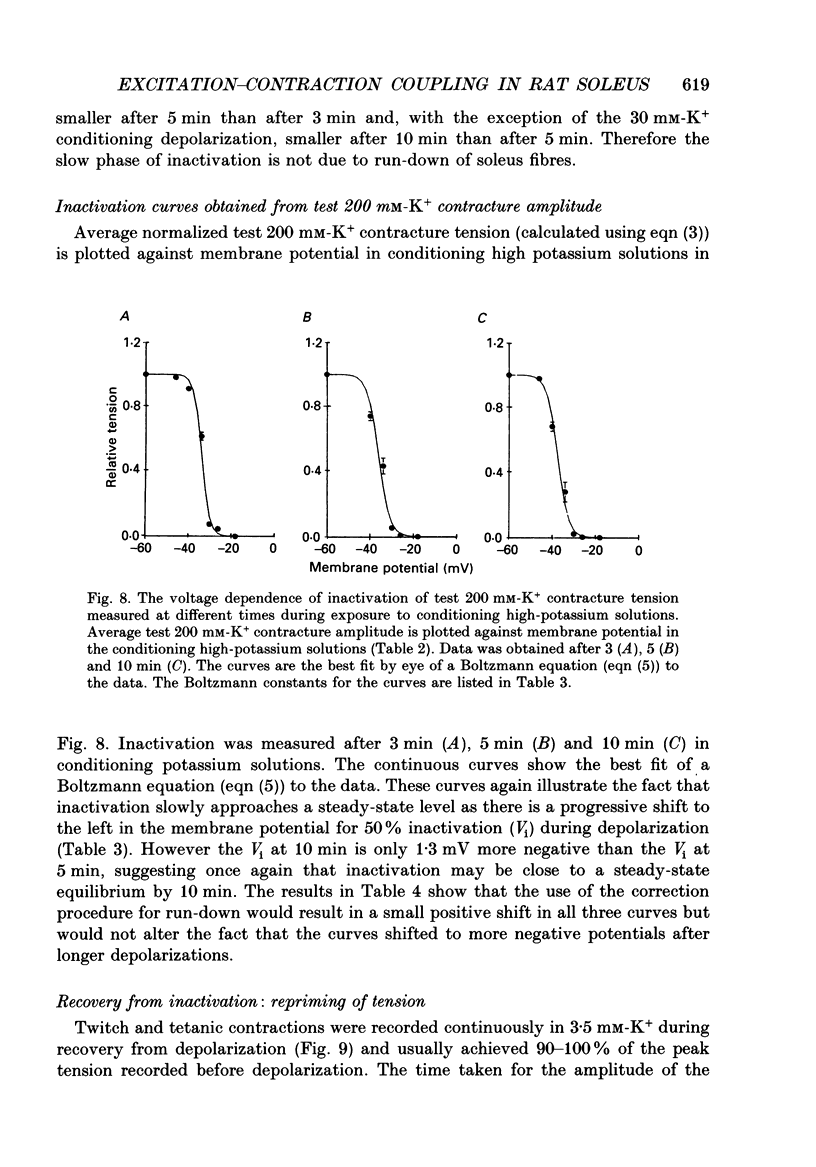
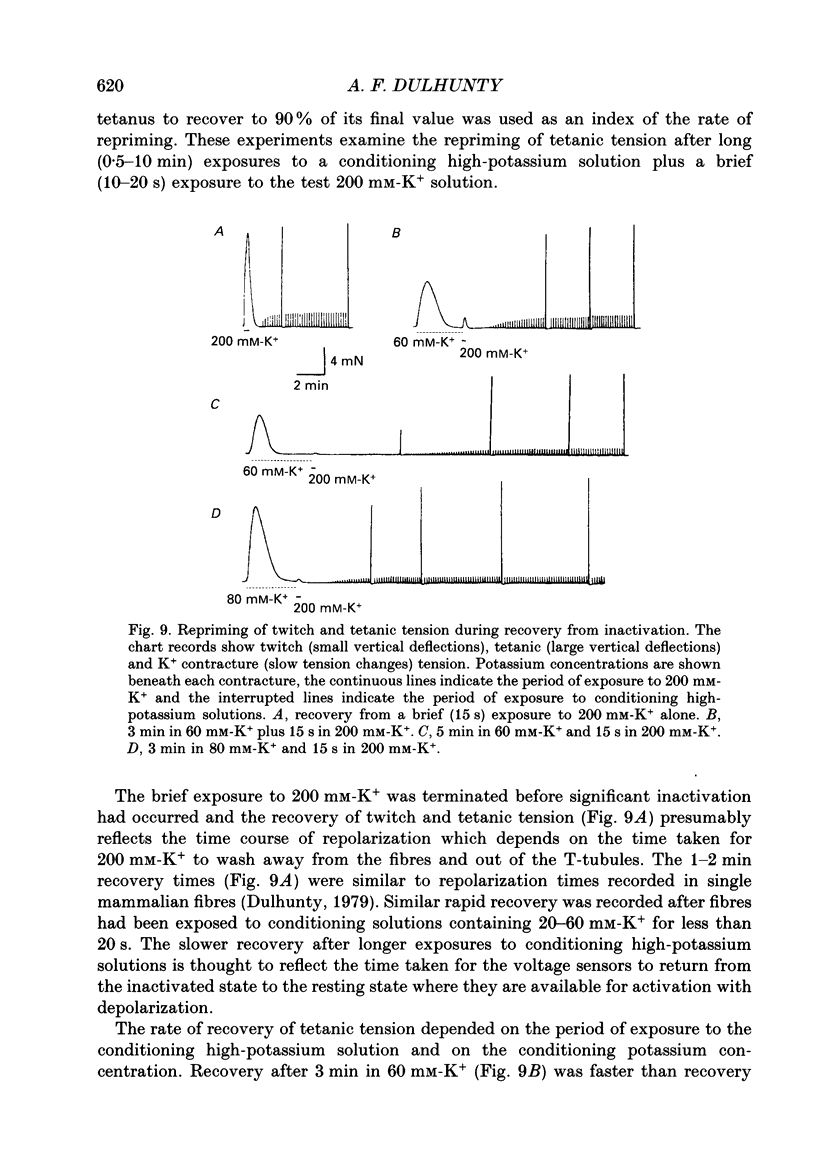
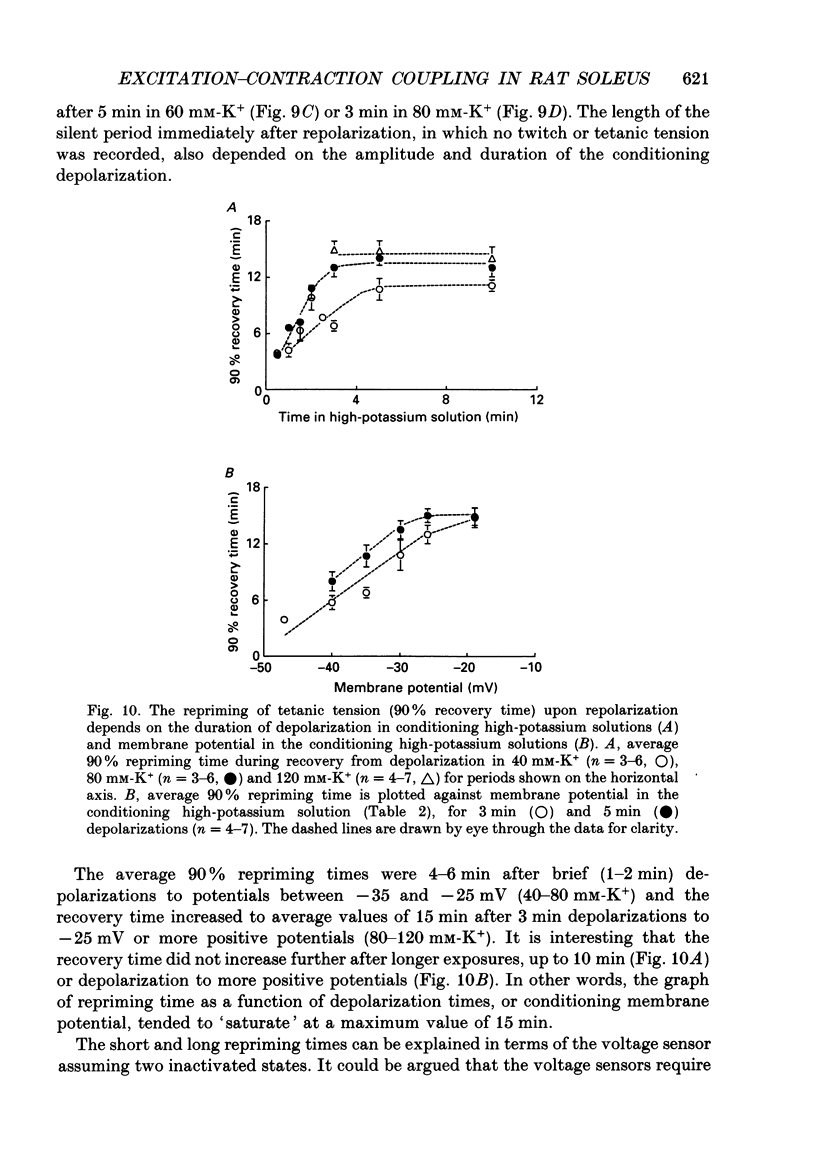
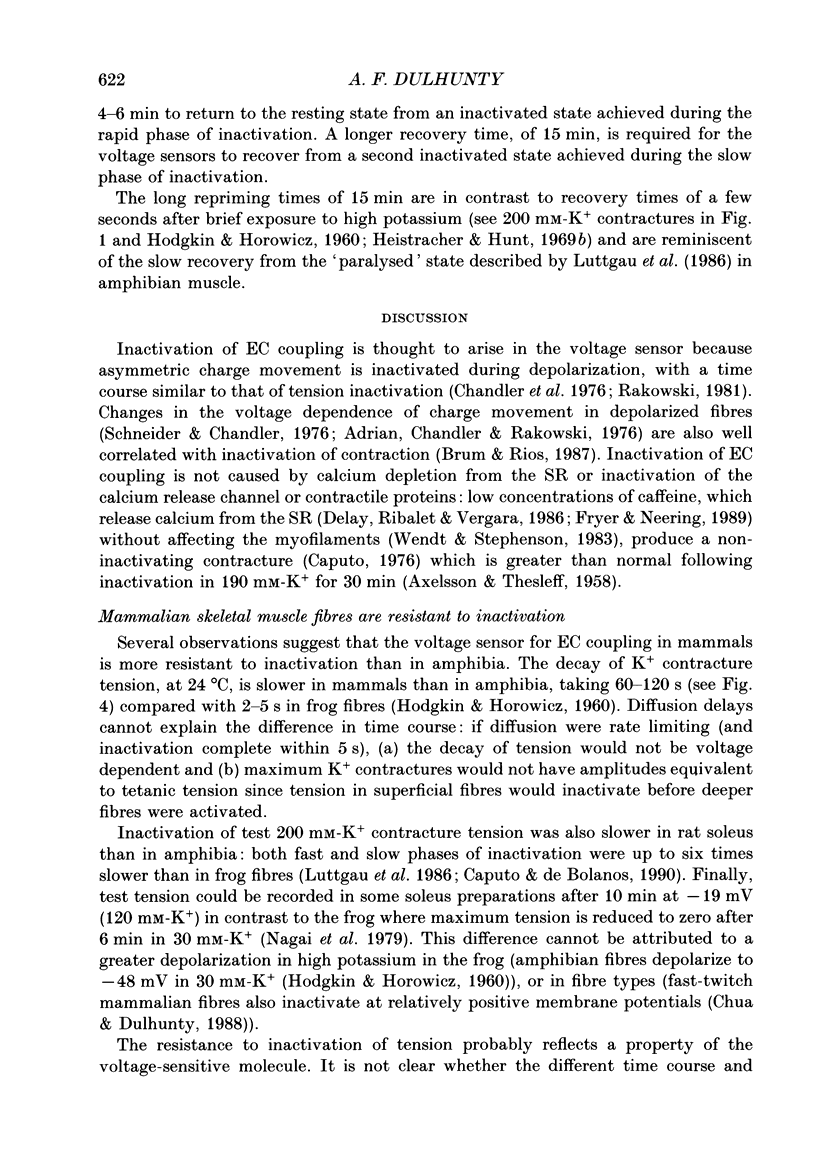
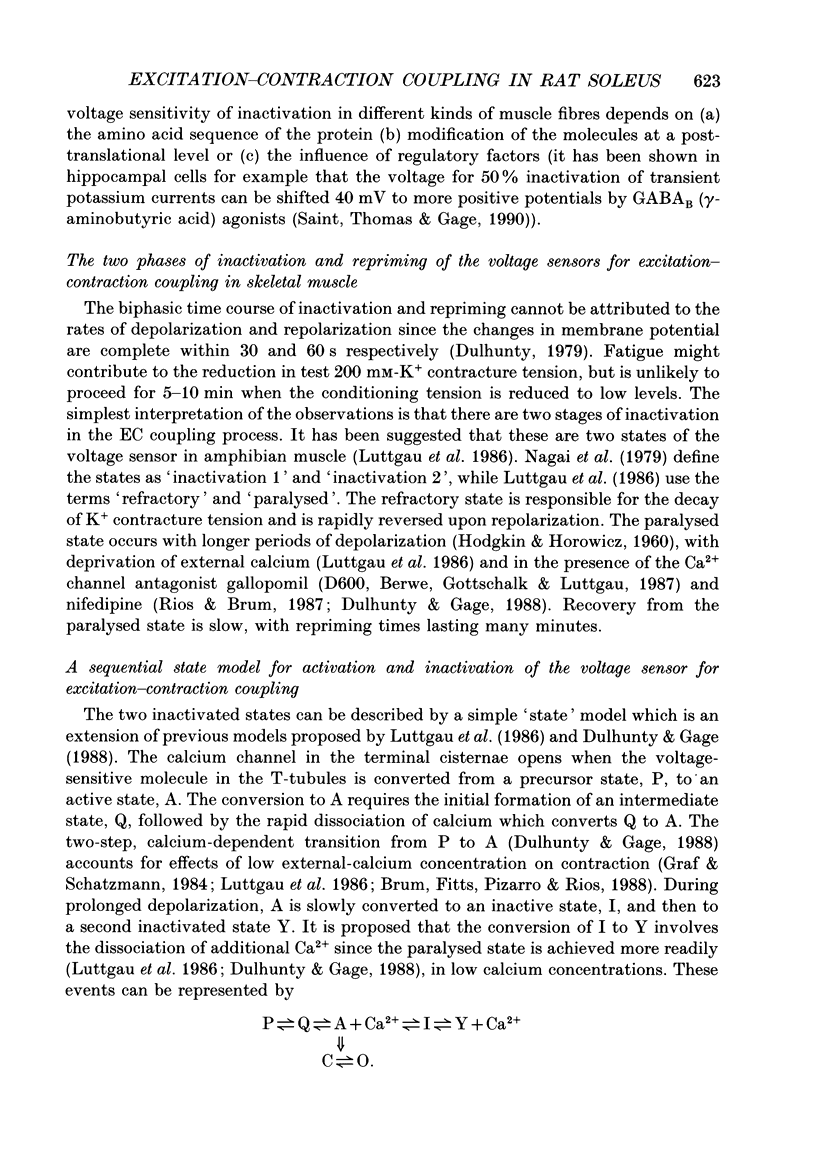
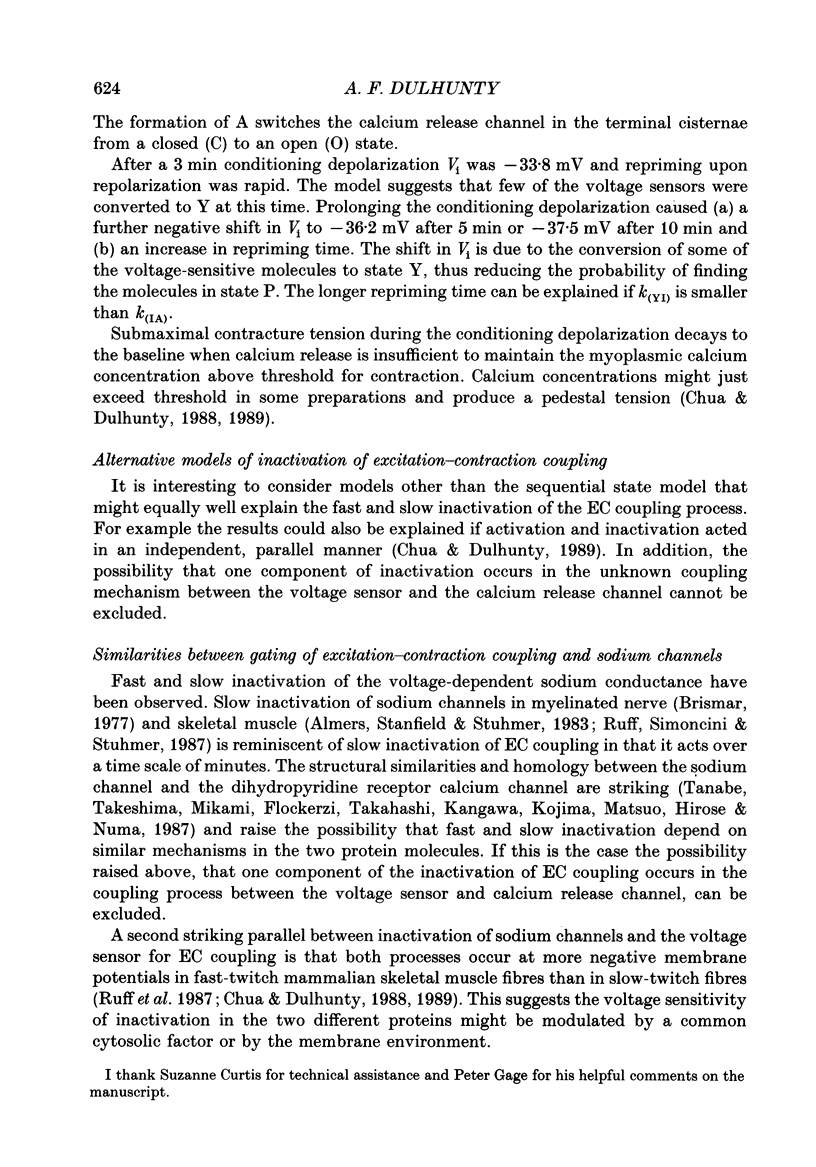
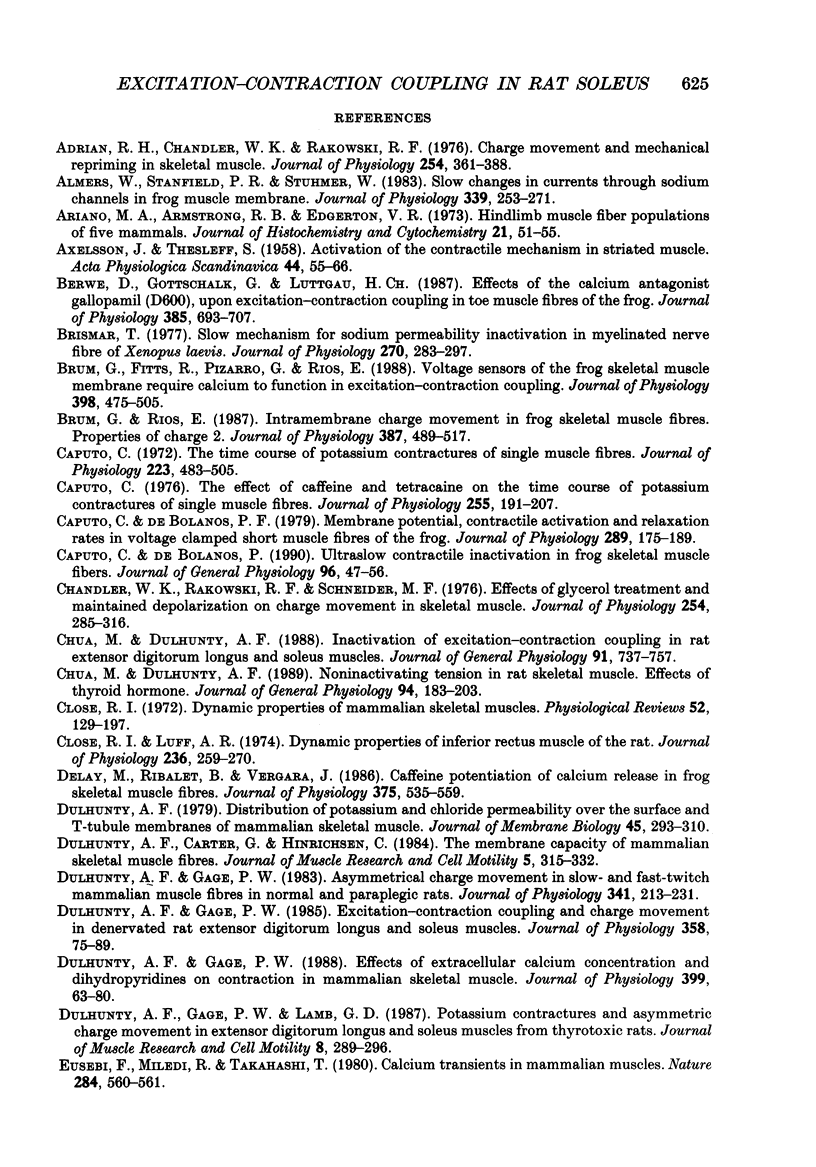
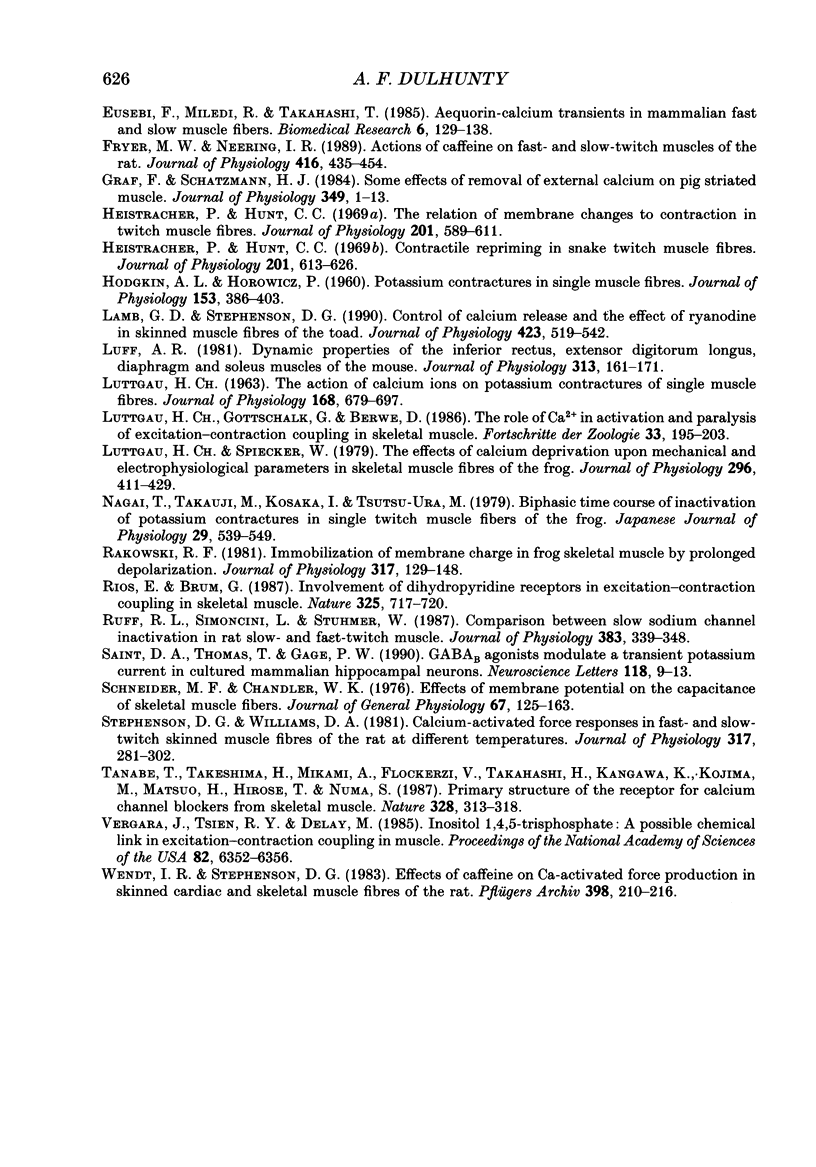
Selected References
These references are in PubMed. This may not be the complete list of references from this article.
- AXELSSON J., THESLEFF S. Activation of the contractile mechanism in striated muscle. Acta Physiol Scand. 1958 Oct 28;44(1):55–66. doi: 10.1111/j.1748-1716.1958.tb01608.x. [DOI] [PubMed] [Google Scholar]
- Adrian R. H., Chandler W. K., Rakowski R. F. Charge movement and mechanical repriming in skeletal muscle. J Physiol. 1976 Jan;254(2):361–388. doi: 10.1113/jphysiol.1976.sp011236. [DOI] [PMC free article] [PubMed] [Google Scholar]
- Almers W., Stanfield P. R., Stühmer W. Slow changes in currents through sodium channels in frog muscle membrane. J Physiol. 1983 Jun;339:253–271. doi: 10.1113/jphysiol.1983.sp014715. [DOI] [PMC free article] [PubMed] [Google Scholar]
- Ariano M. A., Armstrong R. B., Edgerton V. R. Hindlimb muscle fiber populations of five mammals. J Histochem Cytochem. 1973 Jan;21(1):51–55. doi: 10.1177/21.1.51. [DOI] [PubMed] [Google Scholar]
