Abstract
The first study of enzymatic hydrolysis of phospholipid tubules is reported. Phosphatidylcholines with acyl chains containing diacetylene groups are known to form tubular microstructures in which the lipids are tightly packed and crystalline. These tubules can be used to probe the role of microstructural form in the mechanics of interfacial enzymatic degradation by such enzymes as phospholipase A2 (PLA2). Hydrolysis by PLA2 may occur most rapidly in regions having the greatest number of bilayer packing defects, such as those that must be found at tubule ends. A microstructure that degrades primarily from its ends should exhibit zero-order kinetics, because the area of the degrading tubule and remains constant as the length of the microstructure decreases. Free fatty acid concentration was measured to follow the generation of PLA2 hydrolysis products in suspensions of diacetylenic phospholipid tubules. The kinetics of tubule hydrolysis were essentially zero-order until conversion was complete, as predicted. However, microscopy of partially hydrolyzed tubules revealed the formation of multiple discrete anionic product domains along the length of degrading tubules as well as in insoluble reaction product microstructures. Furthermore, the rate of tubule hydrolysis was only moderately enhanced by increasing the number of tubule ends, which is consistent with the conclusion that tubule ends are not the only sites of hydrolysis. A model that reconciles the overall kinetics with the morphological evidence is proposed.
Full text
PDF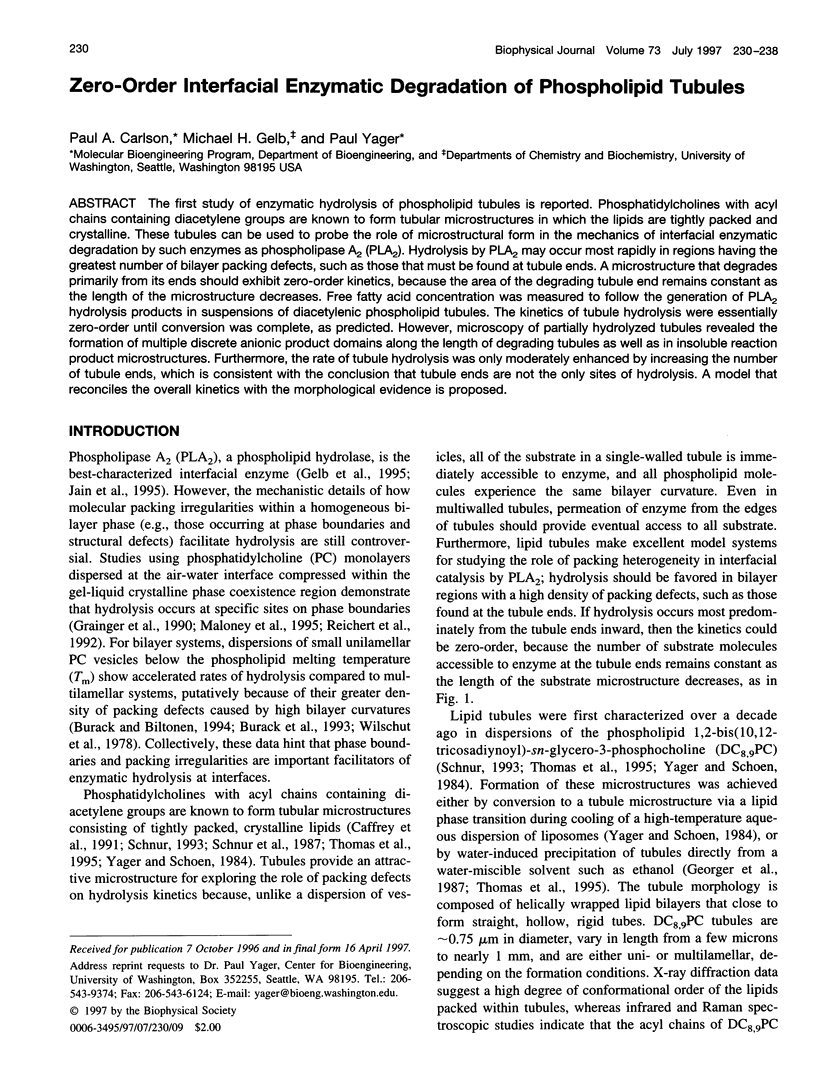
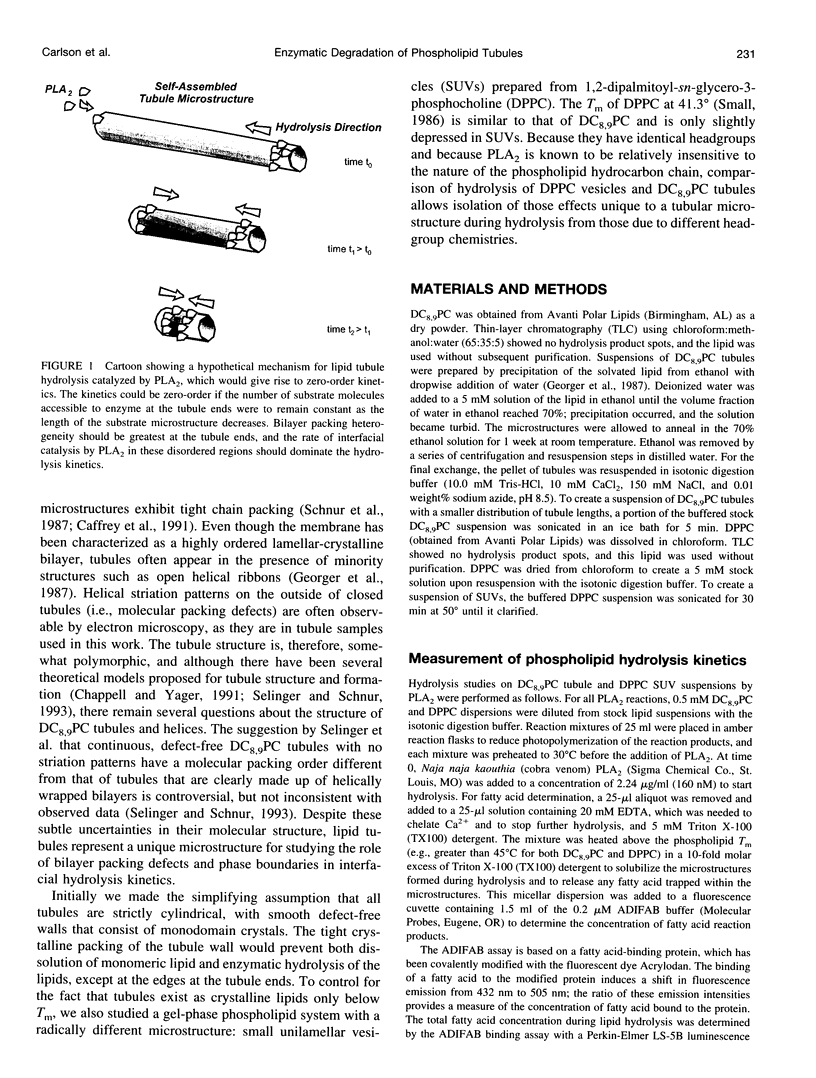
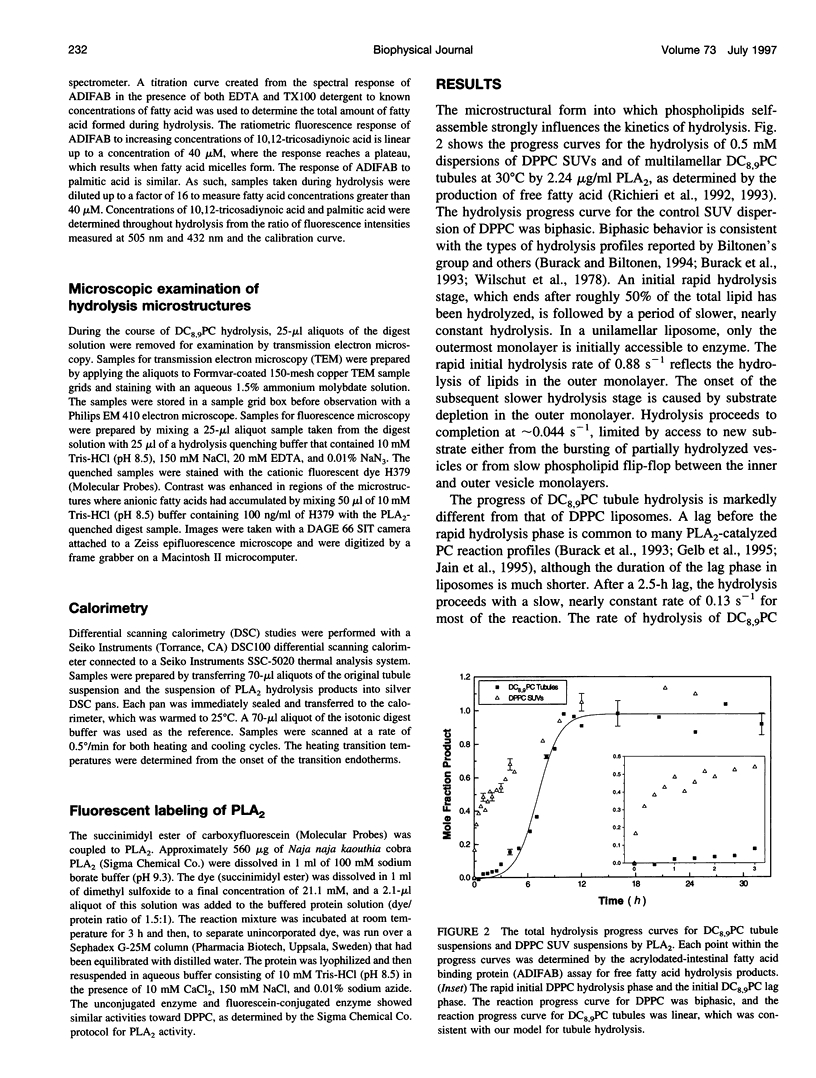
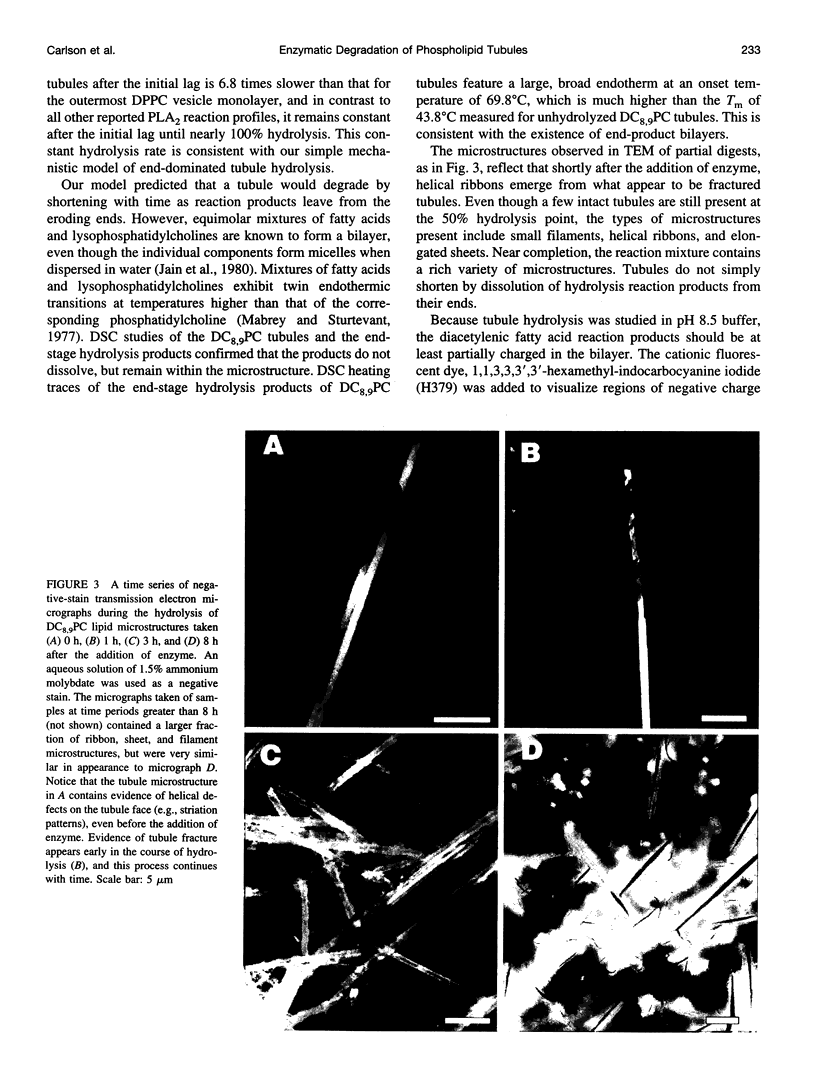
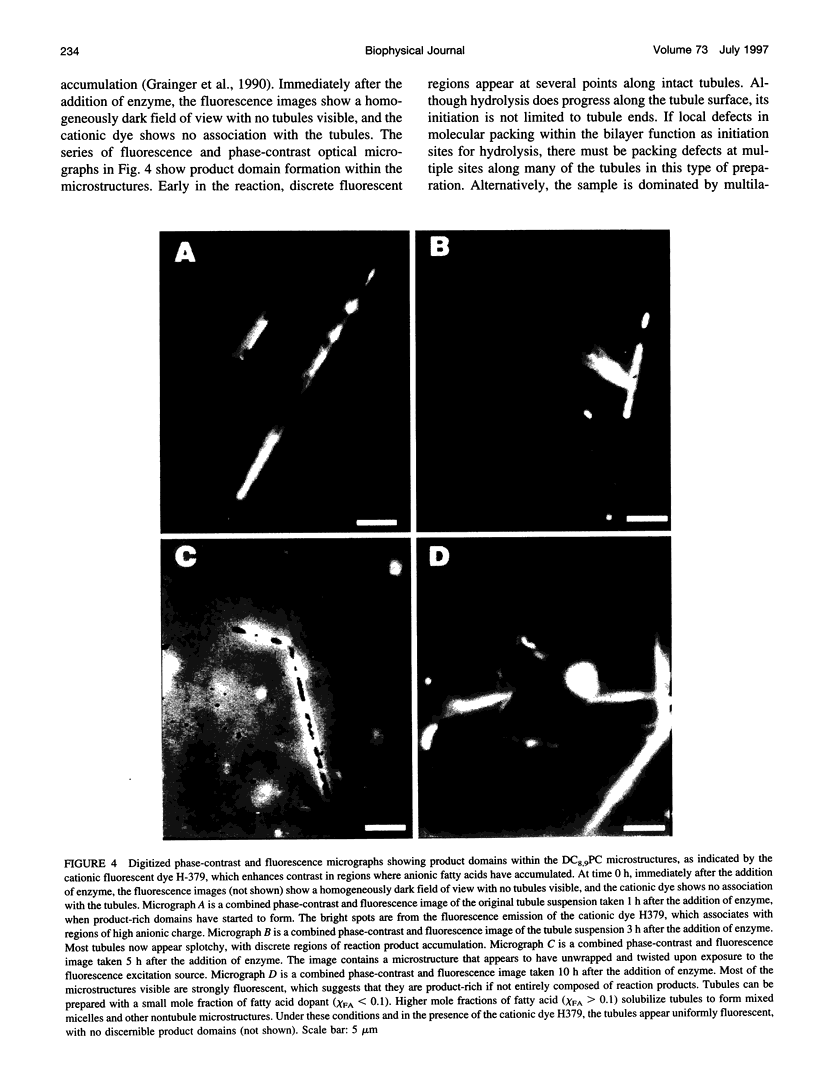
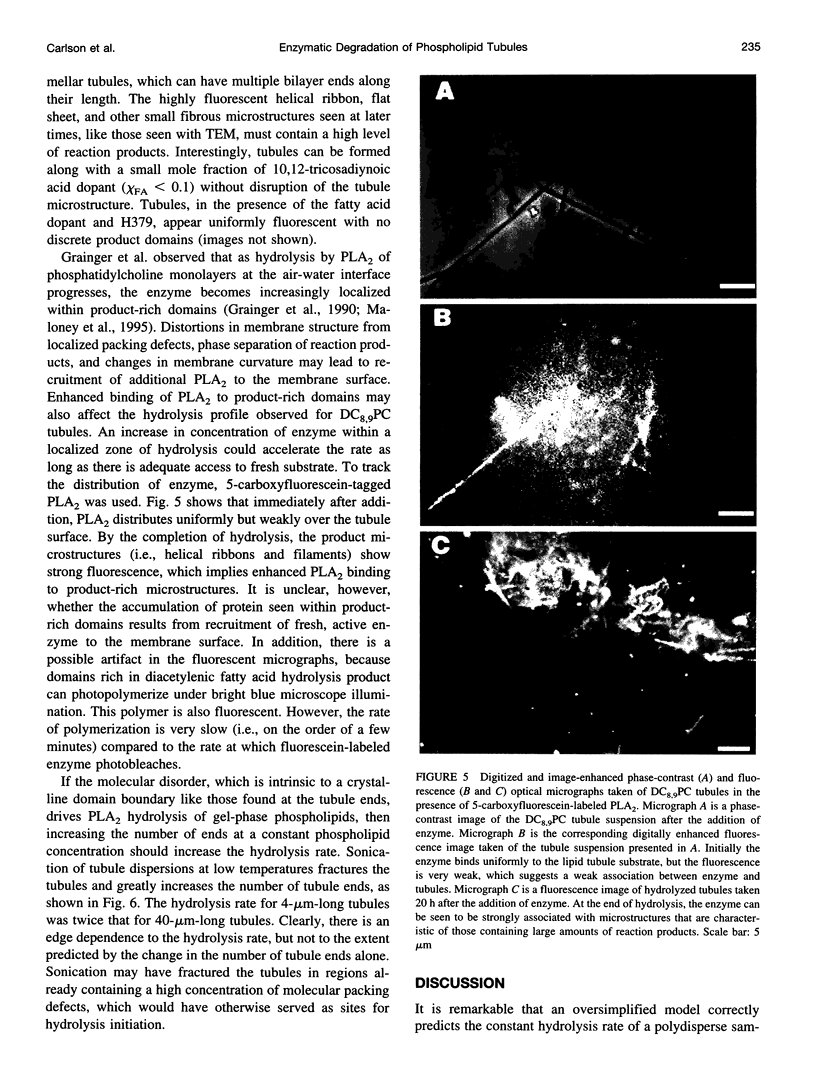
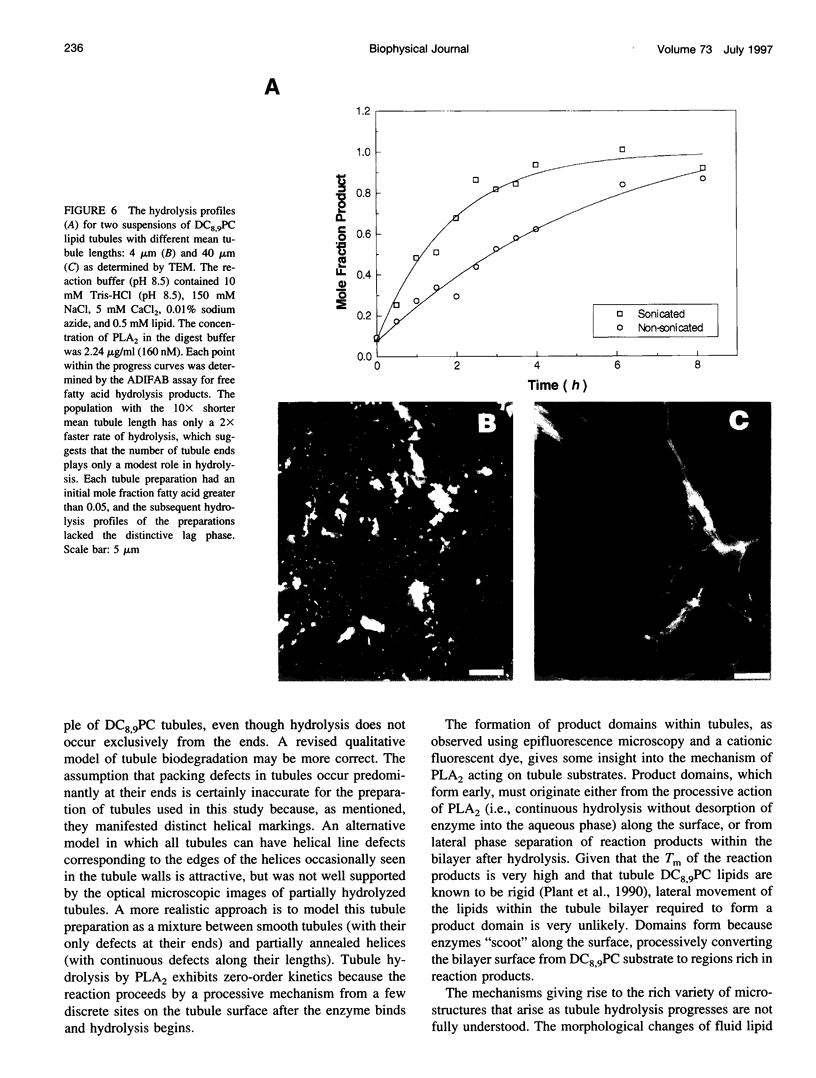
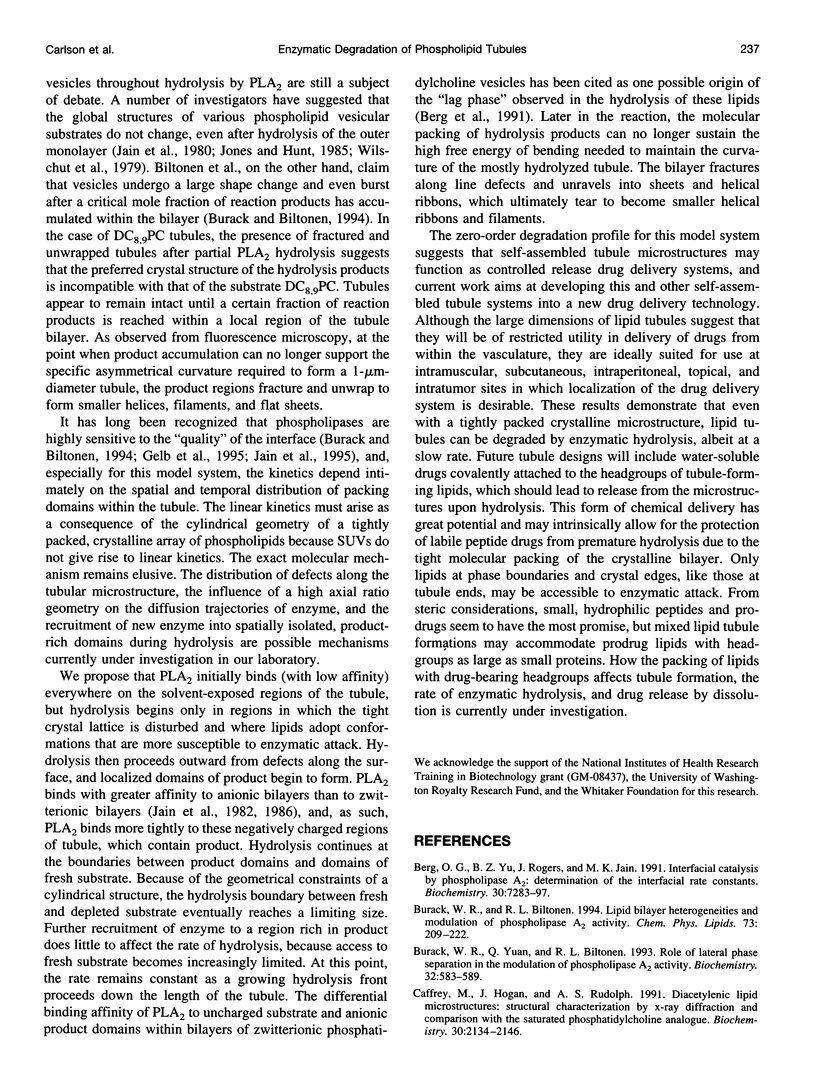
