Abstract
The decay of evanescent field intensity beyond a dielectric interface depends upon beam incident angle, enabling the 3-d distribution of fluorophores to be deduced from total internal reflection fluorescence microscopy (TIRFM) images obtained at multiple incident angles. Instrumentation was constructed for computer-automated multiple angle-TIRFM (MA-TIRFM) using a right angle F2 glass prism (n(r) 1.632) to create the dielectric interface. A laser beam (488 nm) was attenuated by an acoustooptic modulator and directed onto a specified spot on the prism surface. Beam incident angle was set using three microstepper motors controlling two rotatable mirrors and a rotatable optical flat. TIRFM images were acquired by a cooled CCD camera in approximately 0.5 degree steps for >15 incident angles starting from the critical angle. For cell studies, cells were grown directly on the glass prisms (without refractive index-matching fluid) and positioned in the optical path. Images of the samples were acquired at multiple angles, and corrected for angle-dependent evanescent field intensity using "reference" images acquired with a fluorophore solution replacing the sample. A theory was developed to compute fluorophore z-distribution by inverse Laplace transform of angle-resolved intensity functions. The theory included analysis of multiple layers of different refractive index for cell studies, and the anisotropic emission from fluorophores near a dielectric interface. Instrument performance was validated by mapping the thickness of a film of dihexyloxacarbocyanine in DMSO/water (n(r) 1.463) between the F2 glass prism and a plano-convex silica lens (458 mm radius, n(r) 1.463); the MA-TIRFM map accurately reproduced the lens spherical surface. MA-TIRFM was used to compare with nanometer z-resolution the geometry of cell-substrate contact for BCECF-labeled 3T3 fibroblasts versus MDCK epithelial cells. These studies establish MA-TIRFM for measurement of submicroscopic distances between fluorescent probes and cell membranes.
Full text
PDF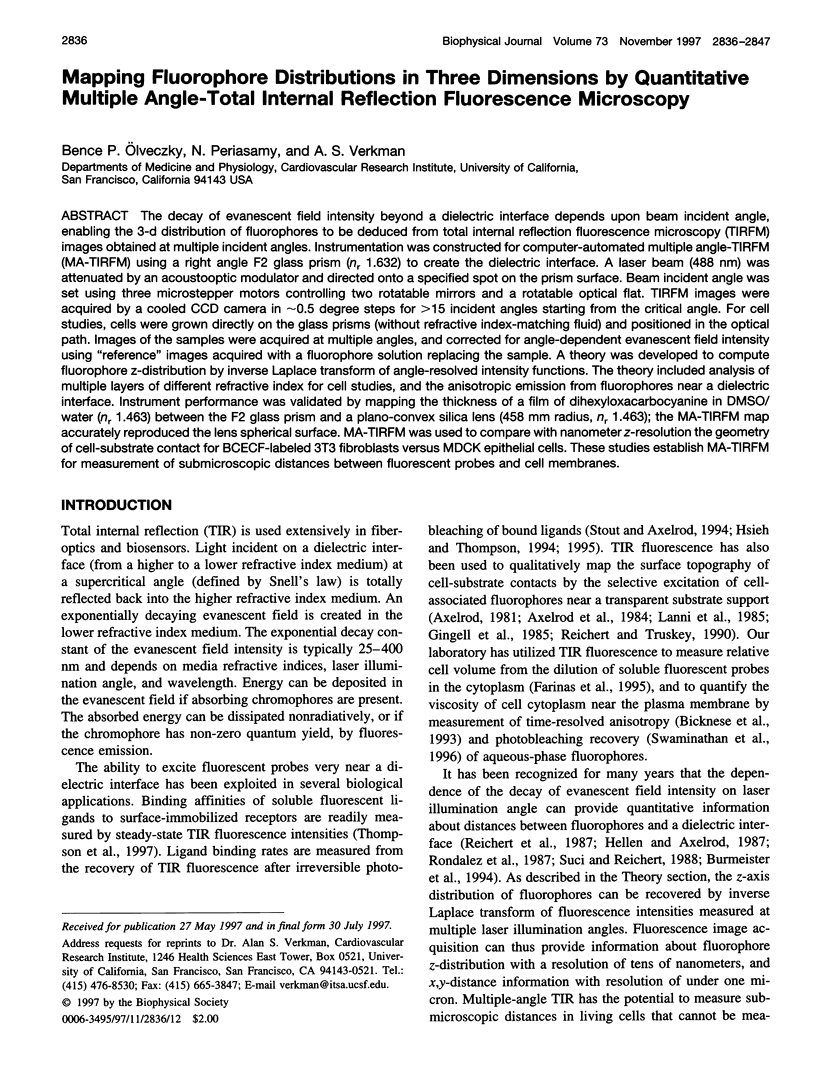
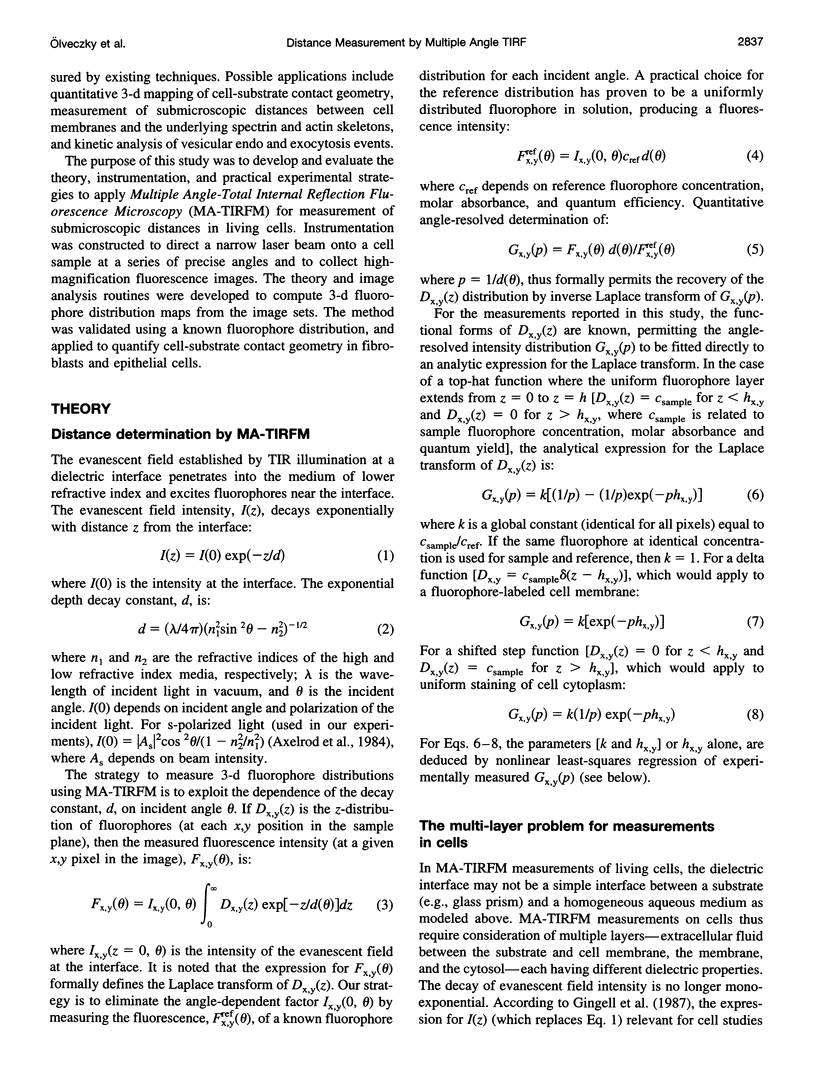
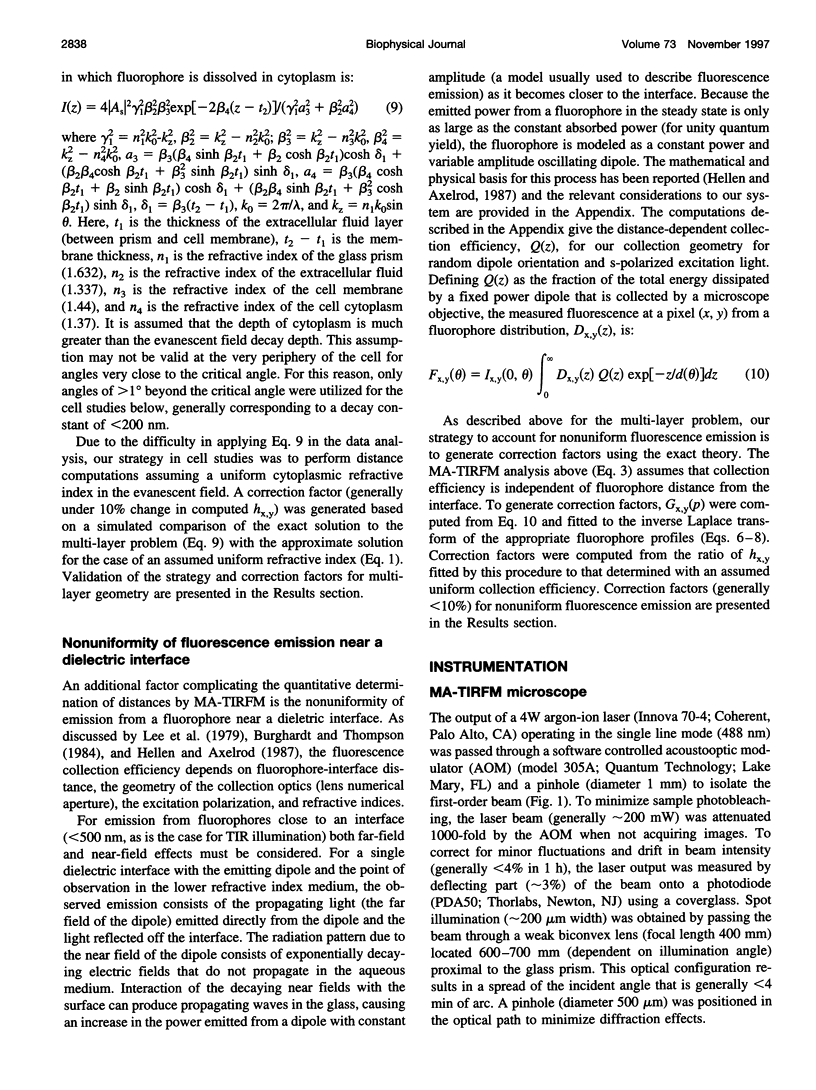
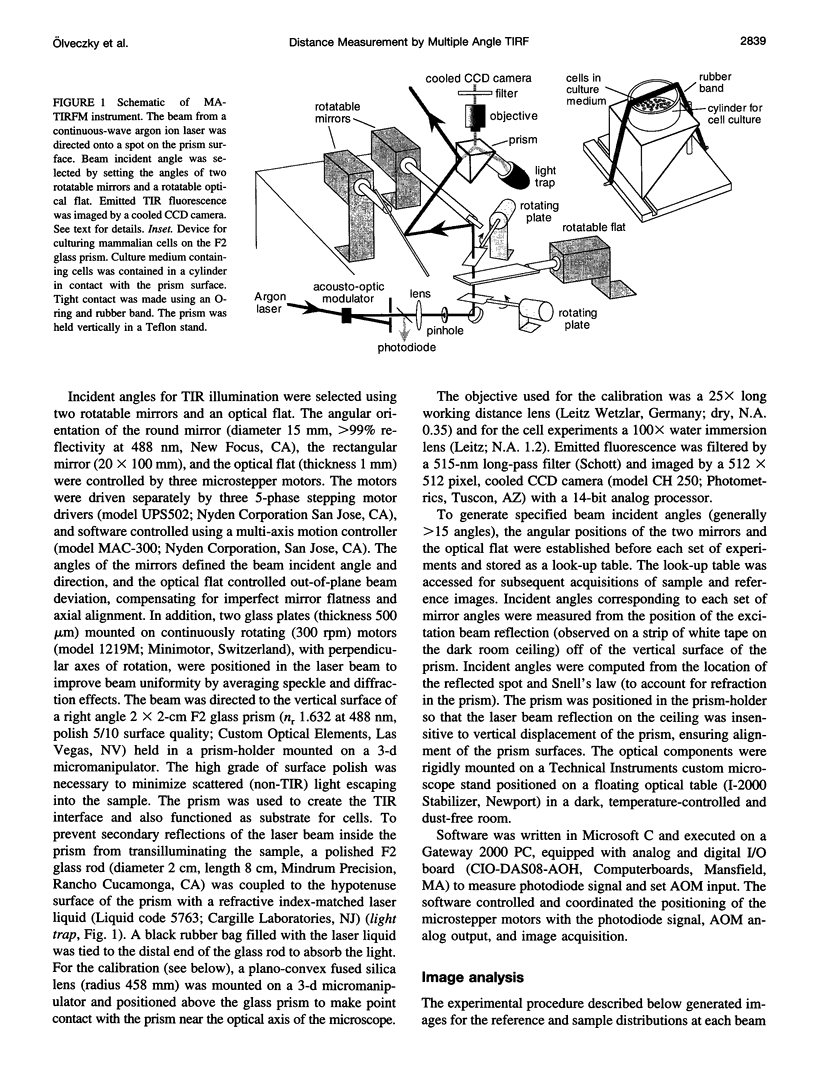
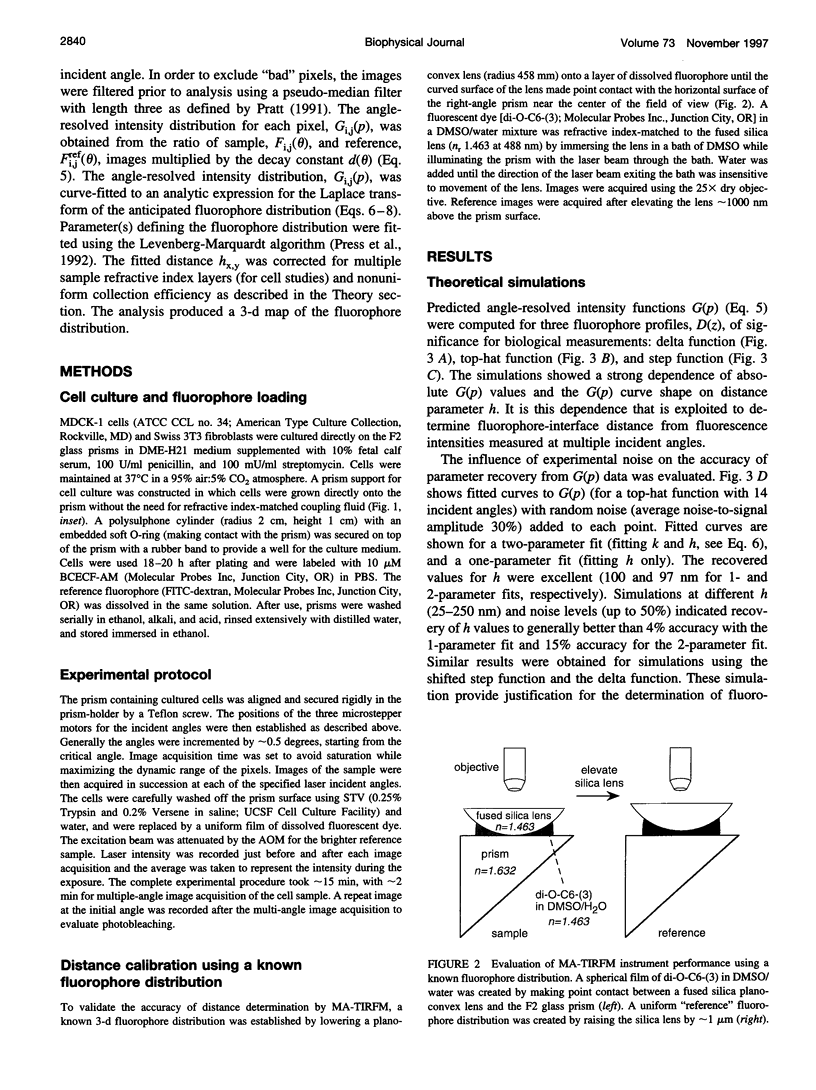
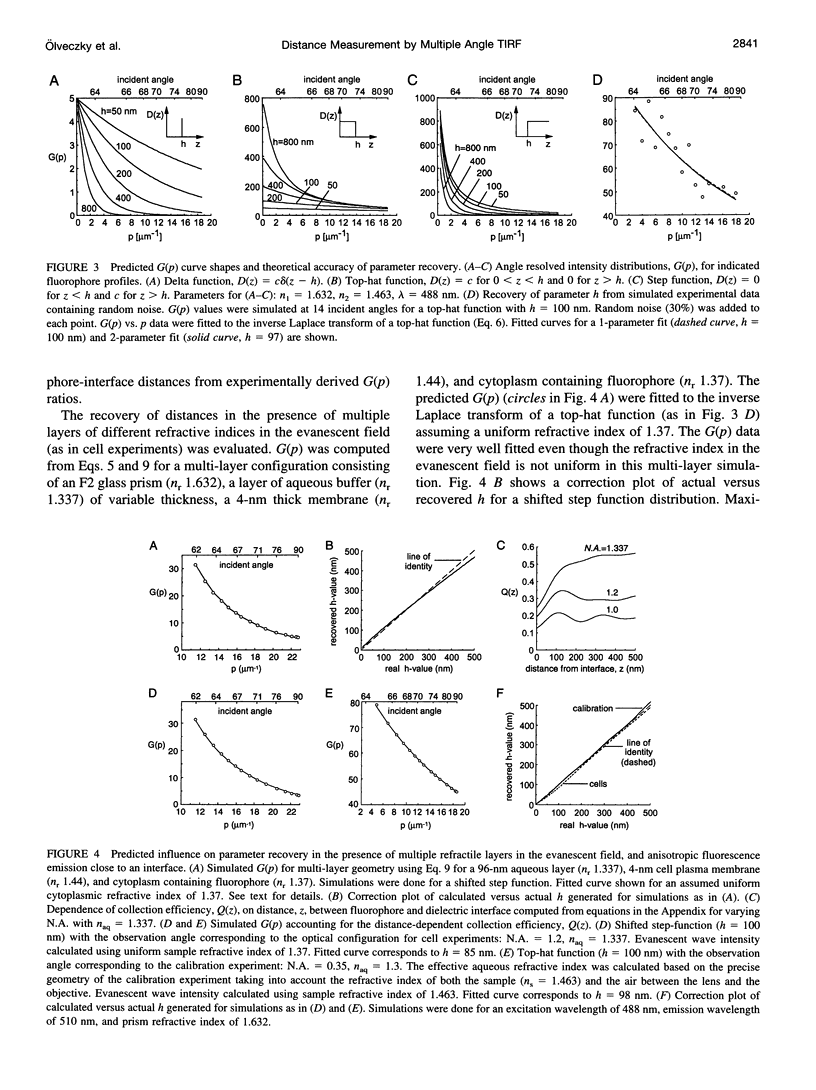
