Abstract
1. To investigate the relative contributions of increases in cardiac output and arteriovenous oxygen difference to the increase in oxygen consumption during exercise, the ventilatory and cardiovascular responses to a sudden transition from unloaded cycling to 70 or 80 W were measured in six normal healthy subjects. 2. Oxygen consumption (VO2) was measured breath-by-breath and corrected for changes in lung gas stores. Cardiac output (Q) was measured beat-by-beat using pulsed Doppler ultrasound, and blood pressure was measured beat-by-beat using a non-invasive finger cuff (Finapres). All data were calculated off-line, second-by-second. 3. Arteriovenous oxygen difference (A-VO2) was calculated from Q and VO2 using the Fick Principle. Left ventricular afterload was calculated by dividing Q by mean blood pressure. 4. The data for Q and VO2 were closely fitted by single exponential curves (mean r2 0.84 and 0.90 respectively; r is the correlation coefficient). These curves yielded mean time constants for the increases in Q and VO2 of 28 and 55 s respectively following the increase in exercise level. In each individual subject, the time course of adjustment of Q was faster than that of VO2. There was a mean lag of 15 s from the start of the new exercise level before the derived A-V O2 began to increase; the mean time constant for A-V O2 was 57 s. 5. If A-V O2 had remained constant, the observed rise in Q alone would have resulted in an average of 87% of the increase in VO2 which was observed after 5 s. If Q had remained constant, the observed increase in A-V O2 would have led to only 8% of the actual increase in VO2 after 5 s. 6. Mean and systolic blood pressure rose and afterload fell immediately after the onset of the increased