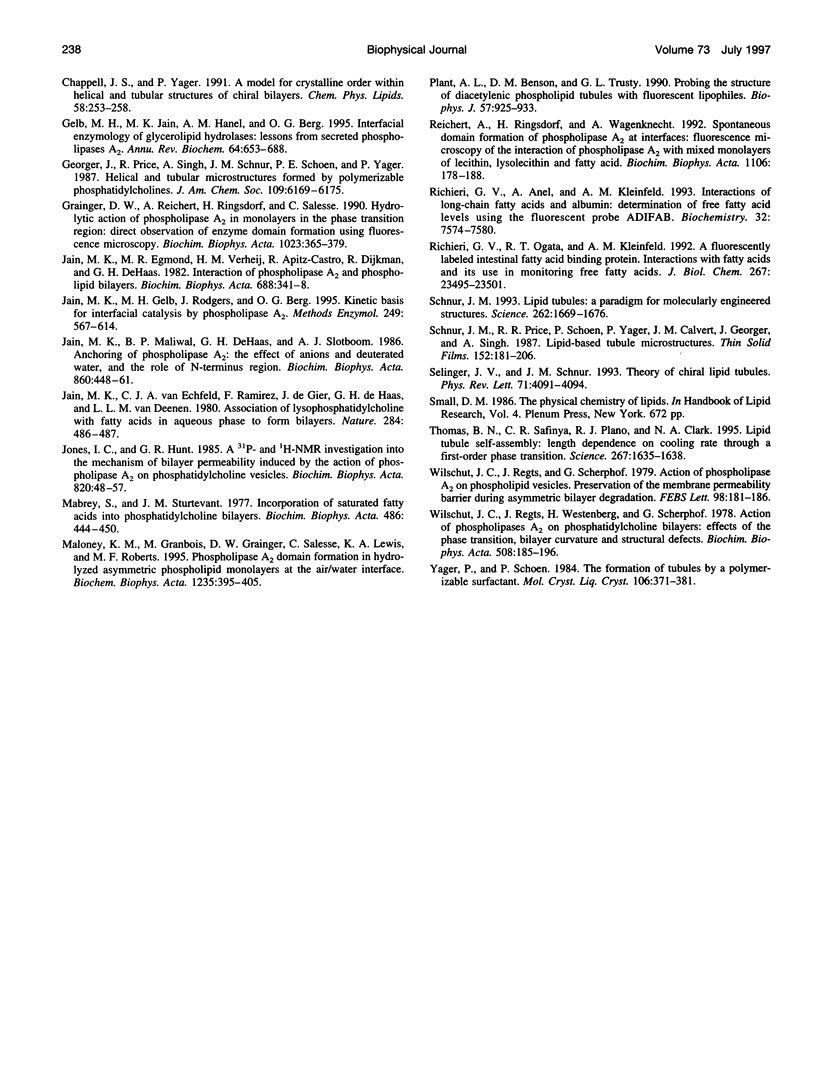
Images in this article
Selected References
These references are in PubMed. This may not be the complete list of references from this article.
- Berg O. G., Yu B. Z., Rogers J., Jain M. K. Interfacial catalysis by phospholipase A2: determination of the interfacial kinetic rate constants. Biochemistry. 1991 Jul 23;30(29):7283–7297. doi: 10.1021/bi00243a034. [DOI] [PubMed] [Google Scholar]
- Burack W. R., Biltonen R. L. Lipid bilayer heterogeneities and modulation of phospholipase A2 activity. Chem Phys Lipids. 1994 Sep 6;73(1-2):209–222. doi: 10.1016/0009-3084(94)90182-1. [DOI] [PubMed] [Google Scholar]
- Burack W. R., Yuan Q., Biltonen R. L. Role of lateral phase separation in the modulation of phospholipase A2 activity. Biochemistry. 1993 Jan 19;32(2):583–589. doi: 10.1021/bi00053a025. [DOI] [PubMed] [Google Scholar]