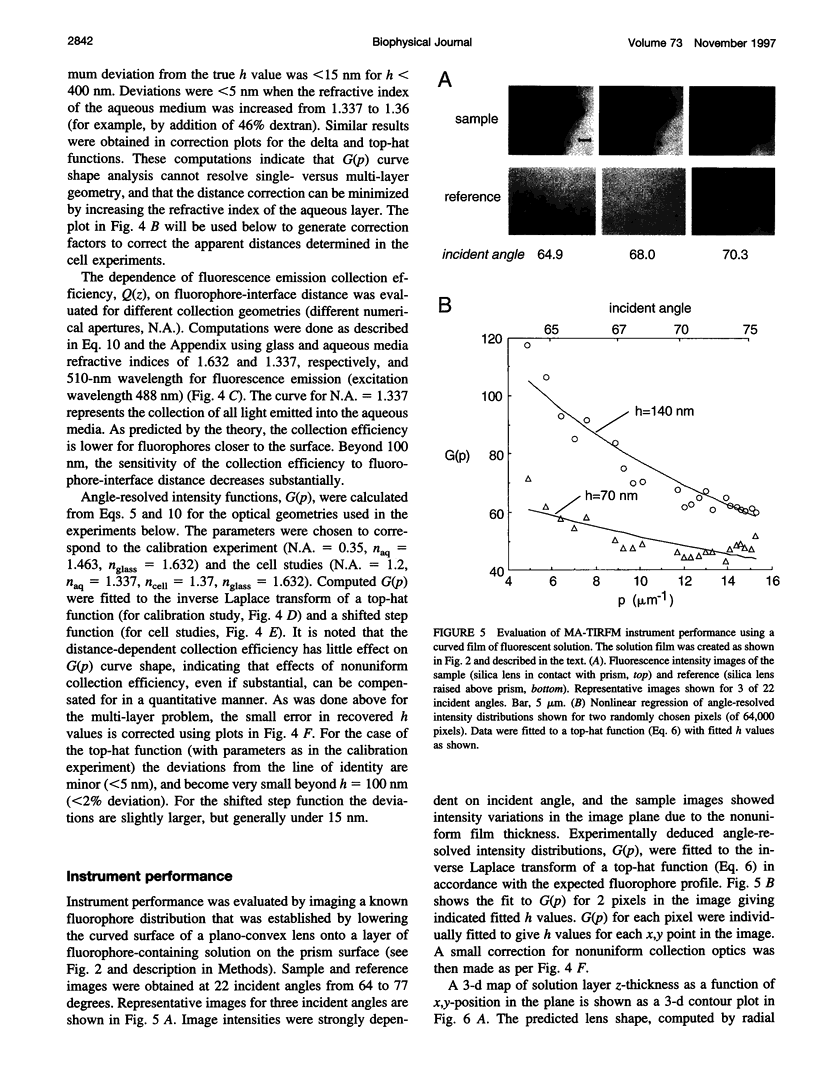
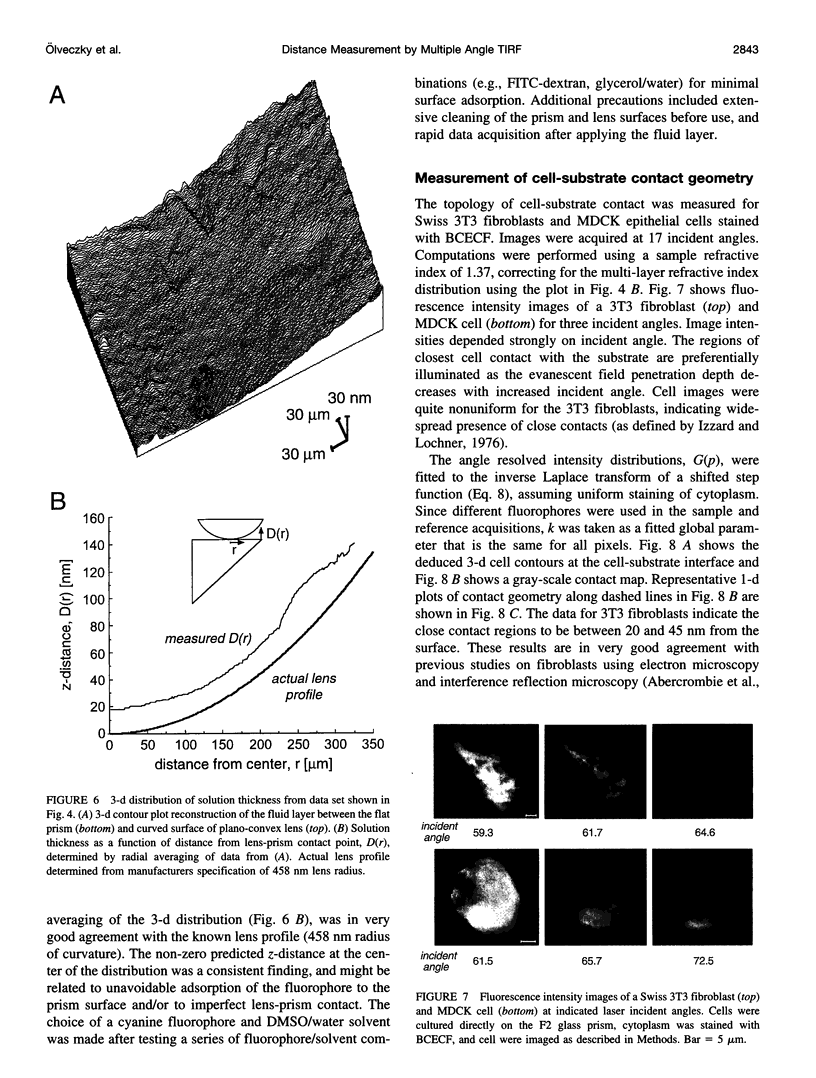
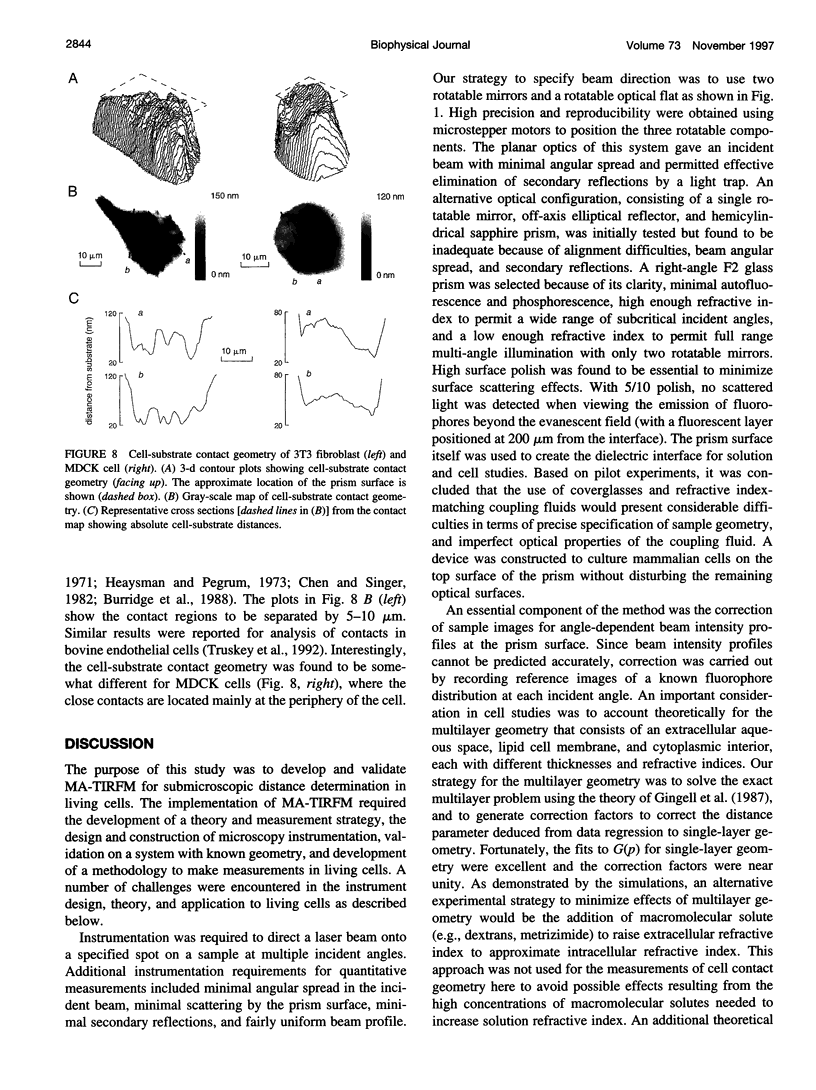
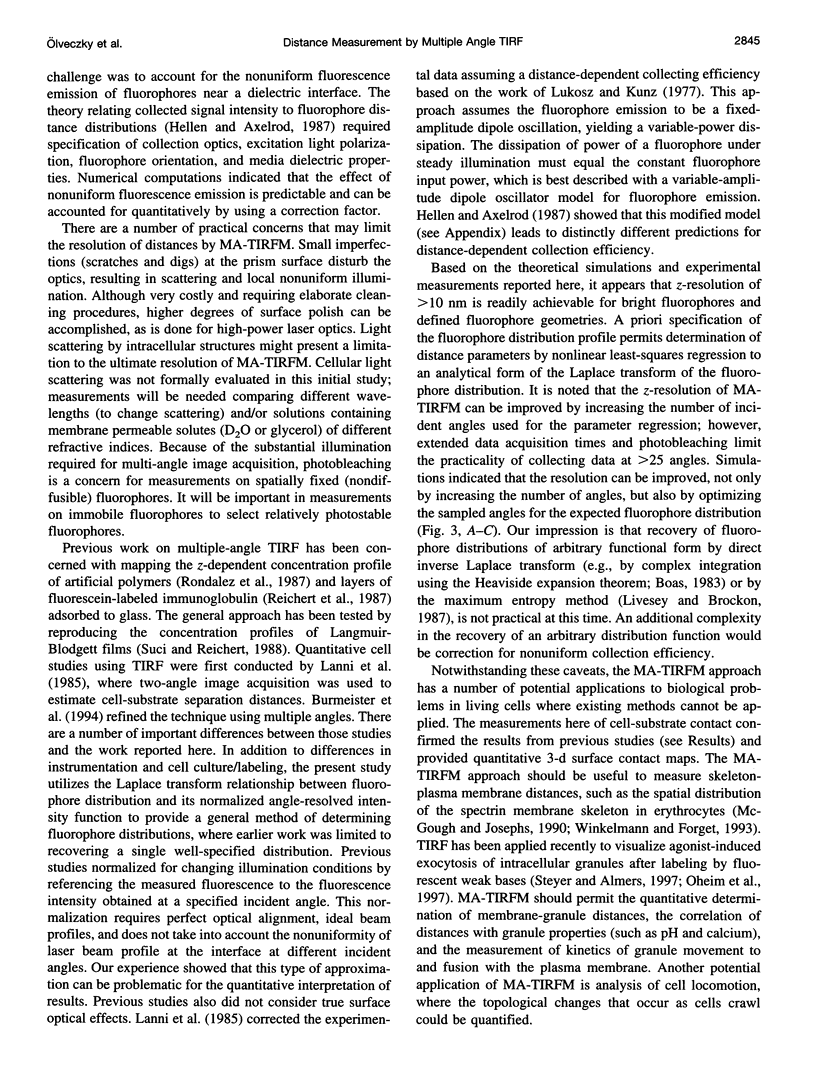
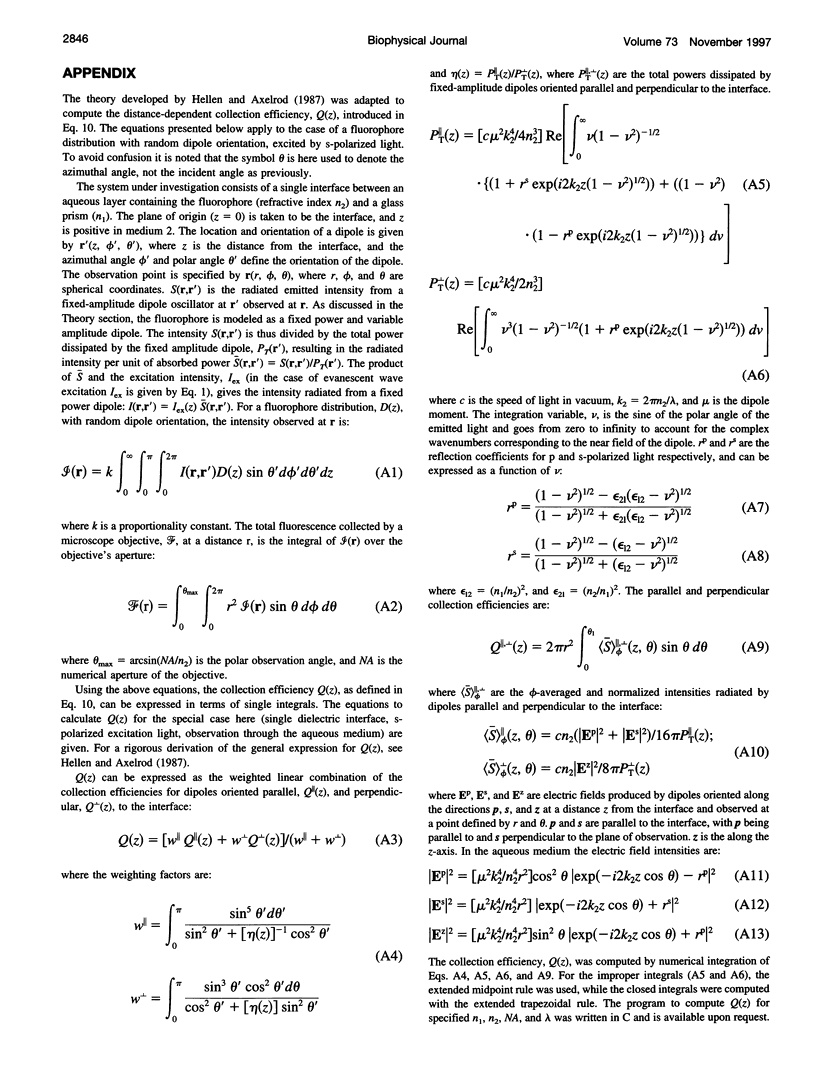
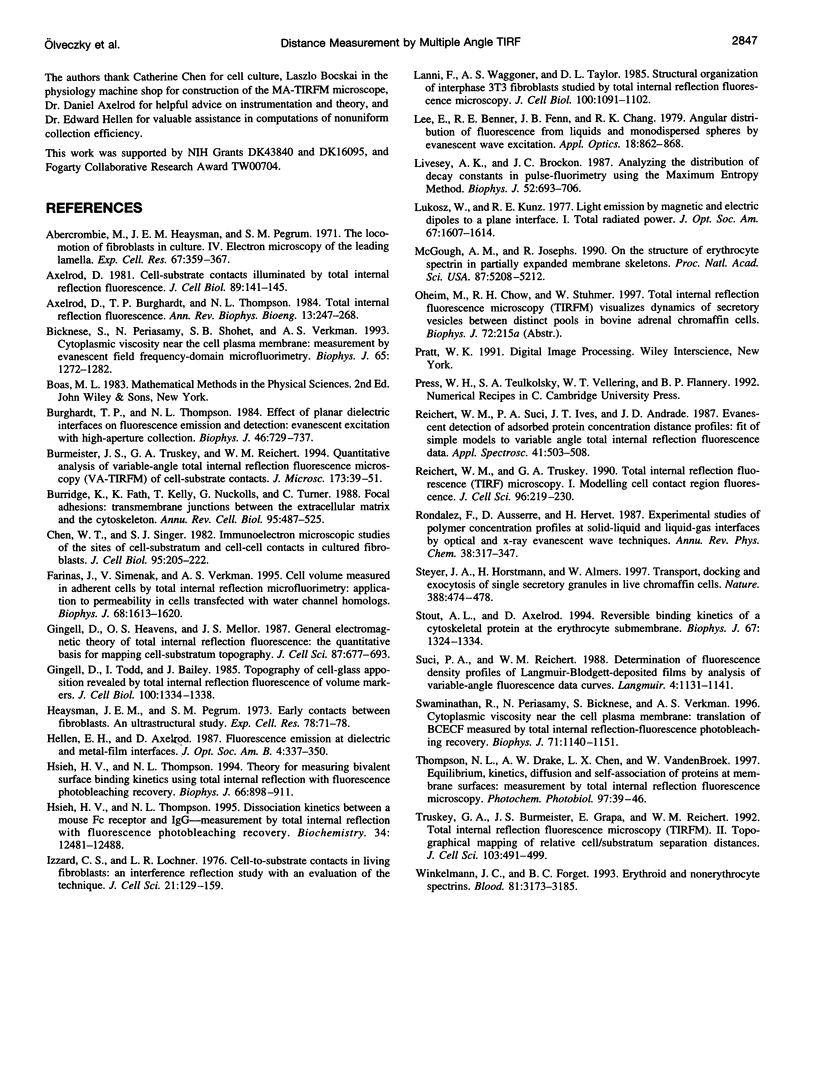
Images in this article
Selected References
These references are in PubMed. This may not be the complete list of references from this article.
- Abercrombie M., Heaysman J. E., Pegrum S. M. The locomotion of fibroblasts in culture. IV. Electron microscopy of the leading lamella. Exp Cell Res. 1971 Aug;67(2):359–367. doi: 10.1016/0014-4827(71)90420-4. [DOI] [PubMed] [Google Scholar]
- Axelrod D., Burghardt T. P., Thompson N. L. Total internal reflection fluorescence. Annu Rev Biophys Bioeng. 1984;13:247–268. doi: 10.1146/annurev.bb.13.060184.001335. [DOI] [PubMed] [Google Scholar]
- Axelrod D. Cell-substrate contacts illuminated by total internal reflection fluorescence. J Cell Biol. 1981 Apr;89(1):141–145. doi: 10.1083/jcb.89.1.141. [DOI] [PMC free article] [PubMed] [Google Scholar]
