Abstract
We previously applied the Poisson-Boltzmann equation to atomic models of phospholipid bilayers and basic peptides to calculate their electrostatic interactions from first principles (Ben-Tal, N., B. Honig, R. M. Peitzsch, G. Denisov, and S. McLaughlan. 1996. Binding of small basic peptides to membranes containing acidic lipids. Theoretical models and experimental results. Biophys. J. 71:561-575). Specifically, we calculated the molar partition coefficient, K (the reciprocal of the lipid concentration at which 1/2 the peptide is bound), of simple basic peptides (e.g., pentalysine) with phospholipid vesicles. The theoretical predictions agreed well with experimental measurements of the binding, but the agreement could have been fortuitous because the structure(s) of these flexible peptides is not known. Here we use the same theoretical approach to calculate the membrane binding of two small proteins of known structure: charybdotoxin (CTx) and iberiotoxin (IbTx); we also measure the binding of these proteins to phospholipid vesicles. The theoretical model describes accurately the dependence of K on the ionic strength and mol % acidic lipid in the membrane for both CTx (net charge +4) and IbTx (net charge +2). For example, the theory correctly predicts that the value of K for the binding of CTx to a membrane containing 33% acidic lipid should decrease by a factor of 10(5) when the salt concentration increases from 10 to 200 mM. We discuss the limitations of the theoretical approach and also consider a simple extension of the theory that incorporates nonpolar interactions.
Full text
PDF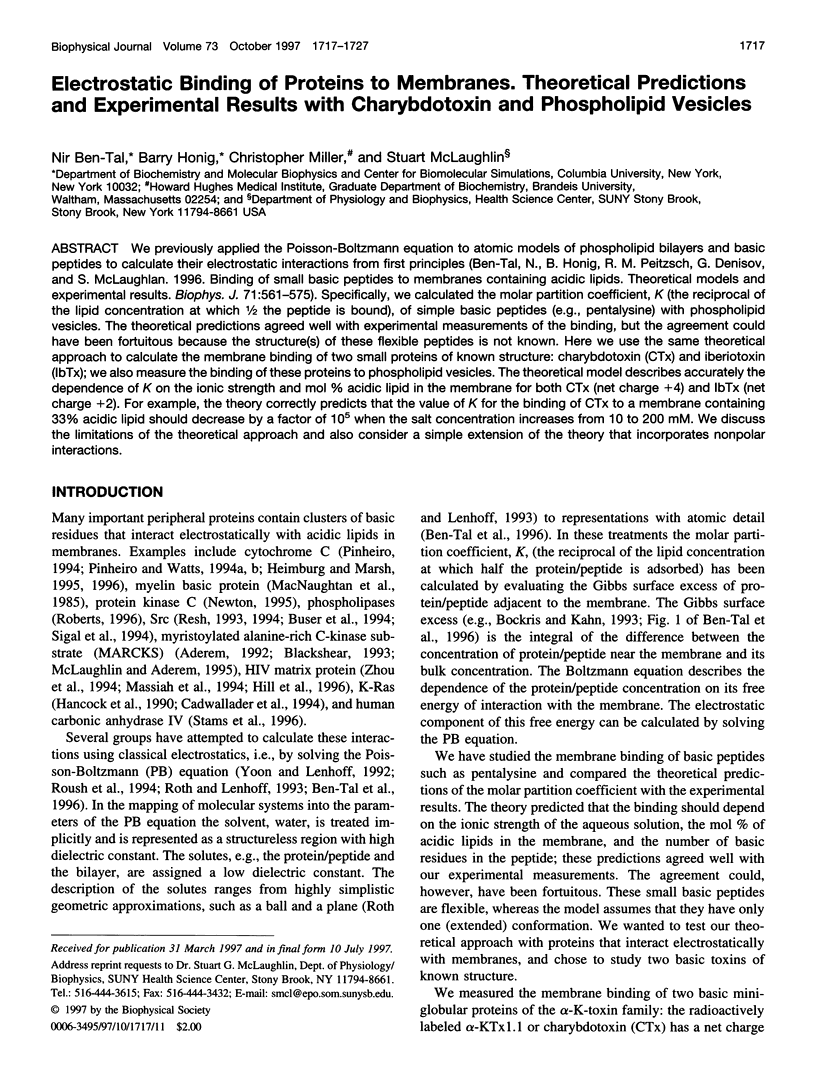
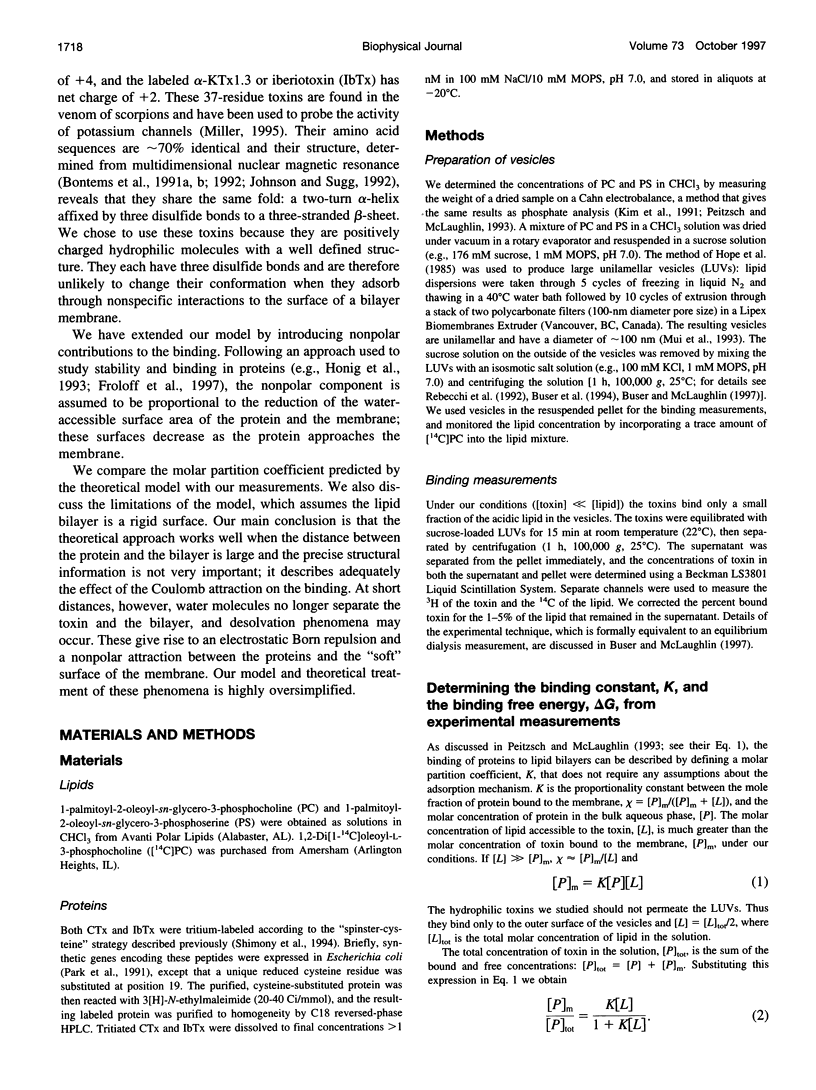
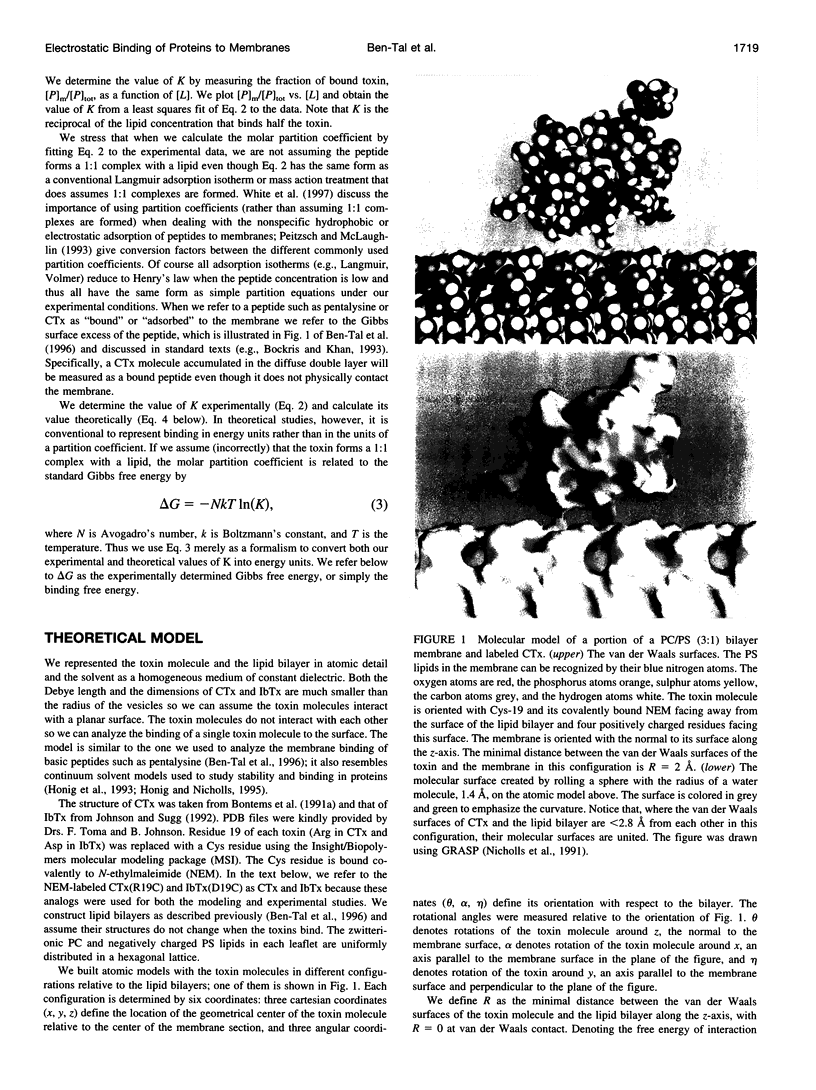
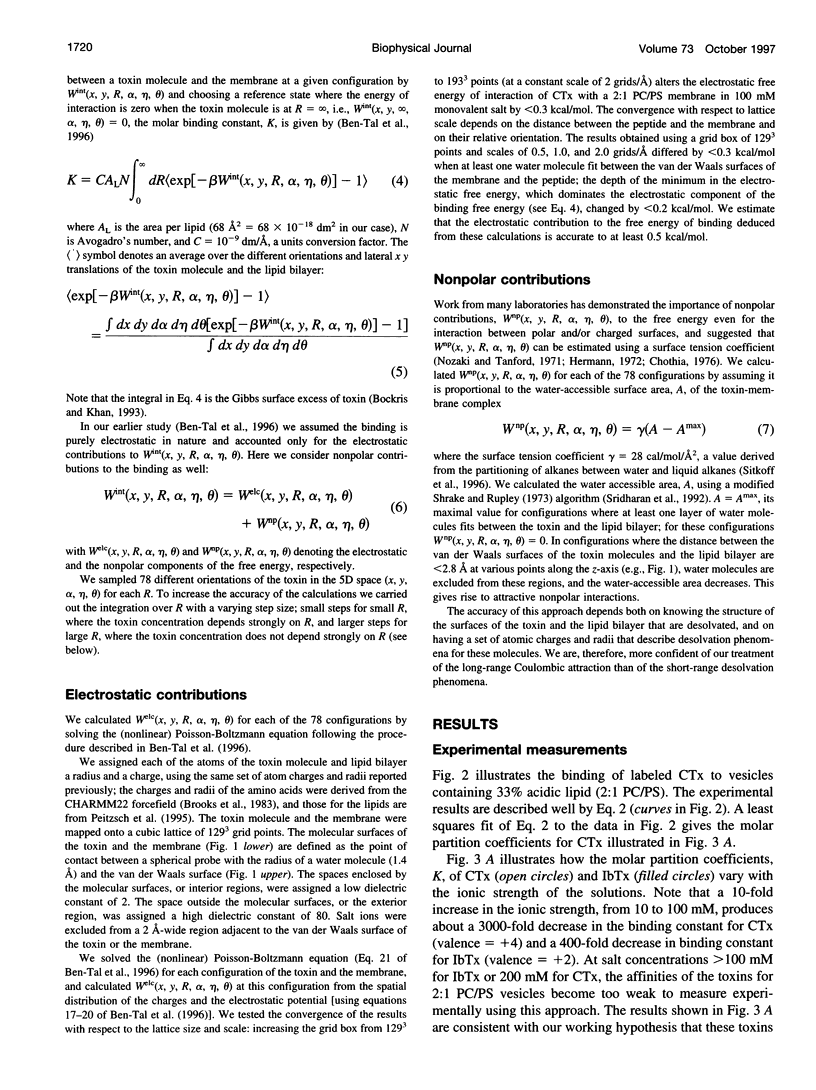
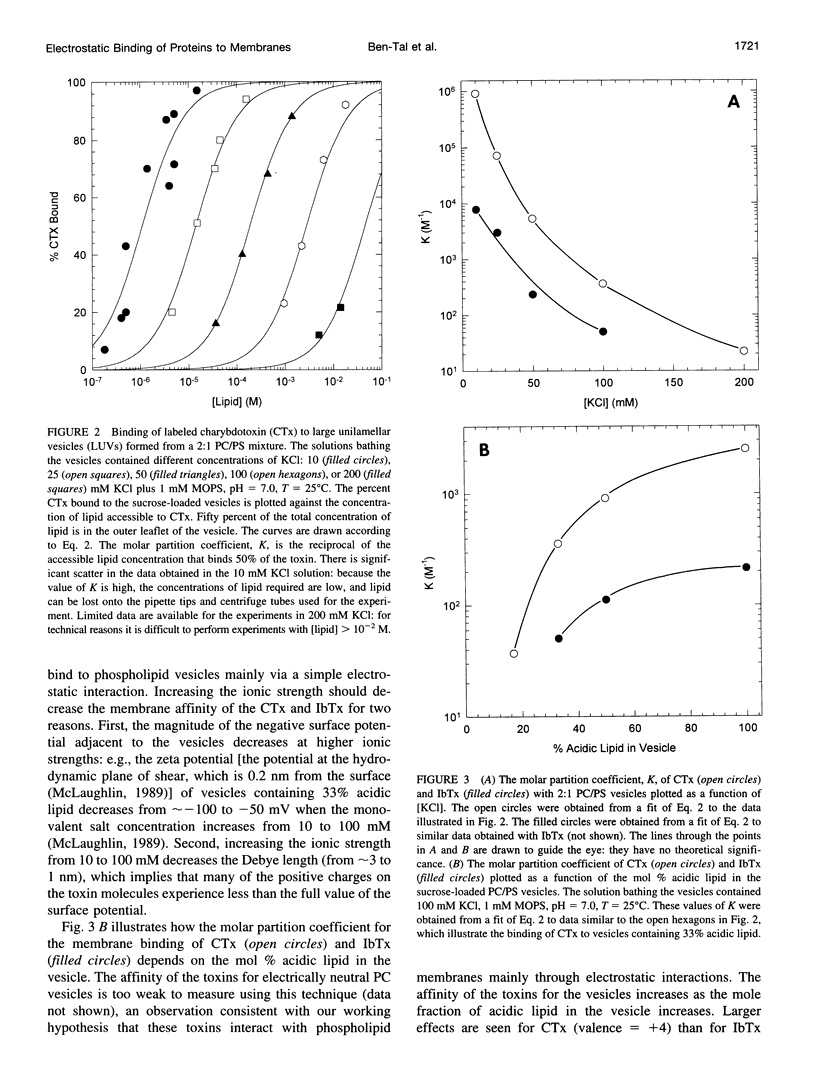
