Abstract
Mobile Ca2+ buffers in hair cells have been postulated to play a dual role. On one hand, they carry incoming Ca2+ away from synaptic areas, allowing synapses to be rapidly reset. On the other hand, they limit the spread of free Ca2+ into the cell, preventing cross-talk between different pathways that employ Ca2+ as a second messenger. We have obtained evidence for such mobile Ca2+ buffers in hair cells by comparing the patterns of Ca2+-induced fluo-3 fluorescence under whole-cell and perforated-patch recording conditions. Fluorescent signals under perforated-patch conditions are relatively weak and are limited to the immediate vicinity of the membrane. These observations can be explained by a diffusion-reaction scheme that, in addition to Ca2+ and fluo-3, incorporates endogenous fixed and mobile Ca2+ buffers. Our experiments also suggest that the mobility of the endogenous buffer might be higher than previously thought. A high buffer mobility is expected to enhance the cell's ability to rapidly modulate transmitter release.
Full text
PDF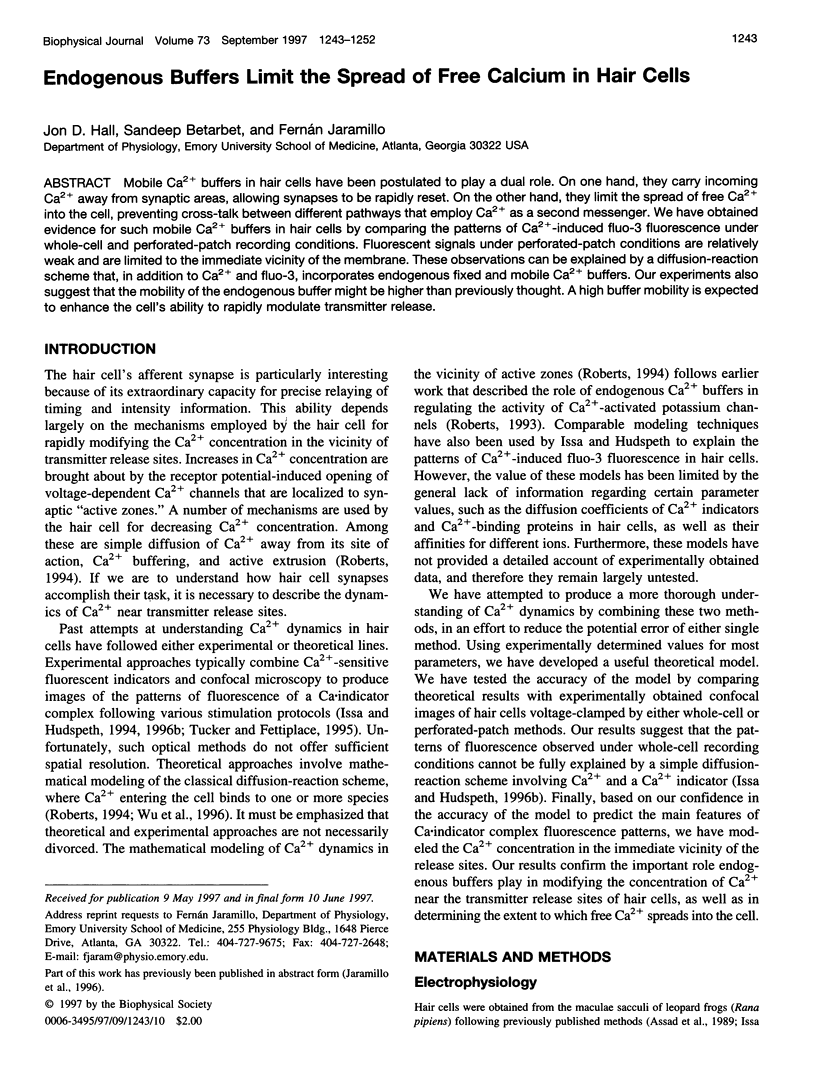
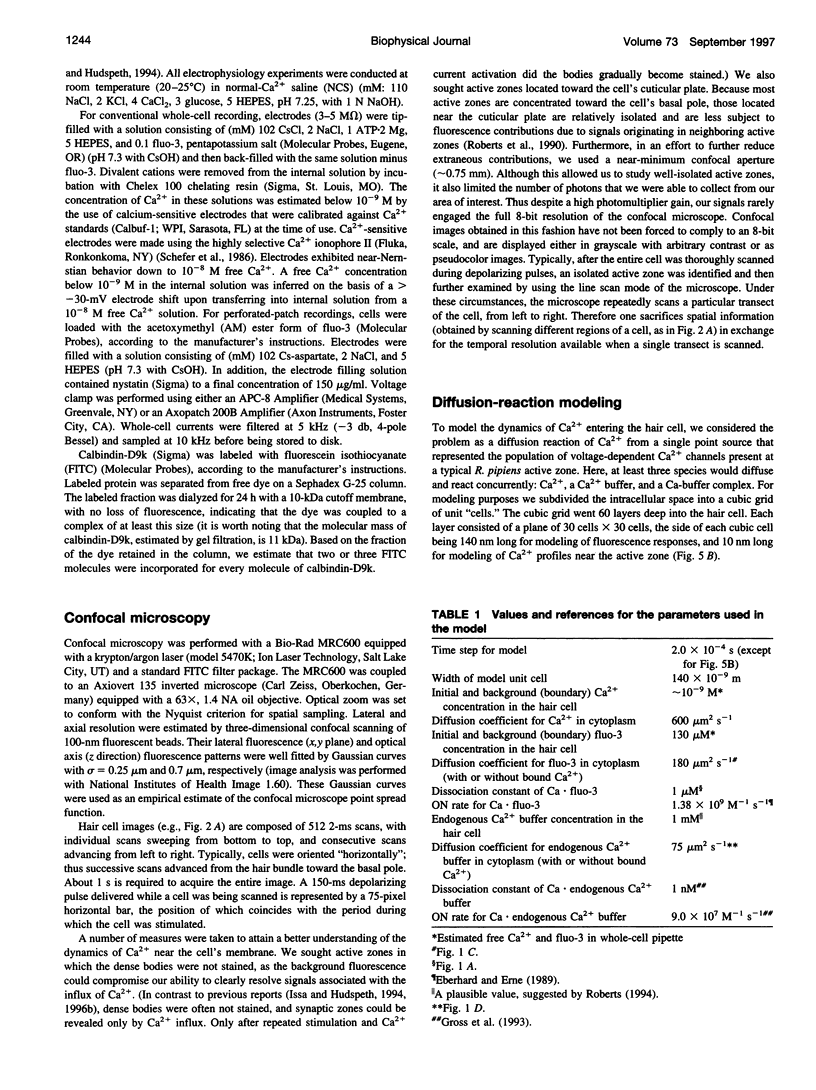
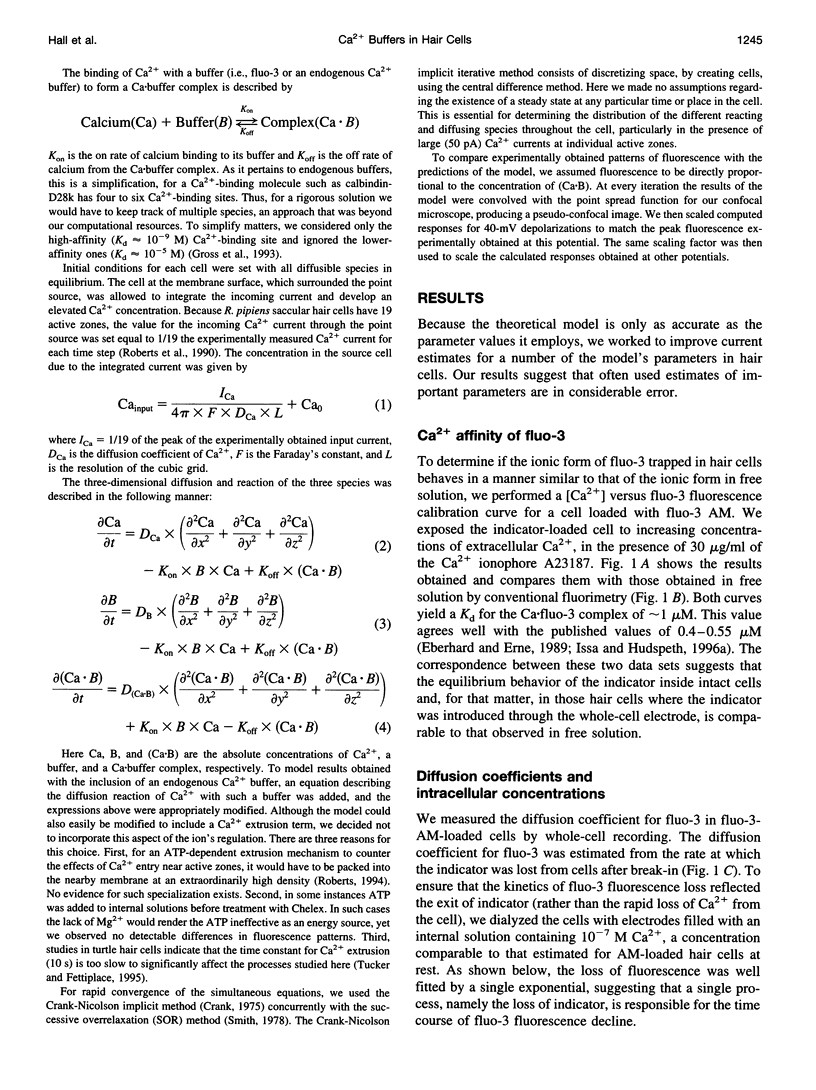
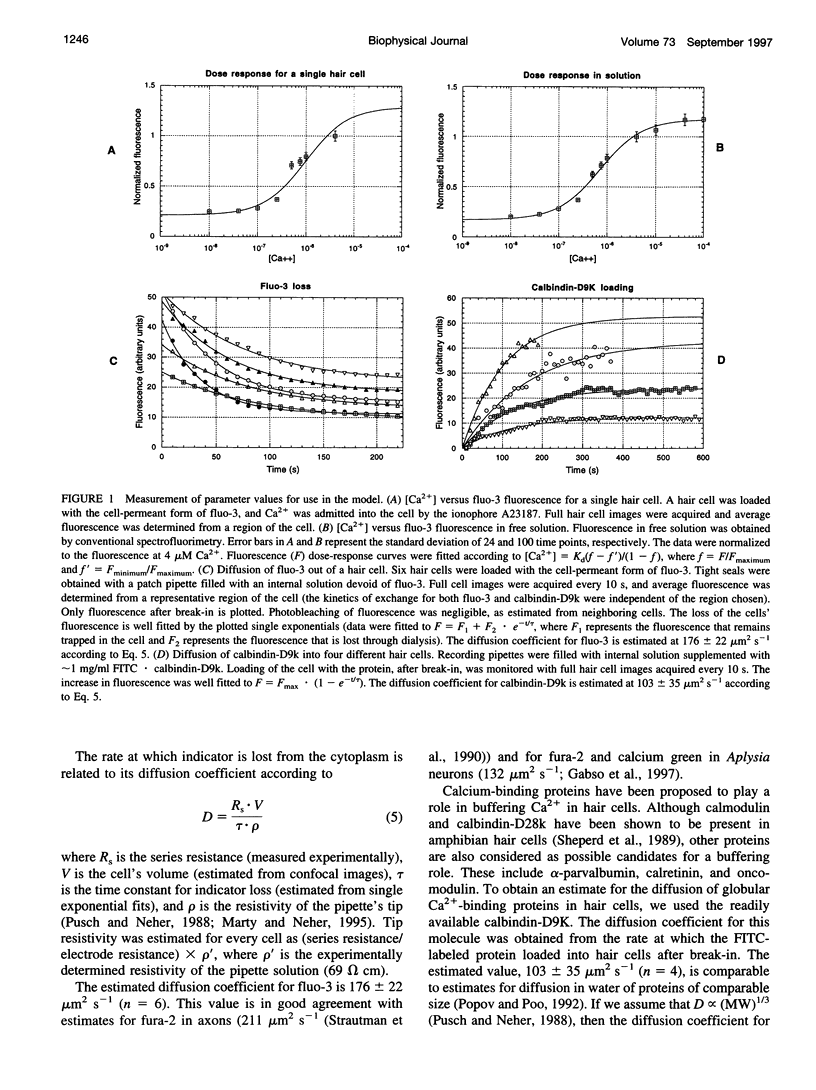
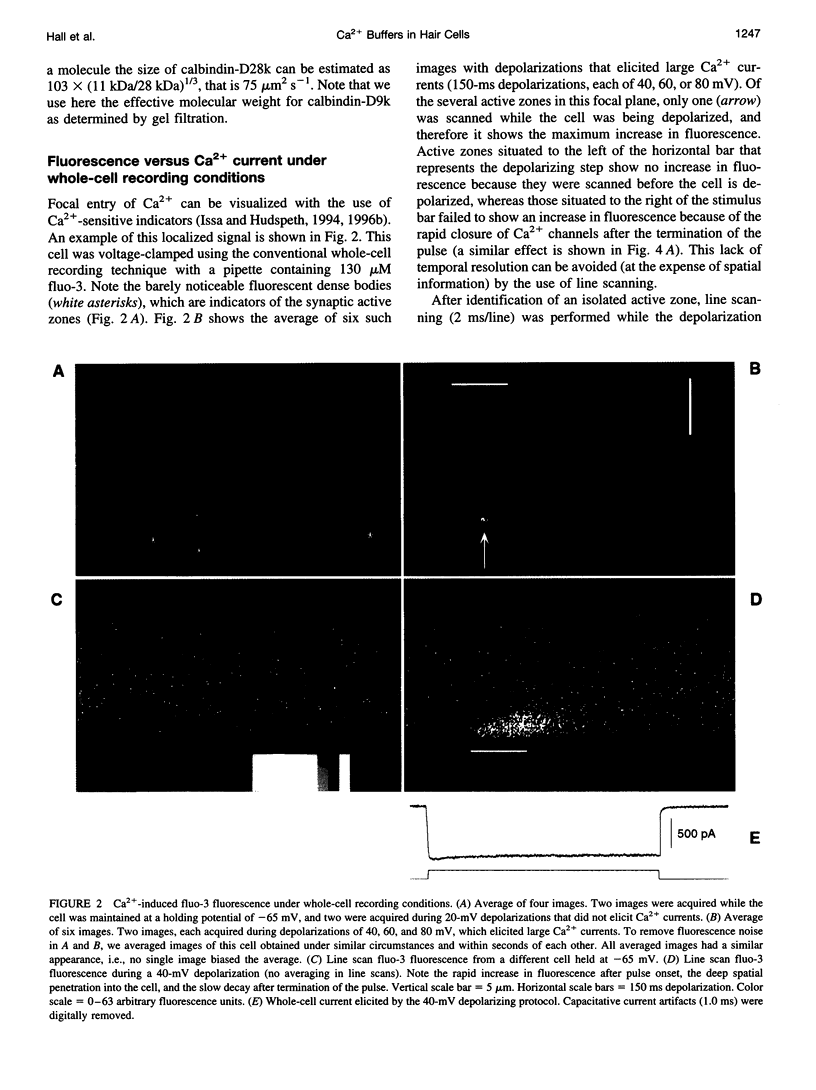
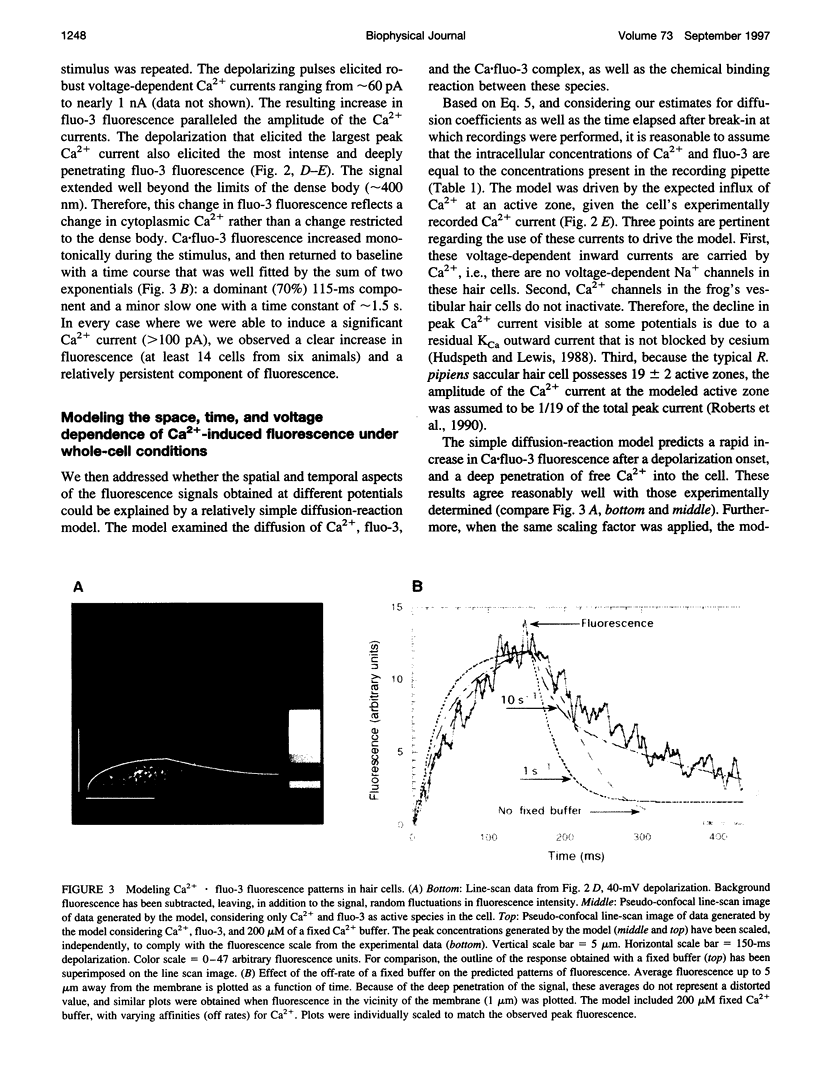
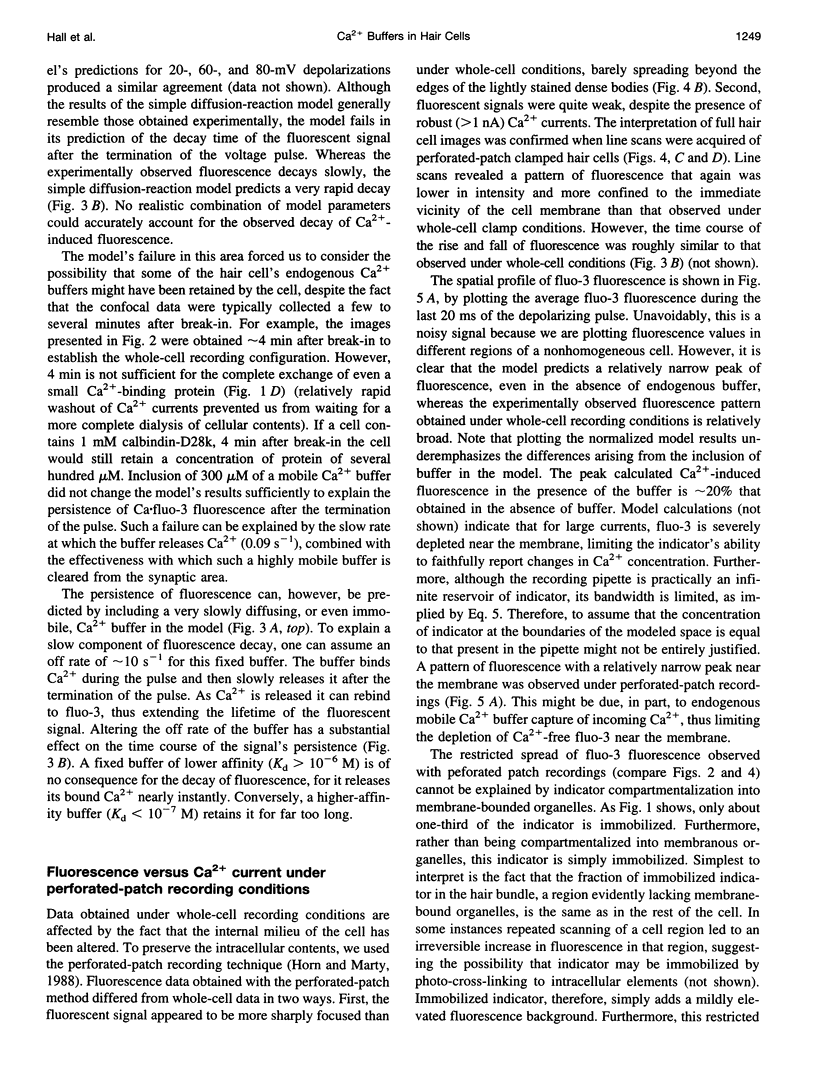
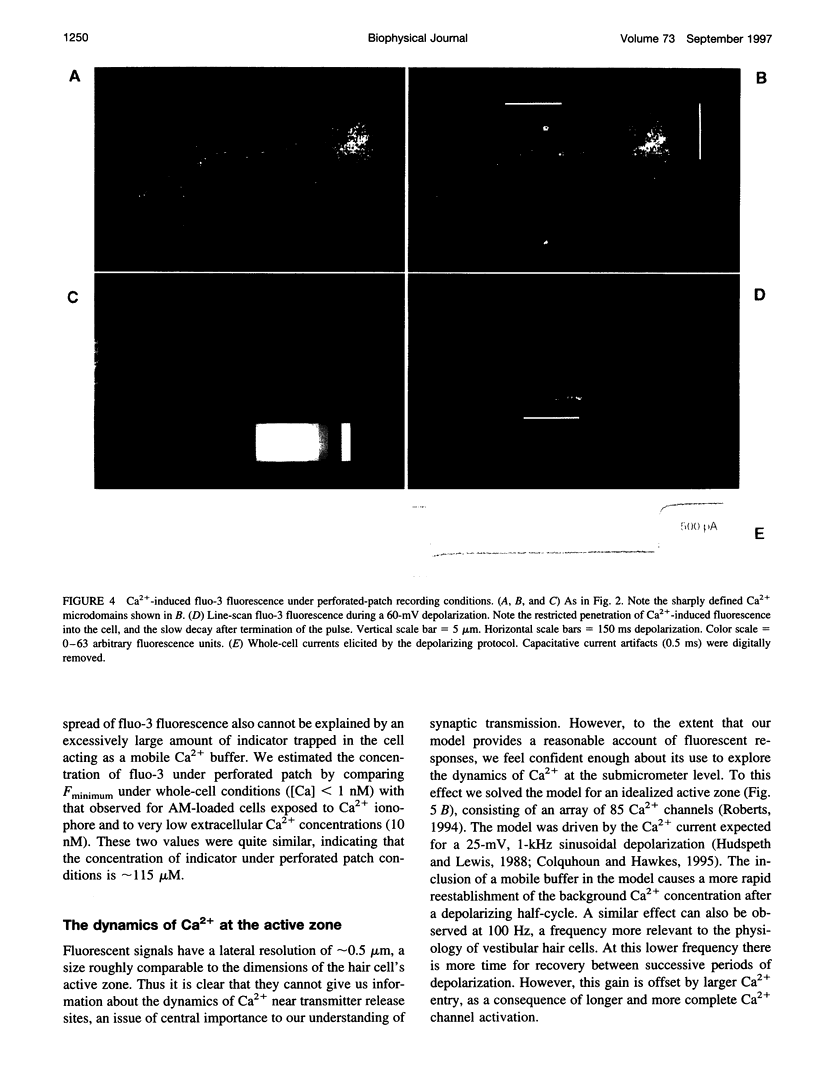
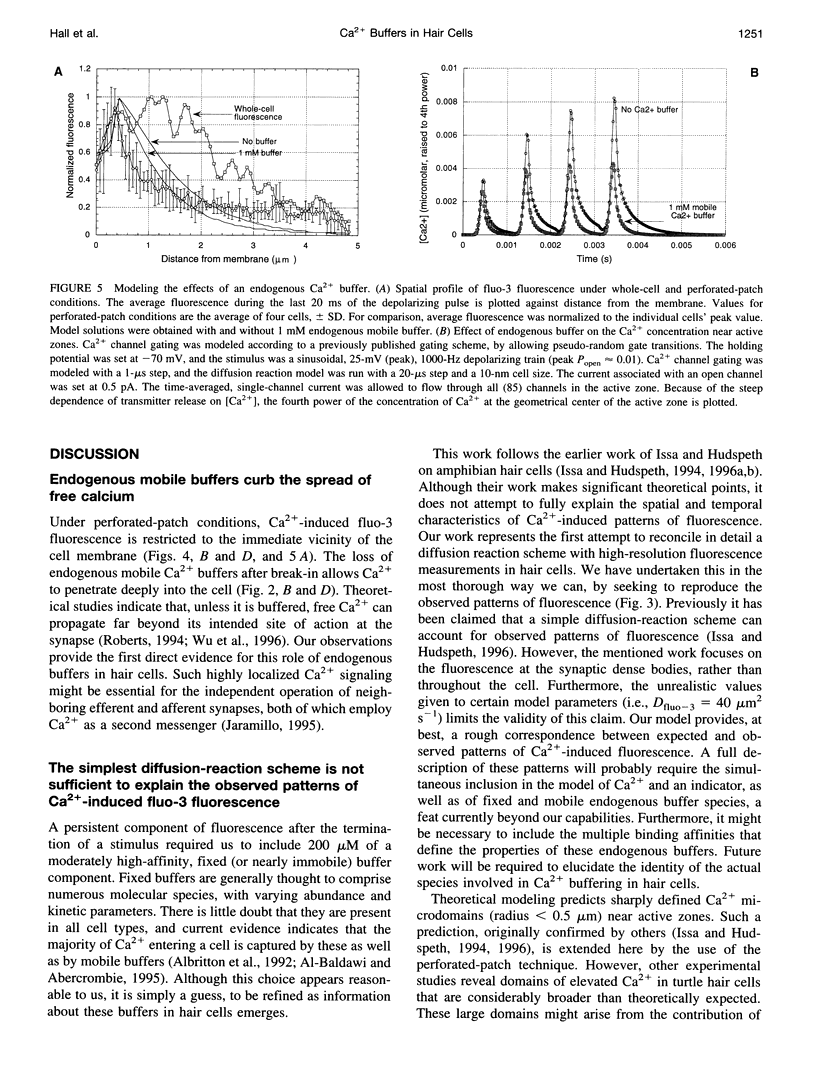
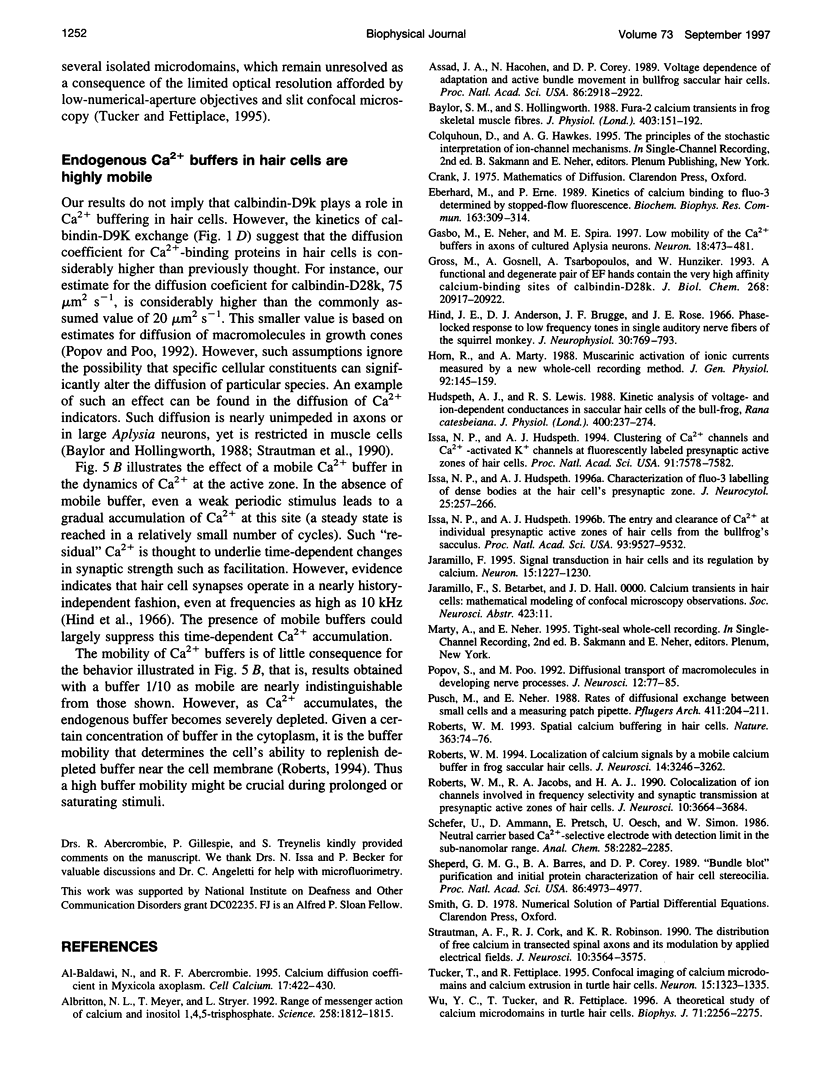
Images in this article
Selected References
These references are in PubMed. This may not be the complete list of references from this article.
- Allbritton N. L., Meyer T., Stryer L. Range of messenger action of calcium ion and inositol 1,4,5-trisphosphate. Science. 1992 Dec 11;258(5089):1812–1815. doi: 10.1126/science.1465619. [DOI] [PubMed] [Google Scholar]