workload. The time constants of the systolic blood pressure and afterload responses to exercise varied widely and ranged from 37 to 81 and 10 to 26 s respectively (n = 4). 7. We conclude that Q is responsible for most of the early increase in VO2 following a sudden increase in exercise workload. Blood pressure responses to exercise are slower than Q and VO2 responses, probably due to the rapid decrease in afterload. 8. The dominant contribution of Q to adaptation to changing workload may be physiologically important particularly in heart disease, where decreased ability to increase cardiac output may limit the capacity to cope with changing metabolic needs during everyday activities.
Full text
PDF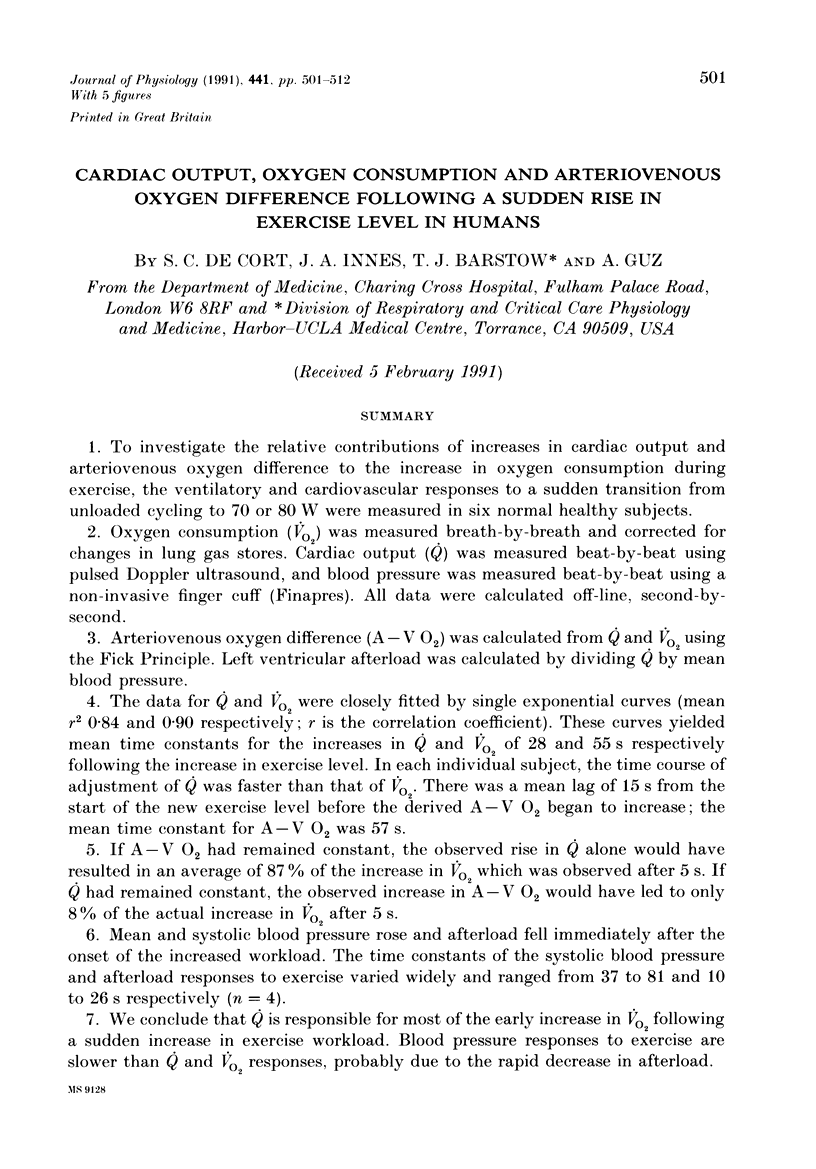
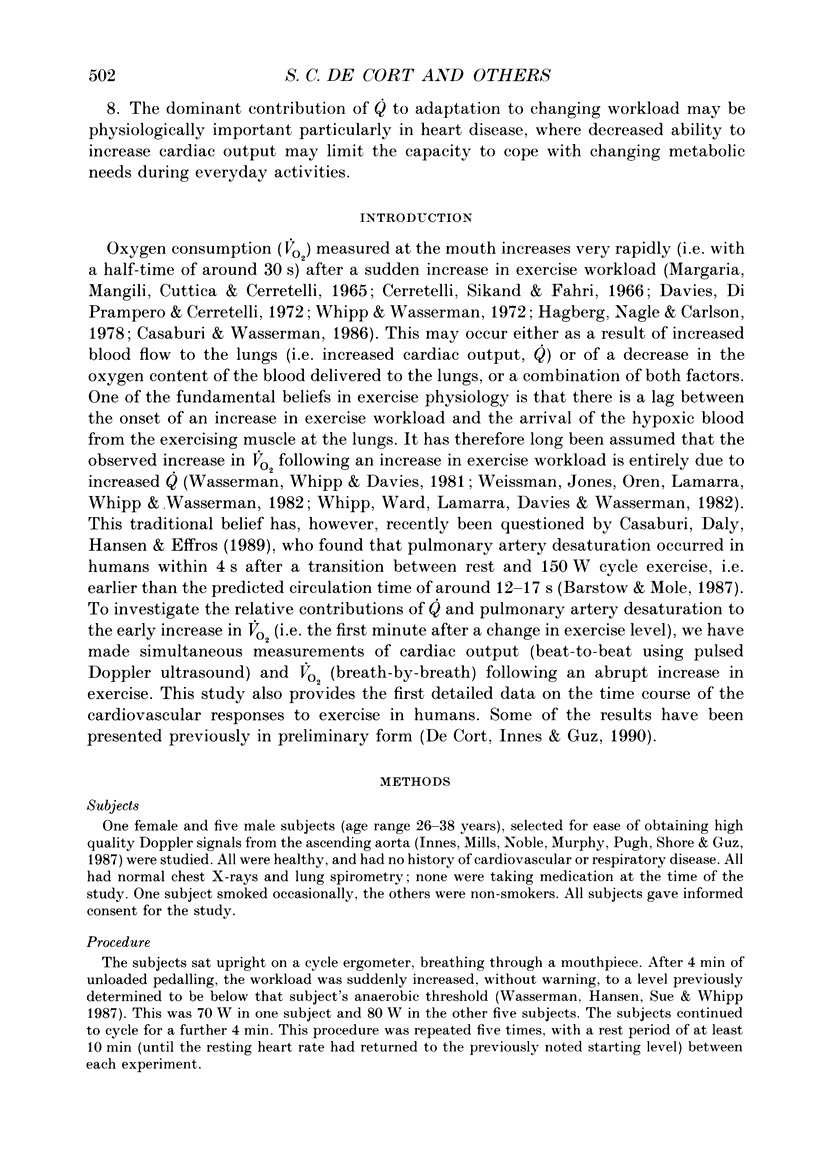
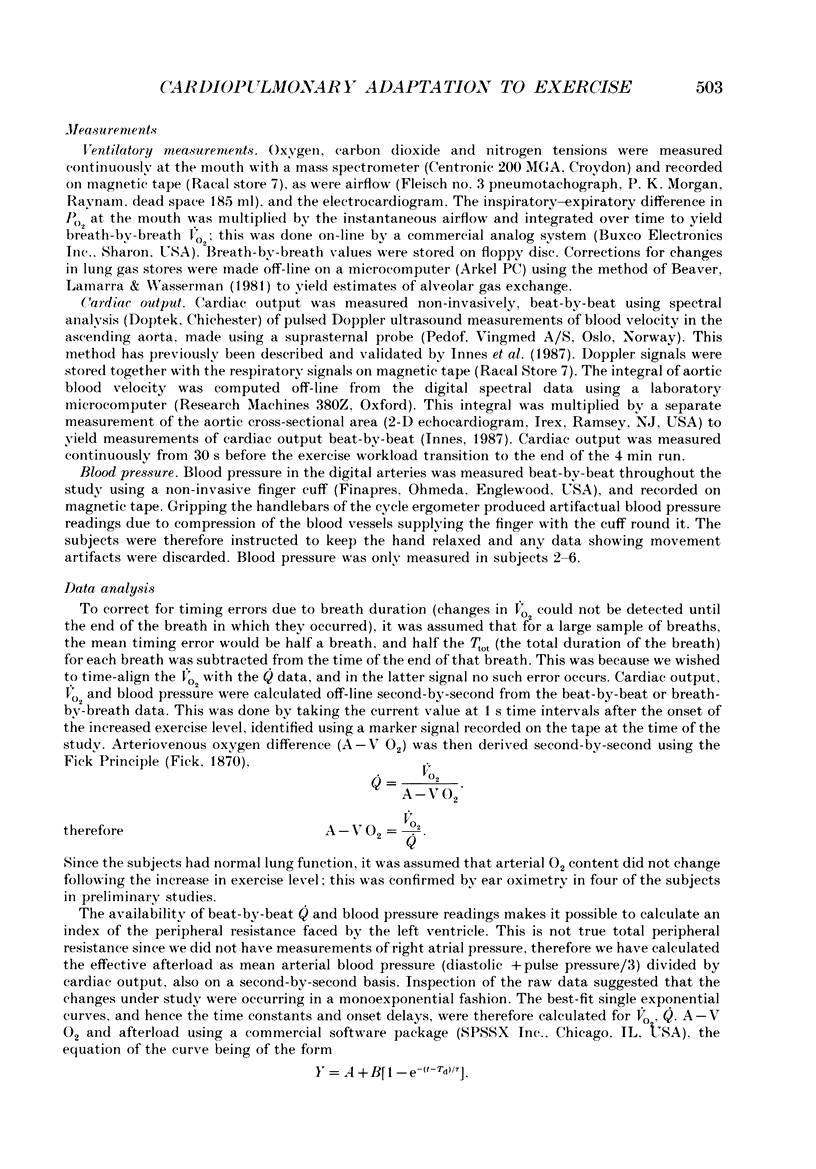
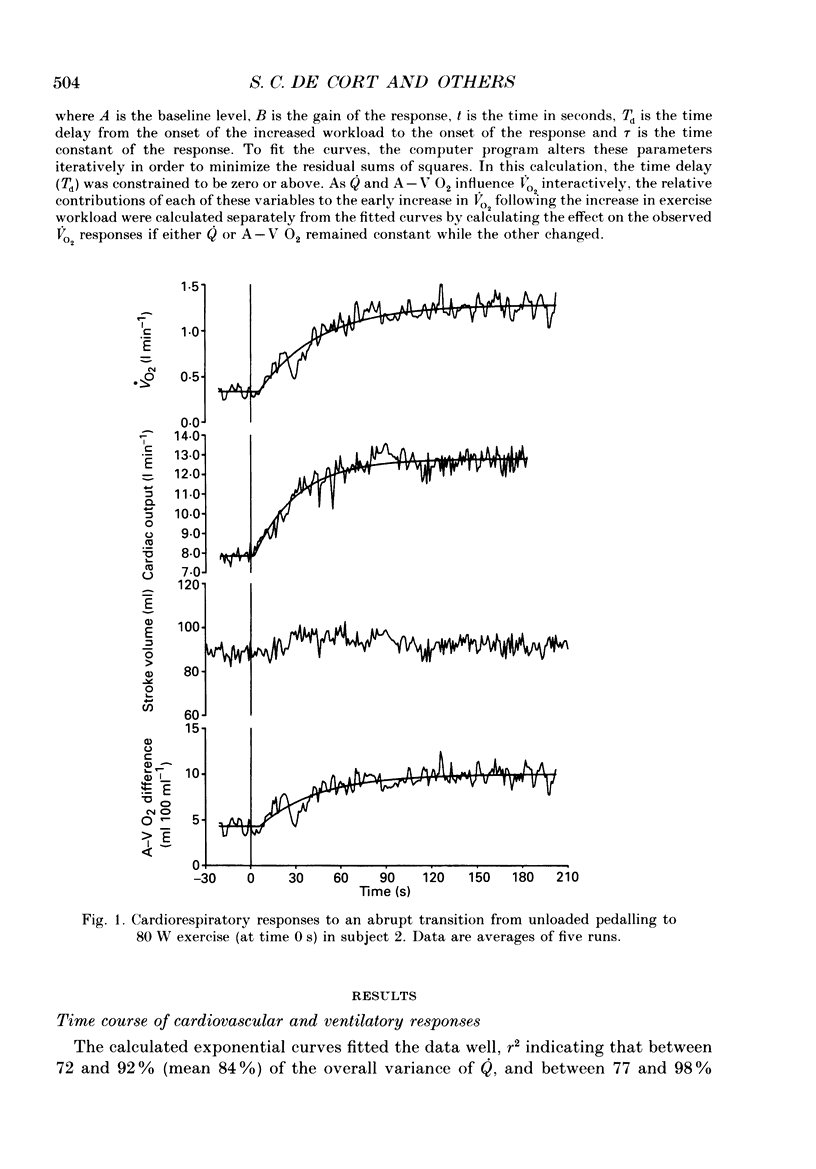