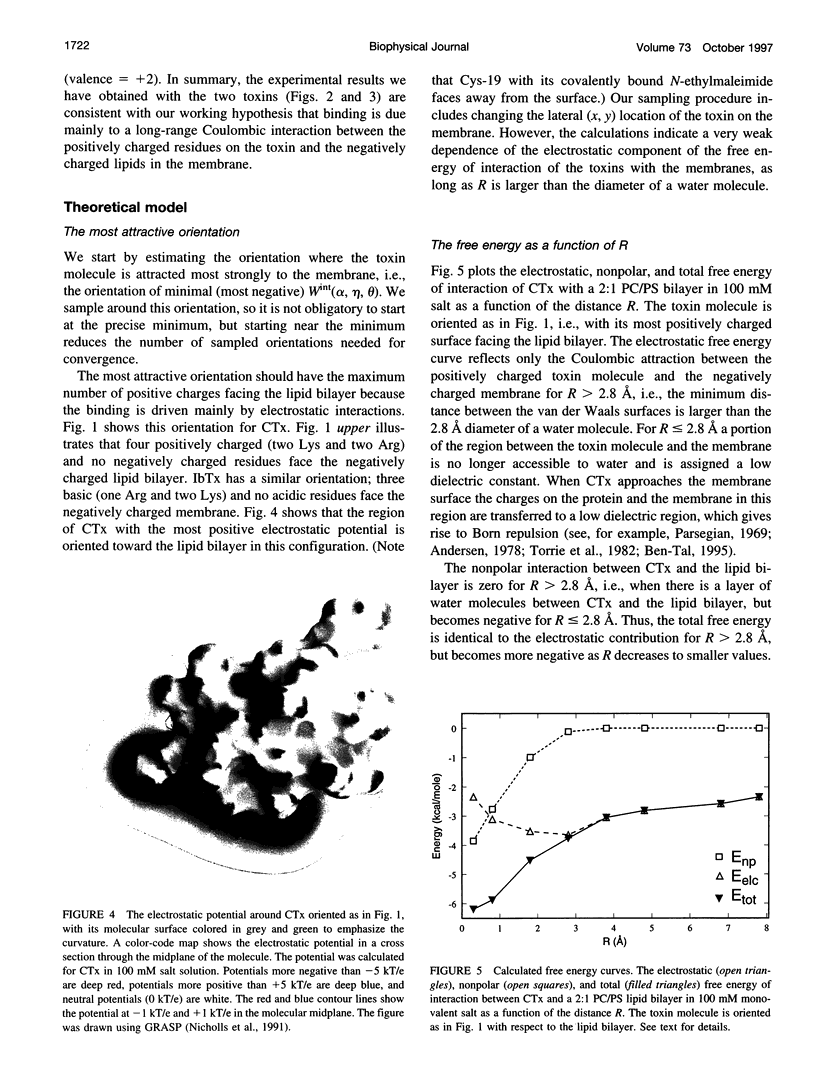
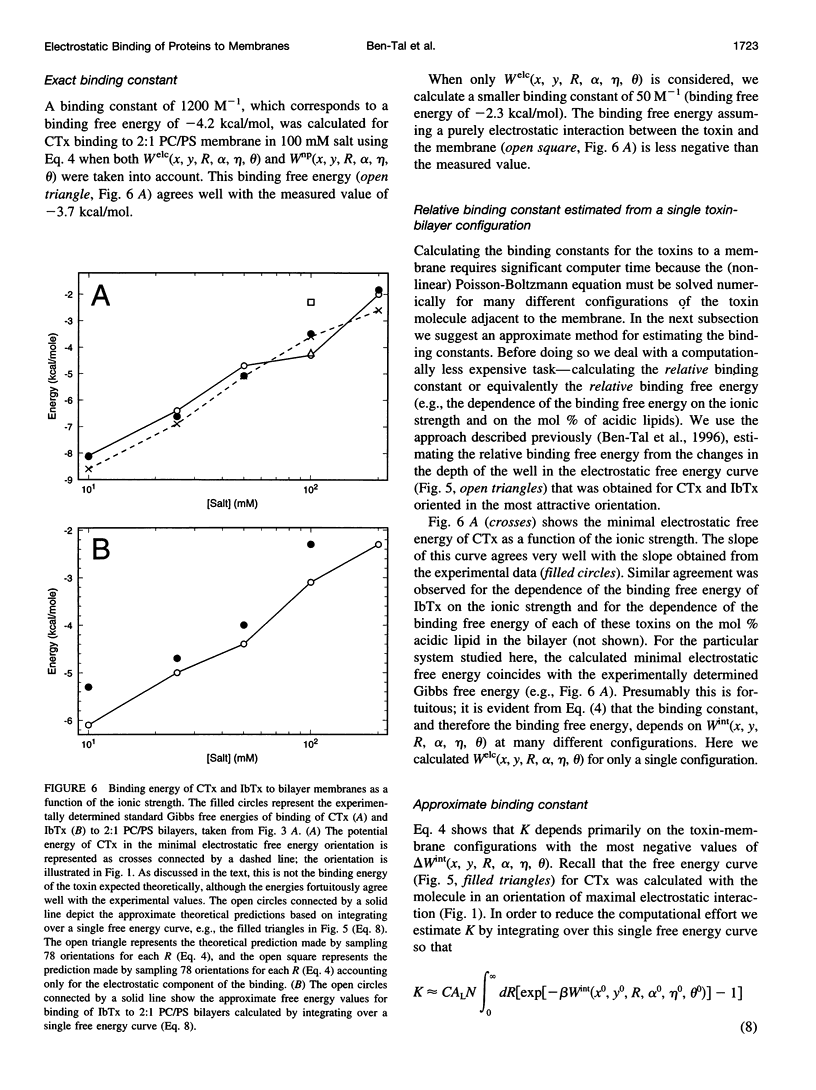
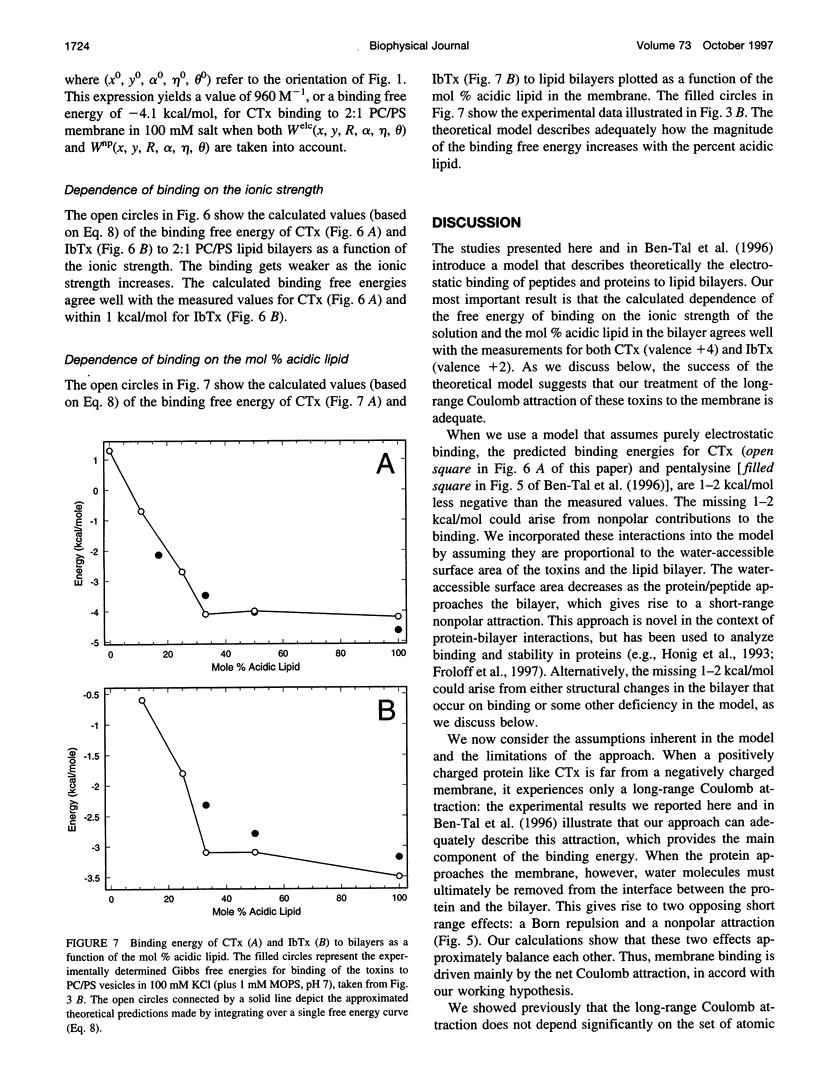
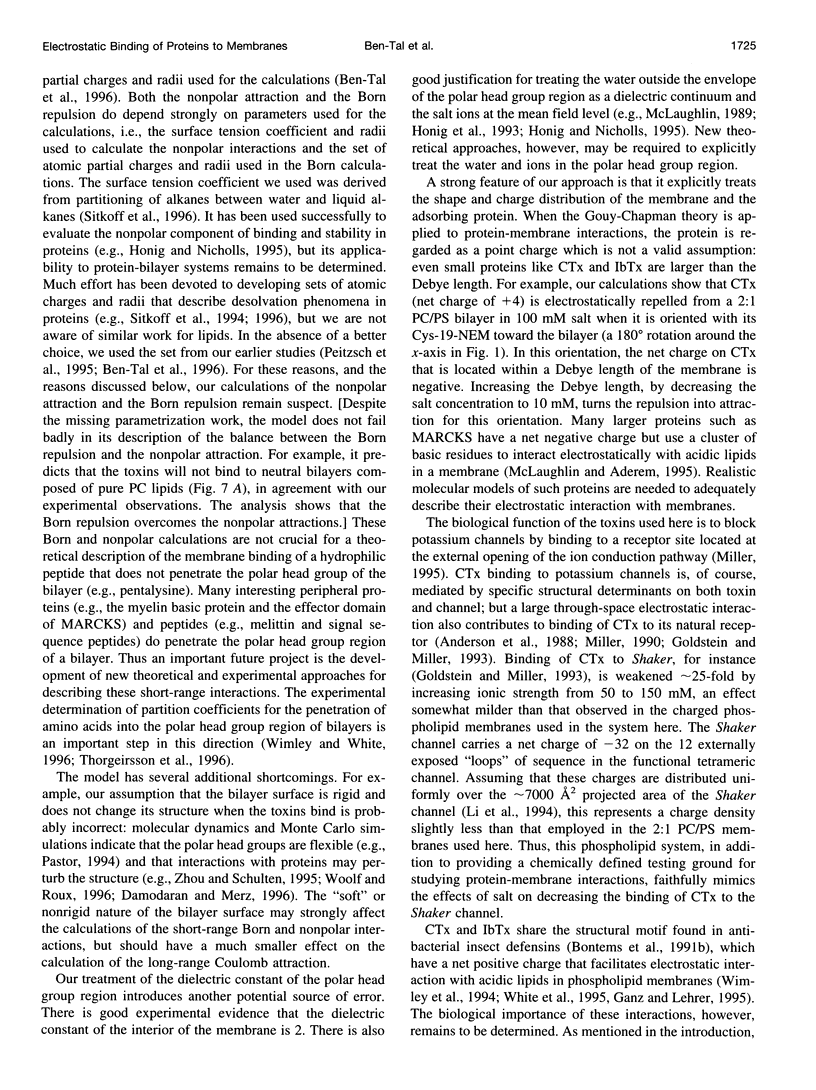
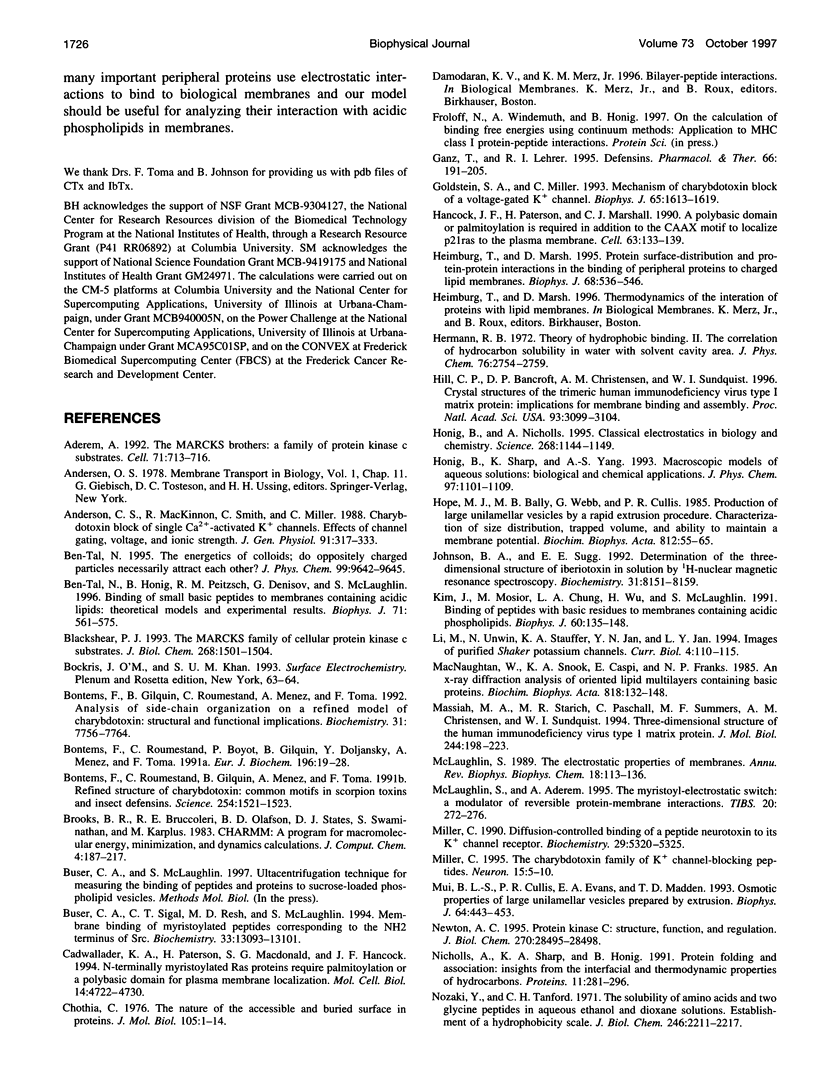
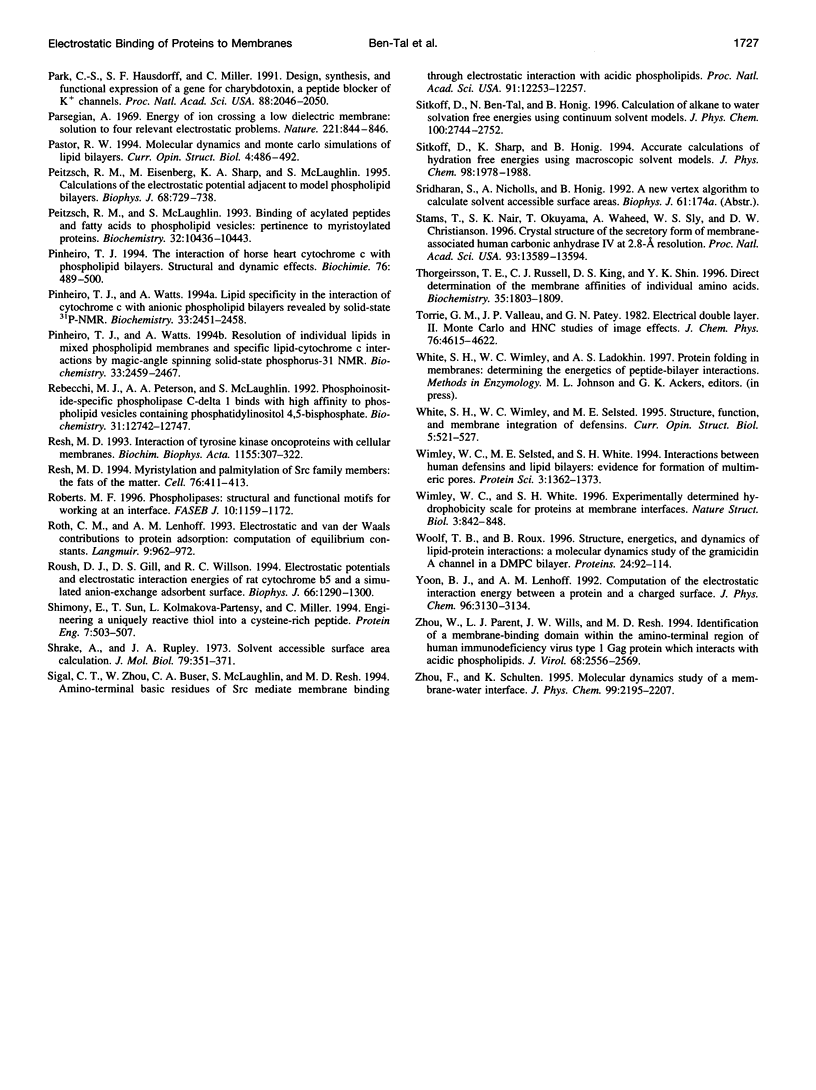
Images in this article
Selected References
These references are in PubMed. This may not be the complete list of references from this article.
- Aderem A. The MARCKS brothers: a family of protein kinase C substrates. Cell. 1992 Nov 27;71(5):713–716. doi: 10.1016/0092-8674(92)90546-o. [DOI] [PubMed] [Google Scholar]
- Anderson C. S., MacKinnon R., Smith C., Miller C. Charybdotoxin block of single Ca2+-activated K+ channels. Effects of channel gating, voltage, and ionic strength. J Gen Physiol. 1988 Mar;91(3):317–333. doi: 10.1085/jgp.91.3.317. [DOI] [PMC free article] [PubMed] [Google Scholar]
- Ben-Tal N., Honig B., Peitzsch R. M., Denisov G., McLaughlin S. Binding of small basic peptides to membranes containing acidic lipids: theoretical models and experimental results. Biophys J. 1996 Aug;71(2):561–575. doi: 10.1016/S0006-3495(96)79280-9. [DOI] [PMC free article] [PubMed] [Google Scholar]
- Blackshear P. J. The MARCKS family of cellular protein kinase C substrates. J Biol Chem. 1993 Jan 25;268(3):1501–1504. [PubMed] [Google Scholar]
- Bontems F., Gilquin B., Roumestand C., Ménez A., Toma F. Analysis of side-chain organization on a refined model of charybdotoxin: structural and functional implications. Biochemistry. 1992 Sep 1;31(34):7756–7764. doi: 10.1021/bi00149a003. [DOI] [PubMed] [Google Scholar]
- Bontems F., Roumestand C., Boyot P., Gilquin B., Doljansky Y., Menez A., Toma F. Three-dimensional structure of natural charybdotoxin in aqueous solution by 1H-NMR. Charybdotoxin possesses a structural motif found in other scorpion toxins. Eur J Biochem. 1991 Feb 26;196(1):19–28. doi: 10.1111/j.1432-1033.1991.tb15780.x. [DOI] [PubMed] [Google Scholar]