- Berwe D., Gottschalk G., Lüttgau H. C. Effects of the calcium antagonist gallopamil (D600) upon excitation-contraction coupling in toe muscle fibres of the frog. J Physiol. 1987 Apr;385:693–707. doi: 10.1113/jphysiol.1987.sp016515. [DOI] [PMC free article] [PubMed] [Google Scholar]
- Brismar T. Slow mechanism for sodium permeability inactivation in myelinated nerve fibre of Xenopus laevis. J Physiol. 1977 Sep;270(2):283–297. doi: 10.1113/jphysiol.1977.sp011952. [DOI] [PMC free article] [PubMed] [Google Scholar]
- Brum G., Fitts R., Pizarro G., Ríos E. Voltage sensors of the frog skeletal muscle membrane require calcium to function in excitation-contraction coupling. J Physiol. 1988 Apr;398:475–505. doi: 10.1113/jphysiol.1988.sp017053. [DOI] [PMC free article] [PubMed] [Google Scholar]
- Brum G., Rios E. Intramembrane charge movement in frog skeletal muscle fibres. Properties of charge 2. J Physiol. 1987 Jun;387:489–517. doi: 10.1113/jphysiol.1987.sp016586. [DOI] [PMC free article] [PubMed] [Google Scholar]
- Caputo C., Bolaños P. Ultraslow contractile inactivation in frog skeletal muscle fibers. J Gen Physiol. 1990 Jul;96(1):47–56. doi: 10.1085/jgp.96.1.47. [DOI] [PMC free article] [PubMed] [Google Scholar]