- Caffrey M., Hogan J., Rudolph A. S. Diacetylenic lipid microstructures: structural characterization by X-ray diffraction and comparison with the saturated phosphatidylcholine analogue. Biochemistry. 1991 Feb 26;30(8):2134–2146. doi: 10.1021/bi00222a018. [DOI] [PubMed] [Google Scholar]
- Gelb M. H., Jain M. K., Hanel A. M., Berg O. G. Interfacial enzymology of glycerolipid hydrolases: lessons from secreted phospholipases A2. Annu Rev Biochem. 1995;64:653–688. doi: 10.1146/annurev.bi.64.070195.003253. [DOI] [PubMed] [Google Scholar]
- Grainger D. W., Reichert A., Ringsdorf H., Salesse C. Hydrolytic action of phospholipase A2 in monolayers in the phase transition region: direct observation of enzyme domain formation using fluorescence microscopy. Biochim Biophys Acta. 1990 Apr 30;1023(3):365–379. doi: 10.1016/0005-2736(90)90128-b. [DOI] [PubMed] [Google Scholar]
- Jain M. K., Egmond M. R., Verheij H. M., Apitz-Castro R., Dijkman R., De Haas G. H. Interaction of phospholipase A2 and phospholipid bilayers. Biochim Biophys Acta. 1982 Jun 14;688(2):341–348. doi: 10.1016/0005-2736(82)90345-5. [DOI] [PubMed] [Google Scholar]