- Bontems F., Roumestand C., Gilquin B., Ménez A., Toma F. Refined structure of charybdotoxin: common motifs in scorpion toxins and insect defensins. Science. 1991 Dec 6;254(5037):1521–1523. doi: 10.1126/science.1720574. [DOI] [PubMed] [Google Scholar]
- Buser C. A., Sigal C. T., Resh M. D., McLaughlin S. Membrane binding of myristylated peptides corresponding to the NH2 terminus of Src. Biochemistry. 1994 Nov 8;33(44):13093–13101. doi: 10.1021/bi00248a019. [DOI] [PubMed] [Google Scholar]
- Cadwallader K. A., Paterson H., Macdonald S. G., Hancock J. F. N-terminally myristoylated Ras proteins require palmitoylation or a polybasic domain for plasma membrane localization. Mol Cell Biol. 1994 Jul;14(7):4722–4730. doi: 10.1128/mcb.14.7.4722. [DOI] [PMC free article] [PubMed] [Google Scholar]
- Chothia C. The nature of the accessible and buried surfaces in proteins. J Mol Biol. 1976 Jul 25;105(1):1–12. doi: 10.1016/0022-2836(76)90191-1. [DOI] [PubMed] [Google Scholar]
- Ganz T., Lehrer R. I. Defensins. Pharmacol Ther. 1995 May;66(2):191–205. doi: 10.1016/0163-7258(94)00076-f. [DOI] [PubMed] [Google Scholar]
- Goldstein S. A., Miller C. Mechanism of charybdotoxin block of a voltage-gated K+ channel. Biophys J. 1993 Oct;65(4):1613–1619. doi: 10.1016/S0006-3495(93)81200-1. [DOI] [PMC free article] [PubMed] [Google Scholar]
- Hancock J. F., Paterson H., Marshall C. J. A polybasic domain or palmitoylation is required in addition to the CAAX motif to localize p21ras to the plasma membrane. Cell. 1990 Oct 5;63(1):133–139. doi: 10.1016/0092-8674(90)90294-o. [DOI] [PubMed] [Google Scholar]
- Heimburg T., Marsh D. Protein surface-distribution and protein-protein interactions in the binding of peripheral proteins to charged lipid membranes. Biophys J. 1995 Feb;68(2):536–546. doi: 10.1016/S0006-3495(95)80215-8. [DOI] [PMC free article] [PubMed] [Google Scholar]
