Abstract
The application of two-dimensional Fourier-transform electron-spin-resonance (2D-FT-ESR) to the study of lipid/gramicidin A (GA) interactions is reported. It is shown that 2D-FT-ESR spectra provide substantially enhanced spectral resolution to changes in the dynamics and ordering of the bulk lipids (as compared with cw-ESR spectra), that result from addition of GA to membrane vesicles of dipalmitoylphosphatidylcholine (DPPC) in excess water containing 16-PC as the lipid spin label. The agreement between the theory of Lee, Budil, and Freed and experimental results is very good in the liquid crystalline phase. Both the rotational and translational diffusion rates of the bulk lipid are substantially decreased by addition of GA, whereas the ordering is only slightly increased, for a 1:5 ratio of GA to lipid. The slowing effect on the diffusive rates of adding GA in the gel phase is less pronounced. It is suggested that the spectral fits in this phase would be improved with a more detailed dynamic model. No significant evidence is found in the 2D-FT-ESR spectra for a second immobilized component upon addition of GA, which is in contrast to cw-ESR. It is shown from simulations of the observed 2D-FT-ESR spectra that the additional component seen in cw-ESR spectra, and usually attributed to "immobilized" lipid, is inconsistent with its being characterized by increased ordering, according to a model proposed by Ge and Freed, but it would be consistent with the more conventional model of a significantly reduced diffusional rate. This is because the 2D-FT-ESR spectra exhibit a selectivity, favoring components with longer homogeneous relaxation times, T2. The homogeneous linewidths of the 2D-FT-ESR autopeaks appear to broaden as a function of mixing time. This apparent broadening is very likely due to the process of cooperative order director fluctuations (ODF) of