- Caputo C., Fernandez de Bolaños P. Membrane potential, contractile activation and relaxation rates in voltage clamped short muscle fibres of the frog. J Physiol. 1979 Apr;289:175–189. doi: 10.1113/jphysiol.1979.sp012731. [DOI] [PMC free article] [PubMed] [Google Scholar]
- Caputo C. The effect of caffeine and tetracaine on the time course of potassium contractures of single muscle fibres. J Physiol. 1976 Feb;255(1):191–207. doi: 10.1113/jphysiol.1976.sp011275. [DOI] [PMC free article] [PubMed] [Google Scholar]
- Caputo C. The time course of potassium contractures of single muscle fibres. J Physiol. 1972 Jun;223(2):483–505. doi: 10.1113/jphysiol.1972.sp009859. [DOI] [PMC free article] [PubMed] [Google Scholar]
- Chandler W. K., Rakowski R. F., Schneider M. F. Effects of glycerol treatment and maintained depolarization on charge movement in skeletal muscle. J Physiol. 1976 Jan;254(2):285–316. doi: 10.1113/jphysiol.1976.sp011233. [DOI] [PMC free article] [PubMed] [Google Scholar]
- Chua M., Dulhunty A. F. Inactivation of excitation-contraction coupling in rat extensor digitorum longus and soleus muscles. J Gen Physiol. 1988 May;91(5):737–757. doi: 10.1085/jgp.91.5.737. [DOI] [PMC free article] [PubMed] [Google Scholar]
- Chua M., Dulhunty A. F. Noninactivating tension in rat skeletal muscle. Effects of thyroid hormone. J Gen Physiol. 1989 Jul;94(1):183–203. doi: 10.1085/jgp.94.1.183. [DOI] [PMC free article] [PubMed] [Google Scholar]