- Hill C. P., Worthylake D., Bancroft D. P., Christensen A. M., Sundquist W. I. Crystal structures of the trimeric human immunodeficiency virus type 1 matrix protein: implications for membrane association and assembly. Proc Natl Acad Sci U S A. 1996 Apr 2;93(7):3099–3104. doi: 10.1073/pnas.93.7.3099. [DOI] [PMC free article] [PubMed] [Google Scholar]
- Honig B., Nicholls A. Classical electrostatics in biology and chemistry. Science. 1995 May 26;268(5214):1144–1149. doi: 10.1126/science.7761829. [DOI] [PubMed] [Google Scholar]
- Johnson B. A., Sugg E. E. Determination of the three-dimensional structure of iberiotoxin in solution by 1H nuclear magnetic resonance spectroscopy. Biochemistry. 1992 Sep 8;31(35):8151–8159. doi: 10.1021/bi00150a006. [DOI] [PubMed] [Google Scholar]
- Kim J., Mosior M., Chung L. A., Wu H., McLaughlin S. Binding of peptides with basic residues to membranes containing acidic phospholipids. Biophys J. 1991 Jul;60(1):135–148. doi: 10.1016/S0006-3495(91)82037-9. [DOI] [PMC free article] [PubMed] [Google Scholar]
- Li M., Unwin N., Stauffer K. A., Jan Y. N., Jan L. Y. Images of purified Shaker potassium channels. Curr Biol. 1994 Feb 1;4(2):110–115. doi: 10.1016/s0960-9822(94)00026-6. [DOI] [PubMed] [Google Scholar]
- MacNaughtan W., Snook K. A., Caspi E., Franks N. P. An X-ray diffraction analysis of oriented lipid multilayers containing basic proteins. Biochim Biophys Acta. 1985 Aug 27;818(2):132–148. doi: 10.1016/0005-2736(85)90556-5. [DOI] [PubMed] [Google Scholar]
- Massiah M. A., Starich M. R., Paschall C., Summers M. F., Christensen A. M., Sundquist W. I. Three-dimensional structure of the human immunodeficiency virus type 1 matrix protein. J Mol Biol. 1994 Nov 25;244(2):198–223. doi: 10.1006/jmbi.1994.1719. [DOI] [PubMed] [Google Scholar]