the lipids in the vesicle. This real-time observation of ODF is distinct from, but appears in reasonable agreement with, NMR results. It is found that addition of GA to give the 1:5 ratio has only a small effect on the ODF, but there is a significant temperature dependence.
Full text
PDF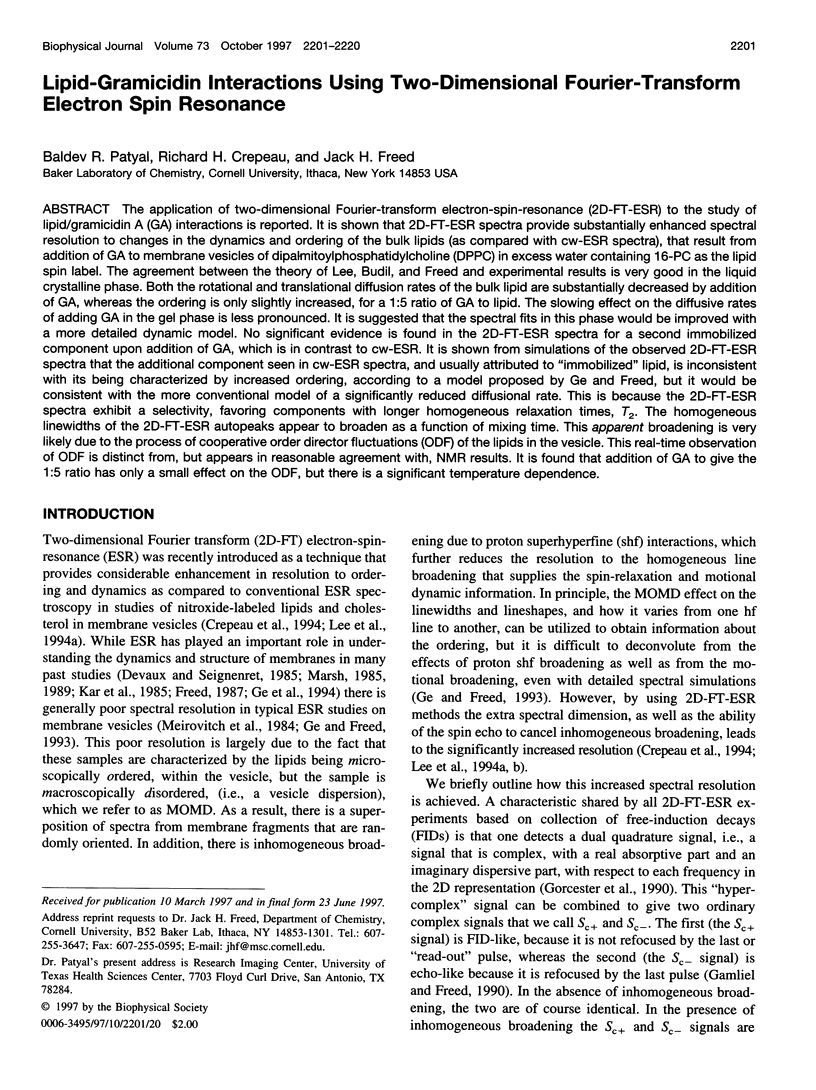
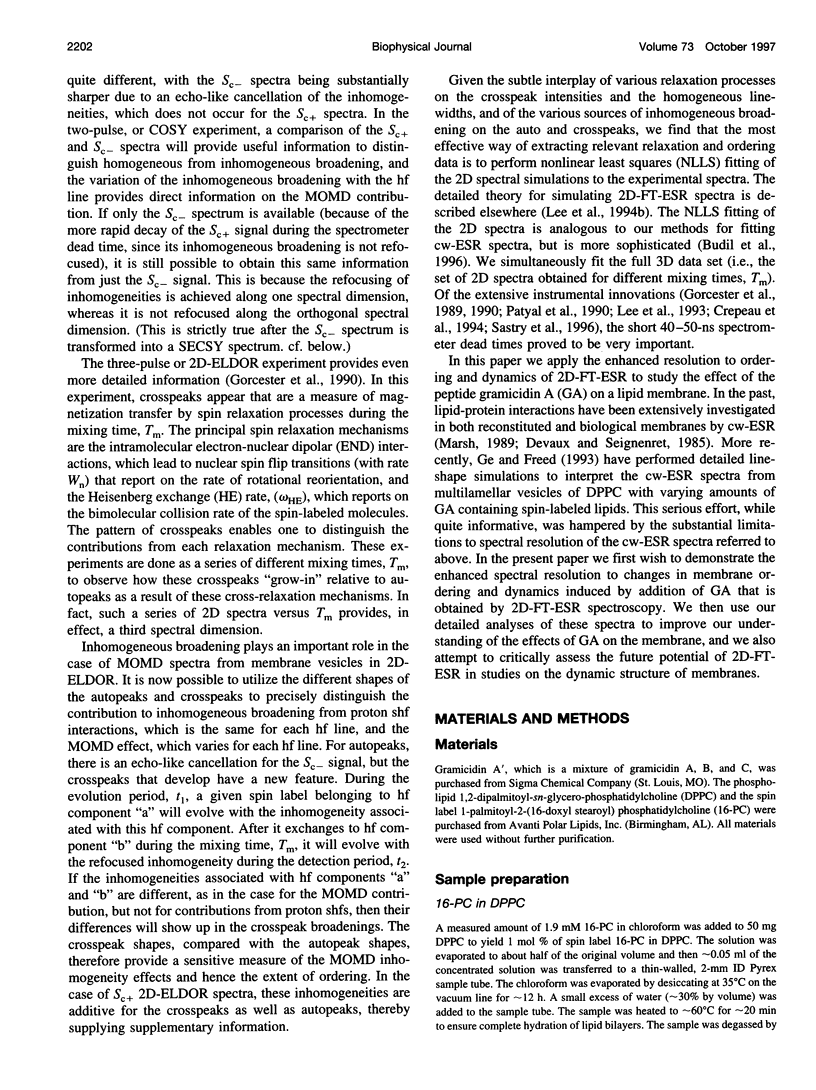
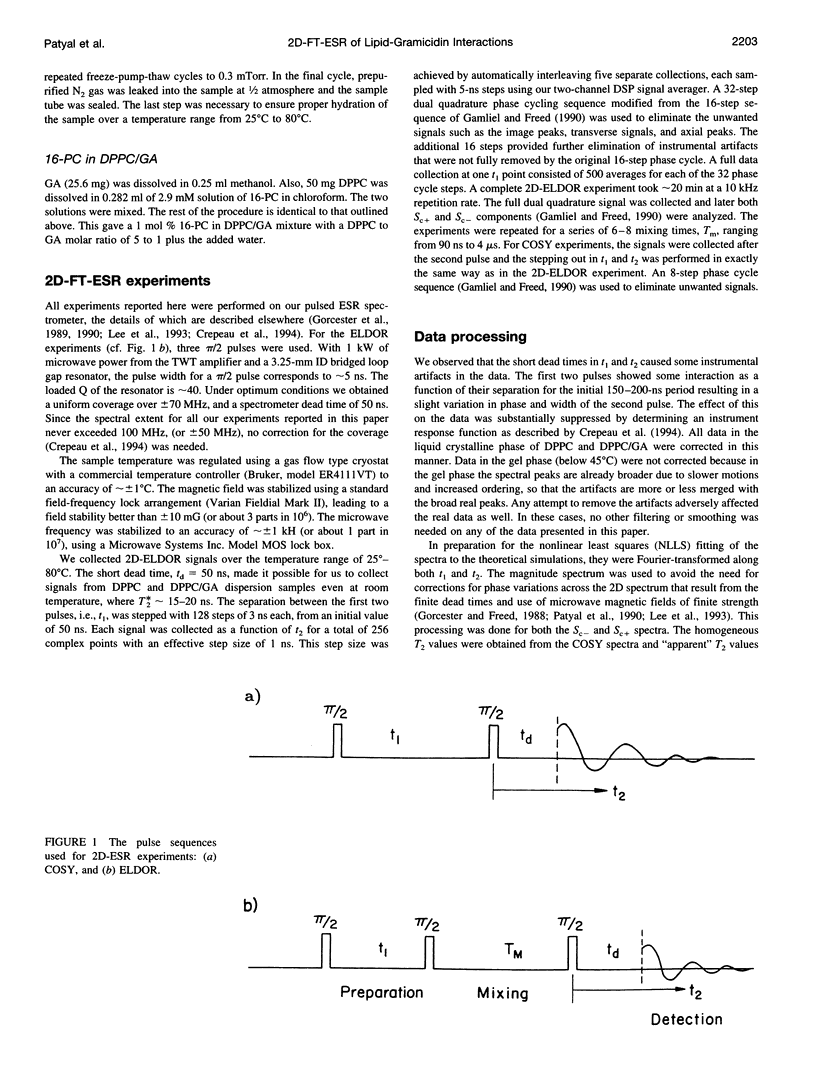
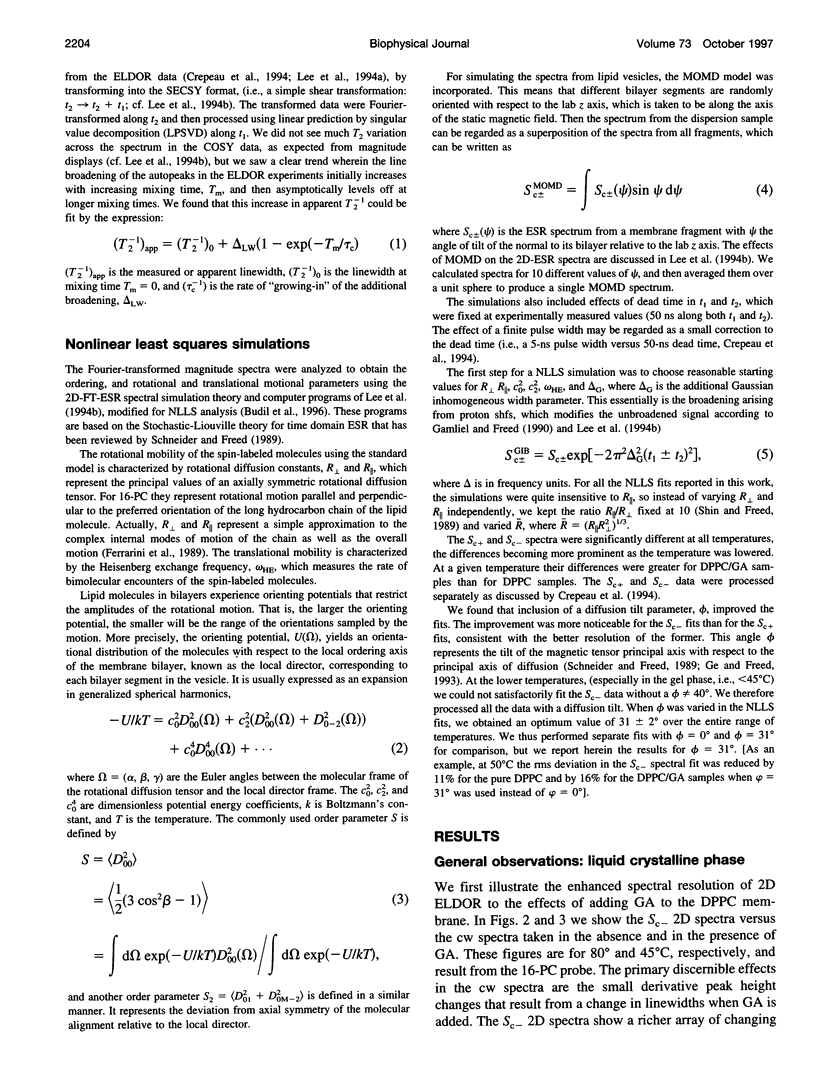
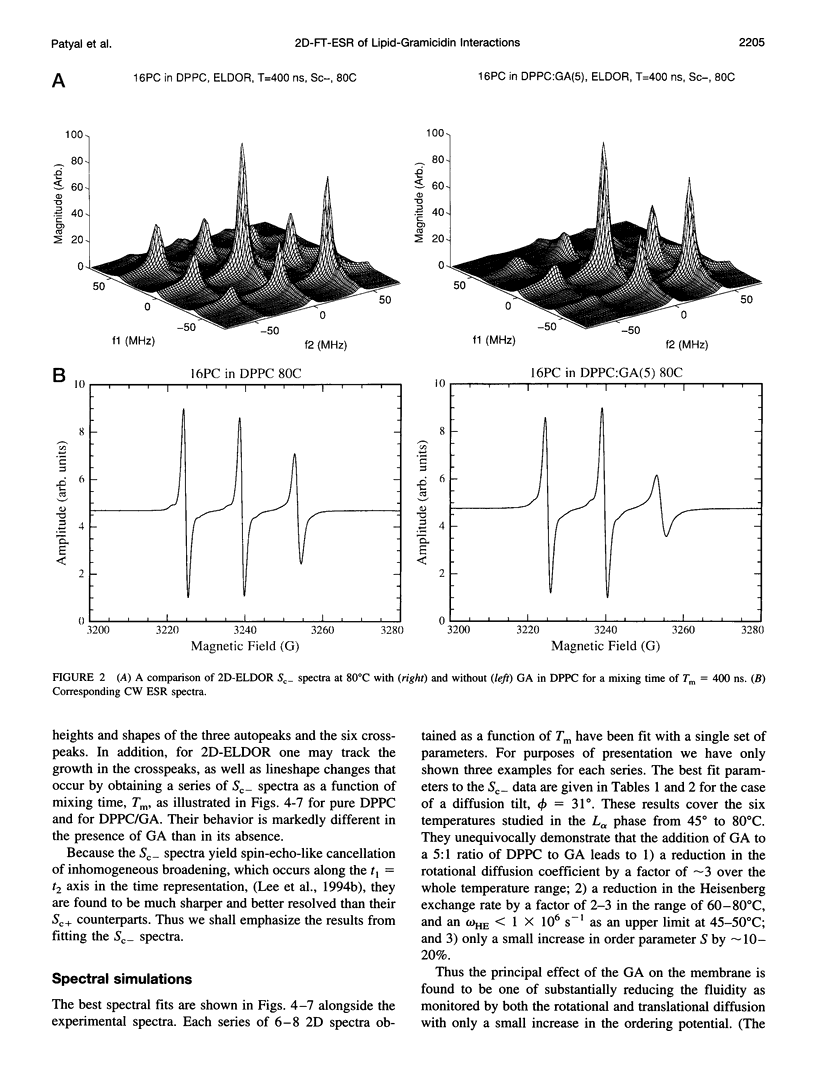
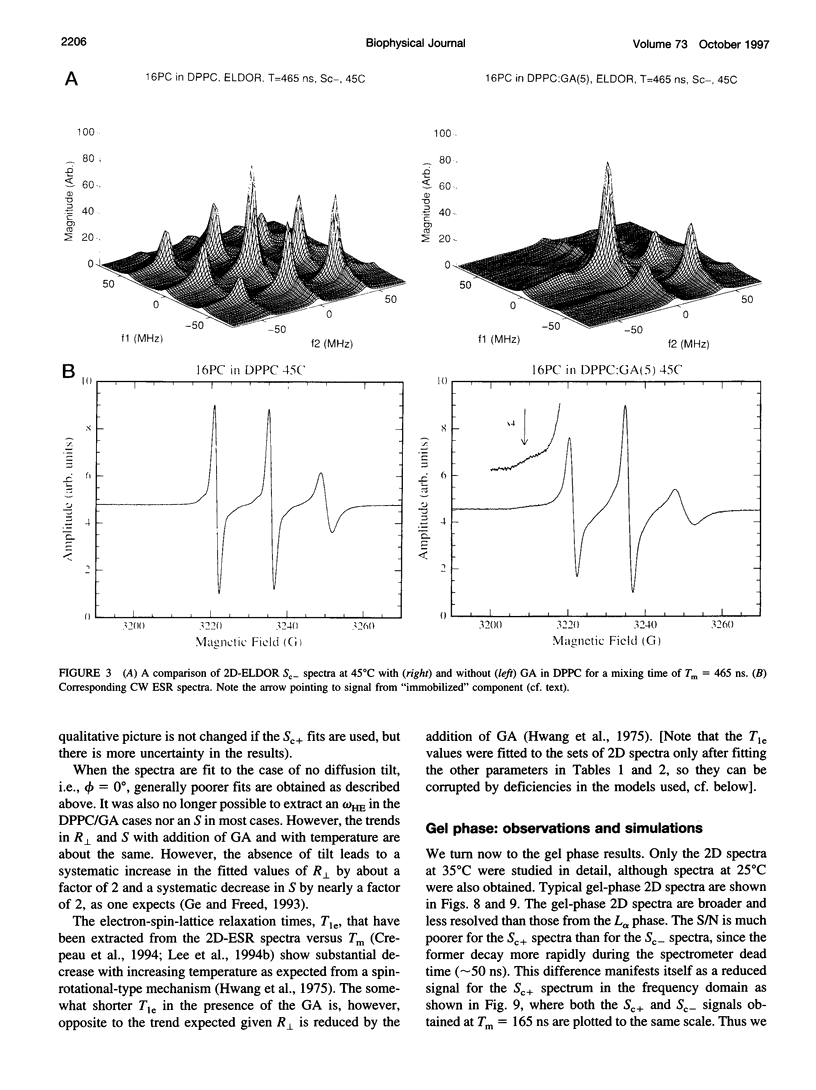
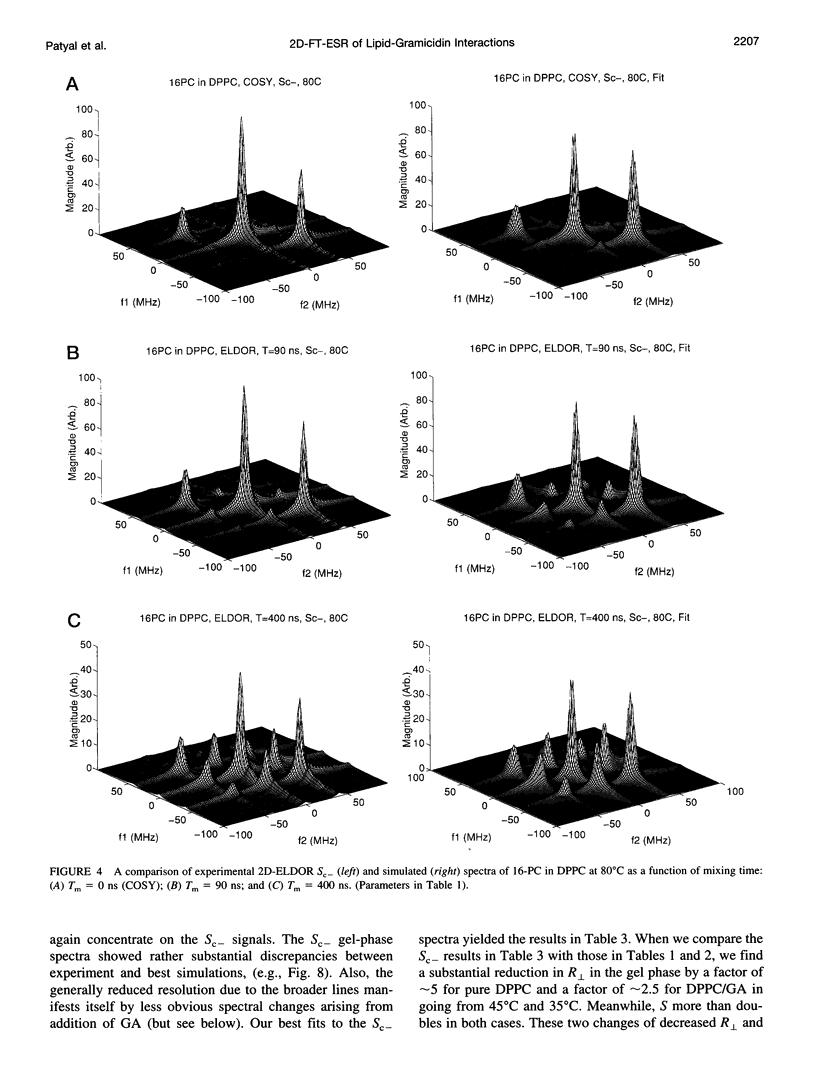
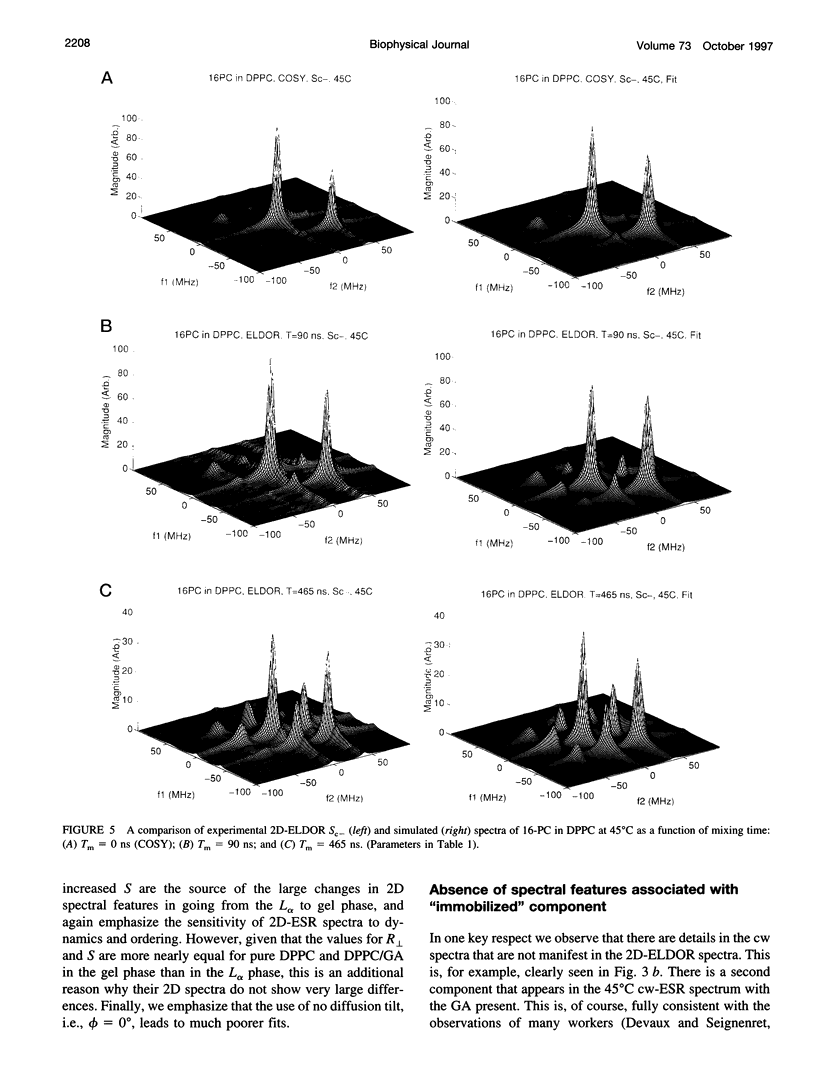
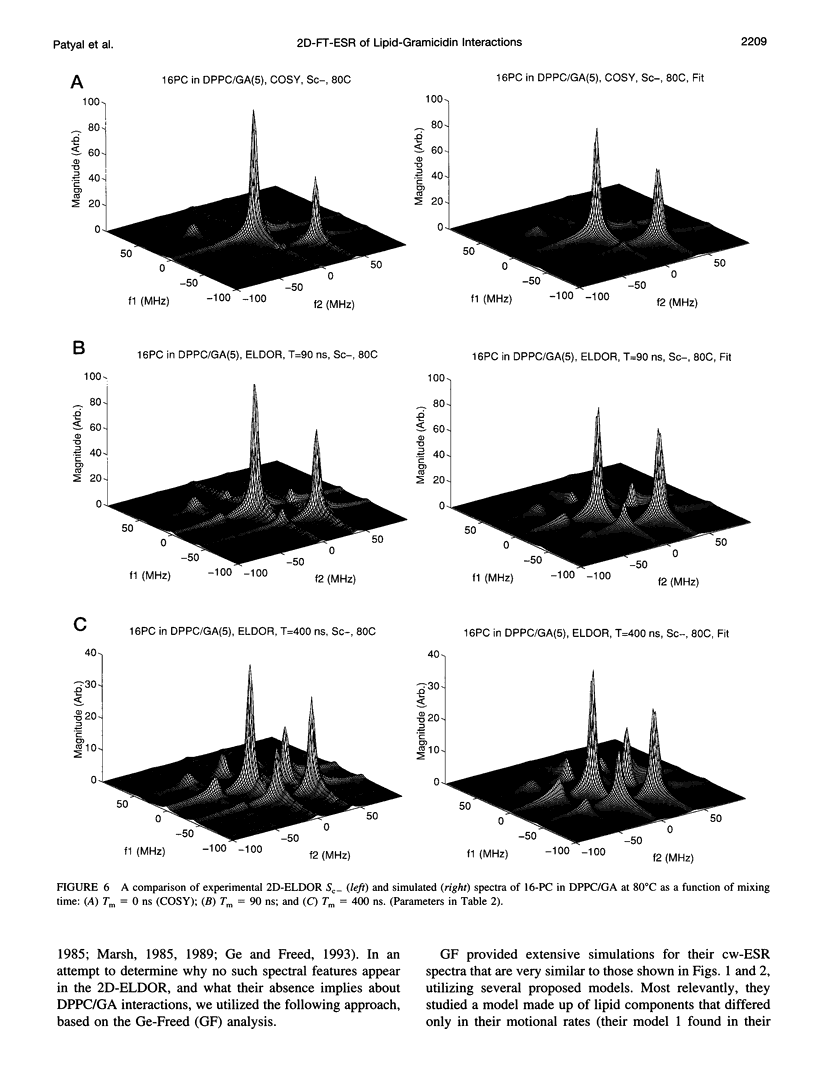
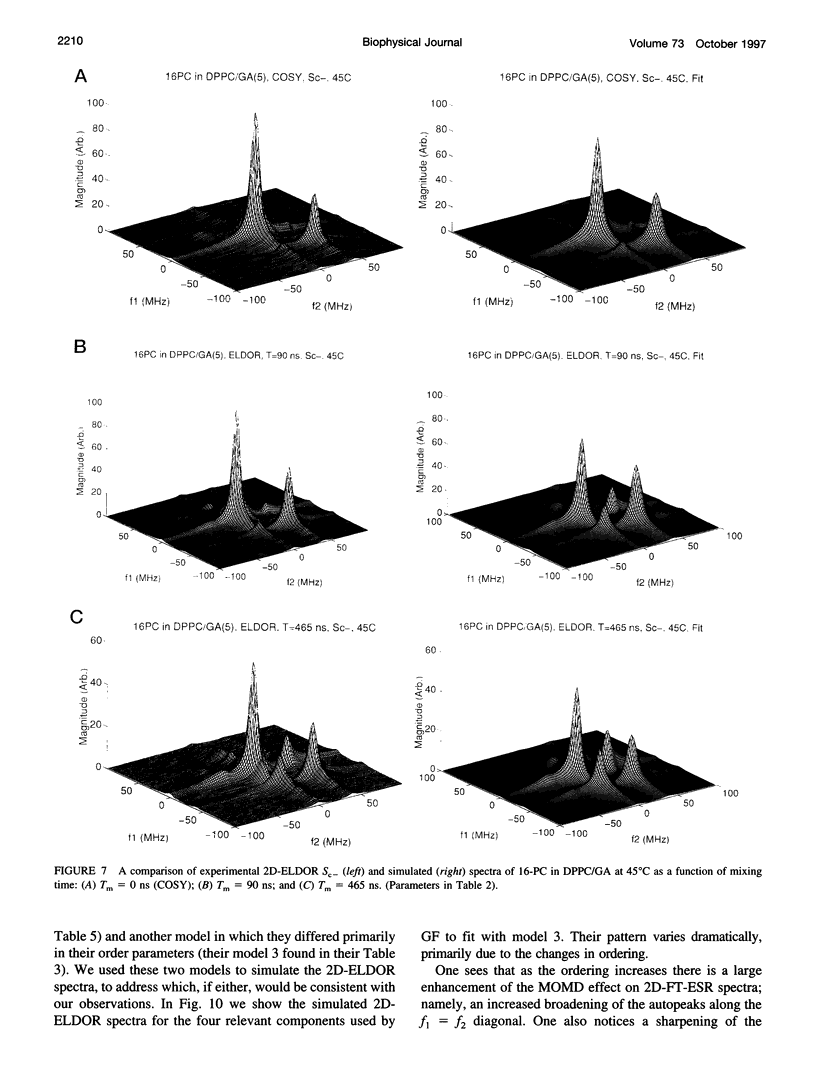
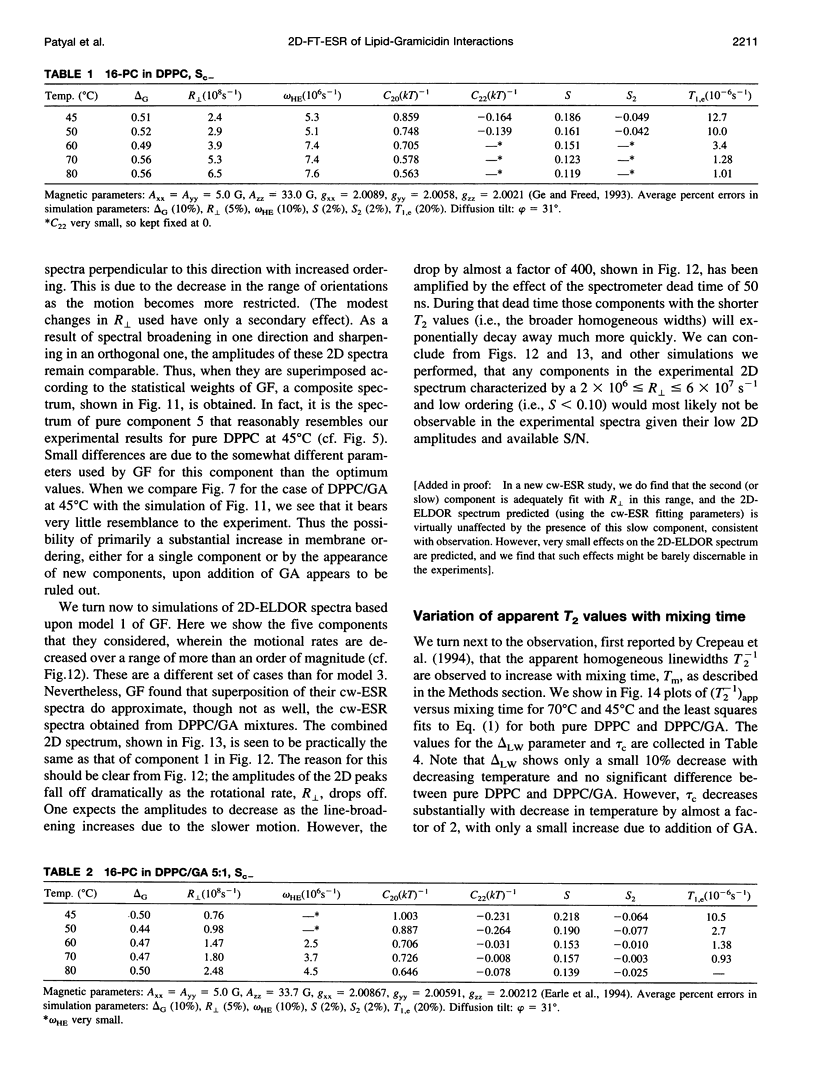
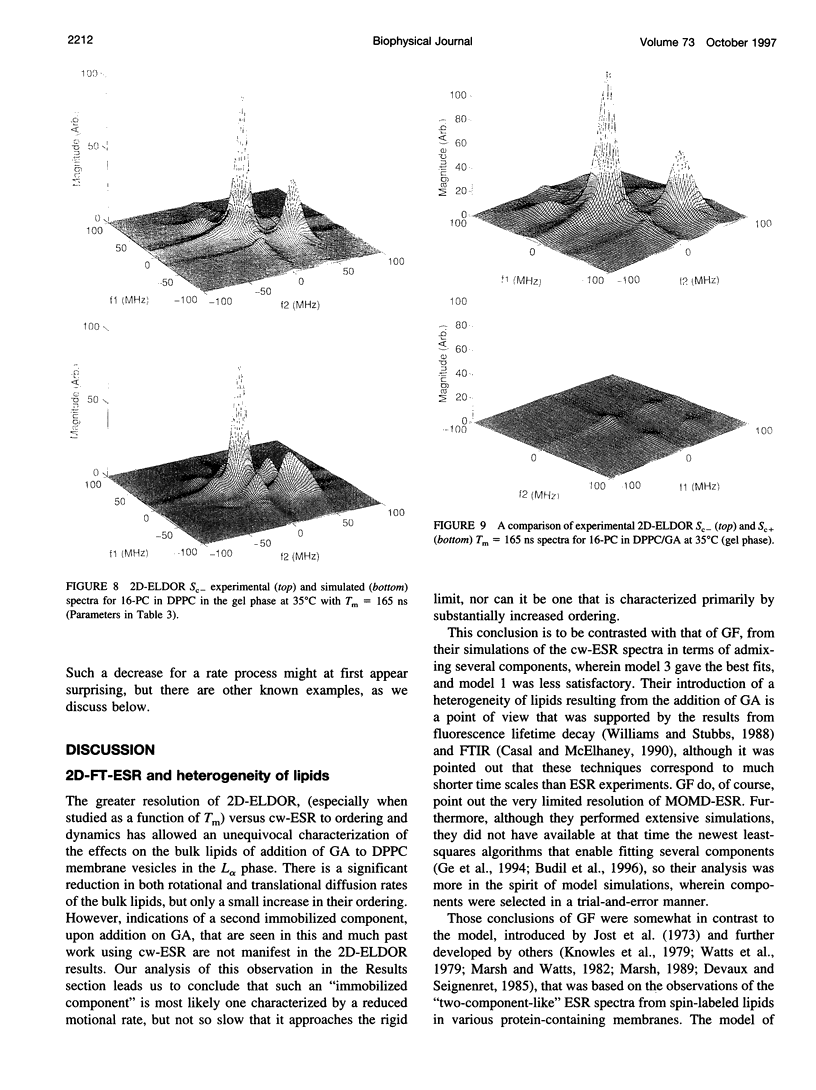
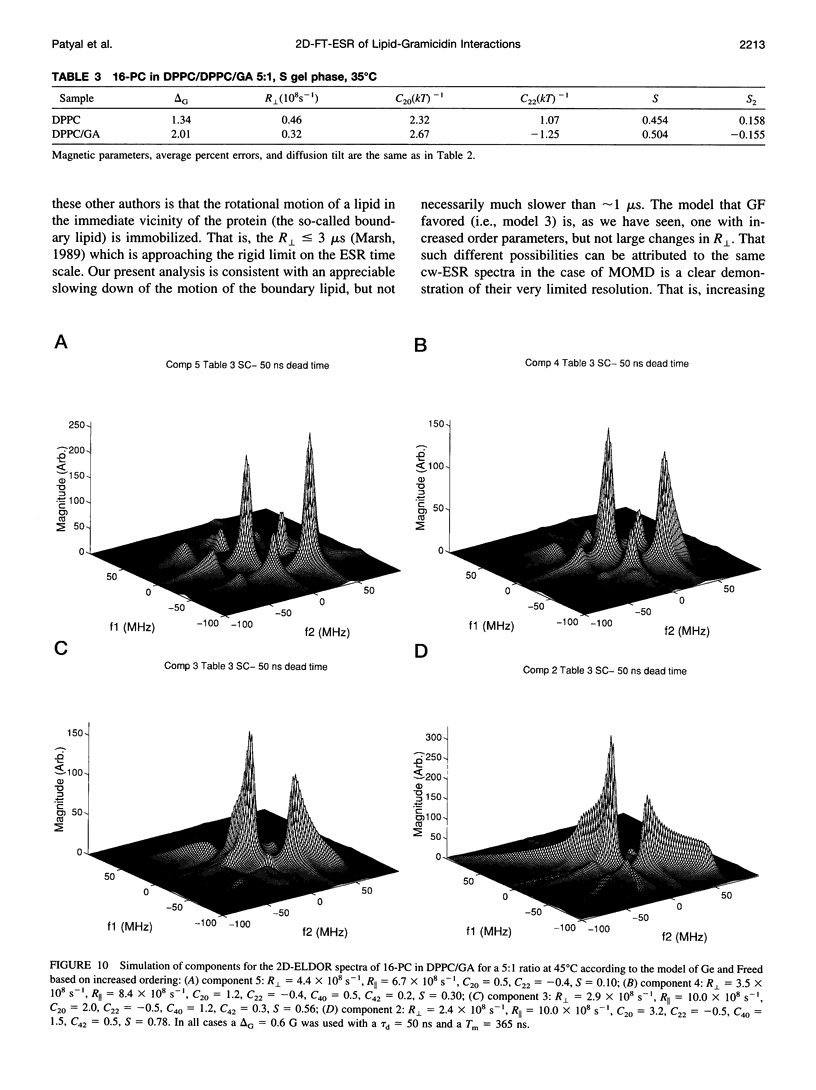
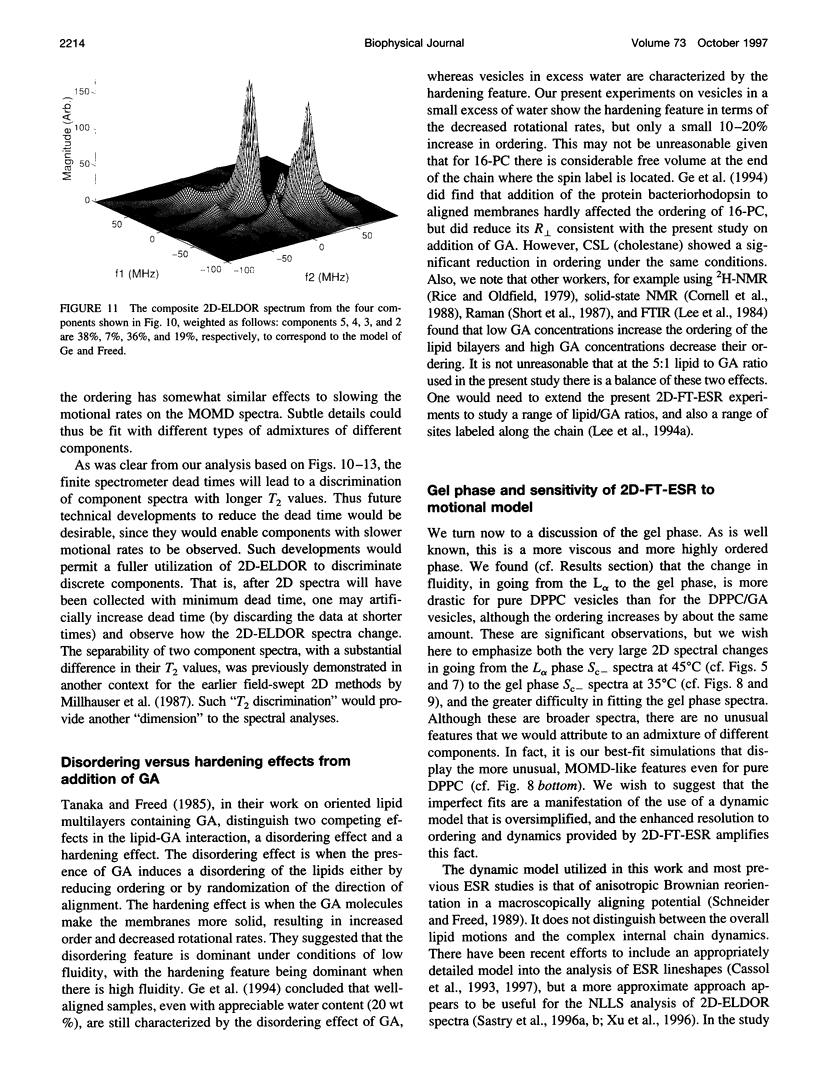
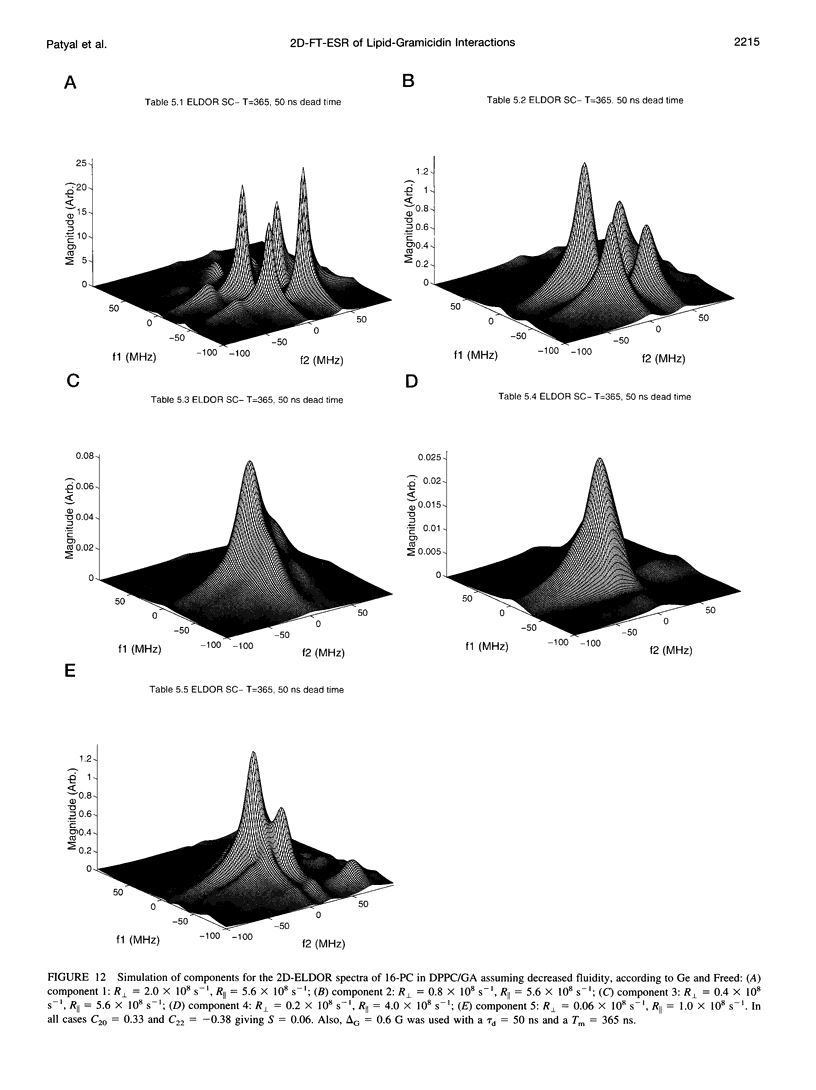
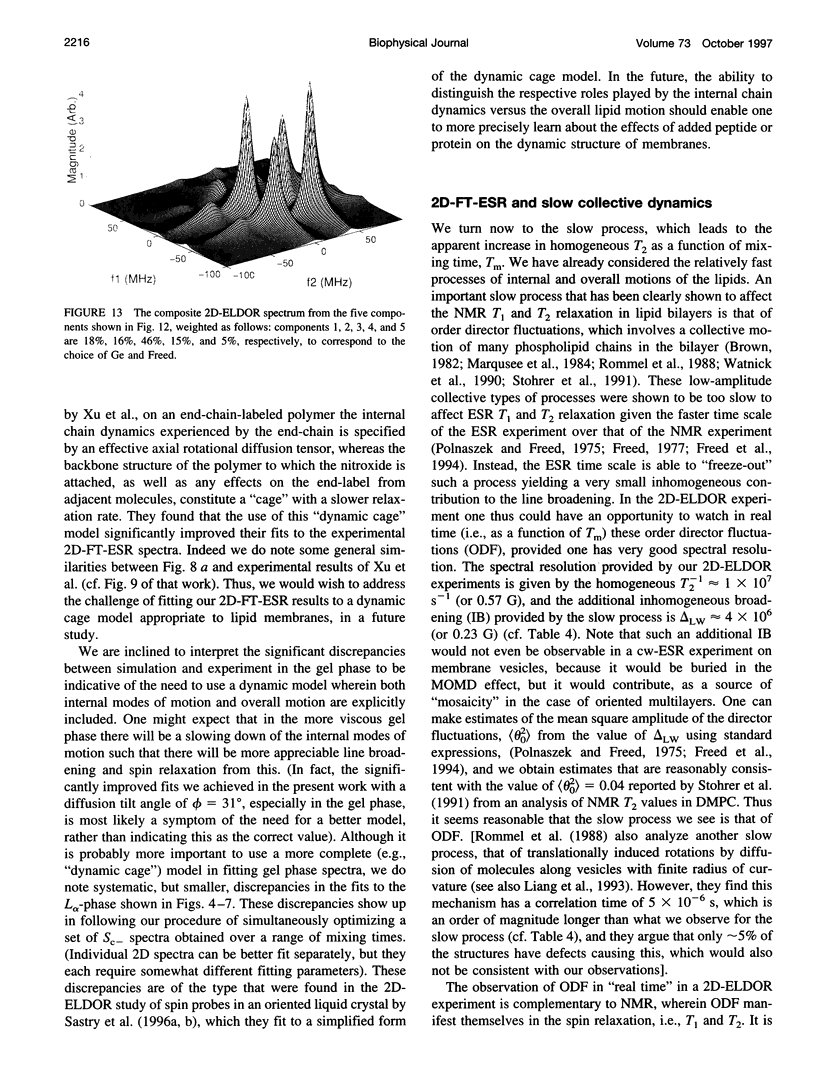