- Close R. I. Dynamic properties of mammalian skeletal muscles. Physiol Rev. 1972 Jan;52(1):129–197. doi: 10.1152/physrev.1972.52.1.129. [DOI] [PubMed] [Google Scholar]
- Close R. I., Luff A. R. Dynamic properties of inferior rectus muscle of the rat. J Physiol. 1974 Jan;236(2):259–270. doi: 10.1113/jphysiol.1974.sp010434. [DOI] [PMC free article] [PubMed] [Google Scholar]
- Delay M., Ribalet B., Vergara J. Caffeine potentiation of calcium release in frog skeletal muscle fibres. J Physiol. 1986 Jun;375:535–559. doi: 10.1113/jphysiol.1986.sp016132. [DOI] [PMC free article] [PubMed] [Google Scholar]
- Dulhunty A. F. Distribution of potassium and chloride permeability over the surface and T-tubule membranes of mammalian skeletal muscle. J Membr Biol. 1979 Apr 9;45(3-4):293–310. doi: 10.1007/BF01869290. [DOI] [PubMed] [Google Scholar]
- Dulhunty A. F., Gage P. W. Asymmetrical charge movement in slow- and fast-twitch mammalian muscle fibres in normal and paraplegic rats. J Physiol. 1983 Aug;341:213–231. doi: 10.1113/jphysiol.1983.sp014802. [DOI] [PMC free article] [PubMed] [Google Scholar]
- Dulhunty A. F., Gage P. W. Effects of extracellular calcium concentration and dihydropyridines on contraction in mammalian skeletal muscle. J Physiol. 1988 May;399:63–80. doi: 10.1113/jphysiol.1988.sp017068. [DOI] [PMC free article] [PubMed] [Google Scholar]
