Abstract
The scanning electrochemical microscope (SECM) is a scanned probe microscope that uses the response of a mobile ultramicroelectrode (UME) tip to determine the reactivity, topography, and mass transport characteristics of interfaces with high spatial resolution. SECM strategies for measuring the rates of solute diffusion and convection through samples of cartilage, using amperometric UMEs, are outlined. The methods are used to determine the diffusion coefficients of oxygen and ruthenium(III) hexamine [Ru(NH3)6(3+)] in laryngeal cartilage. The diffusion coefficient of oxygen in cartilage is found to be approximately 50% of that in aqueous electrolyte solution, assuming a partition coefficient of unity for oxygen between cartilage and aqueous solution. In contrast, diffusion of Ru(NH3)6(3+) within the cartilage sample cannot be detected on the SECM timescale, suggesting a diffusion coefficient at least two orders of magnitude lower than that in solution, given a measured partition coefficient for Ru(NH3)6(3+) between cartilage and aqueous solution, Kp = [Ru(NH3)6(3+)]cartilage/[RU(NH3)6(3+)]solution = 3.4 +/- 0.1. Rates of Ru(NH3)6(3+) osmotically driven convective transport across cartilage samples are imaged at high spatial resolution by monitoring the current response of a scanning UME, with an osmotic pressure