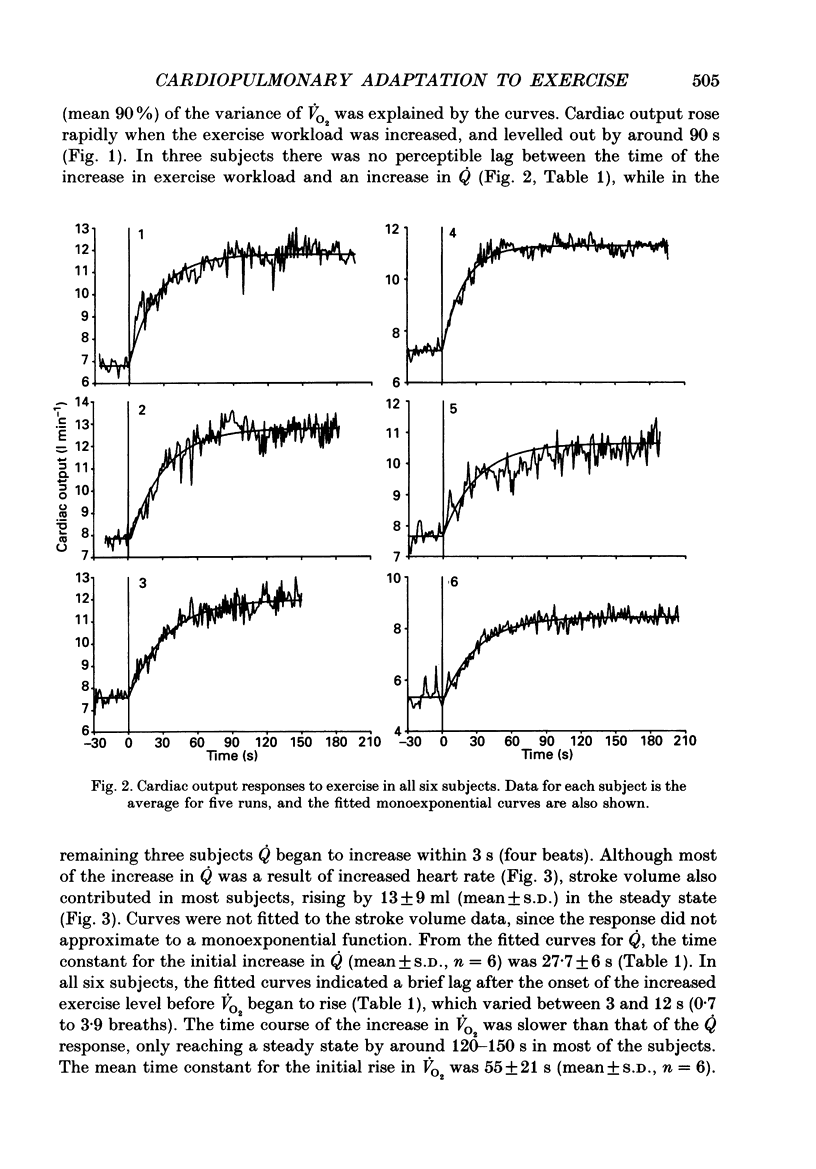
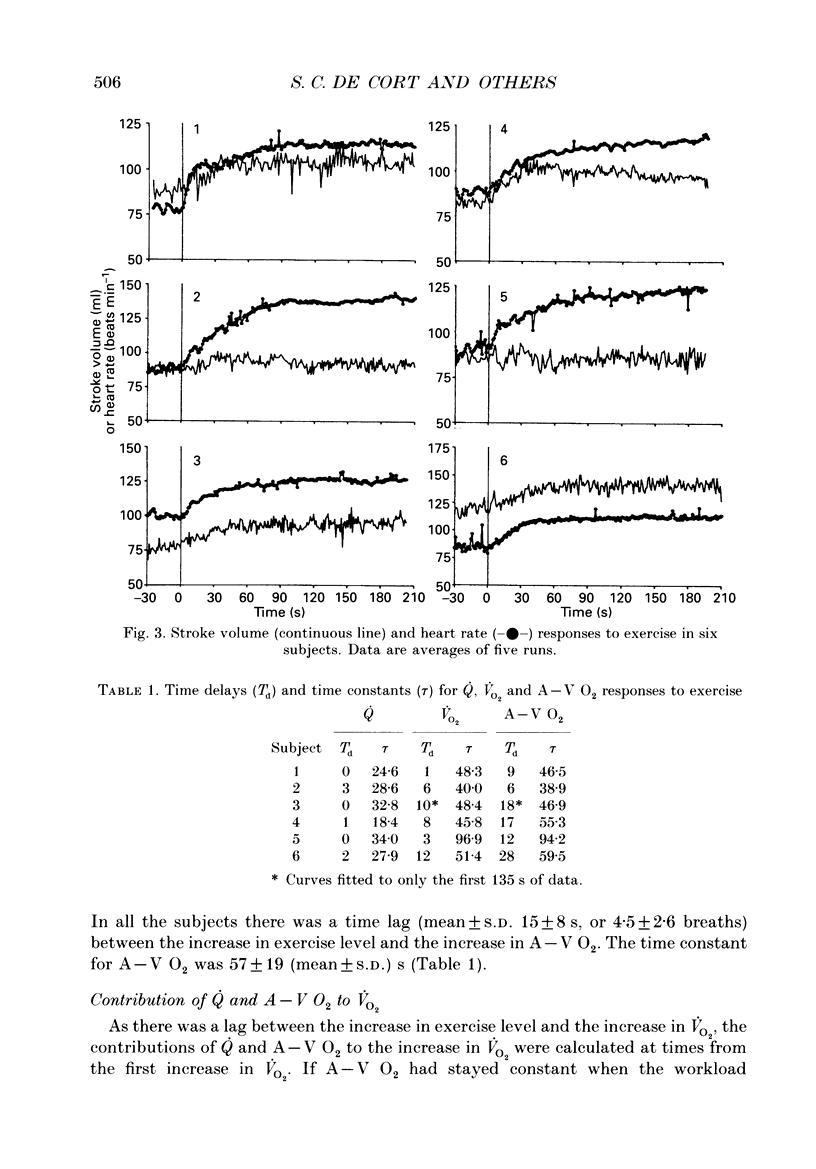
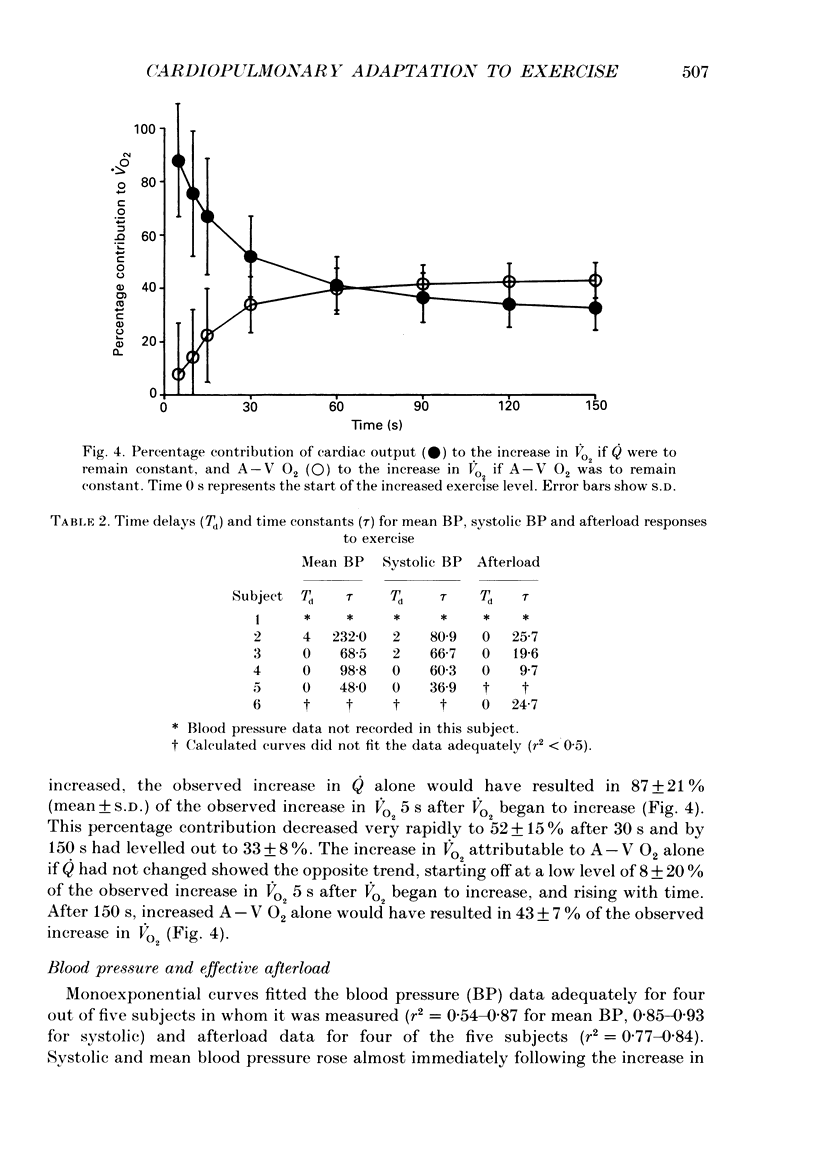
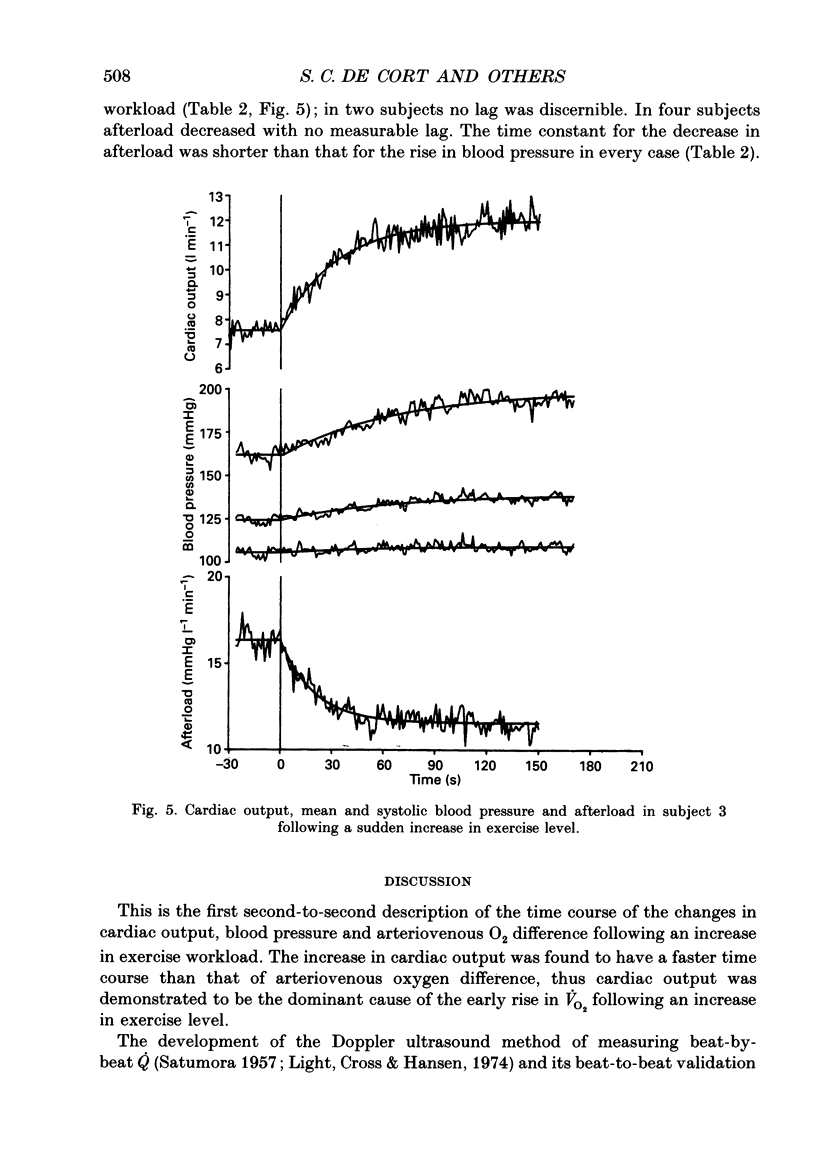
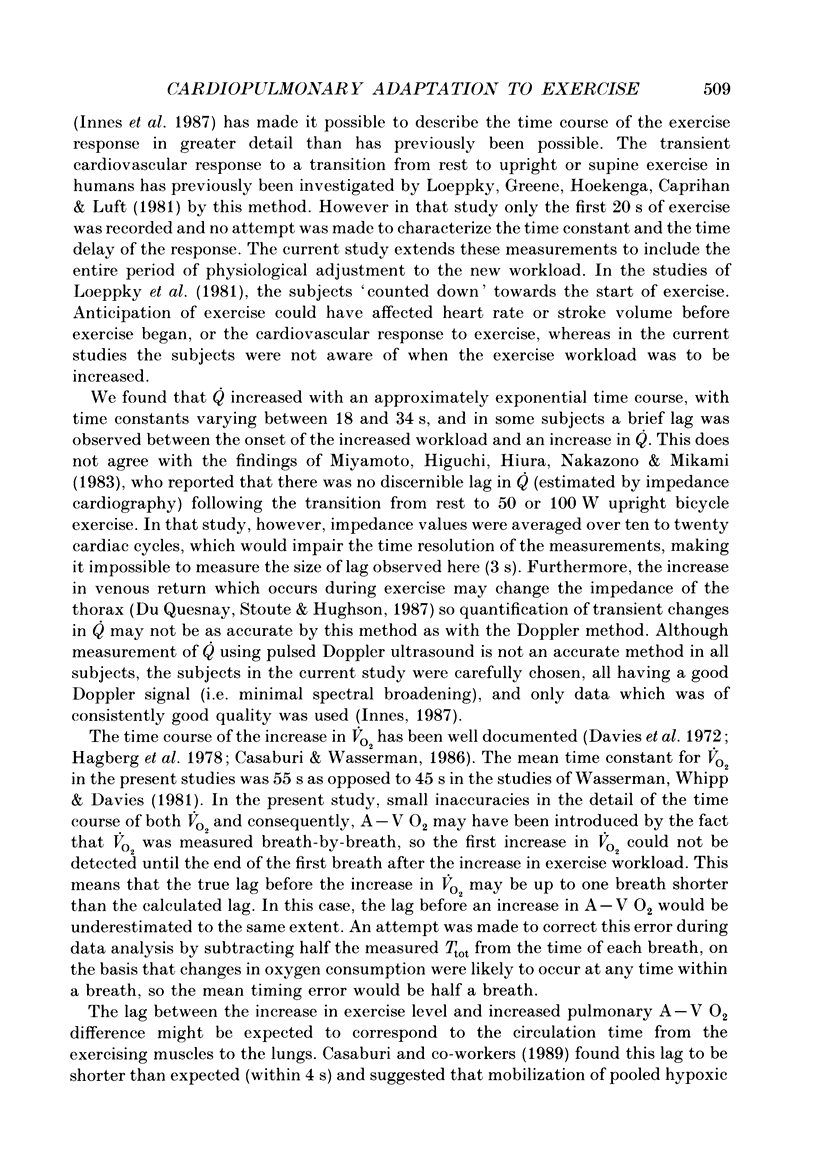
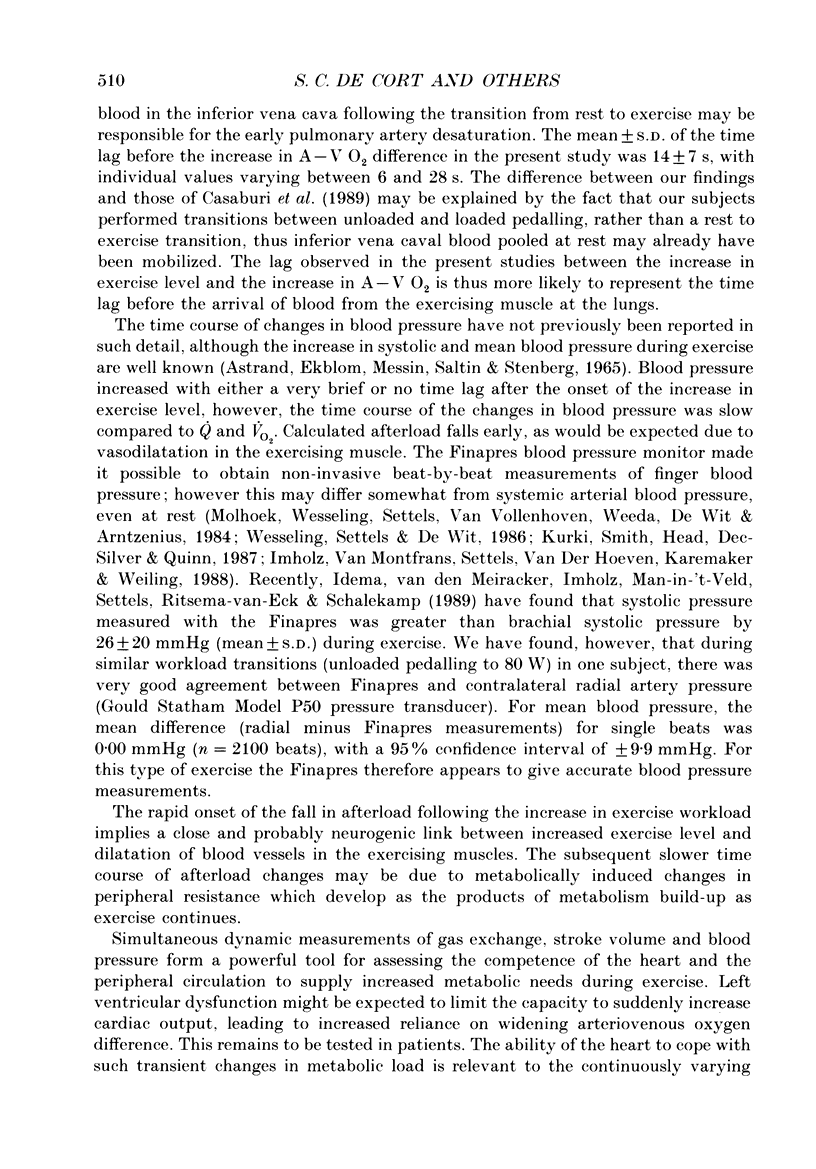
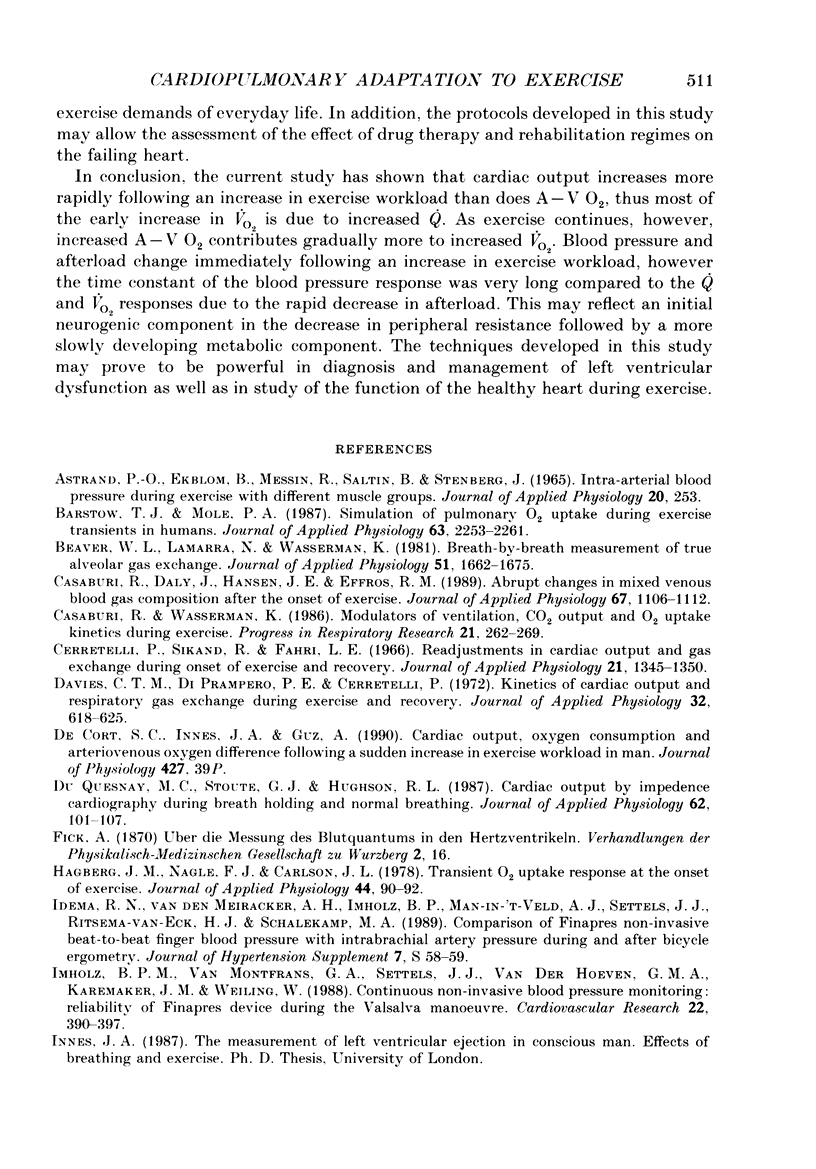
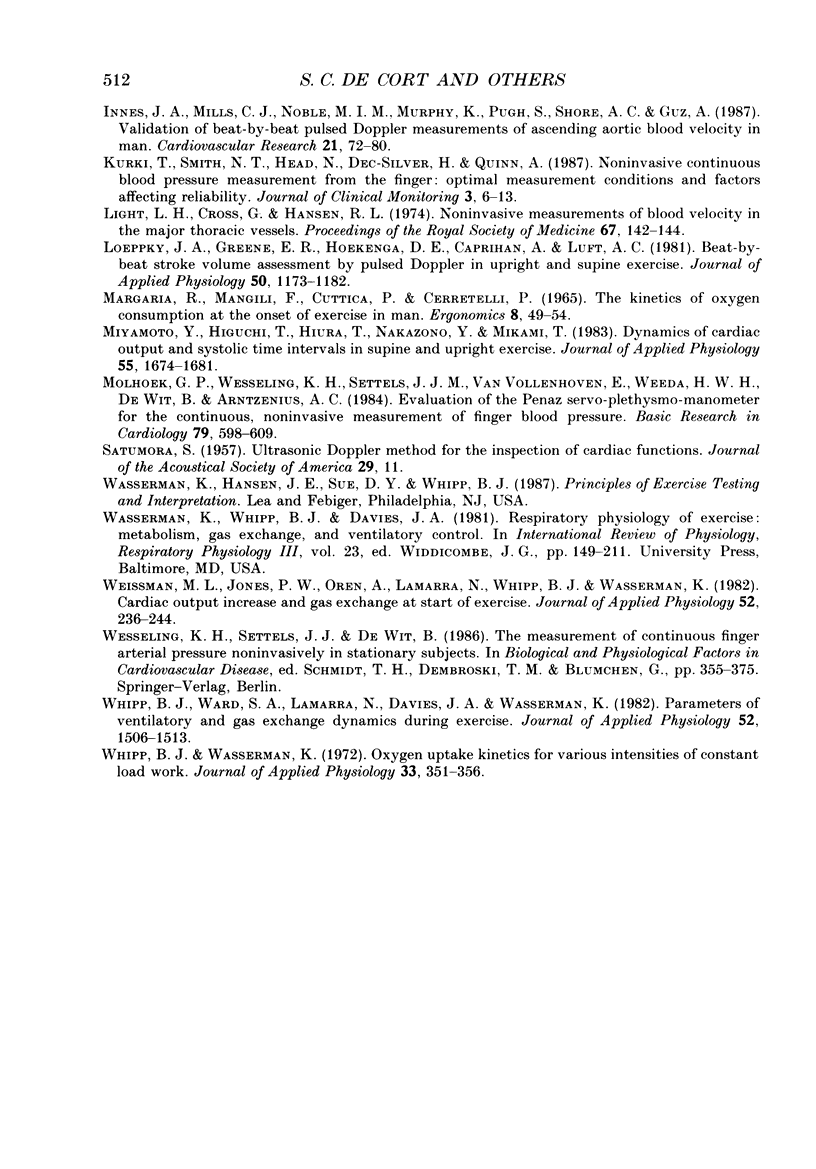
Selected References
These references are in PubMed. This may not be the complete list of references from this article.
