Abstract
1. When chemically skinned muscle fibres are activated by Ca2+ at an ionic strength of 170 mM, the spacing between the filaments has been shown to decrease with increasing force, suggesting that the cross-bridges can generate force not only in the axial but also in the radial direction. In the present study, radial force and radial stiffness of activated single skinned rabbit psoas fibres were studied by X-ray diffraction. The responses of the lattice spacing to changes in osmotic pressure by application of dextran T500, which is equivalent to force applied in the radial direction, was examined. The radial force generated by the attached cross-bridges was calculated, with the approximation that a negligible fraction of cross-bridges was attached in the relaxed muscle at the same ionic strength of 170 mM. 2. The active radial force was found to be a slightly non-linear function of lattice spacing, reaching zero at 34 nm. The radial force was compressive at lattice spacing greater than 34 nm and expansive at less than 34 nm. 3. The active axial force, on the other hand, was found to be much less affected by the application of dextran T500. Active axial force increased by 4% to a plateau at 4% dextran T500 and then decreased by 10% at 8% dextran T500. 4. While not under osmotic pressure, the radial force of the activated fibre was determined to be 400 pN (single thick filament)-1. This is of the same order of magnitude as the axial force. The radial stiffness was also comparable to the axial stiffness at 7 pN (thick filament)-1 (0.1 nm)-1. 5. The radial elasticity of the fully activated fibre differs significantly from that of the fibre in rigor. The radial stiffness exhibited by fibres in rigor was approximately five times higher, at 30 pN (thick filament)-1 (0.1 nm)-1 and the point