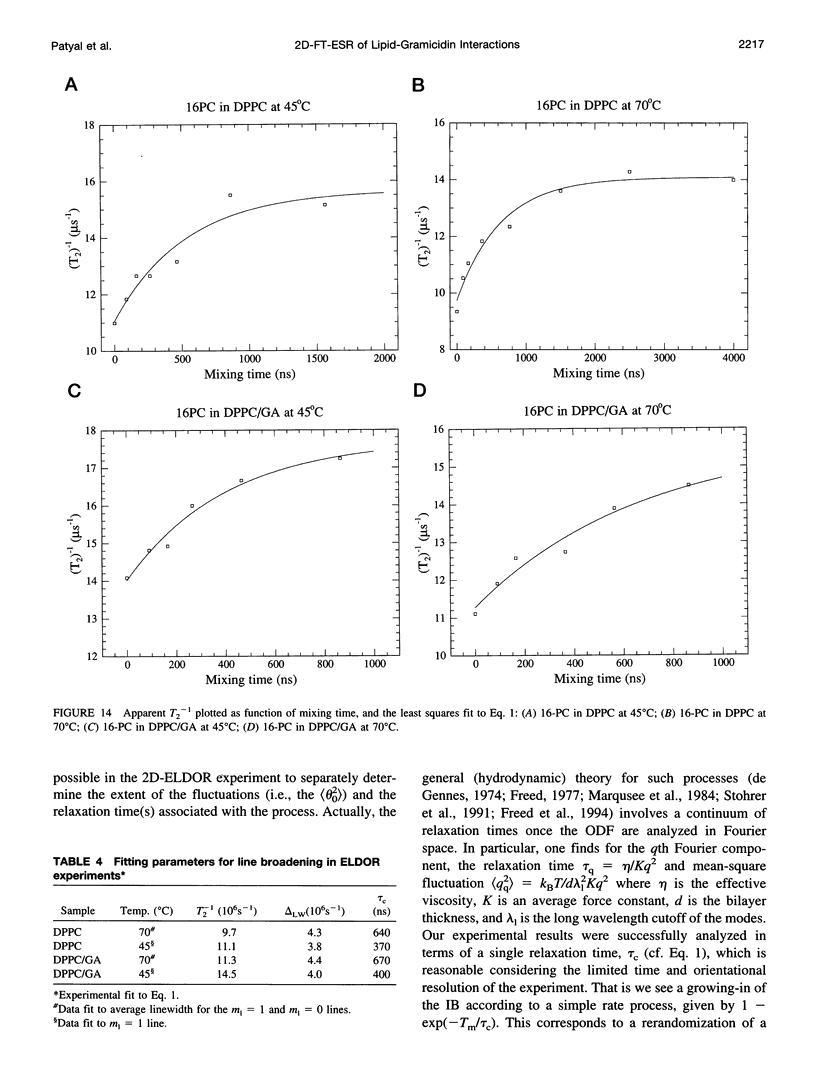
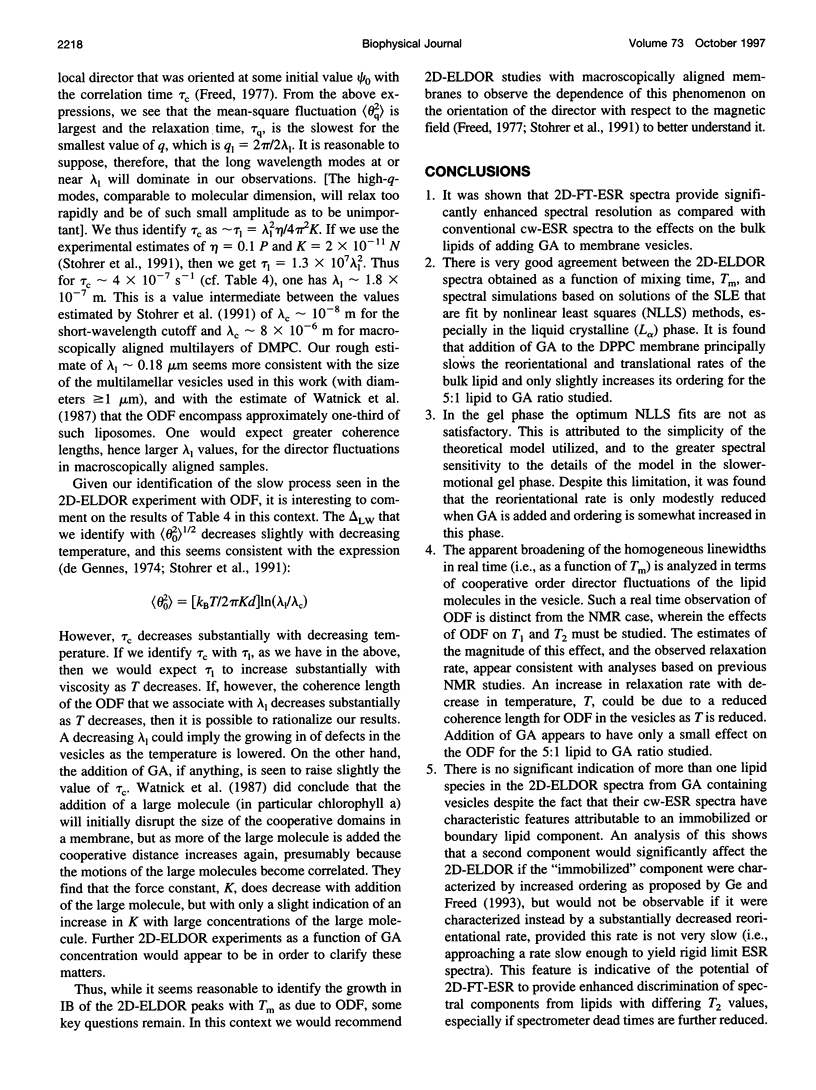
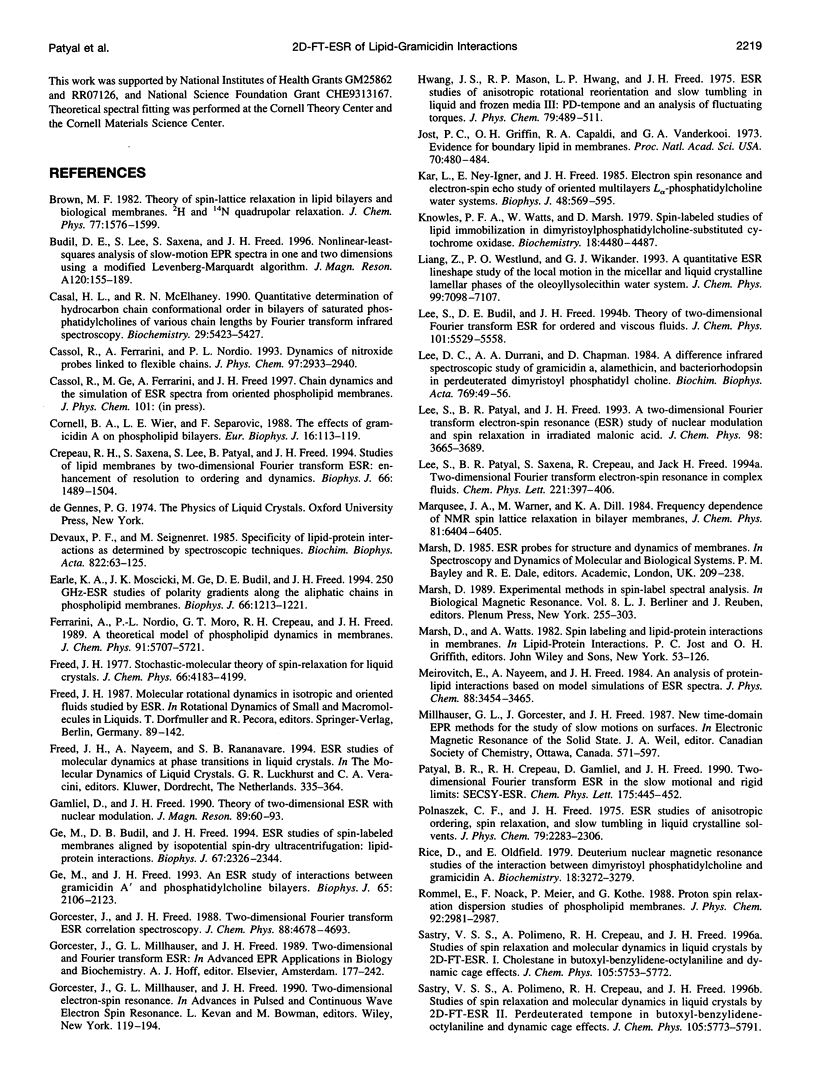
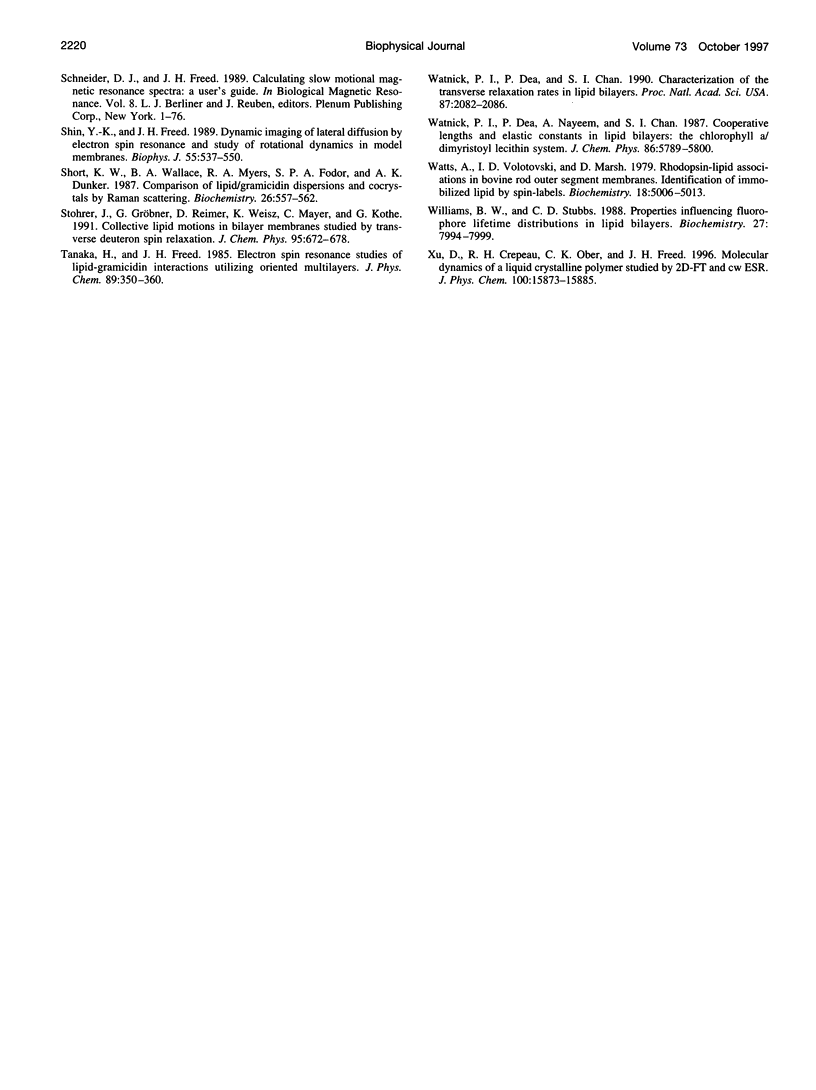
Images in this article
Selected References
These references are in PubMed. This may not be the complete list of references from this article.
- Casal H. L., McElhaney R. N. Quantitative determination of hydrocarbon chain conformational order in bilayers of saturated phosphatidylcholines of various chain lengths by Fourier transform infrared spectroscopy. Biochemistry. 1990 Jun 12;29(23):5423–5427. doi: 10.1021/bi00475a002. [DOI] [PubMed] [Google Scholar]
- Cornell B. A., Weir L. E., Separovic F. The effect of gramicidin A on phospholipid bilayers. Eur Biophys J. 1988;16(2):113–119. doi: 10.1007/BF00255521. [DOI] [PubMed] [Google Scholar]
