Abstract
The mechanical properties of cross-linked microtubule bundles were measured from outer pillar cells isolated from the mammalian inner ear. Measurements were made using a three-point bending test and were incorporated into a mathematical model designed to distinguish between the stiffness contributions from microtubules and their cross-linking proteins. Outer pillar cells were composed of 1000-3000 parallel bundled microtubules in a square array that was interdigitated and cross-linked with actin filaments. The average midpoint bending stiffness of intact cells was 7 x 10(-4) N/m. After removal of both the actin filaments and cross-links with detergent in the presence of DNase I, the square array was disrupted and the stiffness decreased by a factor of 4, to 1.7 x 10(-4) N/m. The bending modulus for individual microtubules was calculated to be 7 x 10(-23) Nm2, and the Young's modulus for these 15 protofilament microtubules was 2 x 10(9) Pa. The shear modulus between microtubules in intact cells was calculated to be 10(3) Pa. It was concluded that cross-linking proteins provided shear resistance between microtubules, which resulted in a fourfold increase in stiffness. The model can be used to estimate the mechanical properties of cross-linked microtubule bundles in cells from which direct measurements are not available.
Full text
PDF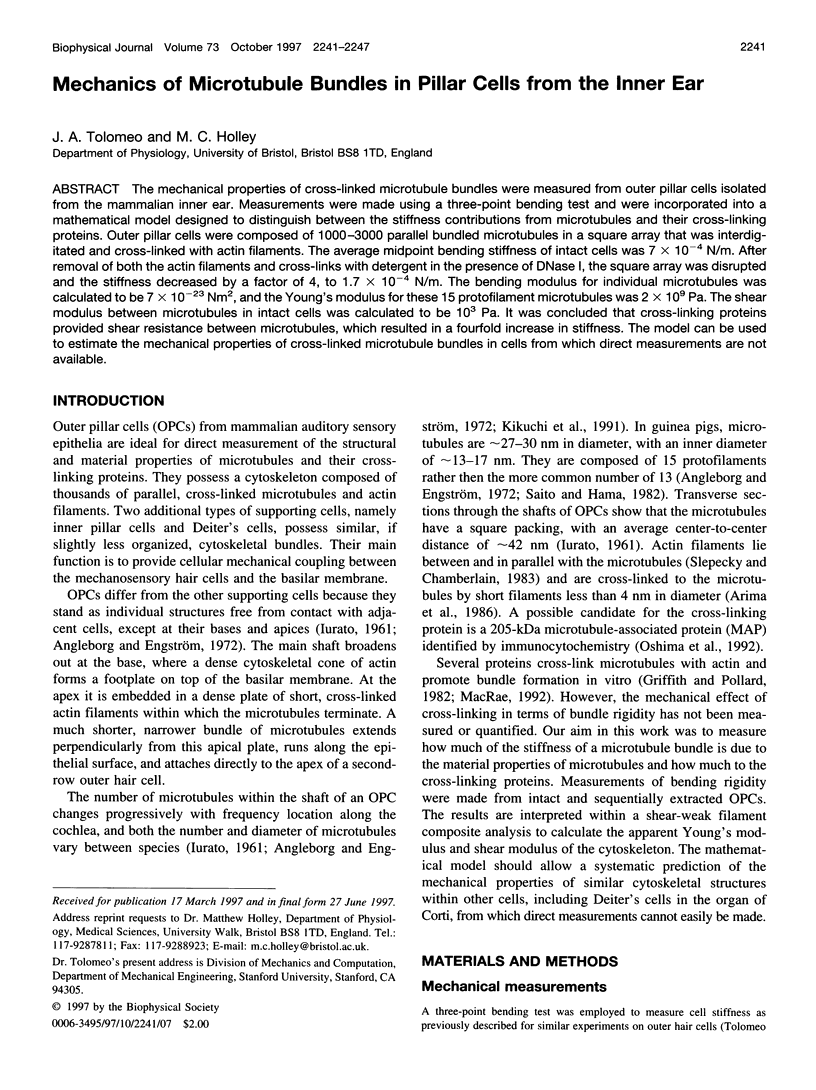
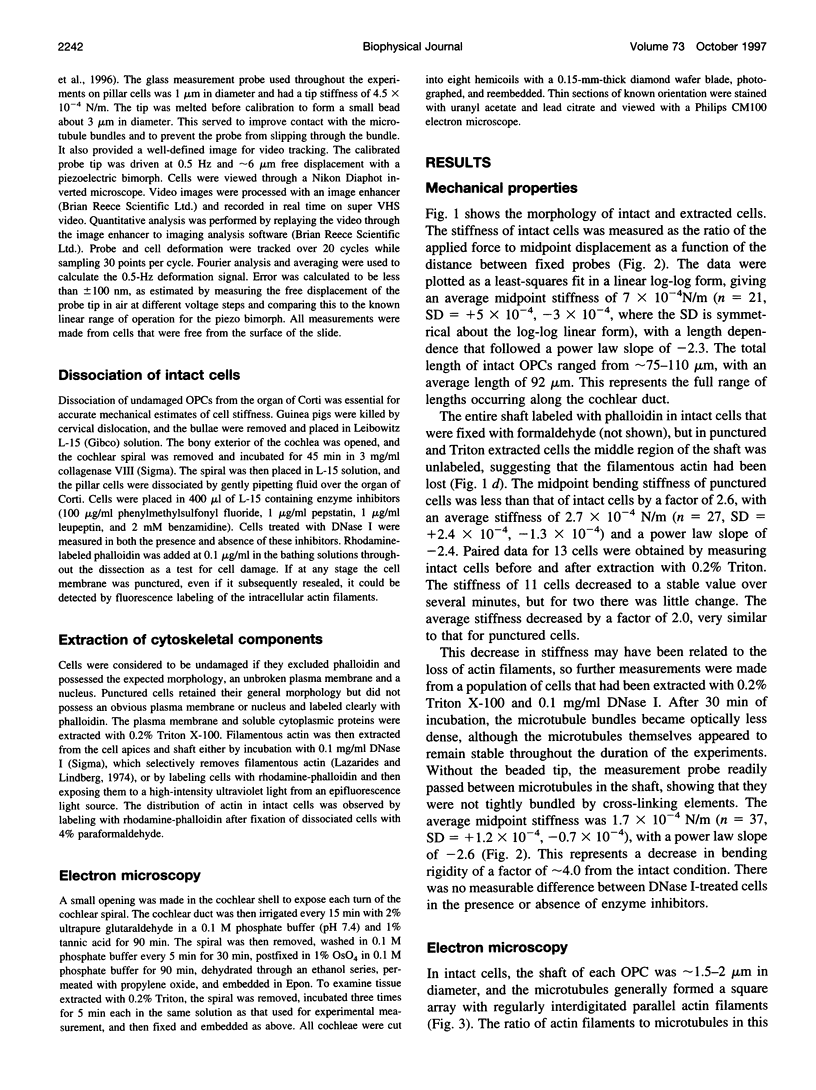