- McLaughlin S., Aderem A. The myristoyl-electrostatic switch: a modulator of reversible protein-membrane interactions. Trends Biochem Sci. 1995 Jul;20(7):272–276. doi: 10.1016/s0968-0004(00)89042-8. [DOI] [PubMed] [Google Scholar]
- McLaughlin S. The electrostatic properties of membranes. Annu Rev Biophys Biophys Chem. 1989;18:113–136. doi: 10.1146/annurev.bb.18.060189.000553. [DOI] [PubMed] [Google Scholar]
- Miller C. Diffusion-controlled binding of a peptide neurotoxin to its K+ channel receptor. Biochemistry. 1990 Jun 5;29(22):5320–5325. doi: 10.1021/bi00474a016. [DOI] [PubMed] [Google Scholar]
- Miller C. The charybdotoxin family of K+ channel-blocking peptides. Neuron. 1995 Jul;15(1):5–10. doi: 10.1016/0896-6273(95)90057-8. [DOI] [PubMed] [Google Scholar]
- Mui B. L., Cullis P. R., Evans E. A., Madden T. D. Osmotic properties of large unilamellar vesicles prepared by extrusion. Biophys J. 1993 Feb;64(2):443–453. doi: 10.1016/S0006-3495(93)81385-7. [DOI] [PMC free article] [PubMed] [Google Scholar]
- Newton A. C. Protein kinase C: structure, function, and regulation. J Biol Chem. 1995 Dec 1;270(48):28495–28498. doi: 10.1074/jbc.270.48.28495. [DOI] [PubMed] [Google Scholar]
- Nicholls A., Sharp K. A., Honig B. Protein folding and association: insights from the interfacial and thermodynamic properties of hydrocarbons. Proteins. 1991;11(4):281–296. doi: 10.1002/prot.340110407. [DOI] [PubMed] [Google Scholar]
- Nozaki Y., Tanford C. The solubility of amino acids and two glycine peptides in aqueous ethanol and dioxane solutions. Establishment of a hydrophobicity scale. J Biol Chem. 1971 Apr 10;246(7):2211–2217. [PubMed] [Google Scholar]