where the radial force reached zero was 38 nm. 6. In the activated state, the point at which radial force reaches zero is independent of the level of Ca2+ activation, i.e. independent of the number of cross-bridges attached to actin in the force-generating state. We suggest that the zero-force point is equivalent to the equilibrium point of a spring and is an intrinsic property of the radial elasticity of the cross-bridge. 7. It is concluded that activated and rigor cross-bridges exhibit a spring-like property in the radial direction.(ABSTRACT TRUNCATED AT 400 WORDS)
Full text
PDF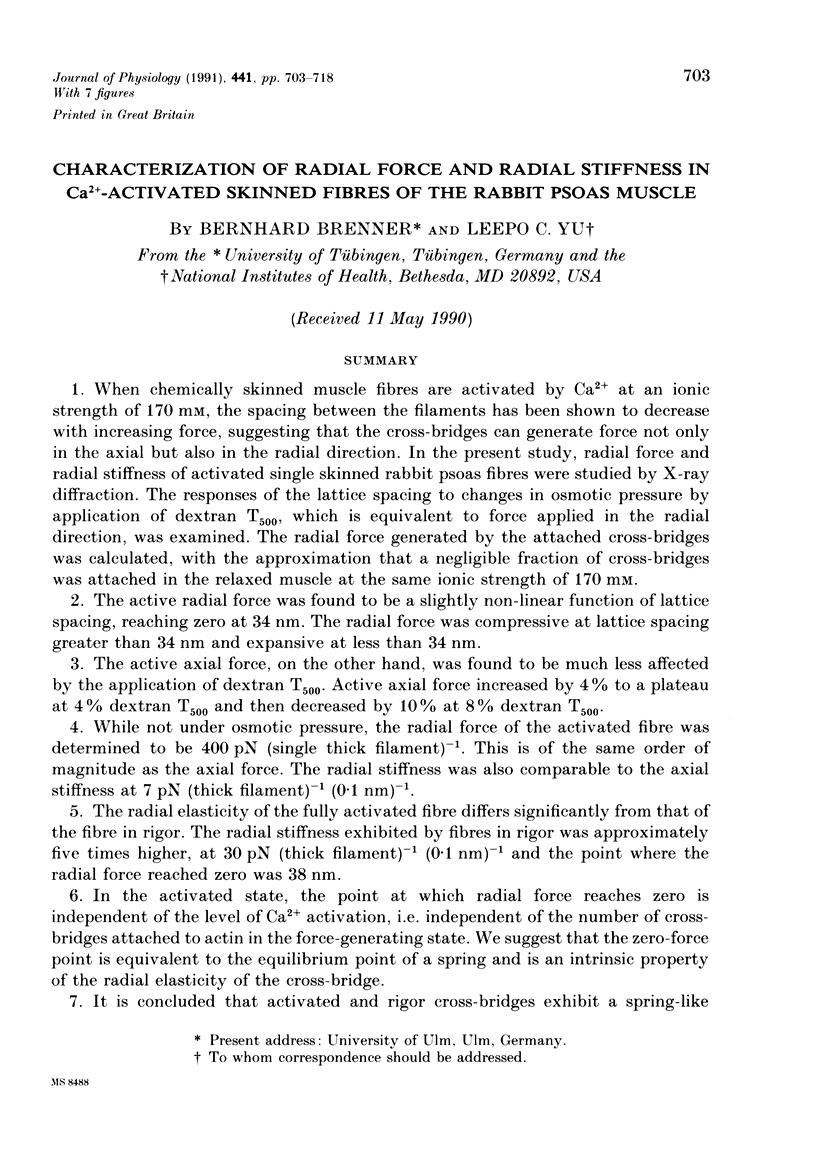
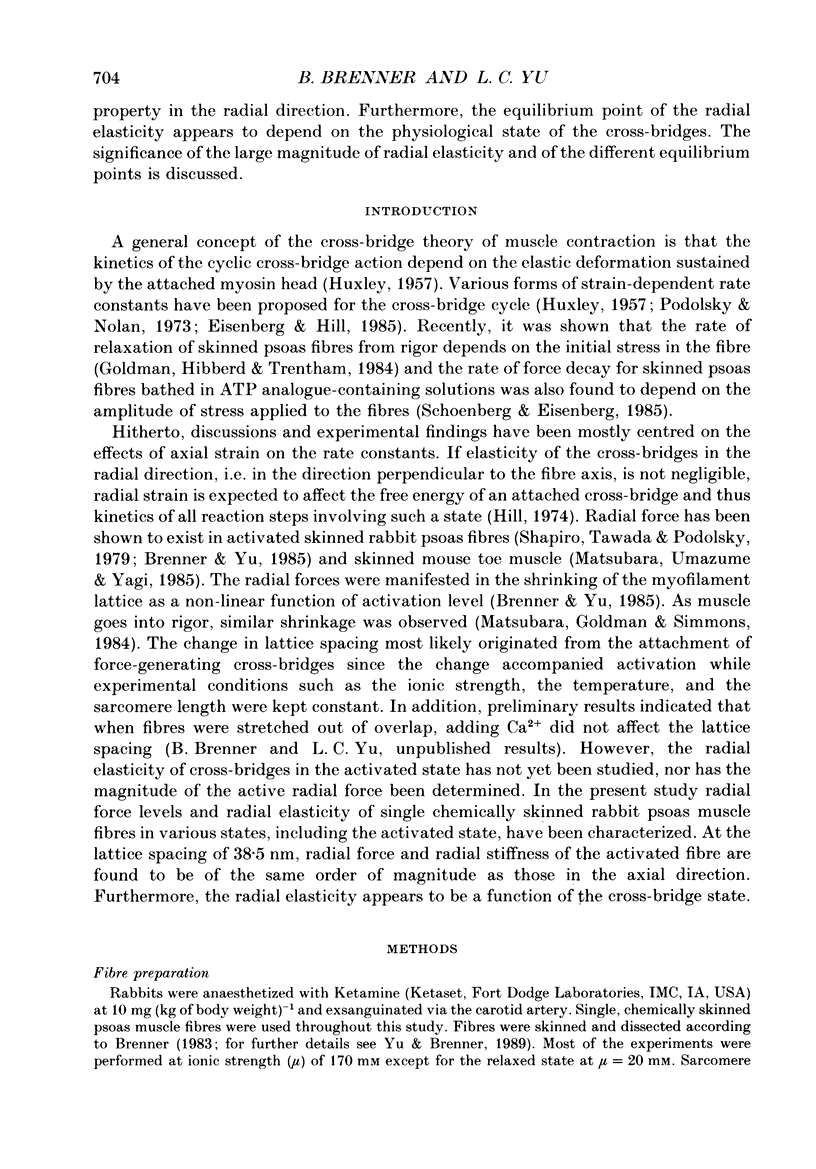
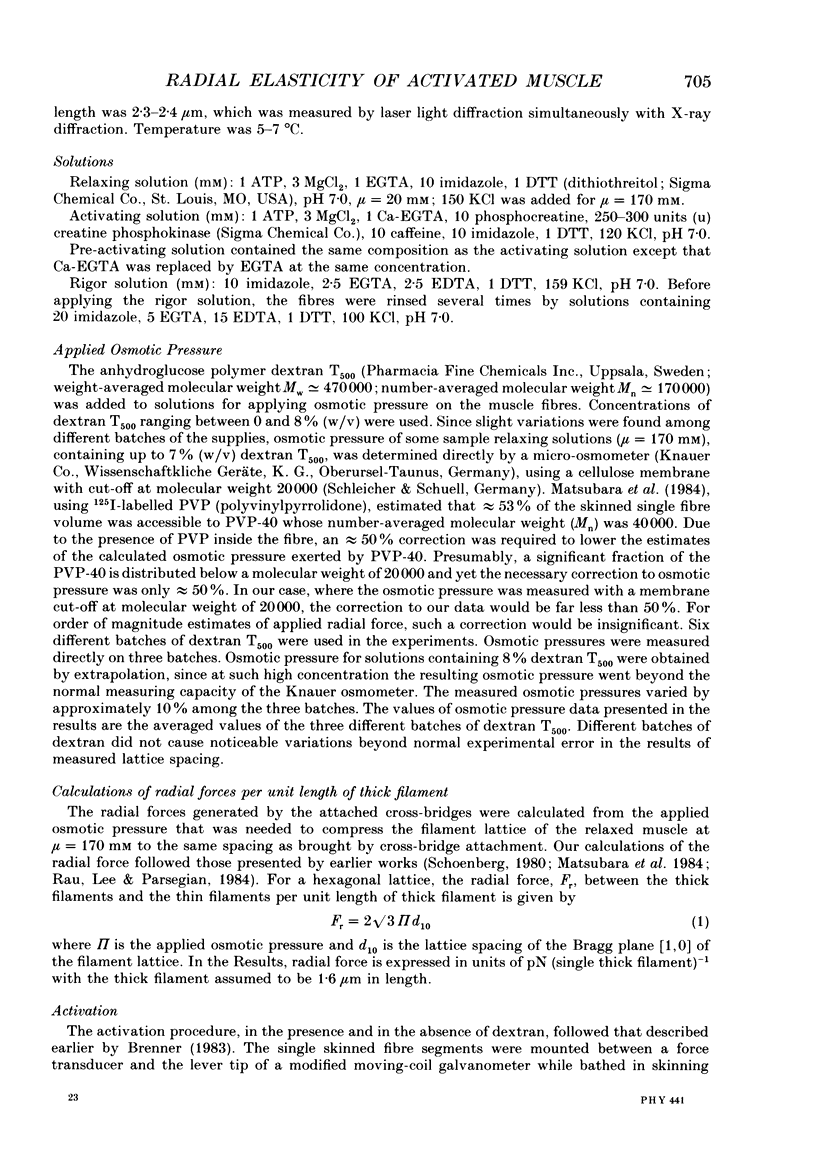
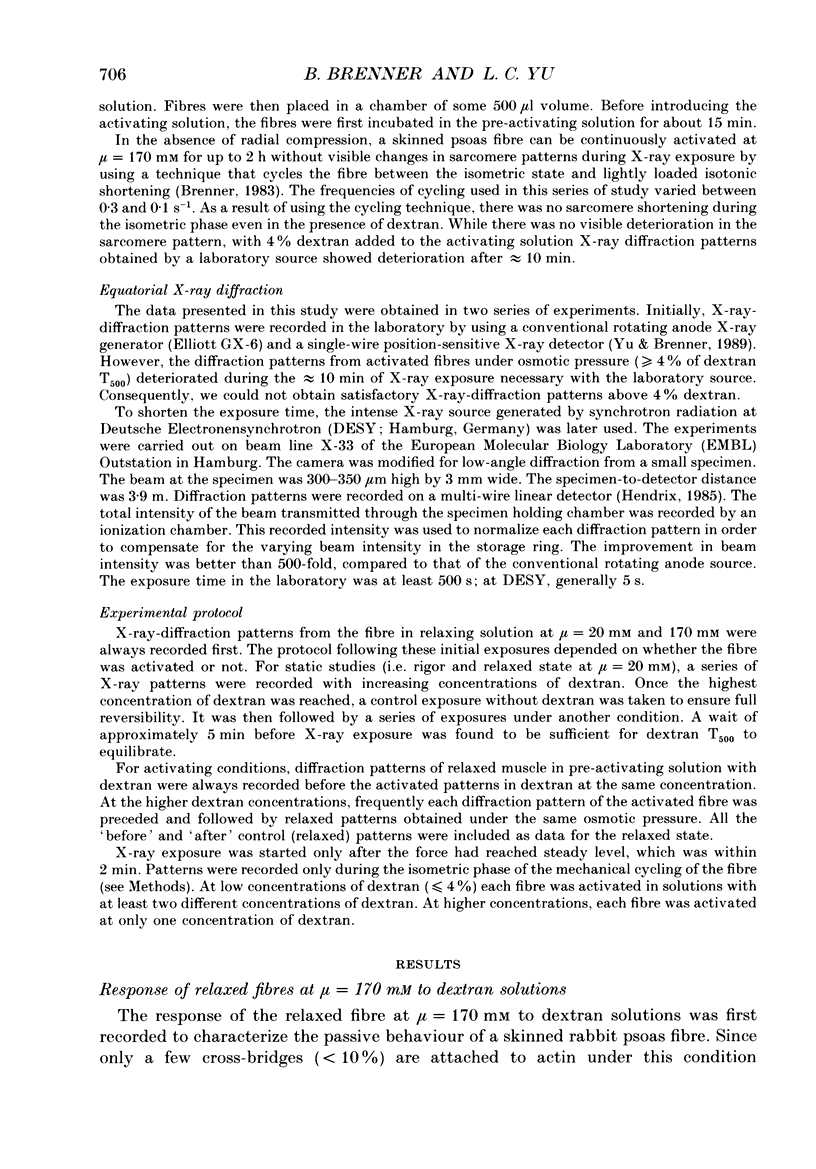
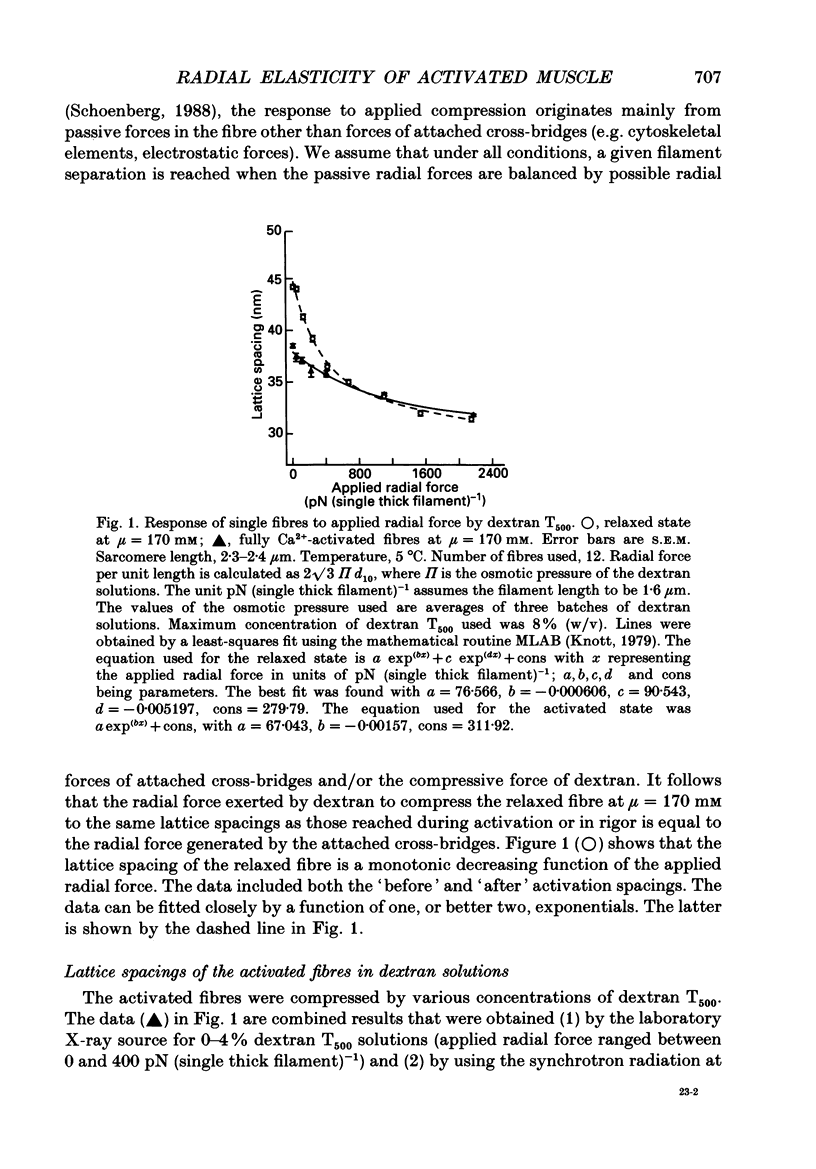
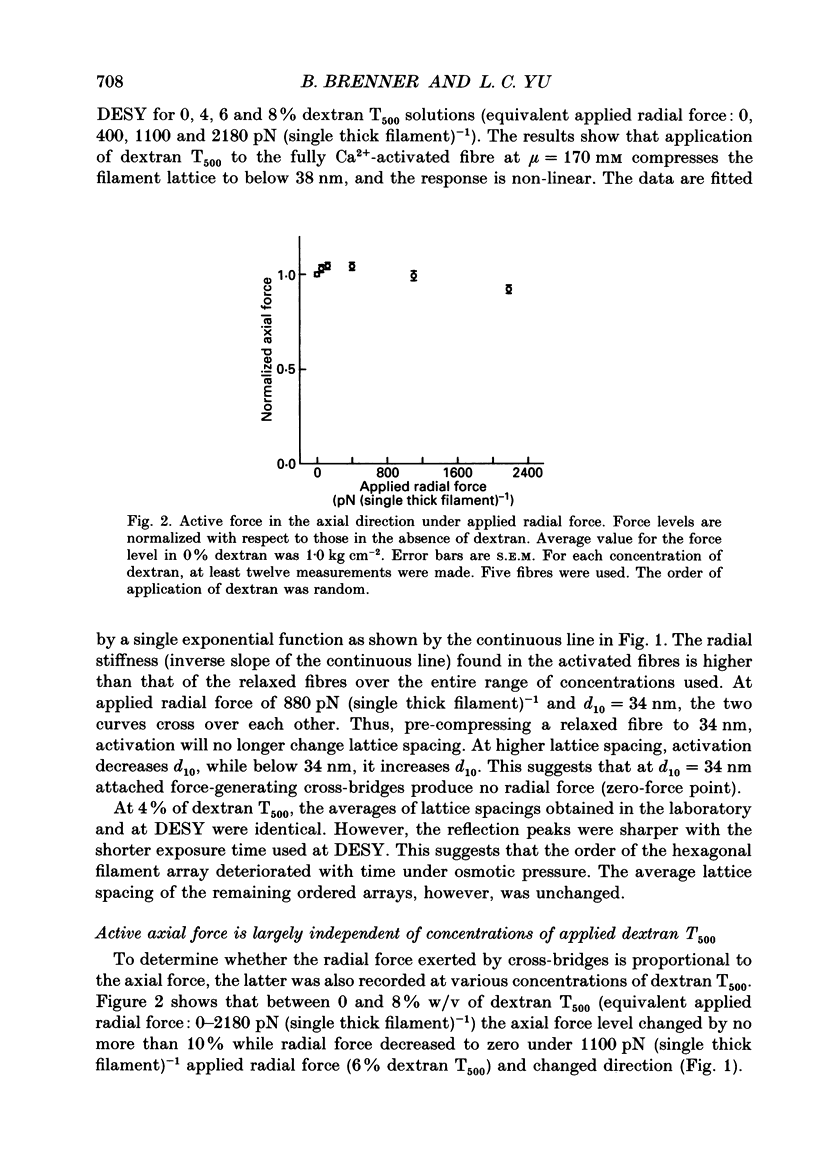
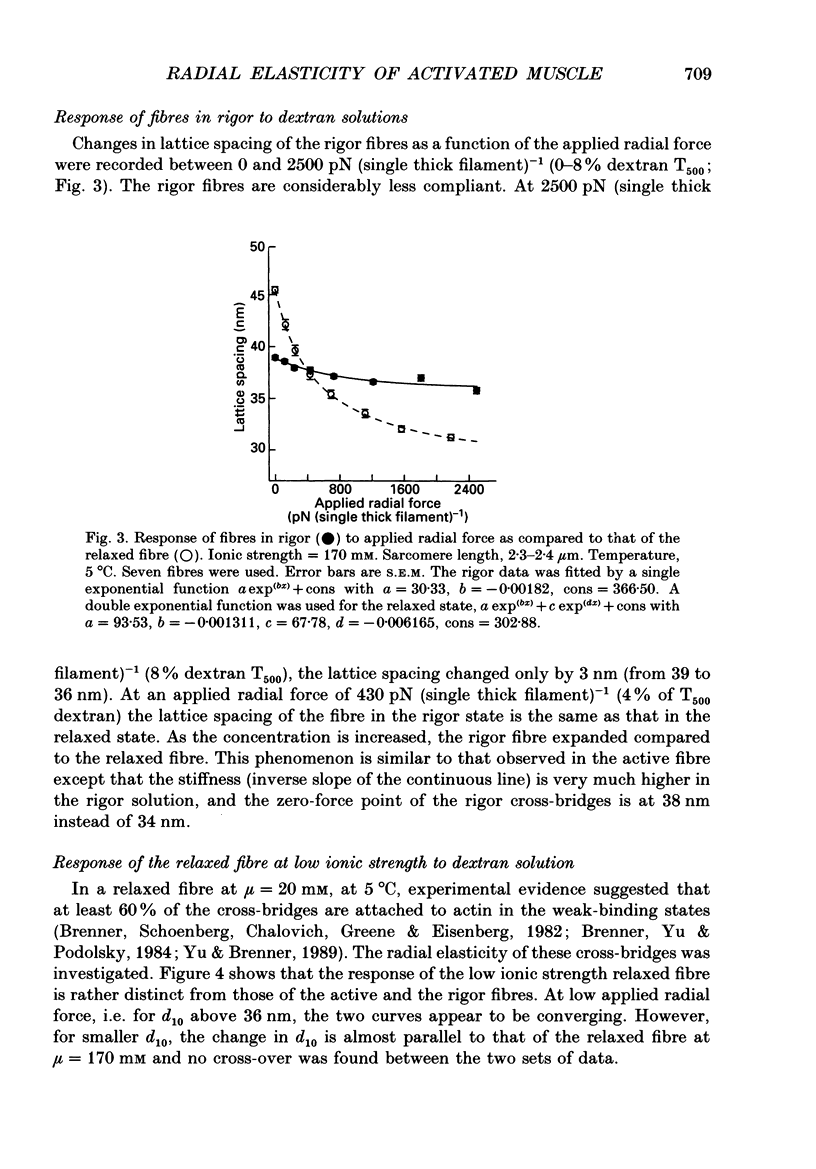
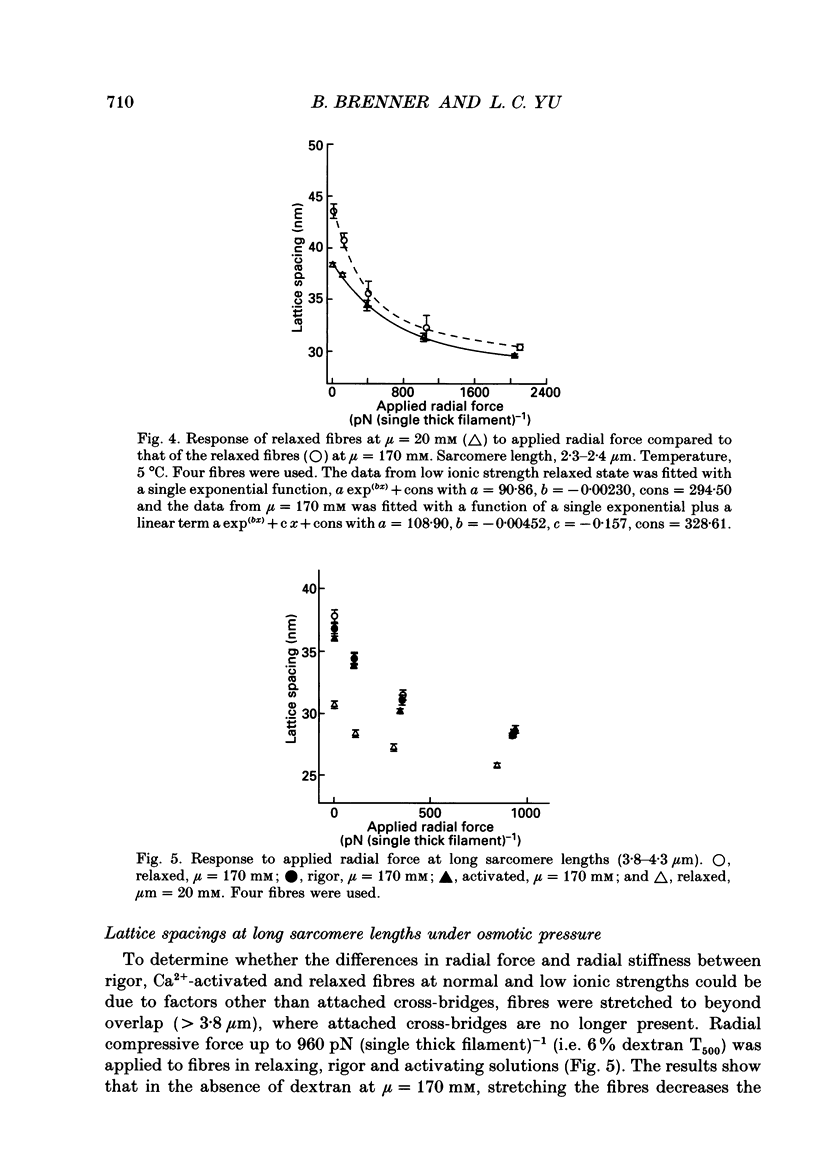