- Bicknese S., Periasamy N., Shohet S. B., Verkman A. S. Cytoplasmic viscosity near the cell plasma membrane: measurement by evanescent field frequency-domain microfluorimetry. Biophys J. 1993 Sep;65(3):1272–1282. doi: 10.1016/S0006-3495(93)81179-2. [DOI] [PMC free article] [PubMed] [Google Scholar]
- Burghardt T. P., Thompson N. L. Effect of planar dielectric interfaces on fluorescence emission and detection. Evanescent excitation with high-aperture collection. Biophys J. 1984 Dec;46(6):729–737. doi: 10.1016/S0006-3495(84)84071-0. [DOI] [PMC free article] [PubMed] [Google Scholar]
- Burmeister J. S., Truskey G. A., Reichert W. M. Quantitative analysis of variable-angle total internal reflection fluorescence microscopy (VA-TIRFM) of cell/substrate contacts. J Microsc. 1994 Jan;173(Pt 1):39–51. doi: 10.1111/j.1365-2818.1994.tb03426.x. [DOI] [PubMed] [Google Scholar]
- Burridge K., Fath K., Kelly T., Nuckolls G., Turner C. Focal adhesions: transmembrane junctions between the extracellular matrix and the cytoskeleton. Annu Rev Cell Biol. 1988;4:487–525. doi: 10.1146/annurev.cb.04.110188.002415. [DOI] [PubMed] [Google Scholar]