- Dulhunty A. F., Gage P. W. Excitation-contraction coupling and charge movement in denervated rat extensor digitorum longus and soleus muscles. J Physiol. 1985 Jan;358:75–89. doi: 10.1113/jphysiol.1985.sp015541. [DOI] [PMC free article] [PubMed] [Google Scholar]
- Dulhunty A. F., Gage P. W., Lamb G. D. Potassium contractures and asymmetric charge movement in extensor digitorum longus and soleus muscles from thyrotoxic rats. J Muscle Res Cell Motil. 1987 Aug;8(4):289–296. doi: 10.1007/BF01568885. [DOI] [PubMed] [Google Scholar]
- Dulhunty A., Carter G., Hinrichsen C. The membrane capacity of mammalian skeletal muscle fibres. J Muscle Res Cell Motil. 1984 Jun;5(3):315–332. doi: 10.1007/BF00713110. [DOI] [PubMed] [Google Scholar]
- Eusebi F., Miledi R., Takahashi T. Calcium transients in mammalian muscles. Nature. 1980 Apr 10;284(5756):560–561. doi: 10.1038/284560a0. [DOI] [PubMed] [Google Scholar]
- Fryer M. W., Neering I. R. Actions of caffeine on fast- and slow-twitch muscles of the rat. J Physiol. 1989 Sep;416:435–454. doi: 10.1113/jphysiol.1989.sp017770. [DOI] [PMC free article] [PubMed] [Google Scholar]
- Graf F., Schatzmann H. J. Some effects of removal of external calcium on pig striated muscle. J Physiol. 1984 Apr;349:1–13. doi: 10.1113/jphysiol.1984.sp015138. [DOI] [PMC free article] [PubMed] [Google Scholar]