- Assad J. A., Hacohen N., Corey D. P. Voltage dependence of adaptation and active bundle movement in bullfrog saccular hair cells. Proc Natl Acad Sci U S A. 1989 Apr;86(8):2918–2922. doi: 10.1073/pnas.86.8.2918. [DOI] [PMC free article] [PubMed] [Google Scholar]
- Baylor S. M., Hollingworth S. Fura-2 calcium transients in frog skeletal muscle fibres. J Physiol. 1988 Sep;403:151–192. doi: 10.1113/jphysiol.1988.sp017244. [DOI] [PMC free article] [PubMed] [Google Scholar]
- Eberhard M., Erne P. Kinetics of calcium binding to fluo-3 determined by stopped-flow fluorescence. Biochem Biophys Res Commun. 1989 Aug 30;163(1):309–314. doi: 10.1016/0006-291x(89)92136-0. [DOI] [PubMed] [Google Scholar]
- Gabso M., Neher E., Spira M. E. Low mobility of the Ca2+ buffers in axons of cultured Aplysia neurons. Neuron. 1997 Mar;18(3):473–481. doi: 10.1016/s0896-6273(00)81247-7. [DOI] [PubMed] [Google Scholar]
- Gross M. D., Gosnell M., Tsarbopoulos A., Hunziker W. A functional and degenerate pair of EF hands contains the very high affinity calcium-binding site of calbindin-D28K. J Biol Chem. 1993 Oct 5;268(28):20917–20922. [PubMed] [Google Scholar]
- Horn R., Marty A. Muscarinic activation of ionic currents measured by a new whole-cell recording method. J Gen Physiol. 1988 Aug;92(2):145–159. doi: 10.1085/jgp.92.2.145. [DOI] [PMC free article] [PubMed] [Google Scholar]
- Hudspeth A. J., Lewis R. S. Kinetic analysis of voltage- and ion-dependent conductances in saccular hair cells of the bull-frog, Rana catesbeiana. J Physiol. 1988 Jun;400:237–274. doi: 10.1113/jphysiol.1988.sp017119. [DOI] [PMC free article] [PubMed] [Google Scholar]