- Chen W. T., Singer S. J. Immunoelectron microscopic studies of the sites of cell-substratum and cell-cell contacts in cultured fibroblasts. J Cell Biol. 1982 Oct;95(1):205–222. doi: 10.1083/jcb.95.1.205. [DOI] [PMC free article] [PubMed] [Google Scholar]
- Farinas J., Simanek V., Verkman A. S. Cell volume measured by total internal reflection microfluorimetry: application to water and solute transport in cells transfected with water channel homologs. Biophys J. 1995 Apr;68(4):1613–1620. doi: 10.1016/S0006-3495(95)80335-8. [DOI] [PMC free article] [PubMed] [Google Scholar]
- Gingell D., Heavens O. S., Mellor J. S. General electromagnetic theory of total internal reflection fluorescence: the quantitative basis for mapping cell-substratum topography. J Cell Sci. 1987 Jun;87(Pt 5):677–693. doi: 10.1242/jcs.87.5.677. [DOI] [PubMed] [Google Scholar]
- Gingell D., Todd I., Bailey J. Topography of cell-glass apposition revealed by total internal reflection fluorescence of volume markers. J Cell Biol. 1985 Apr;100(4):1334–1338. doi: 10.1083/jcb.100.4.1334. [DOI] [PMC free article] [PubMed] [Google Scholar]
- Heaysman J. E., Pegrum S. M. Early contacts between fibroblasts. An ultrastructural study. Exp Cell Res. 1973 Mar 30;78(1):71–78. doi: 10.1016/0014-4827(73)90039-6. [DOI] [PubMed] [Google Scholar]