of approximately 0.75 atm across the slice. A model is outlined that enables the current response to be related to the local flux. By determining the topography of the sample from the current response with no applied osmotic pressure, local transport rates can be correlated with topographical features of the sample surface, at much higher spatial resolution than has previously been achieved.
Full text
PDF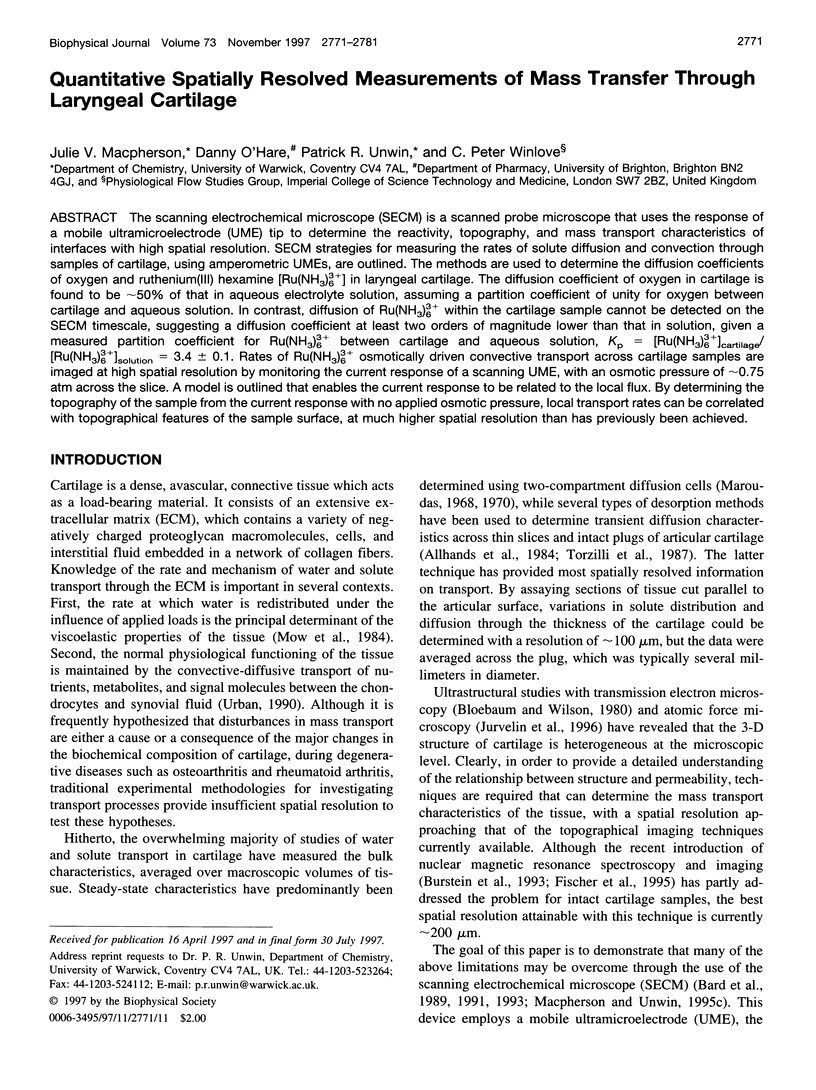
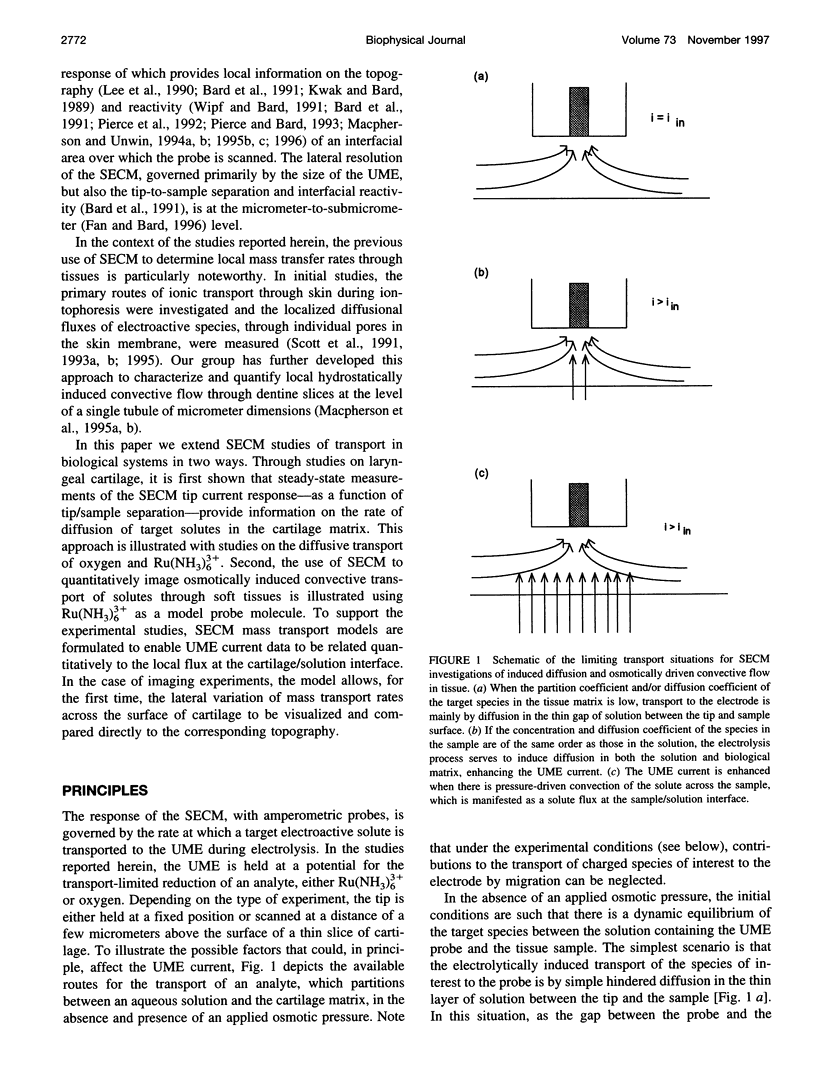
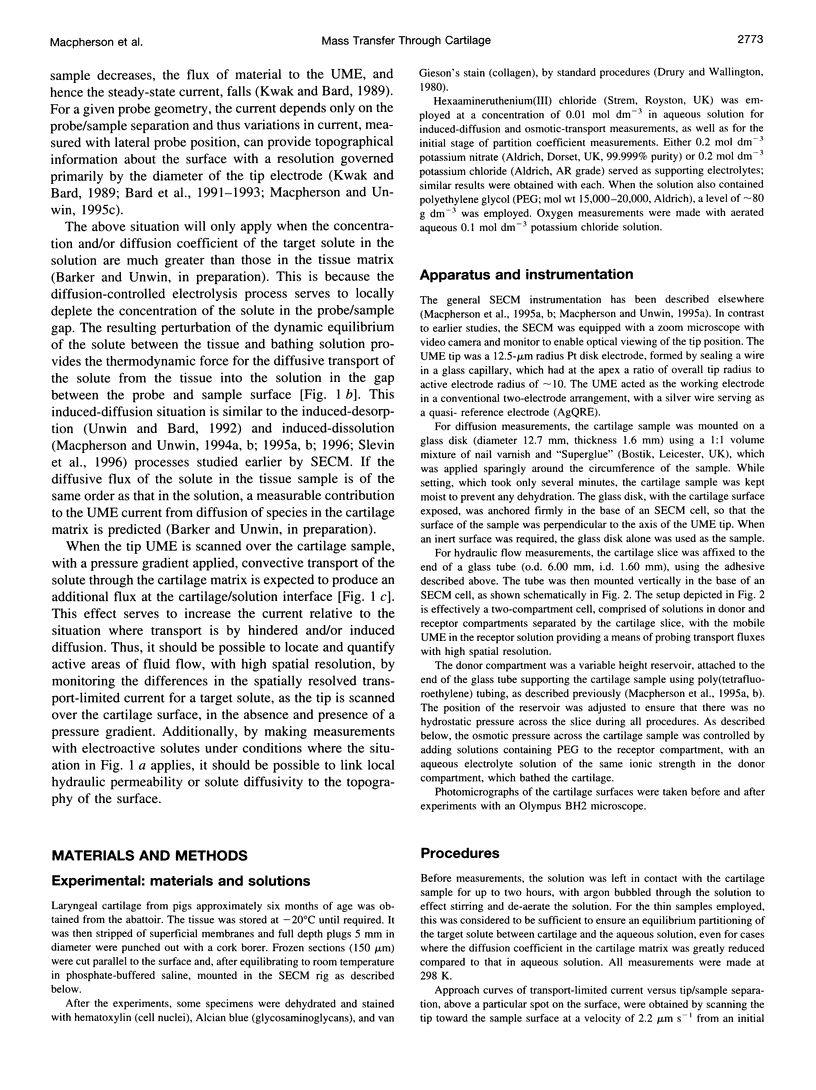
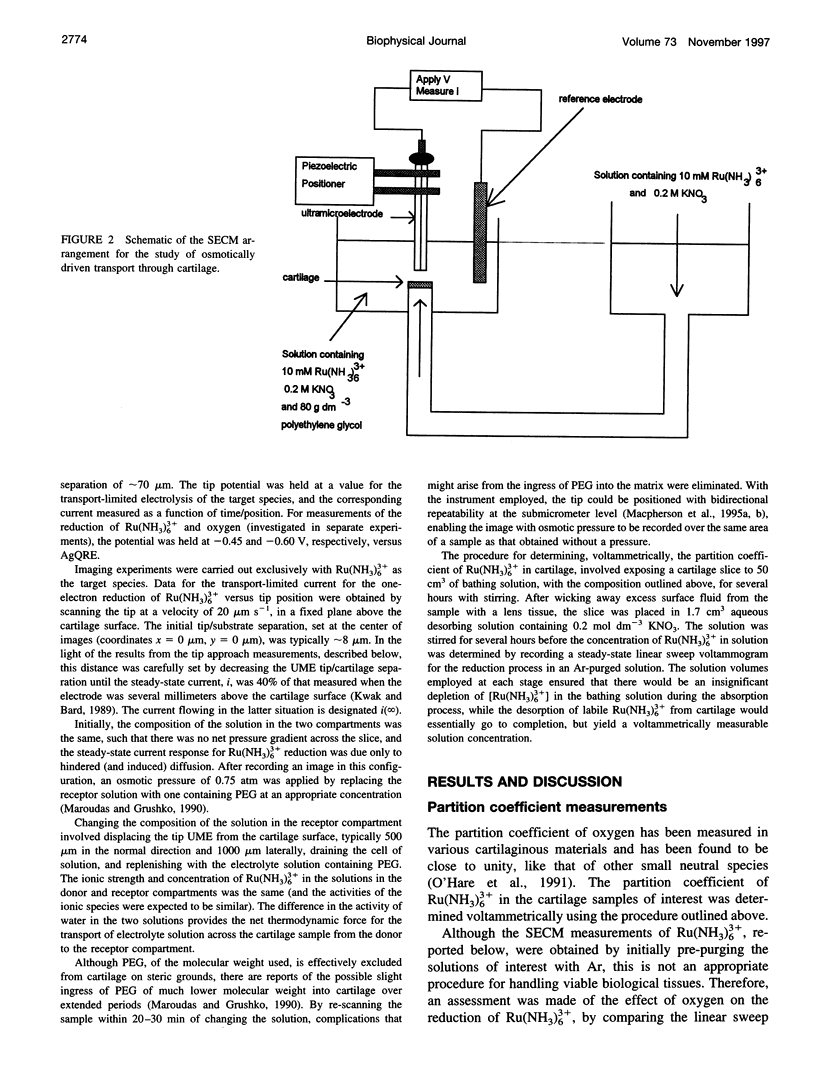
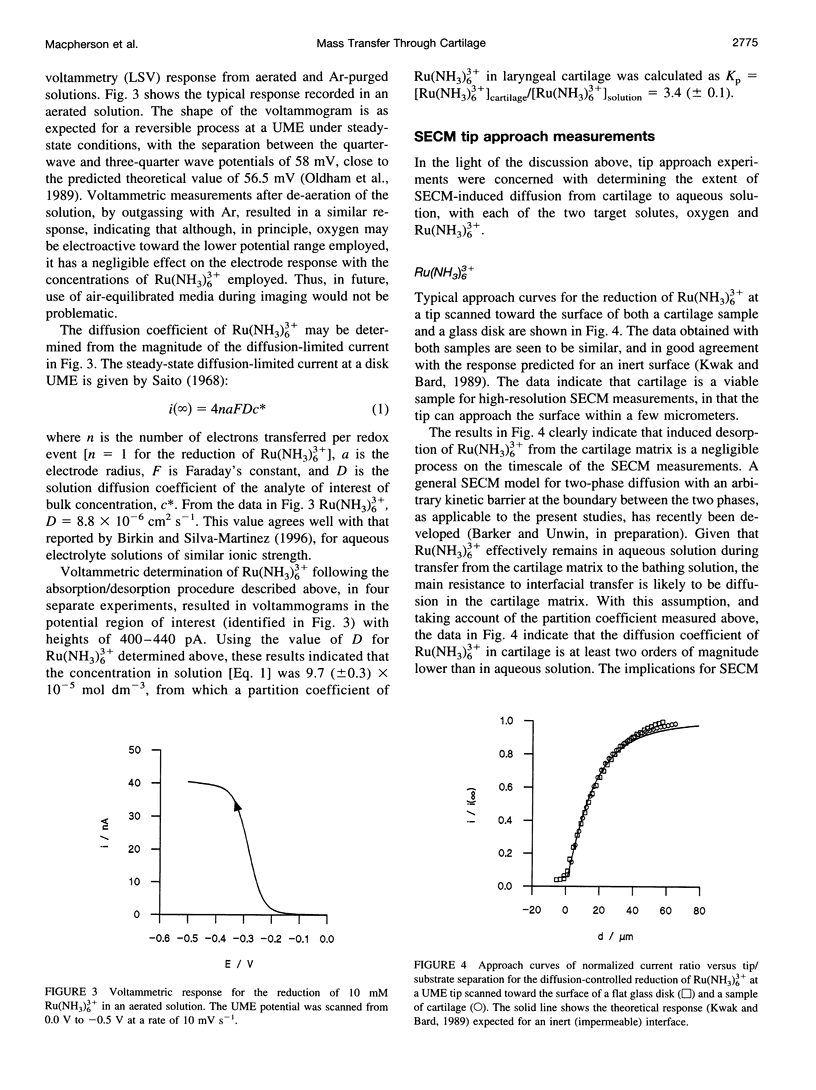
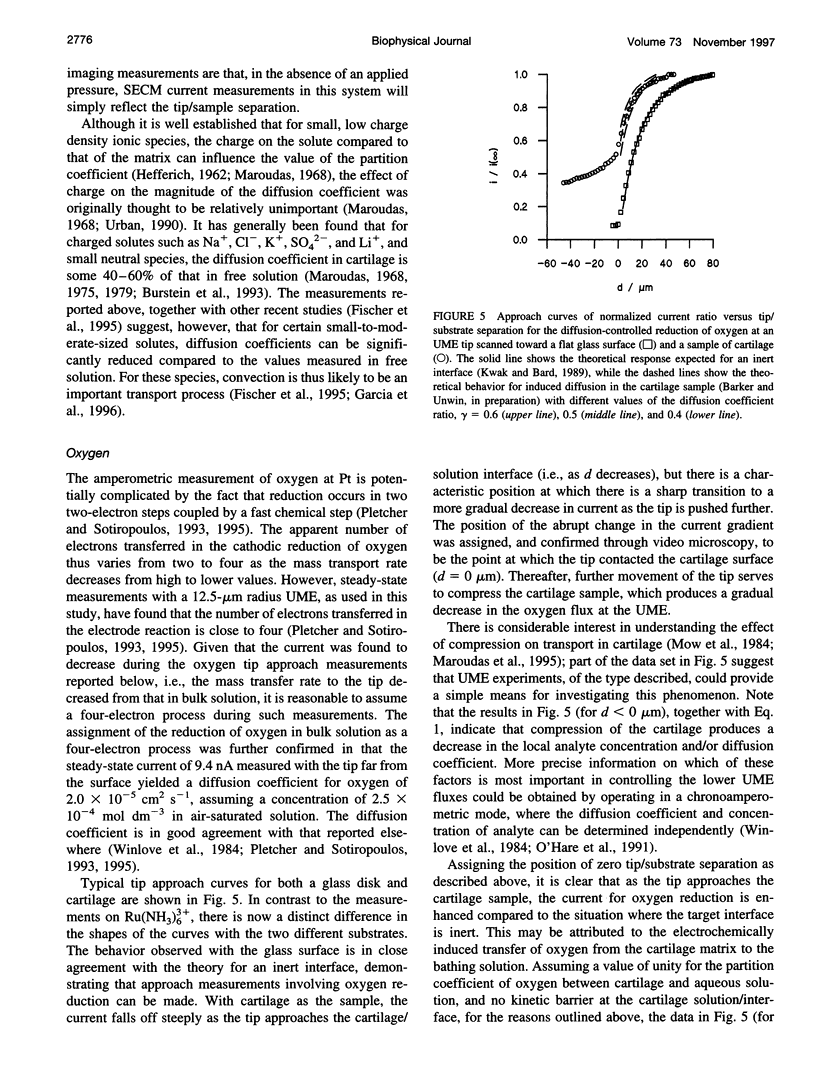