- Issa N. P., Hudspeth A. J. Characterization of fluo-3 labelling of dense bodies at the hair cell's presynaptic active zone. J Neurocytol. 1996 Apr;25(4):257–266. doi: 10.1007/BF02284801. [DOI] [PubMed] [Google Scholar]
- Issa N. P., Hudspeth A. J. Clustering of Ca2+ channels and Ca(2+)-activated K+ channels at fluorescently labeled presynaptic active zones of hair cells. Proc Natl Acad Sci U S A. 1994 Aug 2;91(16):7578–7582. doi: 10.1073/pnas.91.16.7578. [DOI] [PMC free article] [PubMed] [Google Scholar]
- Issa N. P., Hudspeth A. J. The entry and clearance of Ca2+ at individual presynaptic active zones of hair cells from the bullfrog's sacculus. Proc Natl Acad Sci U S A. 1996 Sep 3;93(18):9527–9532. doi: 10.1073/pnas.93.18.9527. [DOI] [PMC free article] [PubMed] [Google Scholar]
- Jaramillo F. Signal transduction in hair cells and its regulation by calcium. Neuron. 1995 Dec;15(6):1227–1230. doi: 10.1016/0896-6273(95)90003-9. [DOI] [PubMed] [Google Scholar]
- Popov S., Poo M. M. Diffusional transport of macromolecules in developing nerve processes. J Neurosci. 1992 Jan;12(1):77–85. doi: 10.1523/JNEUROSCI.12-01-00077.1992. [DOI] [PMC free article] [PubMed] [Google Scholar]
- Pusch M., Neher E. Rates of diffusional exchange between small cells and a measuring patch pipette. Pflugers Arch. 1988 Feb;411(2):204–211. doi: 10.1007/BF00582316. [DOI] [PubMed] [Google Scholar]
- Roberts W. M., Jacobs R. A., Hudspeth A. J. Colocalization of ion channels involved in frequency selectivity and synaptic transmission at presynaptic active zones of hair cells. J Neurosci. 1990 Nov;10(11):3664–3684. doi: 10.1523/JNEUROSCI.10-11-03664.1990. [DOI] [PMC free article] [PubMed] [Google Scholar]