- HODGKIN A. L., HOROWICZ P. Potassium contractures in single muscle fibres. J Physiol. 1960 Sep;153:386–403. doi: 10.1113/jphysiol.1960.sp006541. [DOI] [PMC free article] [PubMed] [Google Scholar]
- Heistracher P., Hunt C. C. Contractile repriming in snake twitch muscle fibres. J Physiol. 1969 May;201(3):613–626. doi: 10.1113/jphysiol.1969.sp008775. [DOI] [PMC free article] [PubMed] [Google Scholar]
- Heistracher P., Hunt C. C. The relation of membrane changes ot contraction in twitch muscle fibres. J Physiol. 1969 May;201(3):589–611. doi: 10.1113/jphysiol.1969.sp008774. [DOI] [PMC free article] [PubMed] [Google Scholar]
- Lamb G. D., Stephenson D. G. Control of calcium release and the effect of ryanodine in skinned muscle fibres of the toad. J Physiol. 1990 Apr;423:519–542. doi: 10.1113/jphysiol.1990.sp018037. [DOI] [PMC free article] [PubMed] [Google Scholar]
- Luff A. R. Dynamic properties of the inferior rectus, extensor digitorum longus, diaphragm and soleus muscles of the mouse. J Physiol. 1981;313:161–171. doi: 10.1113/jphysiol.1981.sp013656. [DOI] [PMC free article] [PubMed] [Google Scholar]
