Abstract
The P segments of the voltage-dependent Na+ channel line the outer mouth and selectivity filter of the pore. The residues that form the cytoplasmic mouth of the pore of the channel have not been identified. To study the structure of the inner pore mouth, the presumed selectivity filter residues (D400, E755, K1237, and A1529), and three amino acids just amino-terminal to each of these residues in the rat skeletal muscle Na+ channel, were mutated to cysteine and expressed in tsA 201 cells. These amino acids are predicted (by analogy to K+ channels) to be on the cytoplasmic side of the putative selectivity filter residues. Inward and outward Na+ currents were measured with the whole-cell configuration of the patch-clamp technique. Cysteinyl side-chain accessibility was gauged by sensitivity to Cd2+ block and by reactivity with methanethiosulfonate (MTS) reagents applied to both the inside and the outside of the cell. Outward currents through the wild-type and all of the mutant channels were unaffected by internal Cd2+ (100 microM). Similarly, 1 mM methanethiosulfonate ethylammonium (MTSEA) applied to the inside of the membrane did not affect wild-type or mutant outward currents. However, two mutants amino-terminal to the selectivity position in domain III (F1236C and T1235C) and one in domain IV (S1528C) were blocked with high affinity by external Cd2+. The Na+ current through F1236C and S1528C channels was inhibited by MTSEA applied to the outside of the cell. The accessibility of these mutants to externally applied