- Roberts W. M. Localization of calcium signals by a mobile calcium buffer in frog saccular hair cells. J Neurosci. 1994 May;14(5 Pt 2):3246–3262. doi: 10.1523/JNEUROSCI.14-05-03246.1994. [DOI] [PMC free article] [PubMed] [Google Scholar]
- Roberts W. M. Spatial calcium buffering in saccular hair cells. Nature. 1993 May 6;363(6424):74–76. doi: 10.1038/363074a0. [DOI] [PubMed] [Google Scholar]
- Rose J. E., Brugge J. F., Anderson D. J., Hind J. E. Phase-locked response to low-frequency tones in single auditory nerve fibers of the squirrel monkey. J Neurophysiol. 1967 Jul;30(4):769–793. doi: 10.1152/jn.1967.30.4.769. [DOI] [PubMed] [Google Scholar]
- Shepherd G. M., Barres B. A., Corey D. P. "Bundle blot" purification and initial protein characterization of hair cell stereocilia. Proc Natl Acad Sci U S A. 1989 Jul;86(13):4973–4977. doi: 10.1073/pnas.86.13.4973. [DOI] [PMC free article] [PubMed] [Google Scholar]
- Strautman A. F., Cork R. J., Robinson K. R. The distribution of free calcium in transected spinal axons and its modulation by applied electrical fields. J Neurosci. 1990 Nov;10(11):3564–3575. doi: 10.1523/JNEUROSCI.10-11-03564.1990. [DOI] [PMC free article] [PubMed] [Google Scholar]
- Tucker T., Fettiplace R. Confocal imaging of calcium microdomains and calcium extrusion in turtle hair cells. Neuron. 1995 Dec;15(6):1323–1335. doi: 10.1016/0896-6273(95)90011-x. [DOI] [PubMed] [Google Scholar]
- Wu Y. C., Tucker T., Fettiplace R. A theoretical study of calcium microdomains in turtle hair cells. Biophys J. 1996 Nov;71(5):2256–2275. doi: 10.1016/S0006-3495(96)79429-8. [DOI] [PMC free article] [PubMed] [Google Scholar]
- al-Baldawi N. F., Abercrombie R. F. Calcium diffusion coefficient in Myxicola axoplasm. Cell Calcium. 1995 Jun;17(6):422–430. doi: 10.1016/0143-4160(95)90088-8. [DOI] [PubMed] [Google Scholar]