- Park C. S., Hausdorff S. F., Miller C. Design, synthesis, and functional expression of a gene for charybdotoxin, a peptide blocker of K+ channels. Proc Natl Acad Sci U S A. 1991 Mar 15;88(6):2046–2050. doi: 10.1073/pnas.88.6.2046. [DOI] [PMC free article] [PubMed] [Google Scholar]
- Parsegian A. Energy of an ion crossing a low dielectric membrane: solutions to four relevant electrostatic problems. Nature. 1969 Mar 1;221(5183):844–846. doi: 10.1038/221844a0. [DOI] [PubMed] [Google Scholar]
- Peitzsch R. M., Eisenberg M., Sharp K. A., McLaughlin S. Calculations of the electrostatic potential adjacent to model phospholipid bilayers. Biophys J. 1995 Mar;68(3):729–738. doi: 10.1016/S0006-3495(95)80253-5. [DOI] [PMC free article] [PubMed] [Google Scholar]
- Peitzsch R. M., McLaughlin S. Binding of acylated peptides and fatty acids to phospholipid vesicles: pertinence to myristoylated proteins. Biochemistry. 1993 Oct 5;32(39):10436–10443. doi: 10.1021/bi00090a020. [DOI] [PubMed] [Google Scholar]
- Pinheiro T. J. The interaction of horse heart cytochrome c with phospholipid bilayers. Structural and dynamic effects. Biochimie. 1994;76(6):489–500. doi: 10.1016/0300-9084(94)90173-2. [DOI] [PubMed] [Google Scholar]
- Pinheiro T. J., Watts A. Lipid specificity in the interaction of cytochrome c with anionic phospholipid bilayers revealed by solid-state 31P NMR. Biochemistry. 1994 Mar 8;33(9):2451–2458. doi: 10.1021/bi00175a013. [DOI] [PubMed] [Google Scholar]
- Pinheiro T. J., Watts A. Resolution of individual lipids in mixed phospholipid membranes and specific lipid-cytochrome c interactions by magic-angle spinning solid-state phosphorus-31 NMR. Biochemistry. 1994 Mar 8;33(9):2459–2467. doi: 10.1021/bi00175a014. [DOI] [PubMed] [Google Scholar]
- Rebecchi M., Peterson A., McLaughlin S. Phosphoinositide-specific phospholipase C-delta 1 binds with high affinity to phospholipid vesicles containing phosphatidylinositol 4,5-bisphosphate. Biochemistry. 1992 Dec 29;31(51):12742–12747. doi: 10.1021/bi00166a005. [DOI] [PubMed] [Google Scholar]