- Crepeau R. H., Saxena S., Lee S., Patyal B., Freed J. H. Studies on lipid membranes by two-dimensional Fourier transform ESR: Enhancement of resolution to ordering and dynamics. Biophys J. 1994 May;66(5):1489–1504. doi: 10.1016/S0006-3495(94)80940-3. [DOI] [PMC free article] [PubMed] [Google Scholar]
- Devaux P. F., Seigneuret M. Specificity of lipid-protein interactions as determined by spectroscopic techniques. Biochim Biophys Acta. 1985 Jun 12;822(1):63–125. doi: 10.1016/0304-4157(85)90004-8. [DOI] [PubMed] [Google Scholar]
- Earle K. A., Moscicki J. K., Ge M., Budil D. E., Freed J. H. 250-GHz electron spin resonance studies of polarity gradients along the aliphatic chains in phospholipid membranes. Biophys J. 1994 Apr;66(4):1213–1221. doi: 10.1016/S0006-3495(94)80905-1. [DOI] [PMC free article] [PubMed] [Google Scholar]
- Ge M., Budil D. E., Freed J. H. ESR studies of spin-labeled membranes aligned by isopotential spin-dry ultracentrifugation: lipid-protein interactions. Biophys J. 1994 Dec;67(6):2326–2344. doi: 10.1016/S0006-3495(94)80719-2. [DOI] [PMC free article] [PubMed] [Google Scholar]