- Hsieh H. V., Thompson N. L. Dissociation kinetics between a mouse Fc receptor (Fc gamma RII) and IgG: measurement by total internal reflection with fluorescence photobleaching recovery. Biochemistry. 1995 Sep 26;34(38):12481–12488. doi: 10.1021/bi00038a047. [DOI] [PubMed] [Google Scholar]
- Hsieh H. V., Thompson N. L. Theory for measuring bivalent surface binding kinetics using total internal reflection with fluorescence photobleaching recovery. Biophys J. 1994 Mar;66(3 Pt 1):898–911. doi: 10.1016/s0006-3495(94)80866-5. [DOI] [PMC free article] [PubMed] [Google Scholar]
- Izzard C. S., Lochner L. R. Cell-to-substrate contacts in living fibroblasts: an interference reflexion study with an evaluation of the technique. J Cell Sci. 1976 Jun;21(1):129–159. doi: 10.1242/jcs.21.1.129. [DOI] [PubMed] [Google Scholar]
- Lanni F., Waggoner A. S., Taylor D. L. Structural organization of interphase 3T3 fibroblasts studied by total internal reflection fluorescence microscopy. J Cell Biol. 1985 Apr;100(4):1091–1102. doi: 10.1083/jcb.100.4.1091. [DOI] [PMC free article] [PubMed] [Google Scholar]