- Resh M. D. Interaction of tyrosine kinase oncoproteins with cellular membranes. Biochim Biophys Acta. 1993 Dec 23;1155(3):307–322. doi: 10.1016/0304-419x(93)90012-2. [DOI] [PubMed] [Google Scholar]
- Resh M. D. Myristylation and palmitylation of Src family members: the fats of the matter. Cell. 1994 Feb 11;76(3):411–413. doi: 10.1016/0092-8674(94)90104-x. [DOI] [PubMed] [Google Scholar]
- Roberts M. F. Phospholipases: structural and functional motifs for working at an interface. FASEB J. 1996 Aug;10(10):1159–1172. doi: 10.1096/fasebj.10.10.8751718. [DOI] [PubMed] [Google Scholar]
- Roush D. J., Gill D. S., Willson R. C. Electrostatic potentials and electrostatic interaction energies of rat cytochrome b5 and a simulated anion-exchange adsorbent surface. Biophys J. 1994 May;66(5):1290–1300. doi: 10.1016/S0006-3495(94)80924-5. [DOI] [PMC free article] [PubMed] [Google Scholar]
- Shimony E., Sun T., Kolmakova-Partensky L., Miller C. Engineering a uniquely reactive thiol into a cysteine-rich peptide. Protein Eng. 1994 Apr;7(4):503–507. doi: 10.1093/protein/7.4.503. [DOI] [PubMed] [Google Scholar]
- Shrake A., Rupley J. A. Environment and exposure to solvent of protein atoms. Lysozyme and insulin. J Mol Biol. 1973 Sep 15;79(2):351–371. doi: 10.1016/0022-2836(73)90011-9. [DOI] [PubMed] [Google Scholar]
- Sigal C. T., Zhou W., Buser C. A., McLaughlin S., Resh M. D. Amino-terminal basic residues of Src mediate membrane binding through electrostatic interaction with acidic phospholipids. Proc Natl Acad Sci U S A. 1994 Dec 6;91(25):12253–12257. doi: 10.1073/pnas.91.25.12253. [DOI] [PMC free article] [PubMed] [Google Scholar]