cysteinyl ligands indicates that the side chains of the mutated residues face outward rather than inward. The K+ channel model of the P segments as protein loops that span the selectivity region is not applicable to the Na+ channel.
Full text
PDF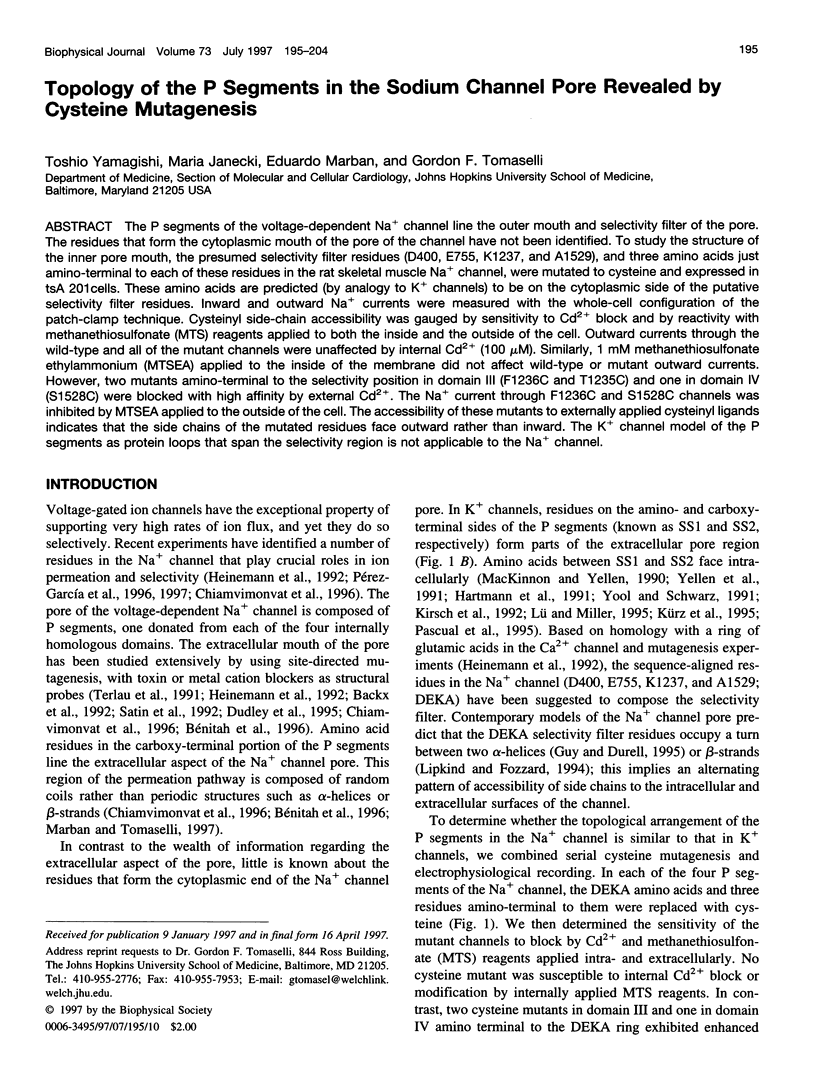
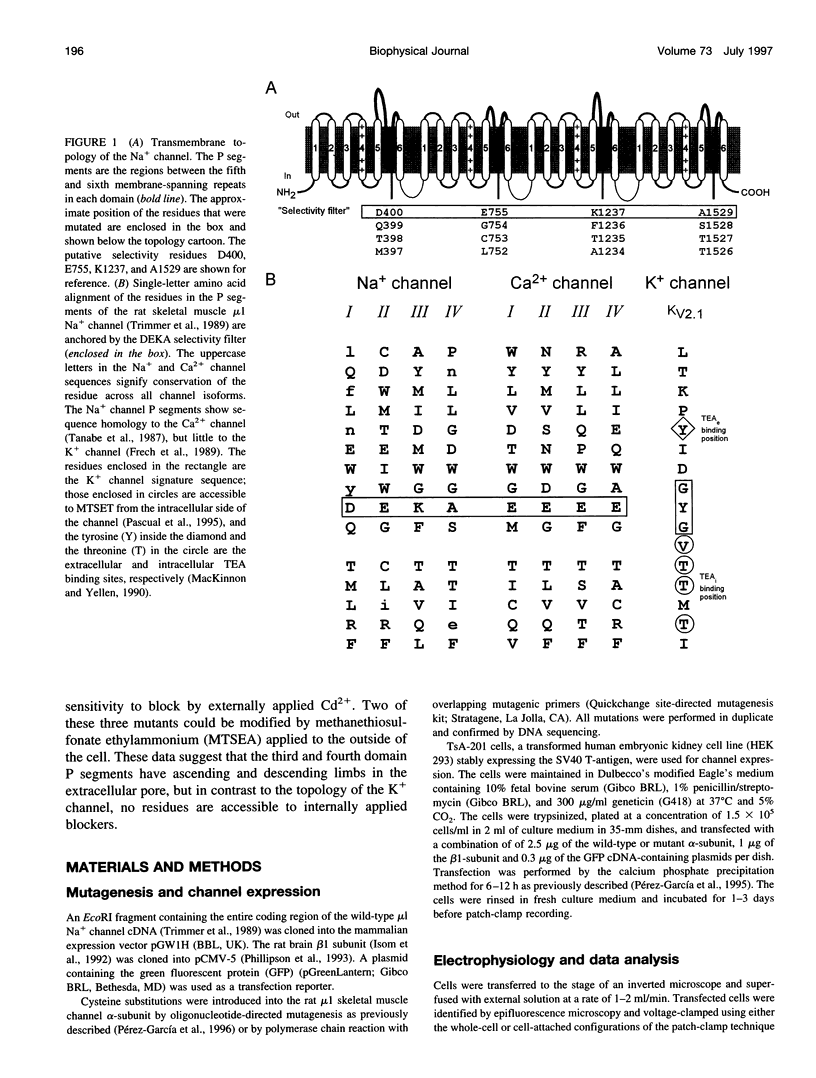
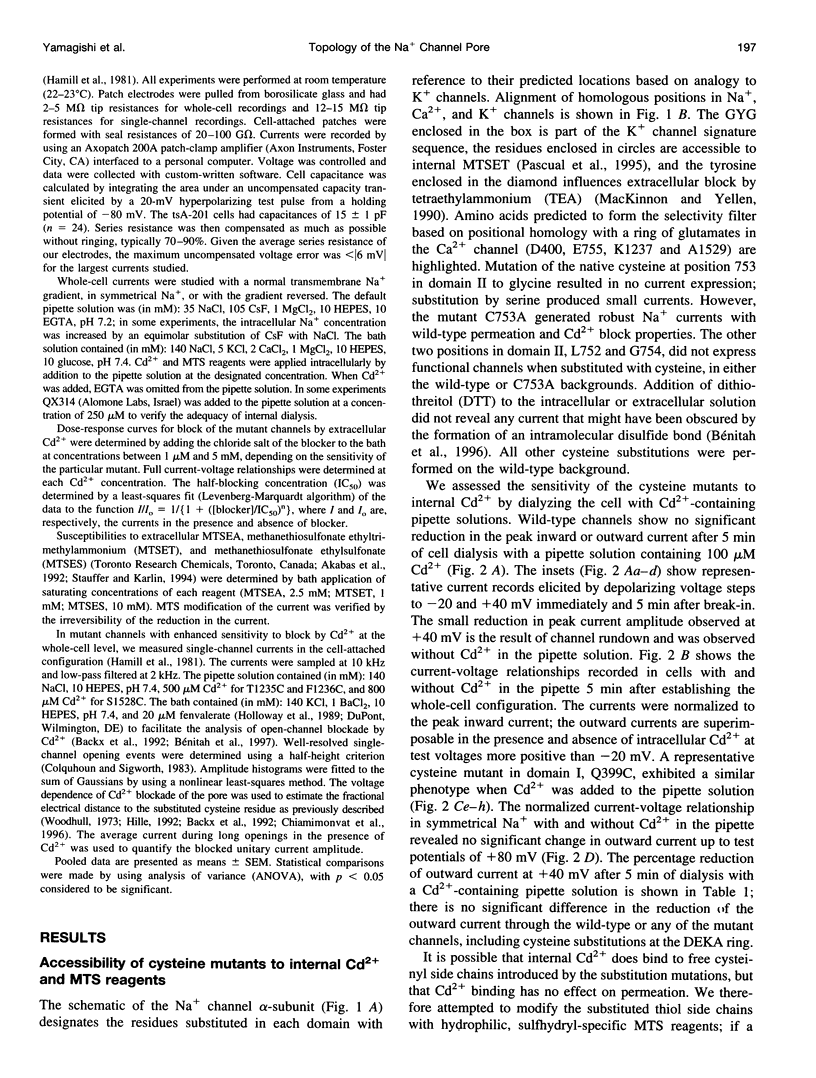