- Ge M., Freed J. H. An electron spin resonance study of interactions between gramicidin A' and phosphatidylcholine bilayers. Biophys J. 1993 Nov;65(5):2106–2123. doi: 10.1016/S0006-3495(93)81255-4. [DOI] [PMC free article] [PubMed] [Google Scholar]
- Jost P. C., Griffith O. H., Capaldi R. A., Vanderkooi G. Evidence for boundary lipid in membranes. Proc Natl Acad Sci U S A. 1973 Feb;70(2):480–484. doi: 10.1073/pnas.70.2.480. [DOI] [PMC free article] [PubMed] [Google Scholar]
- Kar L., Ney-Igner E., Freed J. H. Electron spin resonance and electron-spin-echo study of oriented multilayers of L alpha-dipalmitoylphosphatidylcholine water systems. Biophys J. 1985 Oct;48(4):569–595. doi: 10.1016/S0006-3495(85)83814-5. [DOI] [PMC free article] [PubMed] [Google Scholar]
- Knowles P. F., Watts A., Marsh D. Spin-label studies of lipid immobilization in dimyristoylphosphatidylcholine-substituted cytochrome oxidase. Biochemistry. 1979 Oct 16;18(21):4480–4487. doi: 10.1021/bi00588a005. [DOI] [PubMed] [Google Scholar]