- Lüttgau H. C., Spiecker W. The effects of calcium deprivation upon mechanical and electrophysiological parameters in skeletal muscle fibres of the frog. J Physiol. 1979 Nov;296:411–429. doi: 10.1113/jphysiol.1979.sp013013. [DOI] [PMC free article] [PubMed] [Google Scholar]
- Nagai T., Takauji M., Kosaka I., Tsutsu-ura M. Biphasic time course of inactivation of potassium contractures in single twitch muscle fibers of the frog. Jpn J Physiol. 1979;29(5):539–549. doi: 10.2170/jjphysiol.29.539. [DOI] [PubMed] [Google Scholar]
- Rakowski R. F. Immobilization of membrane charge in frog skeletal muscle by prolonged depolarization. J Physiol. 1981 Aug;317:129–148. doi: 10.1113/jphysiol.1981.sp013817. [DOI] [PMC free article] [PubMed] [Google Scholar]
- Rios E., Brum G. Involvement of dihydropyridine receptors in excitation-contraction coupling in skeletal muscle. Nature. 1987 Feb 19;325(6106):717–720. doi: 10.1038/325717a0. [DOI] [PubMed] [Google Scholar]
- Ruff R. L., Simoncini L., Stühmer W. Comparison between slow sodium channel inactivation in rat slow- and fast-twitch muscle. J Physiol. 1987 Feb;383:339–348. doi: 10.1113/jphysiol.1987.sp016412. [DOI] [PMC free article] [PubMed] [Google Scholar]
- Saint D. A., Thomas T., Gage P. W. GABAB agonists modulate a transient potassium current in cultured mammalian hippocampal neurons. Neurosci Lett. 1990 Oct 2;118(1):9–13. doi: 10.1016/0304-3940(90)90236-3. [DOI] [PubMed] [Google Scholar]