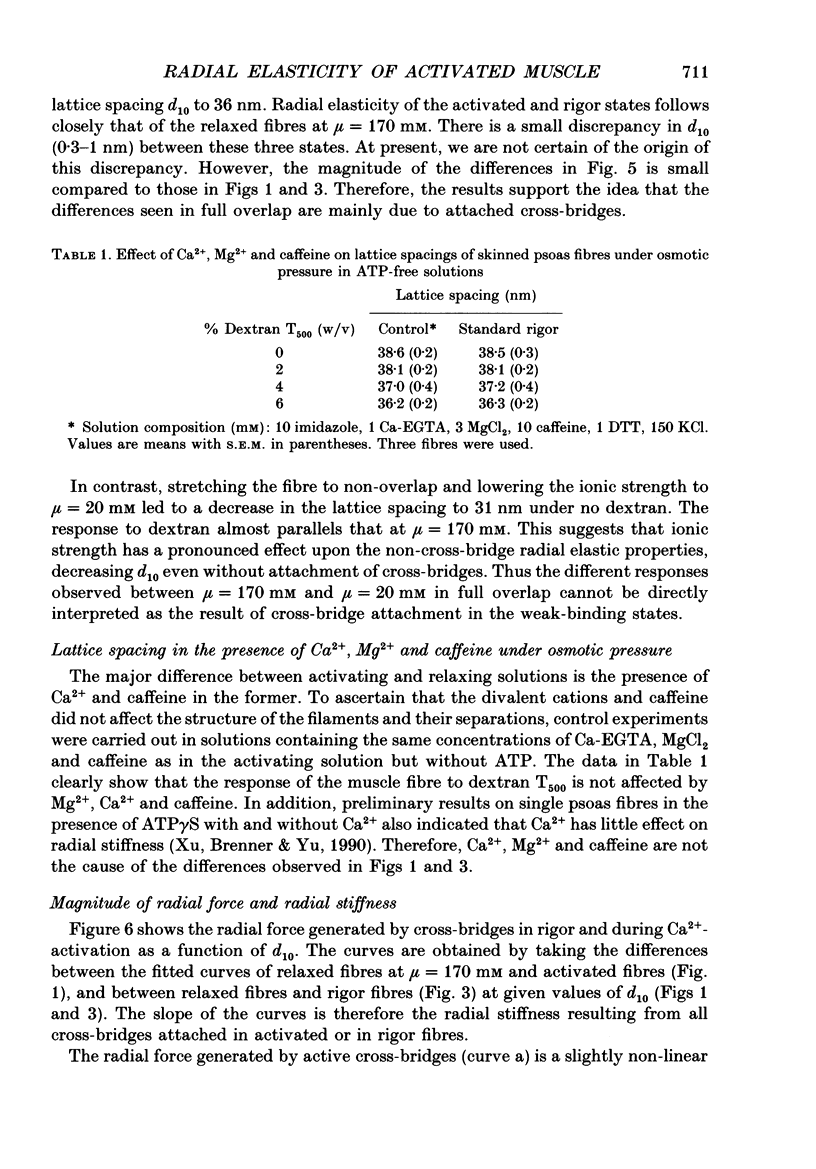
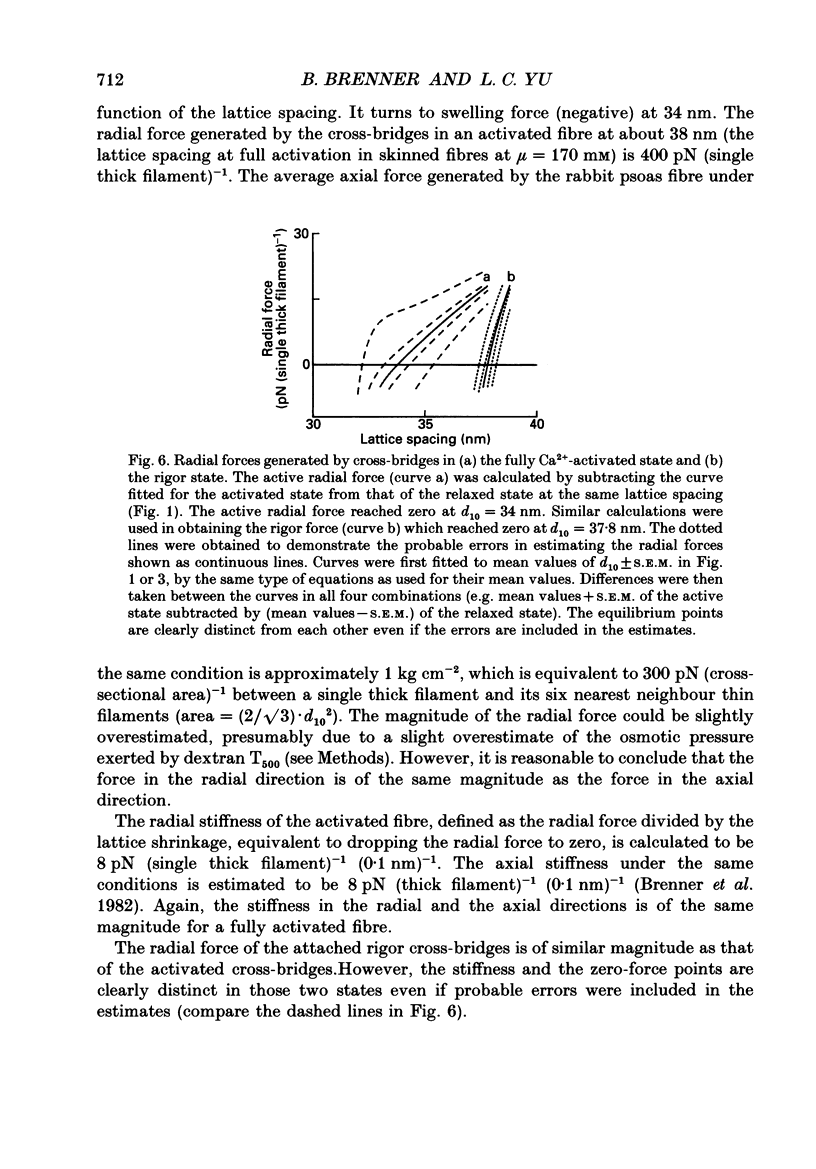
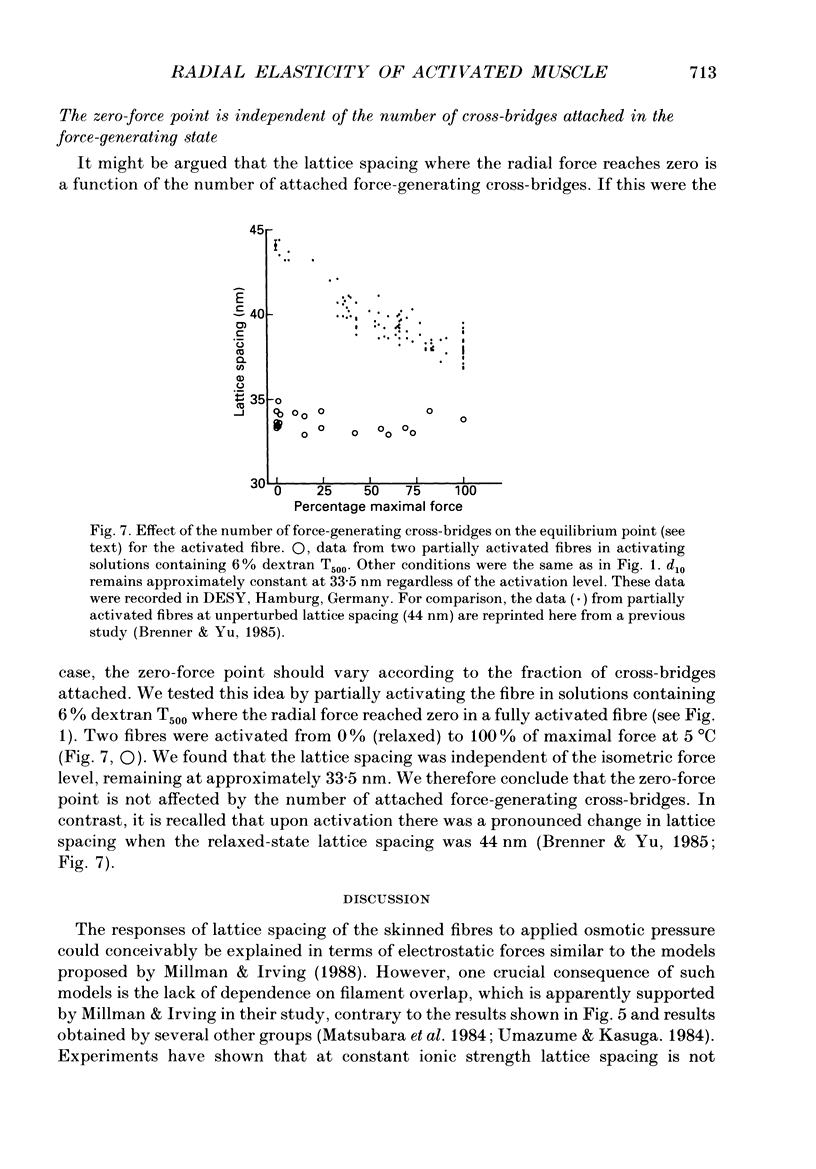
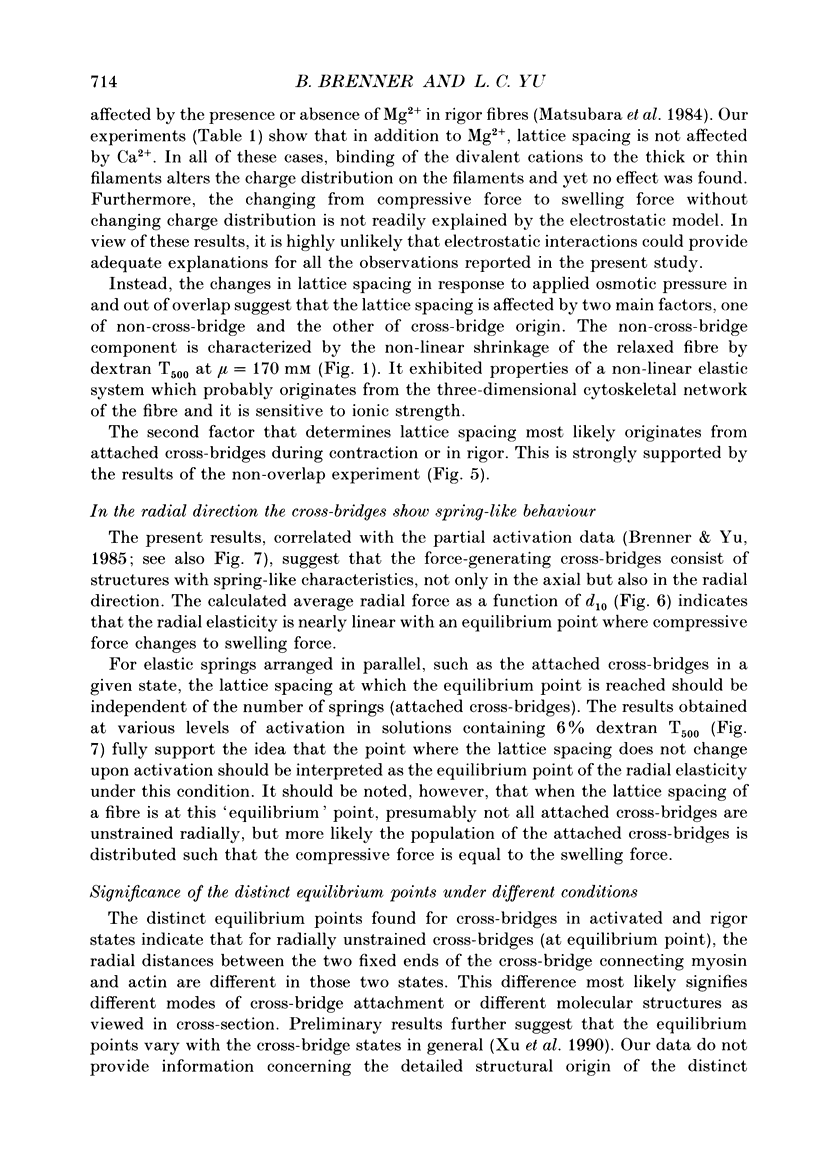
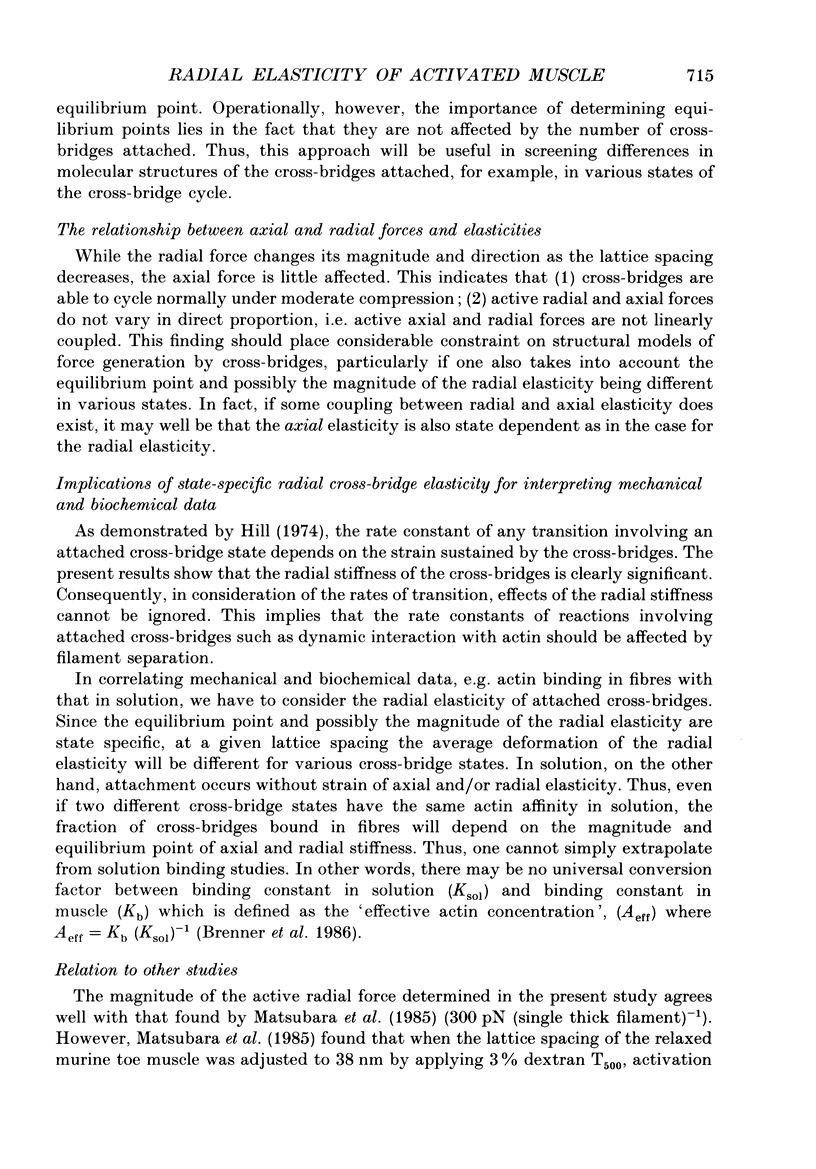
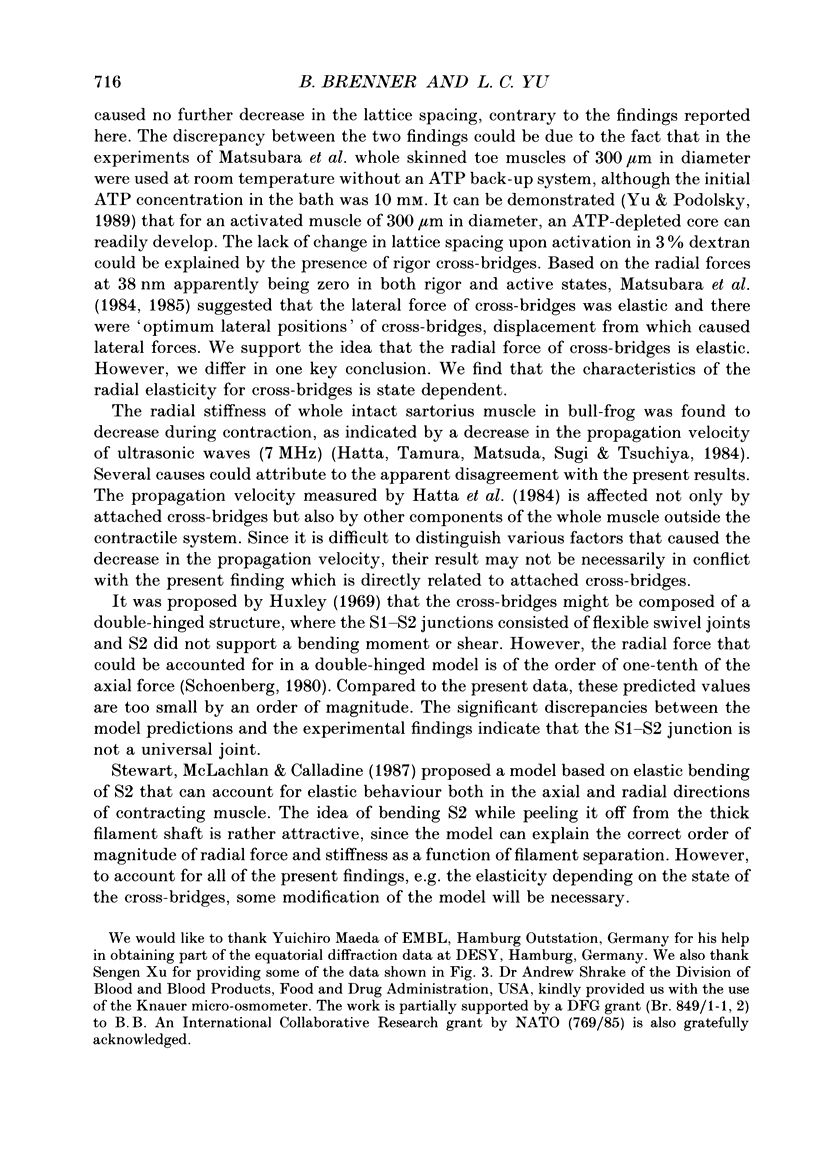
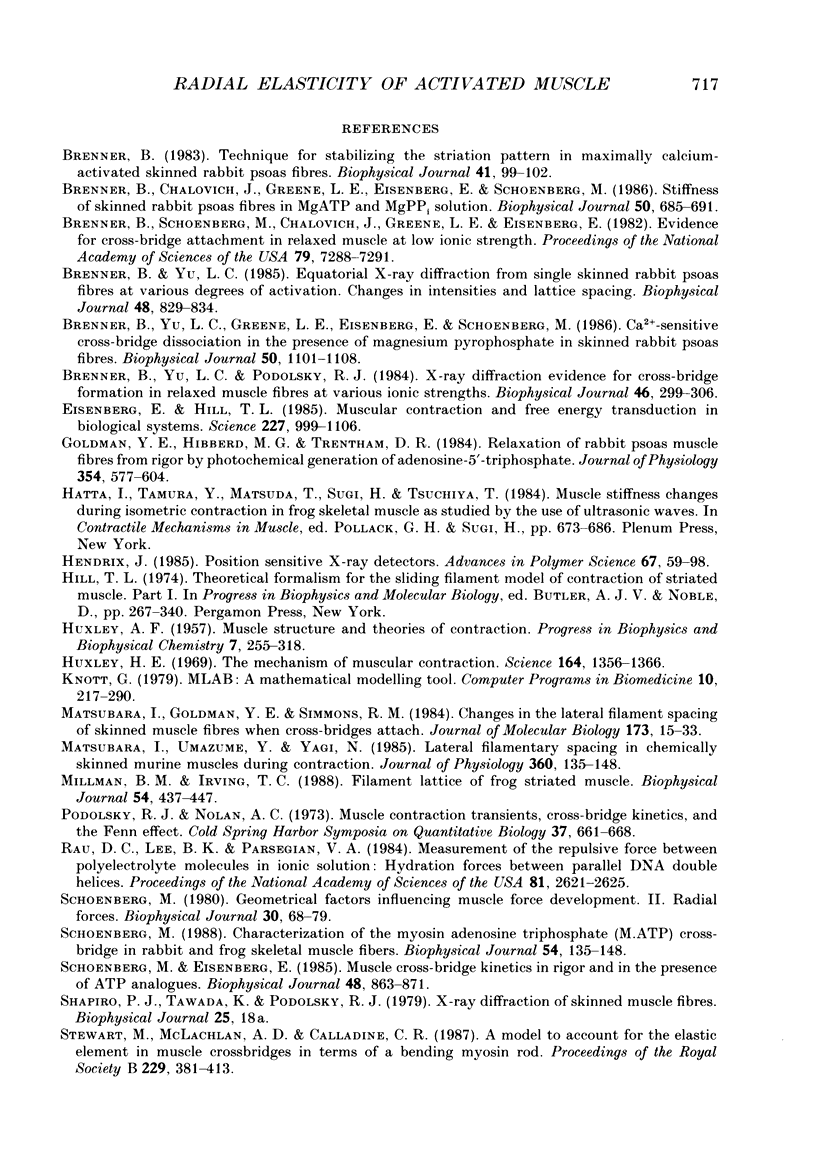
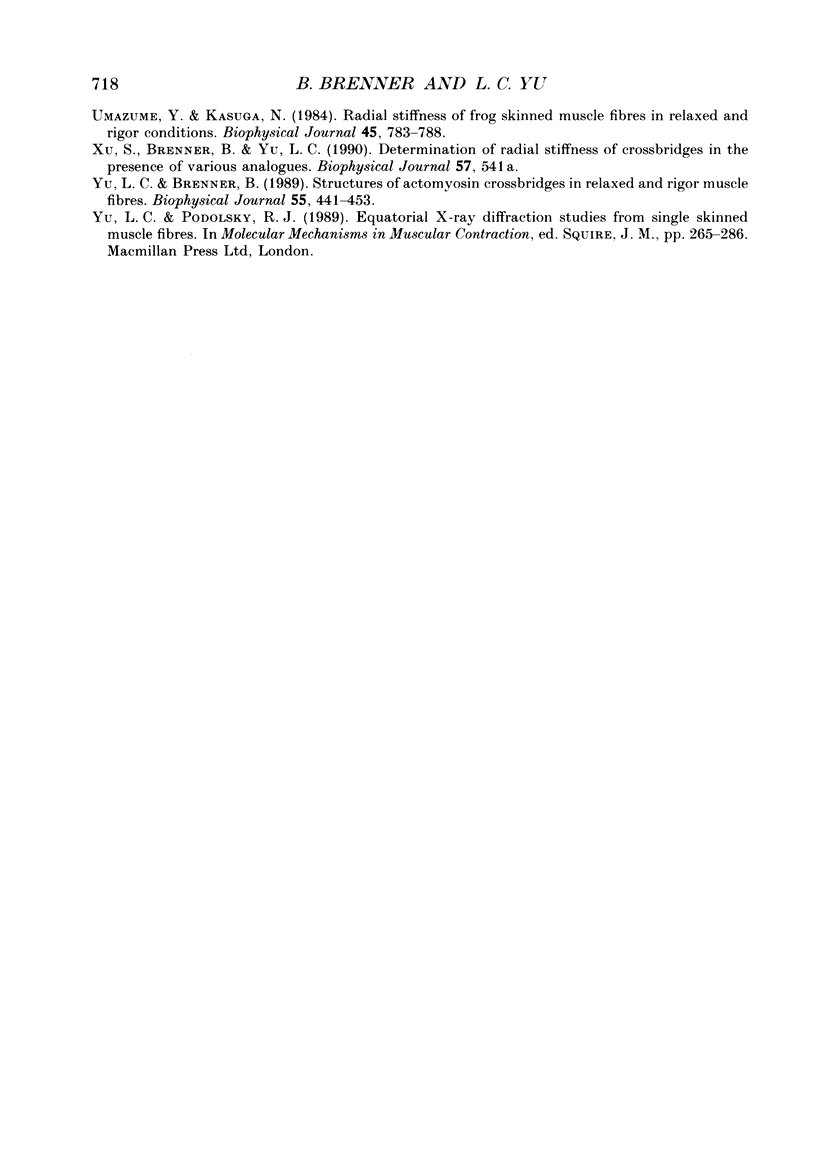
Selected References
These references are in PubMed. This may not be the complete list of references from this article.