- Barstow T. J., Molé P. A. Simulation of pulmonary O2 uptake during exercise transients in humans. J Appl Physiol (1985) 1987 Dec;63(6):2253–2261. doi: 10.1152/jappl.1987.63.6.2253. [DOI] [PubMed] [Google Scholar]
- Beaver W. L., Lamarra N., Wasserman K. Breath-by-breath measurement of true alveolar gas exchange. J Appl Physiol Respir Environ Exerc Physiol. 1981 Dec;51(6):1662–1675. doi: 10.1152/jappl.1981.51.6.1662. [DOI] [PubMed] [Google Scholar]
- Casaburi R., Daly J., Hansen J. E., Effros R. M. Abrupt changes in mixed venous blood gas composition after the onset of exercise. J Appl Physiol (1985) 1989 Sep;67(3):1106–1112. doi: 10.1152/jappl.1989.67.3.1106. [DOI] [PubMed] [Google Scholar]
- Cerretelli P., Sikand R., Farhi L. E. Readjustments in cardiac output and gas exchange during onset of exercise and recovery. J Appl Physiol. 1966 Jul;21(4):1345–1350. doi: 10.1152/jappl.1966.21.4.1345. [DOI] [PubMed] [Google Scholar]
- Davies C. T., Di Prampero P. E., Cerretelli P. Kinetics of cardiac output and respiratory gas exchange during exercise and recovery. J Appl Physiol. 1972 May;32(5):618–625. doi: 10.1152/jappl.1972.32.5.618. [DOI] [PubMed] [Google Scholar]
- Du Quesnay M. C., Stoute G. J., Hughson R. L. Cardiac output in exercise by impedance cardiography during breath holding and normal breathing. J Appl Physiol (1985) 1987 Jan;62(1):101–107. doi: 10.1152/jappl.1987.62.1.101. [DOI] [PubMed] [Google Scholar]
- Hagberg J. M., Nagle F. J., Carlson J. L. Transient O2 uptake response at the onset of exercise. J Appl Physiol Respir Environ Exerc Physiol. 1978 Jan;44(1):90–92. doi: 10.1152/jappl.1978.44.1.90. [DOI] [PubMed] [Google Scholar]
- Imholz B. P., van Montfrans G. A., Settels J. J., van der Hoeven G. M., Karemaker J. M., Wieling W. Continuous non-invasive blood pressure monitoring: reliability of Finapres device during the Valsalva manoeuvre. Cardiovasc Res. 1988 Jun;22(6):390–397. doi: 10.1093/cvr/22.6.390. [DOI] [PubMed] [Google Scholar]
- Innes J. A., Mills C. J., Noble M. I., Murphy K., Pugh S., Shore A. C., Guz A. Validation of beat by beat pulsed Doppler measurements of ascending aortic blood velocity in man. Cardiovasc Res. 1987 Jan;21(1):72–80. doi: 10.1093/cvr/21.1.72. [DOI] [PubMed] [Google Scholar]
- Kurki T., Smith N. T., Head N., Dec-Silver H., Quinn A. Noninvasive continuous blood pressure measurement from the finger: optimal measurement conditions and factors affecting reliability. J Clin Monit. 1987 Jan;3(1):6–13. doi: 10.1007/BF00770876. [DOI] [PubMed] [Google Scholar]
- Light L. H., Cross G., Hansen P. L. Non-invasive measurement of blood velocity in the major thoracic vessels. Proc R Soc Med. 1974 Feb;67(2):142–144. [PMC free article] [PubMed] [Google Scholar]
- Loeppky J. A., Greene E. R., Hoekenga D. E., Caprihan A., Luft U. C. Beat-by-beat stroke volume assessment by pulsed Doppler in upright and supine exercise. J Appl Physiol Respir Environ Exerc Physiol. 1981 Jun;50(6):1173–1182. doi: 10.1152/jappl.1981.50.6.1173. [DOI] [PubMed] [Google Scholar]
- Miyamoto Y., Higuchi J., Abe Y., Hiura T., Nakazono Y., Mikami T. Dynamics of cardiac output and systolic time intervals in supine and upright exercise. J Appl Physiol Respir Environ Exerc Physiol. 1983 Dec;55(6):1674–1681. doi: 10.1152/jappl.1983.55.6.1674. [DOI] [PubMed] [Google Scholar]
- Molhoek G. P., Wesseling K. H., Settels J. J., van Vollenhoven E., Weeda H. W., de Wit B., Arntzenius A. C. Evaluation of the Penàz servo-plethysmo-manometer for the continuous, non-invasive measurement of finger blood pressure. Basic Res Cardiol. 1984 Sep-Oct;79(5):598–609. doi: 10.1007/BF01910489. [DOI] [PubMed] [Google Scholar]
- Wasserman K., Whipp B. J., Davis J. A. Respiratory physiology of exercise: metabolism, gas exchange, and ventilatory control. Int Rev Physiol. 1981;23:149–211. [PubMed] [Google Scholar]
- Weissman M. L., Jones P. W., Oren A., Lamarra N., Whipp B. J., Wasserman K. Cardiac output increase and gas exchange at start of exercise. J Appl Physiol Respir Environ Exerc Physiol. 1982 Jan;52(1):236–244. doi: 10.1152/jappl.1982.52.1.236. [DOI] [PubMed] [Google Scholar]
- Whipp B. J., Ward S. A., Lamarra N., Davis J. A., Wasserman K. Parameters of ventilatory and gas exchange dynamics during exercise. J Appl Physiol Respir Environ Exerc Physiol. 1982 Jun;52(6):1506–1513. doi: 10.1152/jappl.1982.52.6.1506. [DOI] [PubMed] [Google Scholar]
- Whipp B. J., Wasserman K. Oxygen uptake kinetics for various intensities of constant-load work. J Appl Physiol. 1972 Sep;33(3):351–356. doi: 10.1152/jappl.1972.33.3.351. [DOI] [PubMed] [Google Scholar]