- Lee D. C., Durrani A. A., Chapman D. A difference infrared spectroscopic study of gramicidin A, alamethicin and bacteriorhodopsin in perdeuterated dimyristoylphosphatidylcholine. Biochim Biophys Acta. 1984 Jan 11;769(1):49–56. doi: 10.1016/0005-2736(84)90008-7. [DOI] [PubMed] [Google Scholar]
- Rice D., Oldfield E. Deuterium nuclear magnetic resonance studies of the interaction between dimyristoylphosphatidylcholine and gramicidin A'. Biochemistry. 1979 Jul 24;18(15):3272–3279. doi: 10.1021/bi00582a012. [DOI] [PubMed] [Google Scholar]
- Shin Y. K., Freed J. H. Dynamic imaging of lateral diffusion by electron spin resonance and study of rotational dynamics in model membranes. Effect of cholesterol. Biophys J. 1989 Mar;55(3):537–550. doi: 10.1016/S0006-3495(89)82847-4. [DOI] [PMC free article] [PubMed] [Google Scholar]
- Short K. W., Wallace B. A., Myers R. A., Fodor S. P., Dunker A. K. Comparison of lipid/gramicidin dispersions and cocrystals by Raman scattering. Biochemistry. 1987 Jan 27;26(2):557–562. doi: 10.1021/bi00376a030. [DOI] [PubMed] [Google Scholar]
- Watnick P. I., Dea P., Chan S. I. Characterization of the transverse relaxation rates in lipid bilayers. Proc Natl Acad Sci U S A. 1990 Mar;87(6):2082–2086. doi: 10.1073/pnas.87.6.2082. [DOI] [PMC free article] [PubMed] [Google Scholar]
- Watts A., Volotovski I. D., Marsh D. Rhodopsin-lipid associations in bovine rod outer segment membranes. Identification of immobilized lipid by spin-labels. Biochemistry. 1979 Oct 30;18(22):5006–5013. doi: 10.1021/bi00589a031. [DOI] [PubMed] [Google Scholar]
- Williams B. W., Stubbs C. D. Properties influencing fluorophore lifetime distributions in lipid bilayers. Biochemistry. 1988 Oct 18;27(21):7994–7999. doi: 10.1021/bi00421a004. [DOI] [PubMed] [Google Scholar]