- Stams T., Nair S. K., Okuyama T., Waheed A., Sly W. S., Christianson D. W. Crystal structure of the secretory form of membrane-associated human carbonic anhydrase IV at 2.8-A resolution. Proc Natl Acad Sci U S A. 1996 Nov 26;93(24):13589–13594. doi: 10.1073/pnas.93.24.13589. [DOI] [PMC free article] [PubMed] [Google Scholar]
- Thorgeirsson T. E., Russell C. J., King D. S., Shin Y. K. Direct determination of the membrane affinities of individual amino acids. Biochemistry. 1996 Feb 13;35(6):1803–1809. doi: 10.1021/bi952300c. [DOI] [PubMed] [Google Scholar]
- White S. H., Wimley W. C., Selsted M. E. Structure, function, and membrane integration of defensins. Curr Opin Struct Biol. 1995 Aug;5(4):521–527. doi: 10.1016/0959-440x(95)80038-7. [DOI] [PubMed] [Google Scholar]
- Wimley W. C., Selsted M. E., White S. H. Interactions between human defensins and lipid bilayers: evidence for formation of multimeric pores. Protein Sci. 1994 Sep;3(9):1362–1373. doi: 10.1002/pro.5560030902. [DOI] [PMC free article] [PubMed] [Google Scholar]
- Wimley W. C., White S. H. Experimentally determined hydrophobicity scale for proteins at membrane interfaces. Nat Struct Biol. 1996 Oct;3(10):842–848. doi: 10.1038/nsb1096-842. [DOI] [PubMed] [Google Scholar]
- Woolf T. B., Roux B. Structure, energetics, and dynamics of lipid-protein interactions: A molecular dynamics study of the gramicidin A channel in a DMPC bilayer. Proteins. 1996 Jan;24(1):92–114. doi: 10.1002/(SICI)1097-0134(199601)24:1<92::AID-PROT7>3.0.CO;2-Q. [DOI] [PubMed] [Google Scholar]
- Zhou W., Parent L. J., Wills J. W., Resh M. D. Identification of a membrane-binding domain within the amino-terminal region of human immunodeficiency virus type 1 Gag protein which interacts with acidic phospholipids. J Virol. 1994 Apr;68(4):2556–2569. doi: 10.1128/jvi.68.4.2556-2569.1994. [DOI] [PMC free article] [PubMed] [Google Scholar]