- Brenner B., Chalovich J. M., Greene L. E., Eisenberg E., Schoenberg M. Stiffness of skinned rabbit psoas fibers in MgATP and MgPPi solution. Biophys J. 1986 Oct;50(4):685–691. doi: 10.1016/S0006-3495(86)83509-3. [DOI] [PMC free article] [PubMed] [Google Scholar]
- Brenner B., Schoenberg M., Chalovich J. M., Greene L. E., Eisenberg E. Evidence for cross-bridge attachment in relaxed muscle at low ionic strength. Proc Natl Acad Sci U S A. 1982 Dec;79(23):7288–7291. doi: 10.1073/pnas.79.23.7288. [DOI] [PMC free article] [PubMed] [Google Scholar]
- Brenner B. Technique for stabilizing the striation pattern in maximally calcium-activated skinned rabbit psoas fibers. Biophys J. 1983 Jan;41(1):99–102. doi: 10.1016/S0006-3495(83)84411-7. [DOI] [PMC free article] [PubMed] [Google Scholar]
- Brenner B., Yu L. C. Equatorial x-ray diffraction from single skinned rabbit psoas fibers at various degrees of activation. Changes in intensities and lattice spacing. Biophys J. 1985 Nov;48(5):829–834. doi: 10.1016/S0006-3495(85)83841-8. [DOI] [PMC free article] [PubMed] [Google Scholar]
- Brenner B., Yu L. C., Greene L. E., Eisenberg E., Schoenberg M. Ca2+-sensitive cross-bridge dissociation in the presence of magnesium pyrophosphate in skinned rabbit psoas fibers. Biophys J. 1986 Dec;50(6):1101–1108. doi: 10.1016/S0006-3495(86)83554-8. [DOI] [PMC free article] [PubMed] [Google Scholar]
- Brenner B., Yu L. C., Podolsky R. J. X-ray diffraction evidence for cross-bridge formation in relaxed muscle fibers at various ionic strengths. Biophys J. 1984 Sep;46(3):299–306. doi: 10.1016/S0006-3495(84)84026-6. [DOI] [PMC free article] [PubMed] [Google Scholar]
- Eisenberg E., Hill T. L. Muscle contraction and free energy transduction in biological systems. Science. 1985 Mar 1;227(4690):999–1006. doi: 10.1126/science.3156404. [DOI] [PubMed] [Google Scholar]
- Goldman Y. E., Hibberd M. G., Trentham D. R. Relaxation of rabbit psoas muscle fibres from rigor by photochemical generation of adenosine-5'-triphosphate. J Physiol. 1984 Sep;354:577–604. doi: 10.1113/jphysiol.1984.sp015394. [DOI] [PMC free article] [PubMed] [Google Scholar]
- HUXLEY A. F. Muscle structure and theories of contraction. Prog Biophys Biophys Chem. 1957;7:255–318. [PubMed] [Google Scholar]
- Hatta I., Tamura Y., Matsuda T., Sugi H., Tsuchiya T. Muscle stiffness changes during isometric contraction in frog skeletal muscle as studied by the use of ultrasonic waves. Adv Exp Med Biol. 1984;170:673–686. doi: 10.1007/978-1-4684-4703-3_62. [DOI] [PubMed] [Google Scholar]
- Huxley H. E. The mechanism of muscular contraction. Science. 1969 Jun 20;164(3886):1356–1365. doi: 10.1126/science.164.3886.1356. [DOI] [PubMed] [Google Scholar]
- Matsubara I., Goldman Y. E., Simmons R. M. Changes in the lateral filament spacing of skinned muscle fibres when cross-bridges attach. J Mol Biol. 1984 Feb 15;173(1):15–33. doi: 10.1016/0022-2836(84)90401-7. [DOI] [PubMed] [Google Scholar]
- Matsubara I., Umazume Y., Yagi N. Lateral filamentary spacing in chemically skinned murine muscles during contraction. J Physiol. 1985 Mar;360:135–148. doi: 10.1113/jphysiol.1985.sp015608. [DOI] [PMC free article] [PubMed] [Google Scholar]
- Millman B. M., Irving T. C. Filament lattice of frog striated muscle. Radial forces, lattice stability, and filament compression in the A-band of relaxed and rigor muscle. Biophys J. 1988 Sep;54(3):437–447. doi: 10.1016/S0006-3495(88)82977-1. [DOI] [PMC free article] [PubMed] [Google Scholar]
- Rau D. C., Lee B., Parsegian V. A. Measurement of the repulsive force between polyelectrolyte molecules in ionic solution: hydration forces between parallel DNA double helices. Proc Natl Acad Sci U S A. 1984 May;81(9):2621–2625. doi: 10.1073/pnas.81.9.2621. [DOI] [PMC free article] [PubMed] [Google Scholar]
- Schoenberg M. Characterization of the myosin adenosine triphosphate (M.ATP) crossbridge in rabbit and frog skeletal muscle fibers. Biophys J. 1988 Jul;54(1):135–148. doi: 10.1016/S0006-3495(88)82938-2. [DOI] [PMC free article] [PubMed] [Google Scholar]
- Schoenberg M., Eisenberg E. Muscle cross-bridge kinetics in rigor and in the presence of ATP analogues. Biophys J. 1985 Dec;48(6):863–871. doi: 10.1016/S0006-3495(85)83847-9. [DOI] [PMC free article] [PubMed] [Google Scholar]
- Stewart M., McLachlan A. D., Calladine C. R. A model to account for the elastic element in muscle crossbridges in terms of a bending myosin rod. Proc R Soc Lond B Biol Sci. 1987 Jan 22;229(1257):381–413. doi: 10.1098/rspb.1987.0002. [DOI] [PubMed] [Google Scholar]
- Umazume Y., Kasuga N. Radial stiffness of frog skinned muscle fibers in relaxed and rigor conditions. Biophys J. 1984 Apr;45(4):783–788. doi: 10.1016/S0006-3495(84)84222-8. [DOI] [PMC free article] [PubMed] [Google Scholar]
- Yu L. C., Brenner B. Structures of actomyosin crossbridges in relaxed and rigor muscle fibers. Biophys J. 1989 Mar;55(3):441–453. doi: 10.1016/S0006-3495(89)82838-3. [DOI] [PMC free article] [PubMed] [Google Scholar]