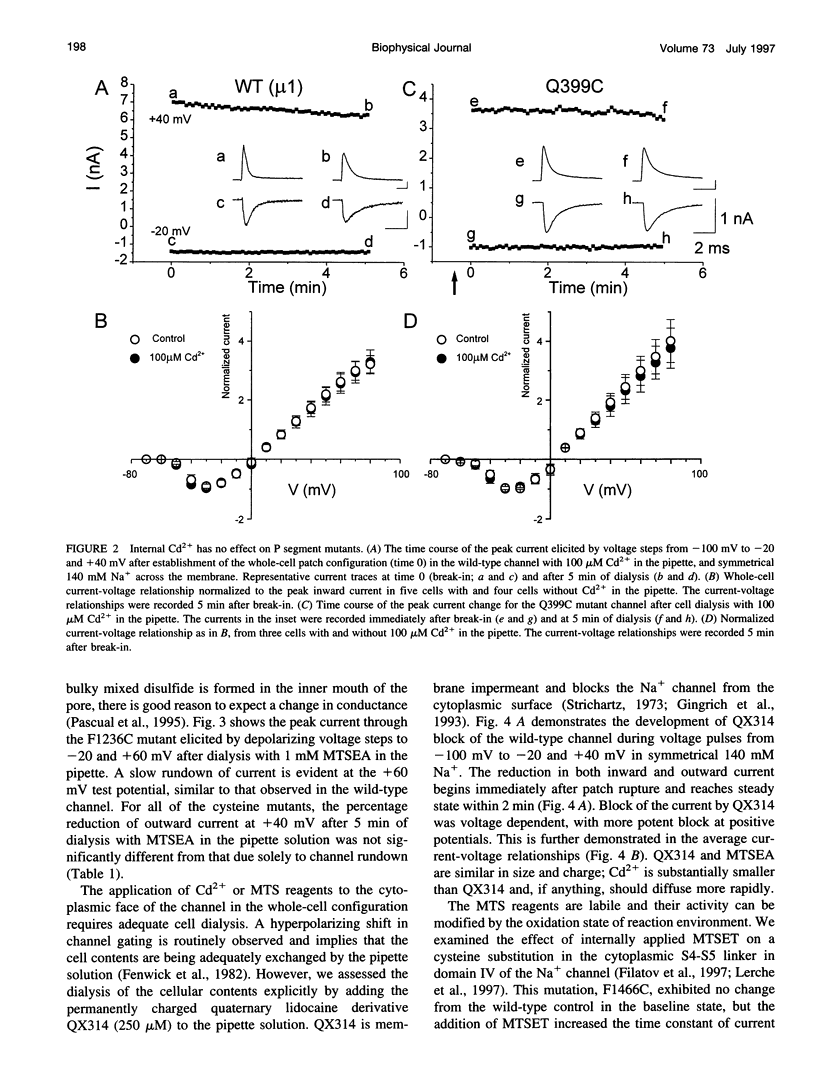
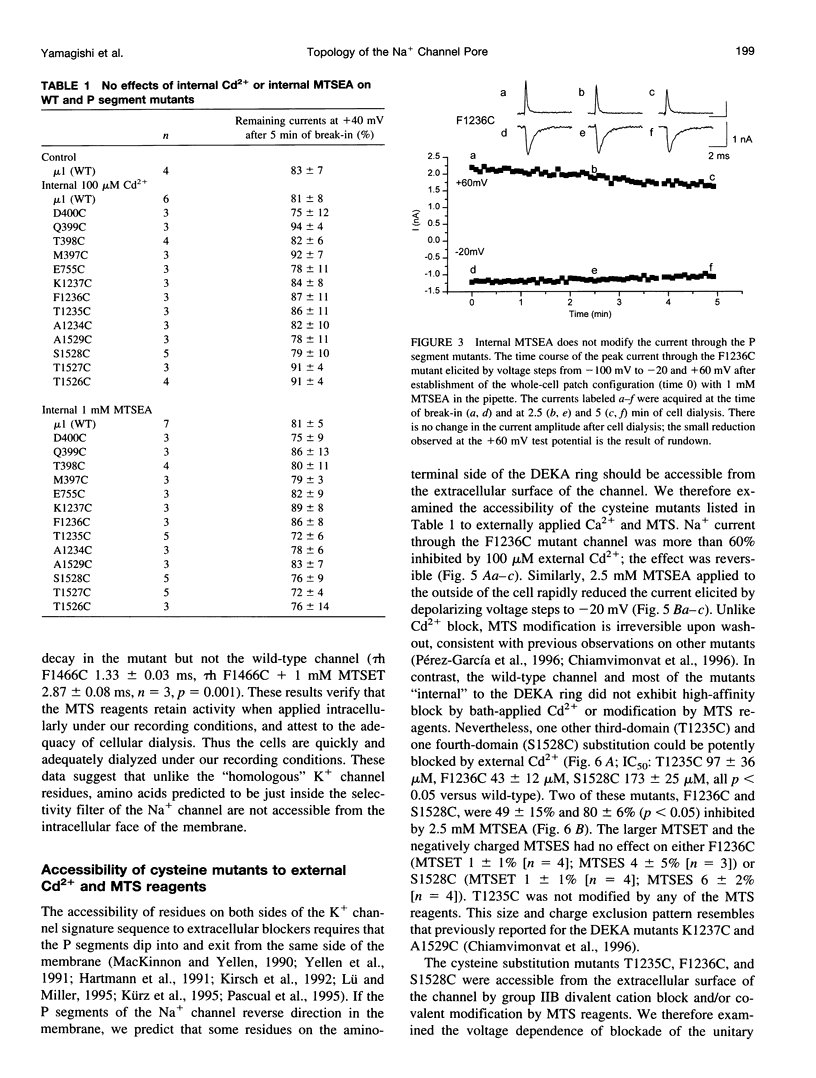
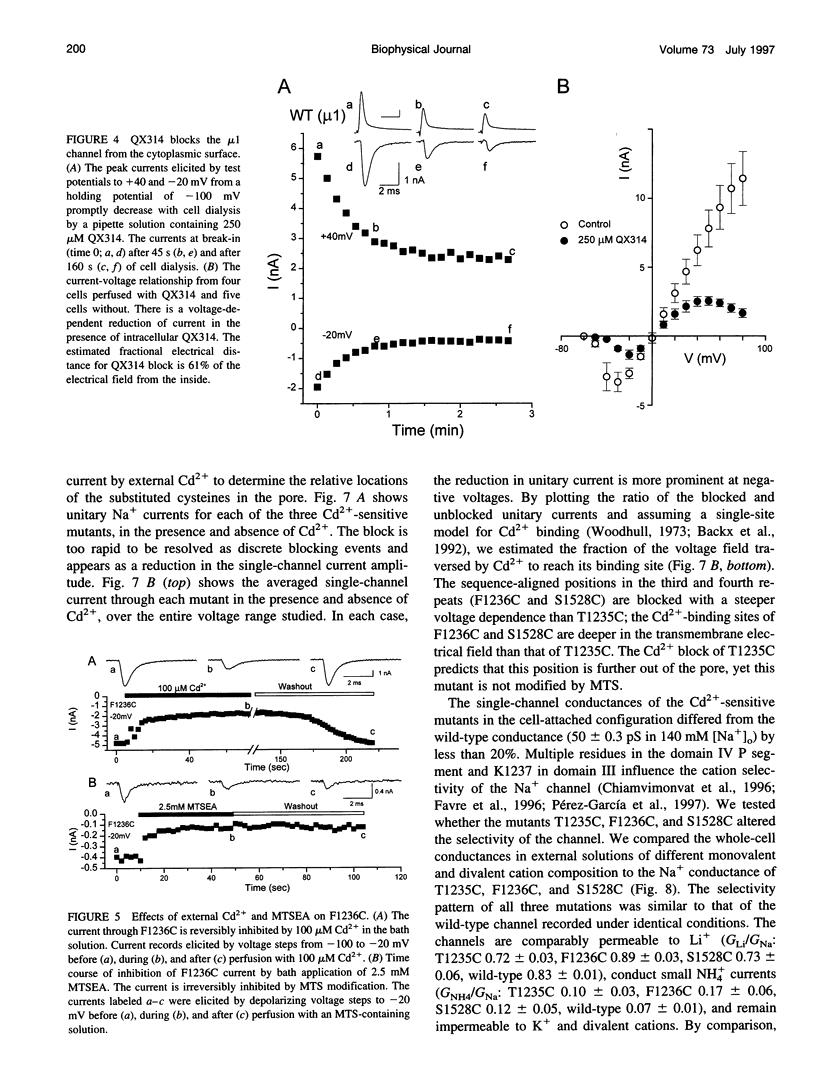
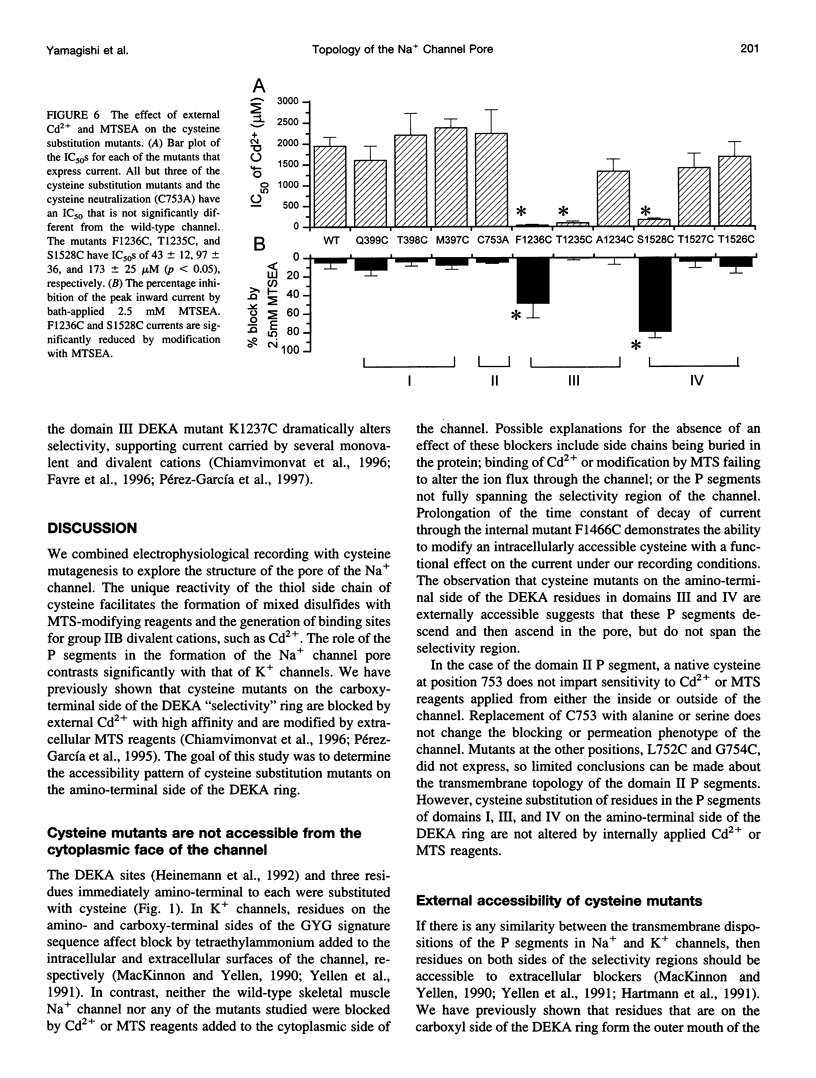
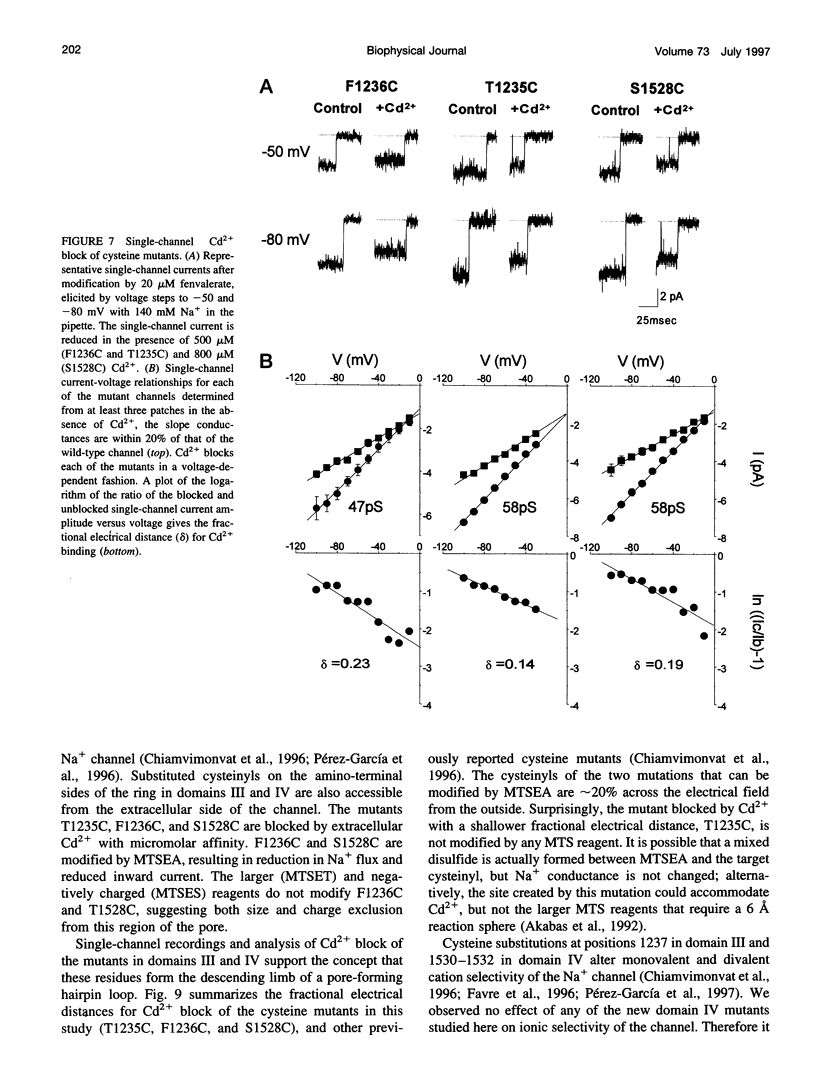
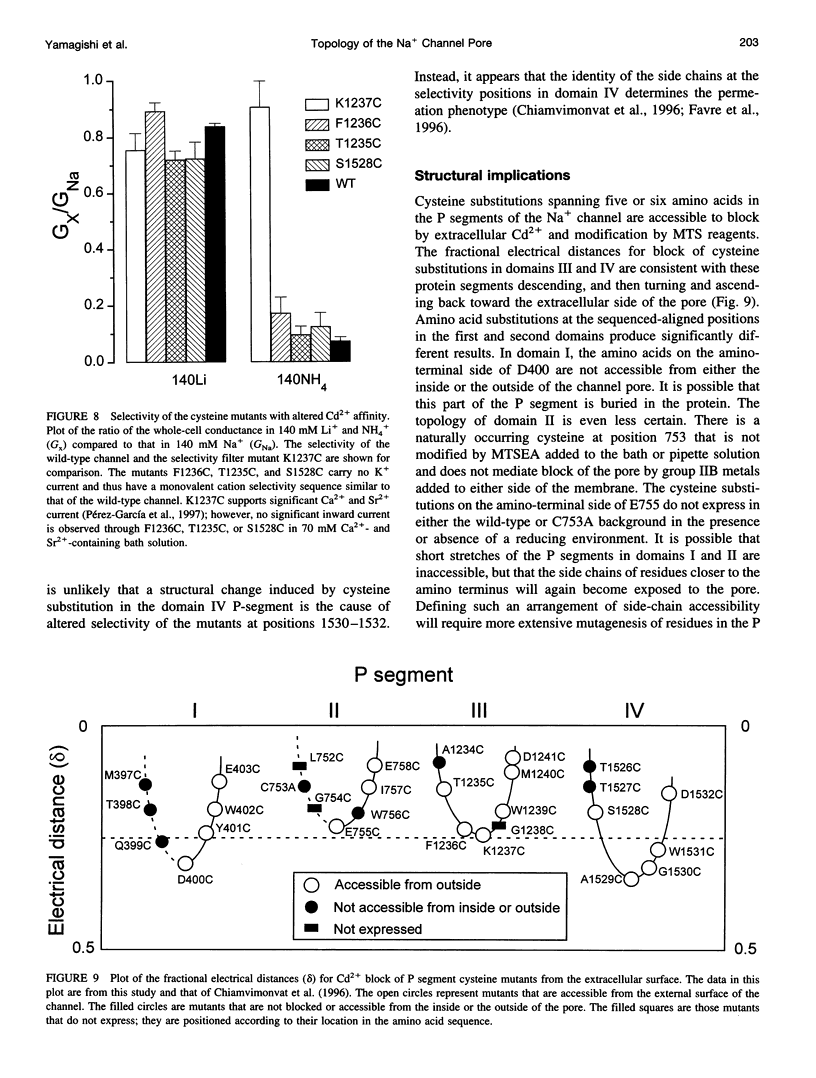
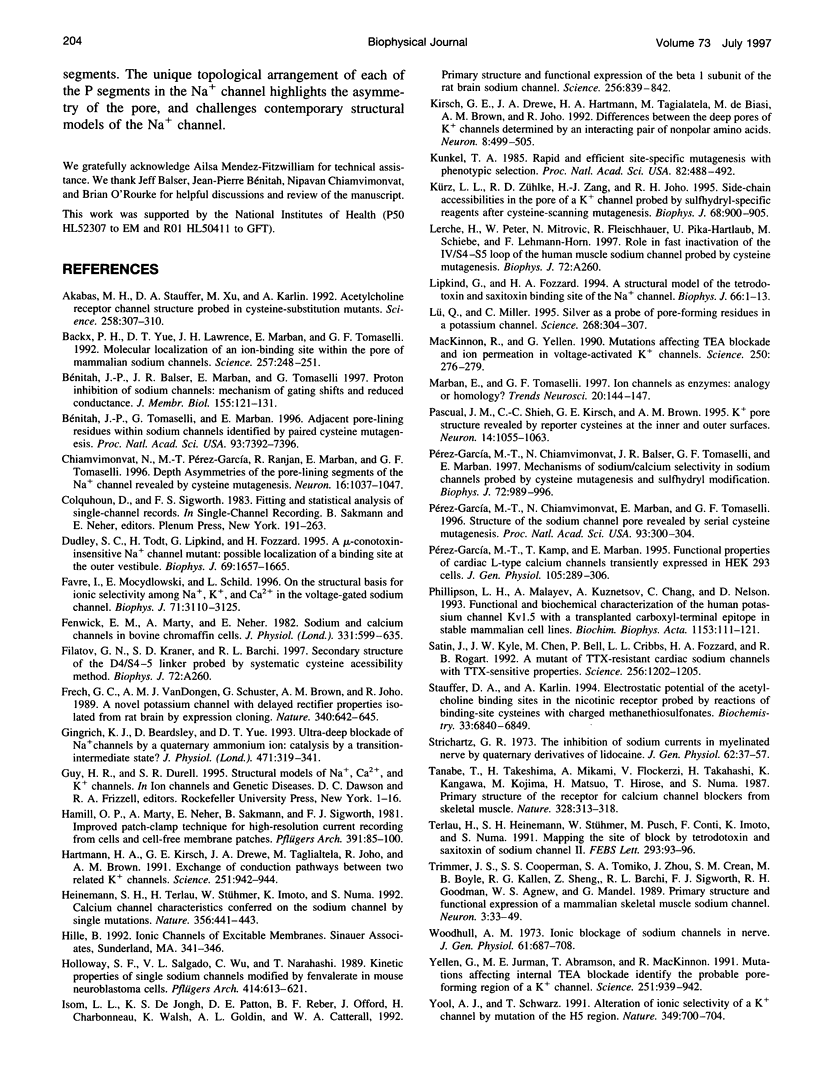
Selected References
These references are in PubMed. This may not be the complete list of references from this article.