- Schneider M. F., Chandler W. K. Effects of membrane potential on the capacitance of skeletal muscle fibers. J Gen Physiol. 1976 Feb;67(2):125–163. doi: 10.1085/jgp.67.2.125. [DOI] [PMC free article] [PubMed] [Google Scholar]
- Stephenson D. G., Williams D. A. Calcium-activated force responses in fast- and slow-twitch skinned muscle fibres of the rat at different temperatures. J Physiol. 1981 Aug;317:281–302. doi: 10.1113/jphysiol.1981.sp013825. [DOI] [PMC free article] [PubMed] [Google Scholar]
- Tanabe T., Takeshima H., Mikami A., Flockerzi V., Takahashi H., Kangawa K., Kojima M., Matsuo H., Hirose T., Numa S. Primary structure of the receptor for calcium channel blockers from skeletal muscle. Nature. 1987 Jul 23;328(6128):313–318. doi: 10.1038/328313a0. [DOI] [PubMed] [Google Scholar]
- Vergara J., Tsien R. Y., Delay M. Inositol 1,4,5-trisphosphate: a possible chemical link in excitation-contraction coupling in muscle. Proc Natl Acad Sci U S A. 1985 Sep;82(18):6352–6356. doi: 10.1073/pnas.82.18.6352. [DOI] [PMC free article] [PubMed] [Google Scholar]
- Wendt I. R., Stephenson D. G. Effects of caffeine on Ca-activated force production in skinned cardiac and skeletal muscle fibres of the rat. Pflugers Arch. 1983 Aug;398(3):210–216. doi: 10.1007/BF00657153. [DOI] [PubMed] [Google Scholar]