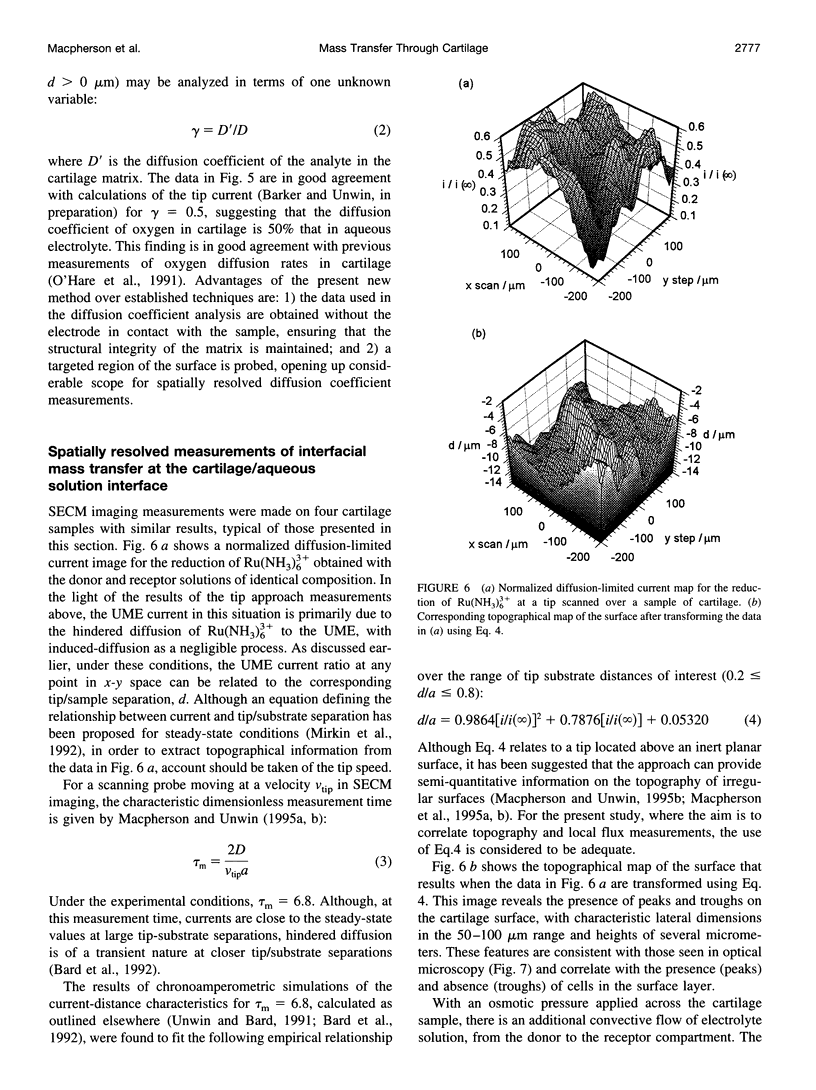
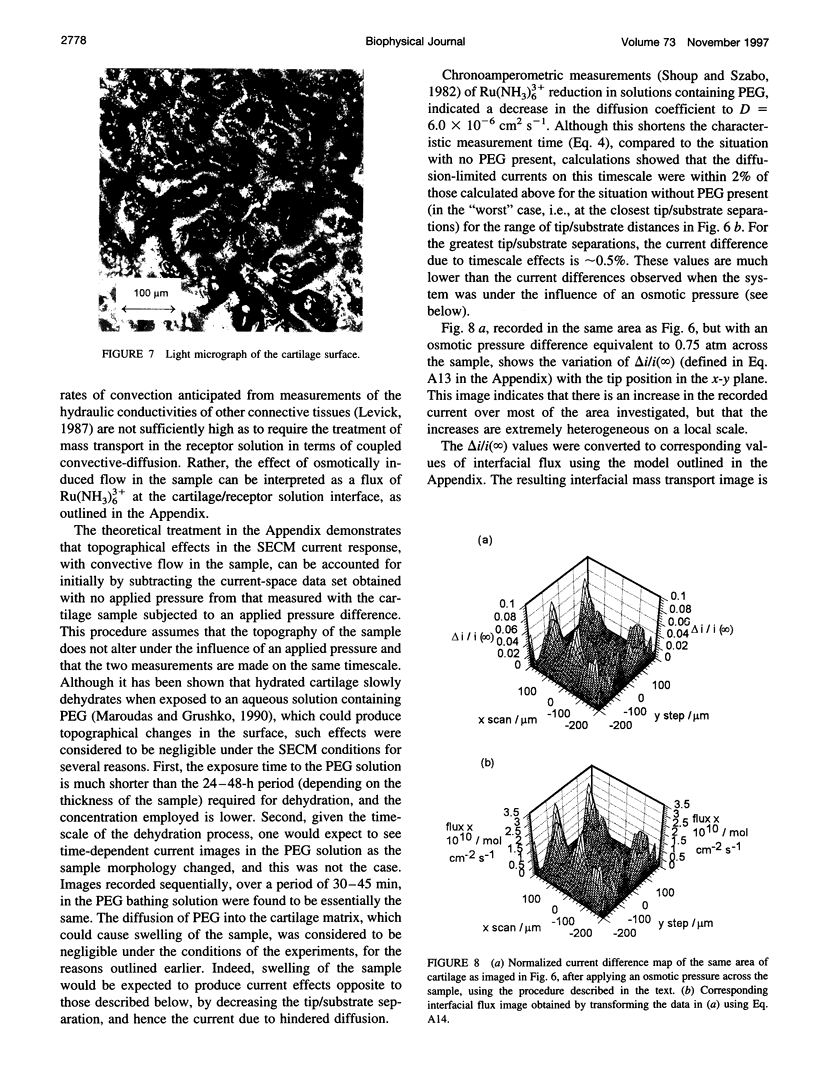
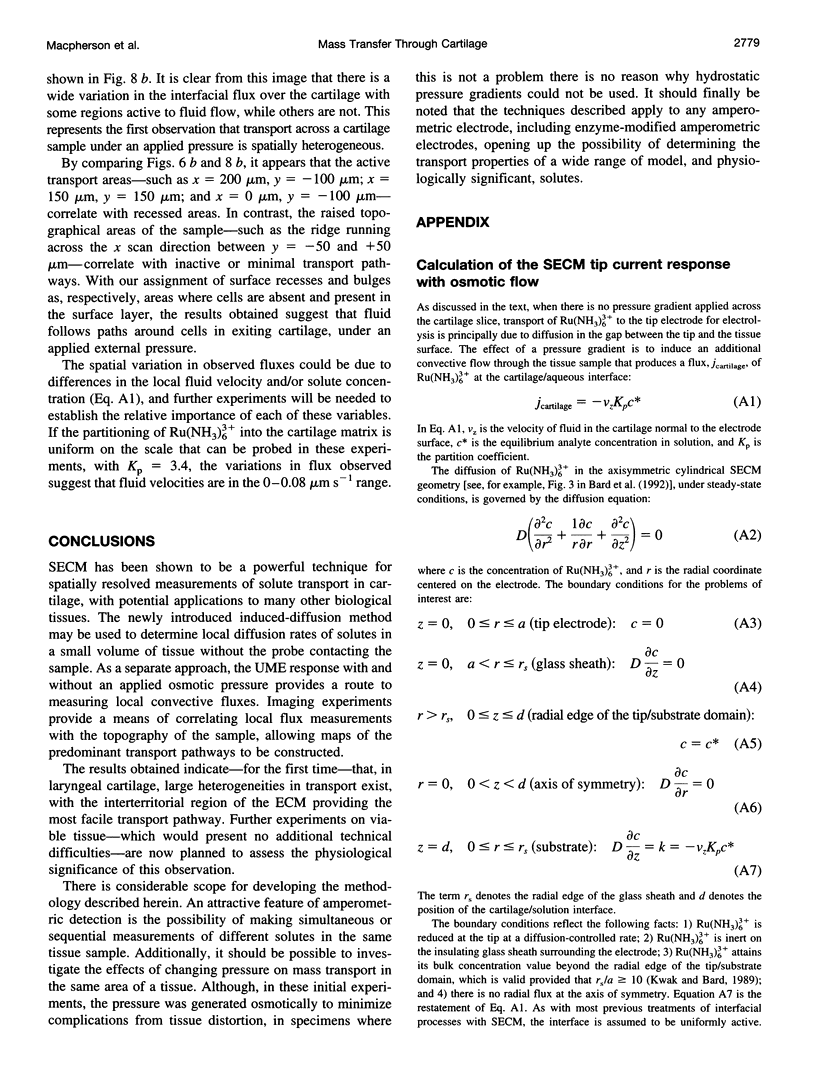
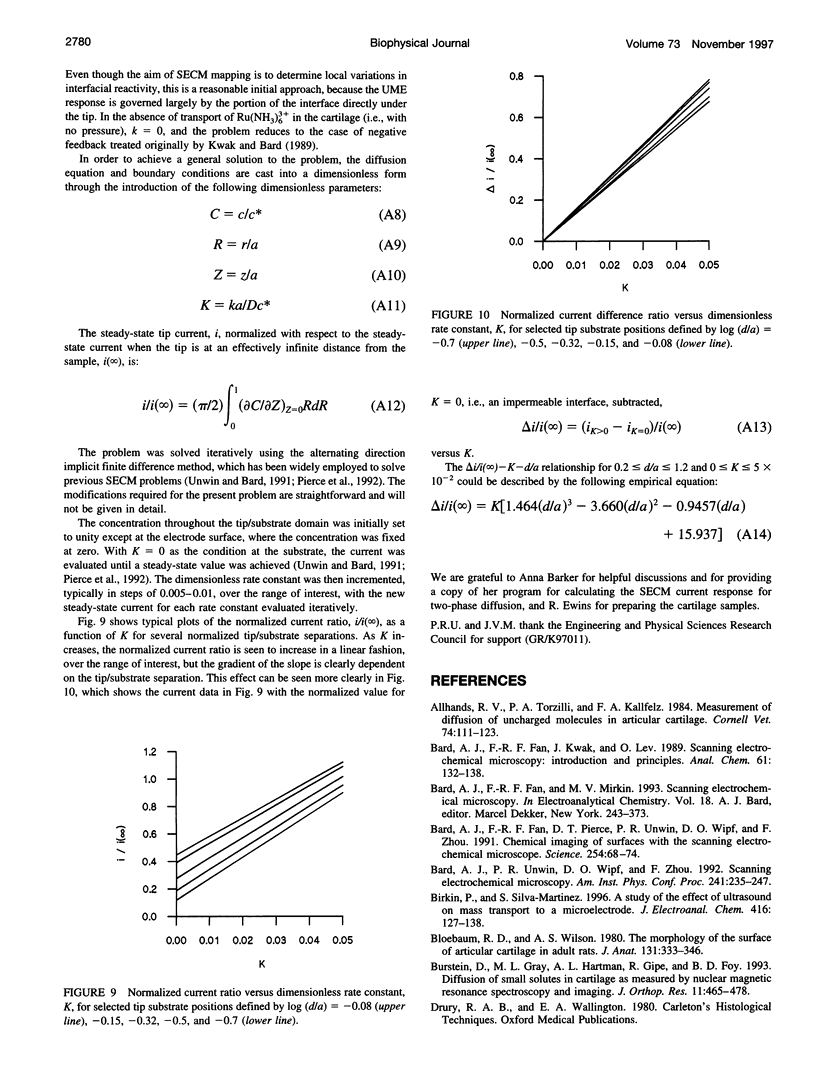
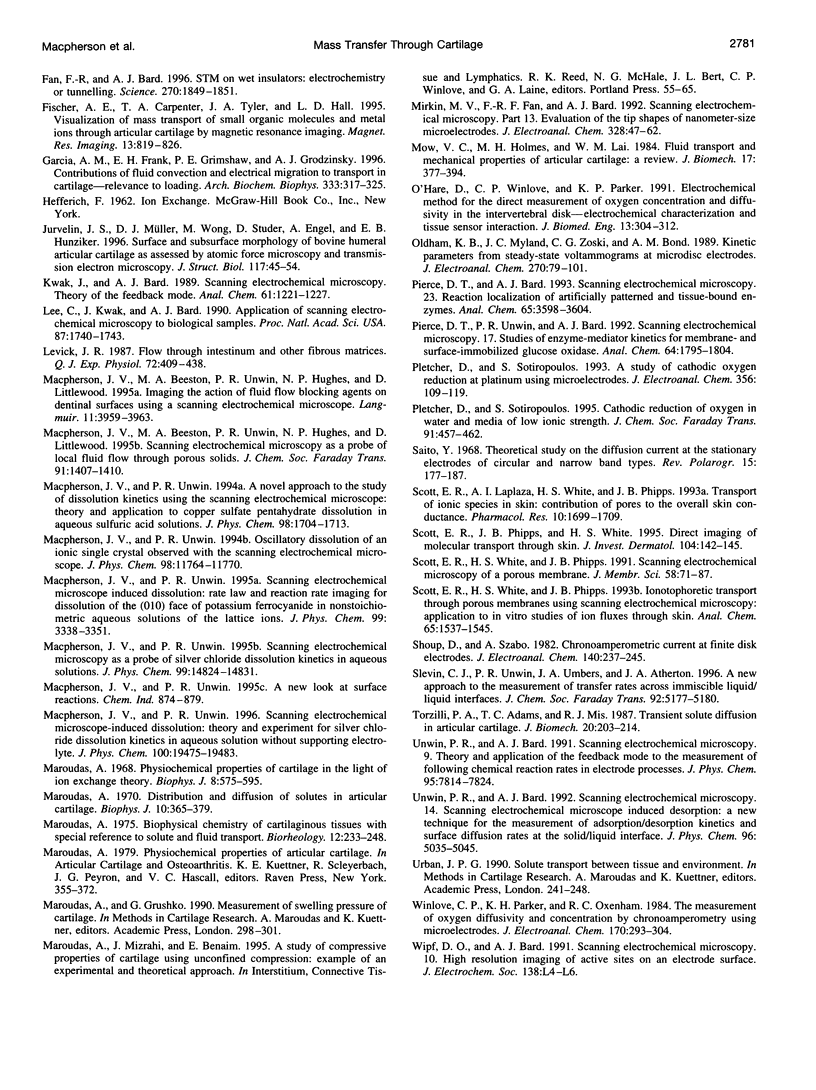
Images in this article
Selected References
These references are in PubMed. This may not be the complete list of references from this article.