- Livesey A. K., Brochon J. C. Analyzing the distribution of decay constants in pulse-fluorimetry using the maximum entropy method. Biophys J. 1987 Nov;52(5):693–706. doi: 10.1016/S0006-3495(87)83264-2. [DOI] [PMC free article] [PubMed] [Google Scholar]
- McGough A. M., Josephs R. On the structure of erythrocyte spectrin in partially expanded membrane skeletons. Proc Natl Acad Sci U S A. 1990 Jul;87(13):5208–5212. doi: 10.1073/pnas.87.13.5208. [DOI] [PMC free article] [PubMed] [Google Scholar]
- Reichert W. M., Truskey G. A. Total internal reflection fluorescence (TIRF) microscopy. I. Modelling cell contact region fluorescence. J Cell Sci. 1990 Jun;96(Pt 2):219–230. doi: 10.1242/jcs.96.2.219. [DOI] [PubMed] [Google Scholar]
- Steyer J. A., Horstmann H., Almers W. Transport, docking and exocytosis of single secretory granules in live chromaffin cells. Nature. 1997 Jul 31;388(6641):474–478. doi: 10.1038/41329. [DOI] [PubMed] [Google Scholar]
- Stout A. L., Axelrod D. Reversible binding kinetics of a cytoskeletal protein at the erythrocyte submembrane. Biophys J. 1994 Sep;67(3):1324–1334. doi: 10.1016/S0006-3495(94)80604-6. [DOI] [PMC free article] [PubMed] [Google Scholar]