- Jain M. K., Gelb M. H., Rogers J., Berg O. G. Kinetic basis for interfacial catalysis by phospholipase A2. Methods Enzymol. 1995;249:567–614. doi: 10.1016/0076-6879(95)49049-3. [DOI] [PubMed] [Google Scholar]
- Jain M. K., Maliwal B. P., DeHaas G. H., Slotboom A. J. Anchoring of phospholipase A2: the effect of anions and deuterated water, and the role of N-terminus region. Biochim Biophys Acta. 1986 Sep 11;860(3):448–461. doi: 10.1016/0005-2736(86)90542-0. [DOI] [PubMed] [Google Scholar]
- Jain M. K., van Echteld C. J., Ramirez F., de Gier J., de Haas G. H., van Deenen L. L. Association of lysophosphatidylcholine with fatty acids in aqueous phase to form bilayers. Nature. 1980 Apr 3;284(5755):486–487. doi: 10.1038/284486a0. [DOI] [PubMed] [Google Scholar]
- Mabrey S., Sturtevant J. M. Incorporation of saturated fatty acids into phosphatidylcholine bilayers. Biochim Biophys Acta. 1977 Mar 25;486(3):444–450. doi: 10.1016/0005-2760(77)90094-7. [DOI] [PubMed] [Google Scholar]
- Maloney K. M., Grandbois M., Grainger D. W., Salesse C., Lewis K. A., Roberts M. F. Phospholipase A2 domain formation in hydrolyzed asymmetric phospholipid monolayers at the air/water interface. Biochim Biophys Acta. 1995 May 4;1235(2):395–405. doi: 10.1016/0005-2736(95)80029-f. [DOI] [PubMed] [Google Scholar]
- Plant A. L., Benson D. M., Trusty G. L. Probing the structure of diacetylenic phospholipid tubules with fluorescent lipophiles. Biophys J. 1990 May;57(5):925–933. doi: 10.1016/S0006-3495(90)82612-6. [DOI] [PMC free article] [PubMed] [Google Scholar]
- Reichert A., Ringsdorf H., Wagenknecht A. Spontaneous domain formation of phospholipase A2 at interfaces: fluorescence microscopy of the interaction of phospholipase A2 with mixed monolayers of lecithin, lysolecithin and fatty acid. Biochim Biophys Acta. 1992 Apr 29;1106(1):178–188. doi: 10.1016/0005-2736(92)90237-g. [DOI] [PubMed] [Google Scholar]