- Allhands R. V., Torzilli P. A., Kallfelz F. A. Measurement of diffusion of uncharged molecules in articular cartilage. Cornell Vet. 1984 Apr;74(2):111–123. [PubMed] [Google Scholar]
- Bard A. J., Fan F. R., Pierce D. T., Unwin P. R., Wipf D. O., Zhou F. Chemical imaging of surfaces with the scanning electrochemical microscope. Science. 1991 Oct 4;254(5028):68–74. doi: 10.1126/science.254.5028.68. [DOI] [PubMed] [Google Scholar]
- Bloebaum R. D., Wilson A. S. The morphology of the surface of artcular cartilage in adult rats. J Anat. 1980 Sep;131(Pt 2):333–346. [PMC free article] [PubMed] [Google Scholar]
- Burstein D., Gray M. L., Hartman A. L., Gipe R., Foy B. D. Diffusion of small solutes in cartilage as measured by nuclear magnetic resonance (NMR) spectroscopy and imaging. J Orthop Res. 1993 Jul;11(4):465–478. doi: 10.1002/jor.1100110402. [DOI] [PubMed] [Google Scholar]
- Fan F. R., Bard A. J. STM on wet insulators: electrochemistry or tunneling? Science. 1995 Dec 15;270(5243):1849–1852. doi: 10.1126/science.270.5243.1849. [DOI] [PubMed] [Google Scholar]
- Fischer A. E., Carpenter T. A., Tyler J. A., Hall L. D. Visualisation of mass transport of small organic molecules and metal ions through articular cartilage by magnetic resonance imaging. Magn Reson Imaging. 1995;13(6):819–826. doi: 10.1016/0730-725x(95)00040-n. [DOI] [PubMed] [Google Scholar]
- Garcia A. M., Frank E. H., Grimshaw P. E., Grodzinsky A. J. Contributions of fluid convection and electrical migration to transport in cartilage: relevance to loading. Arch Biochem Biophys. 1996 Sep 15;333(2):317–325. doi: 10.1006/abbi.1996.0397. [DOI] [PubMed] [Google Scholar]
- Jurvelin J. S., Müller D. J., Wong M., Studer D., Engel A., Hunziker E. B. Surface and subsurface morphology of bovine humeral articular cartilage as assessed by atomic force and transmission electron microscopy. J Struct Biol. 1996 Jul-Aug;117(1):45–54. doi: 10.1006/jsbi.1996.0068. [DOI] [PubMed] [Google Scholar]
- Lee C., Kwak J., Bard A. J. Application of scanning electrochemical microscopy to biological samples. Proc Natl Acad Sci U S A. 1990 Mar;87(5):1740–1743. doi: 10.1073/pnas.87.5.1740. [DOI] [PMC free article] [PubMed] [Google Scholar]
- Levick J. R. Flow through interstitium and other fibrous matrices. Q J Exp Physiol. 1987 Oct;72(4):409–437. doi: 10.1113/expphysiol.1987.sp003085. [DOI] [PubMed] [Google Scholar]
- Maroudas A. Biophysical chemistry of cartilaginous tissues with special reference to solute and fluid transport. Biorheology. 1975 Jun;12(3-4):233–248. doi: 10.3233/bir-1975-123-416. [DOI] [PubMed] [Google Scholar]
- Maroudas A. Distribution and diffusion of solutes in articular cartilage. Biophys J. 1970 May;10(5):365–379. doi: 10.1016/S0006-3495(70)86307-X. [DOI] [PMC free article] [PubMed] [Google Scholar]
- Maroudas A. Physicochemical properties of cartilage in the light of ion exchange theory. Biophys J. 1968 May;8(5):575–595. doi: 10.1016/S0006-3495(68)86509-9. [DOI] [PMC free article] [PubMed] [Google Scholar]
- Mow V. C., Holmes M. H., Lai W. M. Fluid transport and mechanical properties of articular cartilage: a review. J Biomech. 1984;17(5):377–394. doi: 10.1016/0021-9290(84)90031-9. [DOI] [PubMed] [Google Scholar]
- O'Hare D., Winlove C. P., Parker K. H. Electrochemical method for direct measurement of oxygen concentration and diffusivity in the intervertebral disc: electrochemical characterization and tissue-sensor interactions. J Biomed Eng. 1991 Jul;13(4):304–312. doi: 10.1016/0141-5425(91)90112-k. [DOI] [PubMed] [Google Scholar]
- Pierce D. T., Bard A. J. Scanning electrochemical microscopy. 23. Reaction localization of artificially patterned and tissue-bound enzymes. Anal Chem. 1993 Dec 15;65(24):3598–3604. doi: 10.1021/ac00072a012. [DOI] [PubMed] [Google Scholar]
- Scott E. R., Laplaza A. I., White H. S., Phipps J. B. Transport of ionic species in skin: contribution of pores to the overall skin conductance. Pharm Res. 1993 Dec;10(12):1699–1709. doi: 10.1023/a:1018909811672. [DOI] [PubMed] [Google Scholar]
- Scott E. R., Phipps J. B., White H. S. Direct imaging of molecular transport through skin. J Invest Dermatol. 1995 Jan;104(1):142–145. doi: 10.1111/1523-1747.ep12613661. [DOI] [PubMed] [Google Scholar]
- Scott E. R., White H. S., Phipps J. B. Iontophoretic transport through porous membranes using scanning electrochemical microscopy: application to in vitro studies of ion fluxes through skin. Anal Chem. 1993 Jun 1;65(11):1537–1545. doi: 10.1021/ac00059a010. [DOI] [PubMed] [Google Scholar]
- Torzilli P. A., Adams T. C., Mis R. J. Transient solute diffusion in articular cartilage. J Biomech. 1987;20(2):203–214. doi: 10.1016/0021-9290(87)90311-3. [DOI] [PubMed] [Google Scholar]