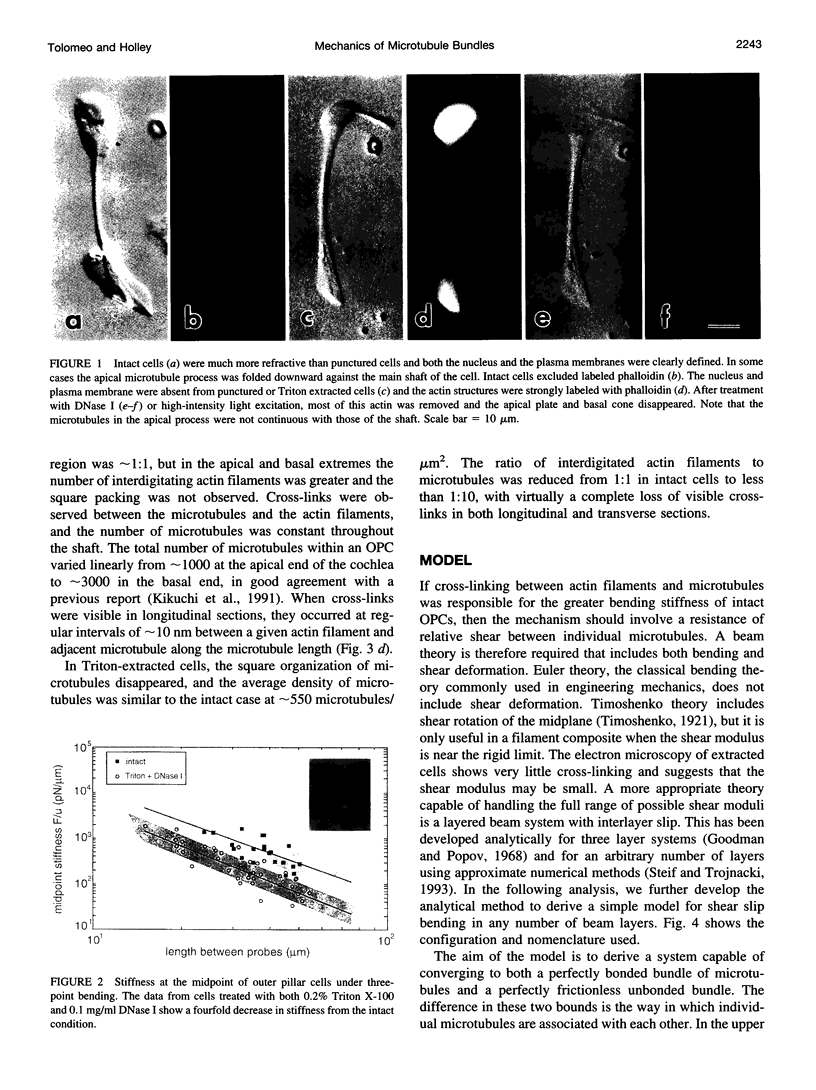
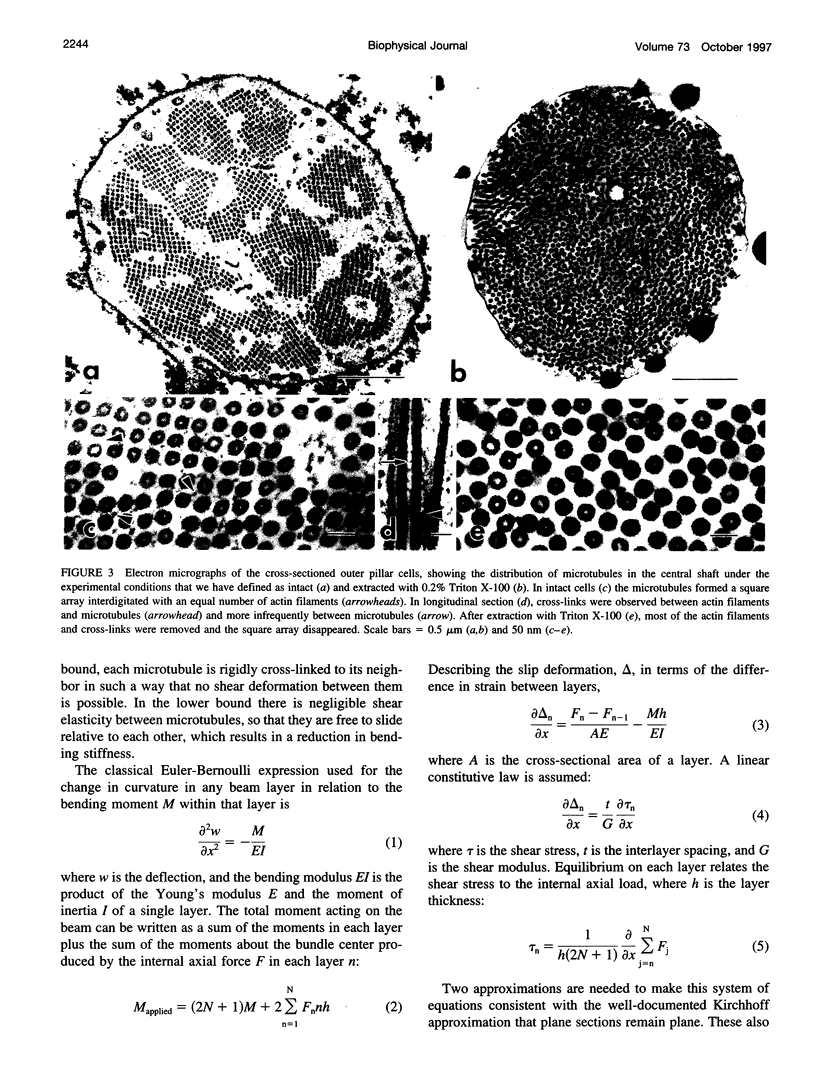
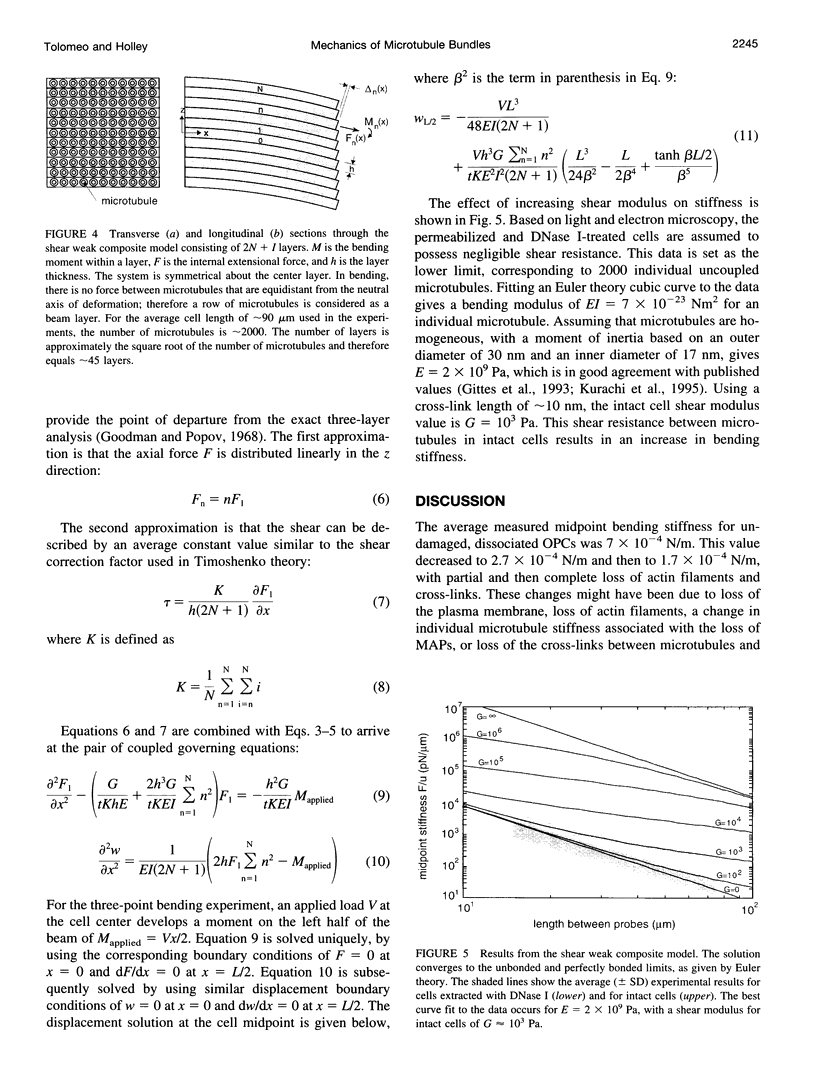
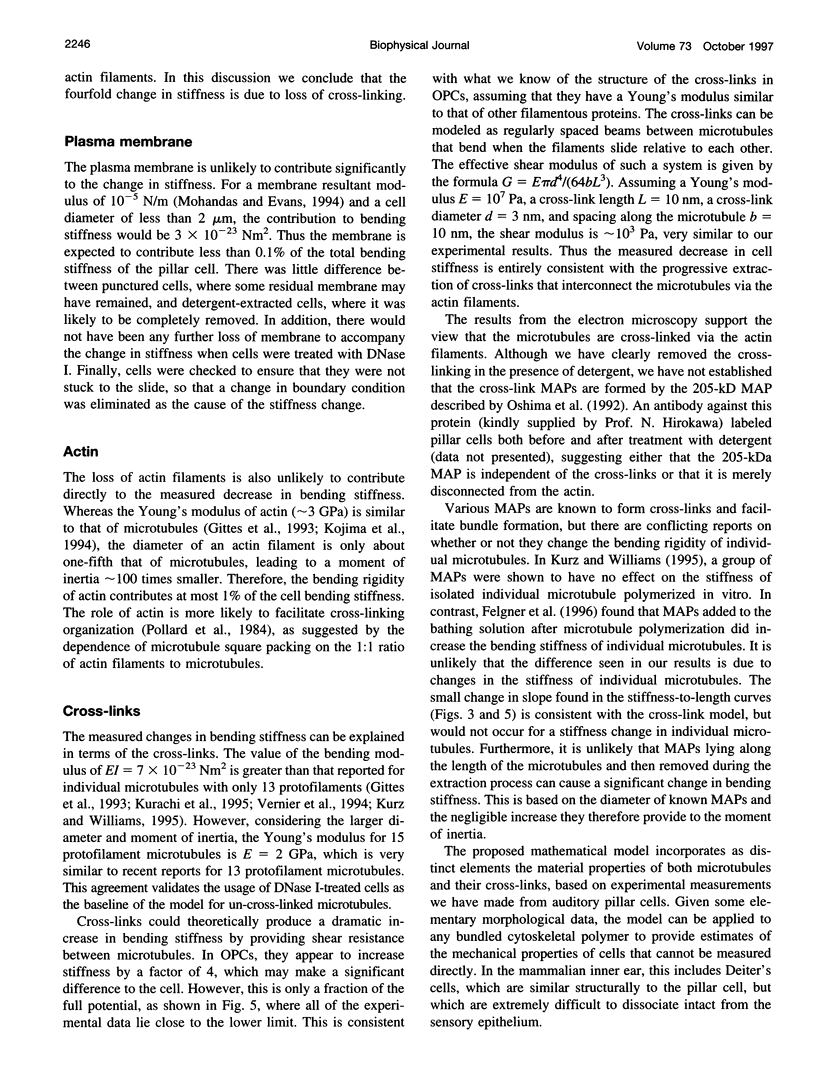
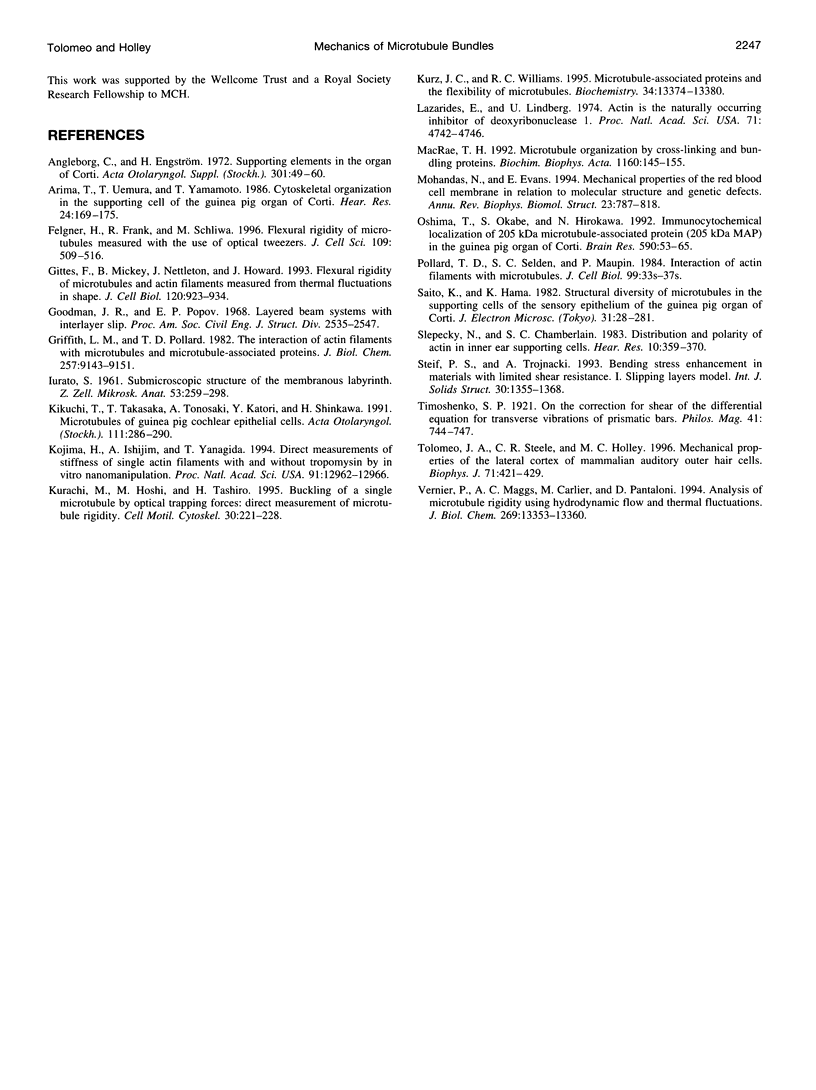
Images in this article
Selected References
These references are in PubMed. This may not be the complete list of references from this article.