- Akabas M. H., Stauffer D. A., Xu M., Karlin A. Acetylcholine receptor channel structure probed in cysteine-substitution mutants. Science. 1992 Oct 9;258(5080):307–310. doi: 10.1126/science.1384130. [DOI] [PubMed] [Google Scholar]
- Backx P. H., Yue D. T., Lawrence J. H., Marban E., Tomaselli G. F. Molecular localization of an ion-binding site within the pore of mammalian sodium channels. Science. 1992 Jul 10;257(5067):248–251. doi: 10.1126/science.1321496. [DOI] [PubMed] [Google Scholar]
- Bénitah J. P., Tomaselli G. F., Marban E. Adjacent pore-lining residues within sodium channels identified by paired cysteine mutagenesis. Proc Natl Acad Sci U S A. 1996 Jul 9;93(14):7392–7396. doi: 10.1073/pnas.93.14.7392. [DOI] [PMC free article] [PubMed] [Google Scholar]
- Bénitah J., Balser J. R., Marban E., Tomaselli G. F. Proton inhibition of sodium channels: mechanism of gating shifts and reduced conductance. J Membr Biol. 1997 Jan 15;155(2):121–131. doi: 10.1007/s002329900164. [DOI] [PubMed] [Google Scholar]
- Chiamvimonvat N., Pérez-García M. T., Ranjan R., Marban E., Tomaselli G. F. Depth asymmetries of the pore-lining segments of the Na+ channel revealed by cysteine mutagenesis. Neuron. 1996 May;16(5):1037–1047. doi: 10.1016/s0896-6273(00)80127-0. [DOI] [PubMed] [Google Scholar]
- Dudley S. C., Jr, Todt H., Lipkind G., Fozzard H. A. A mu-conotoxin-insensitive Na+ channel mutant: possible localization of a binding site at the outer vestibule. Biophys J. 1995 Nov;69(5):1657–1665. doi: 10.1016/S0006-3495(95)80045-7. [DOI] [PMC free article] [PubMed] [Google Scholar]
- Favre I., Moczydlowski E., Schild L. On the structural basis for ionic selectivity among Na+, K+, and Ca2+ in the voltage-gated sodium channel. Biophys J. 1996 Dec;71(6):3110–3125. doi: 10.1016/S0006-3495(96)79505-X. [DOI] [PMC free article] [PubMed] [Google Scholar]
- Fenwick E. M., Marty A., Neher E. Sodium and calcium channels in bovine chromaffin cells. J Physiol. 1982 Oct;331:599–635. doi: 10.1113/jphysiol.1982.sp014394. [DOI] [PMC free article] [PubMed] [Google Scholar]
- Frech G. C., VanDongen A. M., Schuster G., Brown A. M., Joho R. H. A novel potassium channel with delayed rectifier properties isolated from rat brain by expression cloning. Nature. 1989 Aug 24;340(6235):642–645. doi: 10.1038/340642a0. [DOI] [PubMed] [Google Scholar]
- Gingrich K. J., Beardsley D., Yue D. T. Ultra-deep blockade of Na+ channels by a quaternary ammonium ion: catalysis by a transition-intermediate state? J Physiol. 1993 Nov;471:319–341. doi: 10.1113/jphysiol.1993.sp019903. [DOI] [PMC free article] [PubMed] [Google Scholar]
- Hamill O. P., Marty A., Neher E., Sakmann B., Sigworth F. J. Improved patch-clamp techniques for high-resolution current recording from cells and cell-free membrane patches. Pflugers Arch. 1981 Aug;391(2):85–100. doi: 10.1007/BF00656997. [DOI] [PubMed] [Google Scholar]
- Hartmann H. A., Kirsch G. E., Drewe J. A., Taglialatela M., Joho R. H., Brown A. M. Exchange of conduction pathways between two related K+ channels. Science. 1991 Feb 22;251(4996):942–944. doi: 10.1126/science.2000495. [DOI] [PubMed] [Google Scholar]
- Heinemann S. H., Terlau H., Stühmer W., Imoto K., Numa S. Calcium channel characteristics conferred on the sodium channel by single mutations. Nature. 1992 Apr 2;356(6368):441–443. doi: 10.1038/356441a0. [DOI] [PubMed] [Google Scholar]
- Holloway S. F., Salgado V. L., Wu C. H., Narahashi T. Kinetic properties of single sodium channels modified by fenvalerate in mouse neuroblastoma cells. Pflugers Arch. 1989 Sep;414(6):613–621. doi: 10.1007/BF00582125. [DOI] [PubMed] [Google Scholar]
- Isom L. L., De Jongh K. S., Patton D. E., Reber B. F., Offord J., Charbonneau H., Walsh K., Goldin A. L., Catterall W. A. Primary structure and functional expression of the beta 1 subunit of the rat brain sodium channel. Science. 1992 May 8;256(5058):839–842. doi: 10.1126/science.1375395. [DOI] [PubMed] [Google Scholar]
- Kirsch G. E., Drewe J. A., Hartmann H. A., Taglialatela M., de Biasi M., Brown A. M., Joho R. H. Differences between the deep pores of K+ channels determined by an interacting pair of nonpolar amino acids. Neuron. 1992 Mar;8(3):499–505. doi: 10.1016/0896-6273(92)90278-l. [DOI] [PubMed] [Google Scholar]
- Kunkel T. A. Rapid and efficient site-specific mutagenesis without phenotypic selection. Proc Natl Acad Sci U S A. 1985 Jan;82(2):488–492. doi: 10.1073/pnas.82.2.488. [DOI] [PMC free article] [PubMed] [Google Scholar]
- Kürz L. L., Zühlke R. D., Zhang H. J., Joho R. H. Side-chain accessibilities in the pore of a K+ channel probed by sulfhydryl-specific reagents after cysteine-scanning mutagenesis. Biophys J. 1995 Mar;68(3):900–905. doi: 10.1016/S0006-3495(95)80266-3. [DOI] [PMC free article] [PubMed] [Google Scholar]