- Swaminathan R., Bicknese S., Periasamy N., Verkman A. S. Cytoplasmic viscosity near the cell plasma membrane: translational diffusion of a small fluorescent solute measured by total internal reflection-fluorescence photobleaching recovery. Biophys J. 1996 Aug;71(2):1140–1151. doi: 10.1016/S0006-3495(96)79316-5. [DOI] [PMC free article] [PubMed] [Google Scholar]
- Thompson N. L., Drake A. W., Chen L., Vanden Broek W. Equilibrium, kinetics, diffusion and self-association of proteins at membrane surfaces: measurement by total internal reflection fluorescence microscopy. Photochem Photobiol. 1997 Jan;65(1):39–46. doi: 10.1111/j.1751-1097.1997.tb01875.x. [DOI] [PubMed] [Google Scholar]
- Truskey G. A., Burmeister J. S., Grapa E., Reichert W. M. Total internal reflection fluorescence microscopy (TIRFM). II. Topographical mapping of relative cell/substratum separation distances. J Cell Sci. 1992 Oct;103(Pt 2):491–499. doi: 10.1242/jcs.103.2.491. [DOI] [PubMed] [Google Scholar]
- Winkelmann J. C., Forget B. G. Erythroid and nonerythroid spectrins. Blood. 1993 Jun 15;81(12):3173–3185. [PubMed] [Google Scholar]