- Richieri G. V., Anel A., Kleinfeld A. M. Interactions of long-chain fatty acids and albumin: determination of free fatty acid levels using the fluorescent probe ADIFAB. Biochemistry. 1993 Jul 27;32(29):7574–7580. doi: 10.1021/bi00080a032. [DOI] [PubMed] [Google Scholar]
- Richieri G. V., Ogata R. T., Kleinfeld A. M. A fluorescently labeled intestinal fatty acid binding protein. Interactions with fatty acids and its use in monitoring free fatty acids. J Biol Chem. 1992 Nov 25;267(33):23495–23501. [PubMed] [Google Scholar]
- Schnur J. M. Lipid tubules: a paradigm for molecularly engineered structures. Science. 1993 Dec 10;262(5140):1669–1676. doi: 10.1126/science.262.5140.1669. [DOI] [PubMed] [Google Scholar]
- Selinger JV, Schnur JM. Theory of chiral lipid tubules. Phys Rev Lett. 1993 Dec 13;71(24):4091–4094. doi: 10.1103/PhysRevLett.71.4091. [DOI] [PubMed] [Google Scholar]
- Thomas B. N., Safinya C. R., Plano R. J., Clark N. A. Lipid tubule self-assembly: length dependence on cooling rate through a first-order phase transition. Science. 1995 Mar 17;267(5204):1635–1638. doi: 10.1126/science.267.5204.1635. [DOI] [PubMed] [Google Scholar]
- Wilschut J. C., Regts J., Scherphof G. Action of phospholipase A2 on phospholipid vesicles. Preservation of the membrane permeability barrier during asymmetric bilayer degradation. FEBS Lett. 1979 Feb 1;98(1):181–186. doi: 10.1016/0014-5793(79)80179-9. [DOI] [PubMed] [Google Scholar]
- Wilschut J. C., Regts J., Westenberg H., Scherphof G. Action of phospholipases A2 on phosphatidylcholine bilayers. Effects of the phase transition, bilayer curvature and structural defects. Biochim Biophys Acta. 1978 Apr 4;508(2):185–196. doi: 10.1016/0005-2736(78)90324-3. [DOI] [PubMed] [Google Scholar]