- Lipkind G. M., Fozzard H. A. A structural model of the tetrodotoxin and saxitoxin binding site of the Na+ channel. Biophys J. 1994 Jan;66(1):1–13. doi: 10.1016/S0006-3495(94)80746-5. [DOI] [PMC free article] [PubMed] [Google Scholar]
- Lü Q., Miller C. Silver as a probe of pore-forming residues in a potassium channel. Science. 1995 Apr 14;268(5208):304–307. doi: 10.1126/science.7716526. [DOI] [PubMed] [Google Scholar]
- MacKinnon R., Yellen G. Mutations affecting TEA blockade and ion permeation in voltage-activated K+ channels. Science. 1990 Oct 12;250(4978):276–279. doi: 10.1126/science.2218530. [DOI] [PubMed] [Google Scholar]
- Marban E., Tomaselli G. F. Ion channels as enzymes: analogy or homology? Trends Neurosci. 1997 Apr;20(4):144–147. doi: 10.1016/s0166-2236(96)01008-9. [DOI] [PubMed] [Google Scholar]
- Pascual J. M., Shieh C. C., Kirsch G. E., Brown A. M. K+ pore structure revealed by reporter cysteines at inner and outer surfaces. Neuron. 1995 May;14(5):1055–1063. doi: 10.1016/0896-6273(95)90344-5. [DOI] [PubMed] [Google Scholar]
- Philipson L. H., Malayev A., Kuznetsov A., Chang C., Nelson D. J. Functional and biochemical characterization of the human potassium channel Kv1.5 with a transplanted carboxyl-terminal epitope in stable mammalian cell lines. Biochim Biophys Acta. 1993 Nov 21;1153(1):111–121. doi: 10.1016/0005-2736(93)90282-5. [DOI] [PubMed] [Google Scholar]
- Pérez-García M. T., Chiamvimonvat N., Marban E., Tomaselli G. F. Structure of the sodium channel pore revealed by serial cysteine mutagenesis. Proc Natl Acad Sci U S A. 1996 Jan 9;93(1):300–304. doi: 10.1073/pnas.93.1.300. [DOI] [PMC free article] [PubMed] [Google Scholar]
- Pérez-García M. T., Chiamvimonvat N., Ranjan R., Balser J. R., Tomaselli G. F., Marban E. Mechanisms of sodium/calcium selectivity in sodium channels probed by cysteine mutagenesis and sulfhydryl modification. Biophys J. 1997 Mar;72(3):989–996. doi: 10.1016/S0006-3495(97)78751-4. [DOI] [PMC free article] [PubMed] [Google Scholar]
- Pérez-García M. T., Kamp T. J., Marbán E. Functional properties of cardiac L-type calcium channels transiently expressed in HEK293 cells. Roles of alpha 1 and beta subunits. J Gen Physiol. 1995 Feb;105(2):289–305. doi: 10.1085/jgp.105.2.289. [DOI] [PMC free article] [PubMed] [Google Scholar]
- Satin J., Kyle J. W., Chen M., Bell P., Cribbs L. L., Fozzard H. A., Rogart R. B. A mutant of TTX-resistant cardiac sodium channels with TTX-sensitive properties. Science. 1992 May 22;256(5060):1202–1205. doi: 10.1126/science.256.5060.1202. [DOI] [PubMed] [Google Scholar]
- Stauffer D. A., Karlin A. Electrostatic potential of the acetylcholine binding sites in the nicotinic receptor probed by reactions of binding-site cysteines with charged methanethiosulfonates. Biochemistry. 1994 Jun 7;33(22):6840–6849. doi: 10.1021/bi00188a013. [DOI] [PubMed] [Google Scholar]
- Strichartz G. R. The inhibition of sodium currents in myelinated nerve by quaternary derivatives of lidocaine. J Gen Physiol. 1973 Jul;62(1):37–57. doi: 10.1085/jgp.62.1.37. [DOI] [PMC free article] [PubMed] [Google Scholar]
- Tanabe T., Takeshima H., Mikami A., Flockerzi V., Takahashi H., Kangawa K., Kojima M., Matsuo H., Hirose T., Numa S. Primary structure of the receptor for calcium channel blockers from skeletal muscle. Nature. 1987 Jul 23;328(6128):313–318. doi: 10.1038/328313a0. [DOI] [PubMed] [Google Scholar]
- Terlau H., Heinemann S. H., Stühmer W., Pusch M., Conti F., Imoto K., Numa S. Mapping the site of block by tetrodotoxin and saxitoxin of sodium channel II. FEBS Lett. 1991 Nov 18;293(1-2):93–96. doi: 10.1016/0014-5793(91)81159-6. [DOI] [PubMed] [Google Scholar]
- Trimmer J. S., Cooperman S. S., Tomiko S. A., Zhou J. Y., Crean S. M., Boyle M. B., Kallen R. G., Sheng Z. H., Barchi R. L., Sigworth F. J. Primary structure and functional expression of a mammalian skeletal muscle sodium channel. Neuron. 1989 Jul;3(1):33–49. doi: 10.1016/0896-6273(89)90113-x. [DOI] [PubMed] [Google Scholar]
- Woodhull A. M. Ionic blockage of sodium channels in nerve. J Gen Physiol. 1973 Jun;61(6):687–708. doi: 10.1085/jgp.61.6.687. [DOI] [PMC free article] [PubMed] [Google Scholar]
- Yellen G., Jurman M. E., Abramson T., MacKinnon R. Mutations affecting internal TEA blockade identify the probable pore-forming region of a K+ channel. Science. 1991 Feb 22;251(4996):939–942. doi: 10.1126/science.2000494. [DOI] [PubMed] [Google Scholar]
- Yool A. J., Schwarz T. L. Alteration of ionic selectivity of a K+ channel by mutation of the H5 region. Nature. 1991 Feb 21;349(6311):700–704. doi: 10.1038/349700a0. [DOI] [PubMed] [Google Scholar]