- Arima T., Uemura T., Yamamoto T. Cytoskeletal organization in the supporting cell of the guinea pig organ of Corti. Hear Res. 1986;24(2):169–175. doi: 10.1016/0378-5955(86)90061-4. [DOI] [PubMed] [Google Scholar]
- Felgner H., Frank R., Schliwa M. Flexural rigidity of microtubules measured with the use of optical tweezers. J Cell Sci. 1996 Feb;109(Pt 2):509–516. doi: 10.1242/jcs.109.2.509. [DOI] [PubMed] [Google Scholar]
- Gittes F., Mickey B., Nettleton J., Howard J. Flexural rigidity of microtubules and actin filaments measured from thermal fluctuations in shape. J Cell Biol. 1993 Feb;120(4):923–934. doi: 10.1083/jcb.120.4.923. [DOI] [PMC free article] [PubMed] [Google Scholar]
- Griffith L. M., Pollard T. D. The interaction of actin filaments with microtubules and microtubule-associated proteins. J Biol Chem. 1982 Aug 10;257(15):9143–9151. [PubMed] [Google Scholar]
- Kikuchi T., Takasaka T., Tonosaki A., Katori Y., Shinkawa H. Microtubules of guinea pig cochlear epithelial cells. Acta Otolaryngol. 1991;111(2):286–290. doi: 10.3109/00016489109137389. [DOI] [PubMed] [Google Scholar]
- Kojima H., Ishijima A., Yanagida T. Direct measurement of stiffness of single actin filaments with and without tropomyosin by in vitro nanomanipulation. Proc Natl Acad Sci U S A. 1994 Dec 20;91(26):12962–12966. doi: 10.1073/pnas.91.26.12962. [DOI] [PMC free article] [PubMed] [Google Scholar]
- Kurachi M., Hoshi M., Tashiro H. Buckling of a single microtubule by optical trapping forces: direct measurement of microtubule rigidity. Cell Motil Cytoskeleton. 1995;30(3):221–228. doi: 10.1002/cm.970300306. [DOI] [PubMed] [Google Scholar]
- Kurz J. C., Williams R. C., Jr Microtubule-associated proteins and the flexibility of microtubules. Biochemistry. 1995 Oct 17;34(41):13374–13380. doi: 10.1021/bi00041a014. [DOI] [PubMed] [Google Scholar]
- Lazarides E., Lindberg U. Actin is the naturally occurring inhibitor of deoxyribonuclease I. Proc Natl Acad Sci U S A. 1974 Dec;71(12):4742–4746. doi: 10.1073/pnas.71.12.4742. [DOI] [PMC free article] [PubMed] [Google Scholar]
- MacRae T. H. Microtubule organization by cross-linking and bundling proteins. Biochim Biophys Acta. 1992 Nov 20;1160(2):145–155. doi: 10.1016/0167-4838(92)90001-t. [DOI] [PubMed] [Google Scholar]
- Mohandas N., Evans E. Mechanical properties of the red cell membrane in relation to molecular structure and genetic defects. Annu Rev Biophys Biomol Struct. 1994;23:787–818. doi: 10.1146/annurev.bb.23.060194.004035. [DOI] [PubMed] [Google Scholar]
- Oshima T., Okabe S., Hirokawa N. Immunocytochemical localization of 205 kDa microtubule-associated protein (205 kDa MAP) in the guinea pig organ of Corti. Brain Res. 1992 Sep 11;590(1-2):53–65. doi: 10.1016/0006-8993(92)91081-o. [DOI] [PubMed] [Google Scholar]
- Pollard T. D., Selden S. C., Maupin P. Interaction of actin filaments with microtubules. J Cell Biol. 1984 Jul;99(1 Pt 2):33s–37s. doi: 10.1083/jcb.99.1.33s. [DOI] [PMC free article] [PubMed] [Google Scholar]
- Saito K., Hama K. Structural diversity of microtubules in the supporting cells of the sensory epithelium of guinea pig organ of Corti. J Electron Microsc (Tokyo) 1982;31(3):278–281. [PubMed] [Google Scholar]
- Slepecky N., Chamberlain S. C. Distribution and polarity of actin in inner ear supporting cells. Hear Res. 1983 Jun;10(3):359–370. doi: 10.1016/0378-5955(83)90098-9. [DOI] [PubMed] [Google Scholar]
- Tolomeo J. A., Steele C. R., Holley M. C. Mechanical properties of the lateral cortex of mammalian auditory outer hair cells. Biophys J. 1996 Jul;71(1):421–429. doi: 10.1016/S0006-3495(96)79244-5. [DOI] [PMC free article] [PubMed] [Google Scholar]
- Venier P., Maggs A. C., Carlier M. F., Pantaloni D. Analysis of microtubule rigidity using hydrodynamic flow and thermal fluctuations. J Biol Chem. 1994 May 6;269(18):13353–13360. [PubMed] [Google Scholar]